Abstract
Background
Part of quercetin flows into the colon after escaping the absorption of the small intestine and will be degraded by colonic microbiota. The catabolites in the colon partially determine the physiological activity of quercetin.
Methods
Seven gut bacteria isolated from human feces were utilized to in vitro ferment quercetin. Their catabolites were analyzed with high-performance liquid chromatography and mass spectrometry, and the antioxidant activities of their fermented broths were compared with that of quercetin.
Results
One metabolite, 3,4-dihydroxyphenylacetic acid, was produced by both C. perfringens and B. fragilis transforming quercetin. No other metabolites were detected in the other fermented broths. The antioxidant activities of all strains fermenting quercetin reached the highest values at the concentration of 1 mg/mL quercetin in broth; the fermented products of C. perfringens and B. fragilis presented stronger activities than those of other strains at most concentrations of quercetin in broth. Additionally, all of the fermented broths presented a decline of the antioxidant activities compared to quercetin. Therefore, the antioxidant activity of quercetin will be lost when it reaches the human colon because of the gut bacterial fermentation.
Conclusions
This is the first study to report that quercetin can be degraded by C. perfringens and B. fragilis and transformed to the same metabolite, 3,4-dihydroxyphenylacetic acid, and that antioxidant activities decline when quercetin is fermented by seven gut bacteria.
Plant phenolic and polyphenolic compounds have the same antioxidant activity since their structures contain many phenolic hydroxyl groups. The flavonoids are phenolic compounds and therefore prone to oxidation to quinones, which makes flavonoids capable of protecting unsaturated fatty acids in membranes as well as ascorbate against oxidation. Hence, certain brackets of their physiological oxidation–reduction potentials can be estimated (Citation1).
Quercetin is also a flavonoid and often present as its glycoside, quercetin-3-O-rutinoside (rutin), in the human diet. After being ingested, rutin undergoes hydrolysis by the microflora in the colon before absorption (Citation2). The microflora in the colon has enormous catalytic and hydrolytic potential. They will first cut down the glycogen of rutin to utilize as their carbon source (Citation3) and can further catalyze the breakdown of the C6–C3–C6 flavonoid skeleton to a variety of phenolic acid catabolites (Citation2, Citation4) (Citation5). Thus, the bioactivity of polyphenol is very much dependent on its catabolism and catabolites that occur before absorption and disposition in target tissues and cells.
The antioxidant activity of quercetin or rutin and its metabolites has also been reported in many literatures (Citation3, Citation6–Citation8)). Serra et al. (Citation9) and Jaganath et al. (Citation3) deduced the metabolites and metabolic pathways of the colonic metabolism of quercetin in vitro fermentation model using rat colonic microflora. But it is different between human and rat colonic microflora; besides, the catabolites of quercetin cannot be totally separated since the techniques are limited. Yang et al. (Citation10) analyzed the metabolites of hyperoside (quercetin 3-galactoside) by human fecal flora in vitro. No literature was concerned about the catabolites of special gut bacteria fermenting quercetin (not its glucoside) and the changes of antioxidant activity induced by the fermenting process.
Hence, the objective of this study is to analyze the catabolites of seven human fecal bacteria degrading quercetin and to compare their antioxidant activities with quercetin.
Materials and methods
Bacteria and media
Seven strains – Escherichia coli, Streptococcus lutetiensis, Enterococcus gilvus, Clostridium perfringens, Bacteroides fragilis, Lactobacillus acidophilus, and Weissella confuse – were identified and isolated from human feces in our laboratory (Citation11). Two kinds of media, MRS and Brain Heart Infusion (BHI) broths, were purchased from Qingdao Hope Bio-technology Co. and utilized to culture these strains. MRS broth for L. acidophilus and W. confuse; BHI for E. coli, E. gilvus, S. lutetiensis, C. perfringens, and B. fragilis.
In vitro fermentation
Quercetin (Qiyun Biological Science & Technology Limited Co., China) was first dissolved with DMSO, and then 50 mg/mL stock solution was prepared after filtration with a 0.22-µm nylon microporous membrane. The solution was stored at 4°C before use. Before those strains were individually inoculated into their broth media, the quercetin solution was supplemented to a final concentration of 0.2 mg/mL in the broths. After inoculation, the BHI and MRS broths were cultured at 37°C in anaerobic conditions for 24 h.
Collection of metabolites
The above fermented solutions were individually centrifuged at 5,000 rpm for 20 min, and the supernatant solutions were further extracted with an equal volume of ethanol. The extracted solutions were then distilled under reduced pressure at 55°C to remove ethanol and were condensed to five-fold. The remains were filtrated with a 0.45-µm microporous membrane, and the mixture solution of catabolites (MSCs) were individually collected and stored at −20°C before being used.
HPLC and MS analysis
After MSCs were thawed in a 37°C water bath, 20 µL of sample was pipetted by automatic sampler and analyzed with high-performance liquid chromatography (HPLC). The compounds were separated on an analytical Welch Ultimate XB–C18 column (4.6×250 mm i.d., 5 µm; Welch, Shanghai, China) using a binary gradient delivered by a LC-20AT low-pressure gradient HPLC pump (SHIMADZU, Japan) with acetonitrile as solvent A and 0.1% v/v formic acid water solution as solvent B for the detection of quercetin and its catabolites: 0.01 min, 5% A; 10 min, 10% A; 50 min, 50% A; 65 min, 90% A; 70 min, 90% A; 73 min, 5% A. The flow rate was 0.6 mL/min. Data acquisition was carried out with the software Classic-VP Chromatography Workstation (SHIMADZU, Japan). A diode array detection SPD-M20A plus was applied over the wavelength range of 220–600 nm for peak detection. Compounds were identified by comparison of the UV spectra and the retention times. The following wavelengths were monitored for quantitative analysis: 360 nm for quercetin, 280 nm for metabolites (Citation12, Citation13).
The fermentation products separated by the HPLC were used for the further experiment. Those components at new peaks were collected and analyzed by mass spectrometry (MS) (Waters, Milford, MA, USA), and the chromatographic scans of selected multiple reaction monitoring ions (MRM) were used for further identification.
Comparison of free radical scavenging activities
Initially, the broths of each bacterium with 10, 20, 30, 40, and 50 mg/mL quercetin were individually fermented for 24 h. BHI and MRS broths with quercetins but without incubating bacteria were used as controls. Then MSCs were prepared following the procedure described above.
The DPPH radical scavenging activities of all the samples of the fermentations were evaluated by the method of Blois (Citation14) with minor modifications. Twenty microliter of each MSC was added into 10 mL colorimetric tubes, made up with 2 mL of distilled water, and then mixed with 2 mL of 0.2 mM DPPH dissolved in methanol. The reaction mixture was incubated for 30 min at 28°C in the dark. The blank sample contained all reagents without MSC. The DPPH radical scavenging activity was determined by measuring the absorbance at 517 nm using a spectrophotometer. The DPPH free radical scavenging activity (%) was calculated using the following formula (Citation1). The test was paralleled three times.1
where A0 is the absorbance of the control and A1 is the absorbance in the presence of MSCs.
Hydroxyl radical, generated from the Fe3 +–ascorbate–H2O2 (Fenton reaction), was evaluated by degradation of deoxyribose that produced thiobarbituric acid reactive species (TBARS) (Citation15). The reaction mixture contained 25 mmol/L deoxyribose, 10 mmol/L ferric chloride, 100 mmol/L ascorbic acid, and 2.8 mmol/L H2O2 in 10 mmol/L KH2PO4 (pH 7.4), and it was then mixed with of 0.4 mL water solutions, including 20 µL of each MSC. The blank sample contained all reagents without MSC. The reaction mixture was incubated at 37°C for 1 h. Subsequently, 1 mL of 1% thiobarbituric acid and 1 mL of 3% trichloroacetic acid were added, and the mixture was heated at 100°C for 20 min. TBARS was measured spectrophotometrically by taking absorbance at 532 nm. The results were expressed as percentage inhibition of deoxyribose oxidation, as determined by the following formula (Citation2). The test was paralleled three times.2
where A is the malondialdehyde produced by Fenton reaction treated alone, and B is the malondialdehyde produced in the presence of MSCs.
Statistical analyses
Each sample was analyzed in triplicate, and data are presented as mean values±standard error (n=3). Multivariate correlation analysis of appropriate data was performed according to Pearson's correlation.
Results
Metabolites of various bacteria fermenting quercetin by HPLC and MS analysis
It is difficult to separate all the metabolites of bacteria by HPLC because of numerous substances in fermented broths. In this experiment, the metabolites of seven strains transforming quercetin were separated with HPLC. Only one compound was discovered, and it presented among the metabolites of both C. perfringens and B. fragilis at the retention time of 25.024 min in the charts (A and B). The retention time of quercetin was about 49.013 min. Its peak appeared in the fermented solution of E. gilvus, E. coli, S. lutetiensis, W. confuse, and L. acidophilus, but not of C. perfringens and B. fragilis.
Fig. 1 (a) Catabolites of C. perfringens fermenting quercetin, with HPLC analysis. S1 and S2 individually refer to the control and sample. (b) Catabolites of B. fragilis fermenting quercetin, with HPLC analysis. S1 and S2 individually refer to the control and sample.
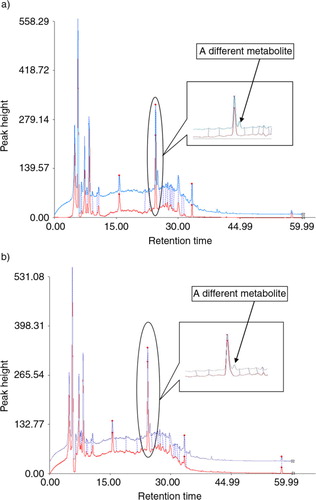
The component at the retention time of 25.024 min was collected for MS. Two significant molecular ion peaks of 167 and 123 m/z were observed () and deduced as 3,4-dihydroxyphenylacetic acid, one main metabolite of gut bacterial fermenting quercetin, according to its possible fractures (Citation16).
DPPH free radical scavenging activities of quercetin and its MSCs
The quercetin in broths removed DPPH free radicals depending on its concentration (). About 70% DPPH was removed by 1 mg/mL quercetin. Compared to quercetin, MSCs of seven bacterial species scavenged less DPPH free radicals (). Among MSCs of all strains, that of L. acidophilus can remove the least amount of DPPH free radicals in a series of doses of quercetin added in broths. The MSCs of W. confuse and E. gilvus, scavenged more DPPH free radicals than that of L. acidophilus, but less than the others when 0.4 mg/mL quercetin or more was added in broths. The MSCs of the other two strains, that is, W. confuse and E. gilvus, scavenged more DPPH free radicals than that of L. acidophilus but less than the others when more than 0.4 mg/mL quercetin was added in broths. Their removal rates of DPPH by MSCs reached the highest value when the concentration of quercetin was 1 mg/mL, the highest concentration of quercetin in broth. The abilities of scavenging free radicals were improved along with more quercetin added in broths.
Fig. 3 The removal rate of DPPH free radicals by quercetin and its MSCs from seven bacterial species in broths. The quercetin in BHI and MRS individually refers to the removal rate of DPPH free radicals by BHI and MRS media supplemented with quercetin and incubated for 24 h but without being inoculated with the bacteria.
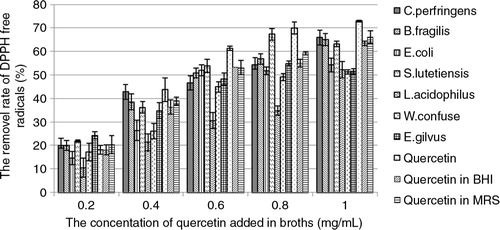
OH free radical scavenging activities of quercetin and its MSCs
The hydroxyl group free radical scavenging activity of quercetin was also related to its concentration (). The removal rate of OH free radicals reached almost 100% when the concentration of quercetin was 1 mg/mL. The MSCs of B. fragilis presented the strongest activity in scavenging OH free radicals in all concentrations of quercetin added in broths. When 1 mg/mL quercetin was added in both, the MSCs of B. fragilis almost removed the same amount of OH free radicals with quercetin (100%) and 80% in the case of C. perfringens. The OH free radicals scavenging activities of MSCs from S. lutetiensis and B. gilvus presented the weakest with only about 30% DPPH removed when more than 0.6 mg/mL quercetin was added in broth. The activities of all strains increased along with more quercetin being added.
Fig. 4 The removal rate of OH free radicals by quercetin and its MSCs from seven bacterial species. The quercetin in BHI and MRS individually refers to the removal rate of OH free radicals by BHI and MRS media supplemented with quercetin and incubated for 24 h but without being inoculated with the bacteria.
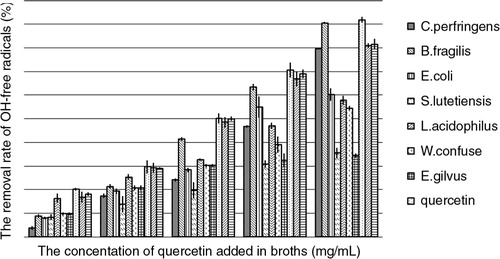
Discussion
This study attempted to analyze the metabolites of seven gut bacterial strains fermenting quercetin. 3,4-Dihydroxyphenylacetic acid, a metabolite that was produced by both C. perfringens and B. fragilis transforming quercetin, was separated and identified with HPLC and MS. No other metabolites were detected in all fermented broths of seven strains. Since the degradation of quercetin was detected in all seven strains (Citation11), the antioxidant activities of seven fermented broths were then compared. Their fermented broths presented different antioxidant activities when their ability of scavenging DPPH and OH free radicals was tested. The results showed that their activities were almost dependent on the concentration of quercetin and reached the highest value when 1 mg/mL was added. Furthermore, the samples of C. perfringens and B. fragilis presented stronger ability in scavenging both DPPH and OH free radicals.
Serra et al. (Citation9) used rat colonic microflora to in vitro ferment quercetin and other flavonoids. They detected the metabolites of quercetin including 3-(3,4-dihydroxyphenyl) propionic acid, 3,4-dihydroxyphenylacetic acid, 3-hydroxyphenylacetic acid, and phenylacetic acid and deduced the metabolic pathway of quercetin in rat gut (). Only 3,4-dihydroxyphenylacetic acid was detected in the fermented broths of C. perfringens and B. fragilis, and no metabolites were found in the other broths, which indicated that only C. perfringens and B. fragilis from the human gut could transform quercetin to 3,4-dihydroxyphenylacetic acid, and the other five bacteria from the human gut could degrade part of quercetin (Citation11) but without 3,4-dihydroxyphenylacetic acid or other metabolites detected. Walle et al. (Citation16) reported that as much as 23.0–81.1% of the quercetin dose was recovered as CO2 in the expired air from volunteers. Hence, part of quercetin might be directly degraded into CO2 by the other five bacteria, which might be the reason that no metabolites were detected in the other five broths. Nonetheless, the results indicated that different bacteria can transform quercetin into the same metabolite(s), and quercetin can be metabolized by different bacteria to different metabolites.
Fig. 5 Proposed metabolic pathway of quercetin by rat colonic microflora. The totally squared metabolites are not detected. From Serra et al. (Citation9).
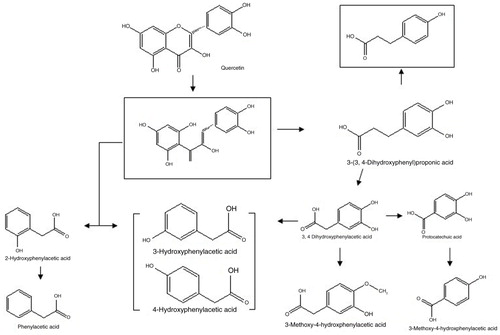
The phenomenon that metabolic conversion alters the antioxidant activity of quercetin had been reported in many literatures (Citation3, Citation8) (Citation17–Citation20)). However, most of these literatures focused on evaluating the antioxidant activities of quercetin and its conjugates such as 3’-O-methyl quercetin and 4’-O-methyl quercetin (Citation18, Citation20), quercetin-3-O-glucuronide, and quercetin-3-O-sulfate (Citation8, Citation19), quercetin 3-O-β-d-glucuronide (Citation17). The conjugates are not the products of gut microbiota degrading quercetin. Only Jaganath et al. (Citation3) investigated the breakdown of quercetin-3-O-rutinoside (rutin) by colonic microflora and deduced that its catabolites may play a key role in the overall antioxidant capacity of the colonic lumen after the ingestion of quercetin-rich foods. This paper showed that the metabolites of all strains had a lower DPPH and OH free radicals scavenging ability than that of quercetin at almost all doses of quercetin. But the MSCs of C. perfringens and B. fragilis showed an approximate activity with about 1 mg/mL quercetin added in broth. However, C. perfringens and B. fragilis are not the major bacteria in the human gut (Citation21–(Citation23)). Therefore, the most antioxidant activity of quercetin will lose a lot when it reaches the human colon.
Even though the metabolites of seven gut bacteria fermenting quercetin were not fully detected (Citation11), this will not affect the significance of the results presented about antioxidant activities of the fermented broths by seven strains since the mixed system (fermented broth) was complicated and similar to the contents of the human colon in some ways.
It is the first study to report that C. perfringens and B. fragilis can degrade quercetin into the same metabolite, 3,4-dihydroxyphenylacetic acid, and that antioxidant activities declined when quercetin was fermented by seven gut bacteria.
Conflict of interest and funding
The program was supported by the funds of the National Natural Science Funds (No. 31271849), the Open Project Program of State Key Laboratory of Food Science and Technology of Nanchang University (No. SKLF-KF-201212), National Innovation Experiment Program for University Students (No. 101055902), the Fundamental Research Funds for the Central Universities (No. 21611414).
Acknowledgements
We thank Zhen Zhang from the Department of Food Science and Engineering and technicians from Analytical and Testing Center, Jinan University for their contribution to the experiments of this study.
References
- Havsteen BH. The biochemistry and medical significance of the flavonoids. Pharmacol Ther. 2002; 96: 167–202.
- Crozier A, Jaganath IB, Clifford MN. Dietary phenolics: chemistry, bioavailability and effects on health. Nat Prod Rep. 2009; 26: 1001–43.
- Jaganath B, Mullen W, Lean MEJ, Edwards CA, Crozier A. In vitro catabolism of rutin by human fecal bacteria and the antioxidant capacity of its catabolites. Free Radic Biol Med. 2009; 47: 1180–9.
- Spencer JPE. Metabolism of tea flavonoids in the gastrointestinal tract. J Nutr. 2003; 133: 3255–61.
- Halliwell B, Rafter J, Jenner A. Health promotion by flavonoids, tocopherols, tocotrienols, and other phenols: direct or indirect effects? Antioxidants or not?. Am J Clin Nutr. 2005; 81: 268–76.
- Gordon MH, Roedig-Penman A. Antioxidant activity of quercetinand myricetin in liposomes. Chem Phys Lipids. 1988; 97: 79–85.
- Ameho CK, Chen CYO, Smith D, Sánchez-Moreno C, Milbury PE, Blumberg JB. Antioxidant activity and metabolite profile of quercetin in vitamin-E-depleted rats. J Nutr Biochem. 2008; 19: 467–74.
- Lotito SB, Zhang W, Yang CS, Crozier A, Frei B. Metabolic conversion of dietary flavonoids alters their anti-inflammatory and antioxidant properties. Free Radical Biol Med. 2011; 51: 454–63.
- Serra A, Macia A, Romero M, Reguant J, Ortega N, Motilva M. Metabolic pathways of the colonic metabolism of flavonoids (flavonols, flavones and flavanones) and phenolic acids. Food Chem. 2012; 130: 383–93.
- Yang J, Qian D, Guo J, Jiang S, Shang E, Duan J, Xu Jun. Identification of the major metabolites of hyperoside produced by the human intestinal bacteria using the ultra performance liquid chromatography/quadrupole-time-of-flight mass spectrometry. J Ethnopharmacol. 2013; 147: 174–9.
- Zhang Z, Peng X, Li S, Zhang N, Wang Y, Wei H. Isolation and identification of quercetin degrading bacteria from human fecal microbes. PLOS one. 2014; 9: 1–4.
- Schneider H, Simmering R, Hartmann L, Pforte H, Blaut M. Degradation of quercetin-3-glucoside in gnotobiotic rats associated with human intestinal bacteria. J Appl Microbiol. 2000; 89: 1027–37.
- Keppler K, Hein EM, Humpf HU. Metabolism of quercetin and rutin by the pig caecal microflora prepared by freeze-preservation. Mol Nutr Food Res. 2006; 50: 686–95.
- Blois MS. Antioxidants determination by the use of a stable free radical. Nature. 1958; 181: 1199–200.
- Halliwell B, Gutteridge JM, Aruoma OI. The deoxyribose method: a simple “test tube” assay for determination of rate constants for reactions of hydroxyl radicals. Anal Biochem. 1987; 165: 215–19.
- Walle TU, Walle K, Halushka PV. Carbon dioxide is the major metabolite of quercetin in humans. J Nutr. 2001; 131: 2648–52. [PubMed Abstract] [PubMed Abstract].
- Shirai M, Moon J, Tsushida T, Terao J. Inhibitory effect of a quercetin metabolite, quercetin 3-O-β-D-glucuronide, on lipid peroxidation in liposomal membranes. J Agric Food Chem. 2001; 49: 5602–8.
- Angeloni C, Spencer JPE, Leoncini E, Biagi PL, Hrelia S. Role of quercetin and its in vivo metabolites in protecting H9c2 cells against oxidative stress. Biochimie. 2007; 89: 73–82.
- Chao C, Hou Y, Chao PL, Weng C, Ho F. The antioxidant effects of quercetin metabolites on the prevention of high glucose-induced apoptosis of human umbilical vein endothelial cells. Br J Nutr. 2009; 101: 1165–70.
- Dueñas M, González-Manzano S, González-Paramás A, Santos-Buelga C. Antioxidant evaluation of O-methylated metabolites of catechin, epicatechin and quercetin. J Pharm Biomed Anal. 2010; 51: 443–9.
- Wexler HM. Bacteroides: the good, the bad, and the nitty-gritty. Clin Microbiol Rev. 2007; 20: 593–621.
- Tannock GW. The bowel microbiota and inflammatory bowel diseases. Int J Inflamm. 2011; 34: 147–54.
- Tannock GW, Lawley B, Munro Lay KC, Taylor C, Daynes C, Baladjay L, etal. Comprehensive analysis of the bacterial content of stool from patients with chronic pouchitis, normal pouches, or familialadenomatous polyposis pouches. Inflamm Bowel Dis. 2012; 18: 925–34.