Abstract
Lyme borreliosis (LB) is a tick-transmitted infectious disease caused by Borrelia burgdorferi sensu lato (s.l.). In Europe, three different Borrelia species are the main causative agents of LB: B. burgdorferi sensu stricto (s.s.), Borrelia afzelii, and Borrelia garinii. The latter depends heavily on birds as its main reservoir hosts. In fact, birds can act both as biological carriers of Borrelia and transporters of infected ticks. The seasonal migration of many bird species not only aid in the spread of B. garinii to new foci but also influence the high level of diversity found within this species. B. garinii have been isolated not only from terrestrial birds in Europe, but also from seabirds worldwide, and homology between isolates in these two different infection cycles suggests an overlap and exchange of strains. In addition, it has been shown that birds can maintain and spread B. garinii genotypes associated with LB in humans. This review article discusses the importance of birds in the ecology and epidemiology of B. garinii spirochetes.
Lyme borreliosis (LB) is the most common vector-borne disease in the northern hemisphere. The multisystem illness is due to infection by Borrelia burgdorferi sensu lato (s.l.) bacteria, spread by different Ixodes ticks. In Northern America, only B. burgdorferi sensu stricto (s.s.) is regularly isolated from human patients. However, on the Eurasian continent, the situation is more complex because three different species cause the disease, namely Borrelia afzelii, B. garinii, and B. burgdorferi s.s. Citation1. In addition, these species are spread by different vectors, Ixodes ricinus in Europe and I. persulcatus in Asia. The habitats of these two tick species overlap in Eastern Europe Citation2. Also, the reservoir hosts for the three species are different. B. afzelii depend on various rodents as their main reservoir host, whereas B. garinii mainly depend on birds. B. burgdorferi, s.s., on the other hand, appear to be more of a generalist and can use both birds and rodents as its reservoir host Citation3.
Borrelia garinii has not only been isolated from terrestrial birds but also from seabirds Citation4Citation5Citation6. The relationship between seabirds and seabird-oriented ticks was recently described in a review by Dietrich et al. Citation7. As a consequence of seasonal bird migration, B. garinii is widely spread around the globe in a marine infection cycle Citation8Citation9Citation10. Possibly, bird migration can be one explanation to why B. garinii is the most heterogeneous of all LB-causing species studied so far Citation11Citation12Citation13.
In Europe, B. garinii is second only to B. afzelii as causative agent of LB and even though this species is dominating among patients with neuroborreliosis, it is not thoroughly studied Citation14. Explanatory factors for this could be the complicated ecology involving different reservoir hosts, the wide geographical distribution, and the heterogeneity among its genotypes. This is further discussed in subsequent sections of this review.
The role of birds in the epidemiology of different pathogens
Birds are known to play an important role in the epidemiology of many pathogens also infective to humans; their relatively long lifespan and the fact that they can fly aid in dispersing infections worldwide. Additionally, birds of diverse species tend to congregate at migration stopover sites and thereby allow for horizontal transfer of pathogens. In some cases, the bird itself is a relatively poor host but, spreading pathogens to new distant foci where it can infect a more suitable reservoir host may be of great importance Citation15. There are three main mechanisms by which birds can be involved in spreading pathogens Citation16. Firstly, as biological carriers meaning that the bird is infected and the pathogen multiplies in the avian body. Some of the more well-known pathogens that have been isolated from wild migrating birds include: West Nile virus, Influenza A virus, Vibrio cholerae, Campylobacter jejuni, and Salmonella typhimurium Citation17Citation18Citation19Citation20. The two latter species can also contaminate water through the feces of birds. Secondly, birds can act as both external and internal carriers without pathogen amplification. Fungal spores from different species, including Candida albicans and Aspergillus fumigatus simply stick to feathers or other avian body parts as a means of transportation Citation21Citation22. Thirdly, birds can act as transporters of infected ectoparasites. Medically, the most important ectoparasites on birds are ticks, i.e. Ixodes spp. and Argas spp. Tick-Borne Encephalitis virus has been found in ticks feeding on birds of many different species Citation22Citation23. These birds often originate from Russia or Eastern Europe. Also, isolates of Anaplasma (Ehrlichia) phagocytophila identical to clinical strains causing human granulocytic anaplasmosis (ehrlichiosis) have been isolated from ticks feeding on wild birds Citation24. There are also reports of Rickettsia sibirica, Coxiella burnetii, and Babesia microti in ticks parasitizing on birds Citation25Citation26Citation27. In the case of Borrelia spirochetes and B. garinii in particular, birds can function both as biological carriers with and without amplification in the host and as transporters of attached ticks infected with Borrelia spirochetes for long-range dispersion of both the vector and the pathogen.
The influence of ticks and host reservoir competence on B. garinii ecology
In adult form, only the female tick seeks a blood meal and the mating often takes place on the blood host itself, sometimes even while the female tick is feeding. The female tick then lays eggs that hatch into larvae and the life cycle restart Citation28. B. burgdorferi s.l. spirochetes are found among different species of Ixodes ticks and a wide range of vertebrate hosts. In general, to maintain a large pool of infected reservoir animals, young individuals need to be infected and be able to stay infected for a long period of time. Similarly, the tick transmission cycle is dependent on tick larvae becoming infected when feeding on an infected host. After having completed the molting and the ticks feed again as nymphs, they transmit the infection to a new host. It is important to emphasize that infected larval or nymphal ticks remain infected after having molted to the next developing stage, even though bacterial numbers may have decreased dramatically Citation29. Abiotic factors such as temperature and humidity influence the Ixodes tick life cycle and therefore also infecting spirochete numbers Citation28. Lower temperatures slow down the life cycle, and a decrease in ambient temperature from 24°C to 15°C has shown to extend the time needed for molting of certain Ixodidae ticks by an average of 30 days in laboratory settings. This in turn means that the incubation time for the spirochetes infecting the ticks will be extended. On the other hand, ambient temperatures in the range of 27–33°C also negatively influence the spirochete numbers in infected Ixodes ticks and render them non-infective to mice Citation30Citation31. The relative humidity can also have an indirect impact on the choice of blood host because it influences the height at which the tick quest in the herbage and therefore possibly the size of the animal Citation32. The competence of a particular animal to function as reservoir host is thought, at least in part, to depend on the complement system, indicating that not all animal species are equally susceptible to a B. garinii infection Citation3. In vitro studies and sampling of wild animals have shown that B. garinii Outer surface protein A (OspA) serotype 3, 5, 6, and 7 type of strains are resistant to normal bird sera but are sensitive to sera from different rodents. These four serotypes are therefore thought to rely on different birds as reservoir hosts. On the other hand, B. garinii OspA serotype 4 strains show the complete opposite pattern of serum resistance and in nature are also not found in birds but different species of rodents Citation3. Based on the ecological characteristics together with multilocus sequence analysis, it has also been suggested that B. garinii OspA serotype 4 strains should be raised to species status and be named Borrelia bavariensis sp. nov. Citation12.
Borrelia and birds
Anderson and Magnarelli Citation33 isolated B. burgdorferi s.s. from Ixodes scapularis collected from different species of birds. Later, they also isolated spirochetes from the livers of passerine birds, finally corroborating that birds are indeed important reservoir host for LB and as such important for the ecology and epidemiology of B. burgdorferi s.s. Citation34. Since then, a number of studies focusing on wild birds have expanded the knowledge regarding birds as reservoirs for Borrelia Citation4Citation6Citation35Citation36.
Today we know that birds spread and maintain Borrelia spirochetes very similar to clinical isolates and therefore, we believe that the role of birds in the ecology and epidemiology of LB is greater than previously understood Citation4Citation8. For a long time, birds were ruled out as potential hosts for B. burgdorferi s.l. spirochetes, mainly because of their high body temperature. As the optimal growth temperature for many LB-causing strains had been determined to 34–37°C and since birds have an elevated body temperature compared to many mammals, they were not considered to be likely reservoir hosts Citation37Citation38. However, unlike other species, some B. garinii isolates are reported to grow at temperatures of up to 41°C; this has also been suggested to be an adaptation to the avian host Citation39. Possibly, this is one reason why B. garinii is the species most frequently infecting birds in Europe and Asia Citation4Citation6Citation40.
Terrestrial birds as reservoir hosts
Some experimental studies suggest that birds are relatively poor reservoir hosts for B. burgdorferi s.l. Piesman et al. showed that 1-week-old chickens infected with B. burgdorferi s.s. were only able to transmit the spirochetes to new larvae during the first 3 weeks post infection. In addition, the reservoir competence also seemed to decline dramatically with age. When 3-week-old chickens were challenged with B. burgdorferi s.s., only a fraction of feeding ticks were positive for spirochetes 1 week post challenge Citation41. Other studies show that birds indeed have the potential to serve as competent reservoir hosts. When Isogai et al. infected Japanese quails (Coturnix japonica) with B. garinii, they were able to reisolate spirochetes from lung and kidney tissue 1 month post infection and from skin samples up to 2 months post infection. Citation42. Also, Canary finches (Serinus canaria) have been assessed for reservoir competence when challenged with B. burgdorferi s.s. Spirochetes could be detected in blood 2 weeks after challenge using microscopy. Furthermore, Borrelia DNA could be detected by polymerase chain reaction (PCR) up to 3 months post infection in several internal organs, suggesting a persistent infection Citation43.
Apparently, B. burgdorferi s.s. can infect both rodents and birds Citation3. However, differences in reservoir competence have been reported for some ground-foraging bird species in North America, meaning all are not equally competent in transmitting B. burgdorferi s.s. to naïve ticks. For example, in one field experiment using xenodiagnosis, American robins (Turdus migratorius) were suggested to be competent reservoir hosts. On the other hand, Song sparrows (Melospiza melodia) and Gray cat birds (Dumetella carolinensis) were shown to have intermediate and low reservoir competence respectively Citation44. Some of the data are also supported by others performing experimental infections Citation45. For instance, the reservoir competence for American robins has been shown to be comparable to that of some rodents, measured by the capacity to infect feeding larvae. Even though the infection in the birds disappeared after 6 months, they remained susceptible to reinfection. In addition, ticks that acquired an infection by feeding on birds could subsequently infect mice, showing the potential influence of birds on the ecology of B. burgdorferi s.s. in North America Citation45.
Reservoir competence of passerine birds in Europe
In the first large-scale study focusing on migrating terrestrial birds, the importance of passerine birds as reservoirs for B. burgdorferi s.l. spirochetes and as carriers of infected ticks was shown Citation4, where 13,123 migrating passerine birds, comprising 38 different species, were captured (at Ottenby bird observatory in Southern Sweden) and examined for infected ticks. The birds were divided into two groups, ground foragers and non-ground foragers. In total, 1,120 I. ricinus larvae and nymphs were collected and screened for the presence of B. burgdorferi s.l. spirochetes by quantitative PCR (qPCR). Aggregation of infestation risk was indicated by the finding that once a bird was infested with one tick, the likelihood of infestation with two or more ticks was higher than would be expected. Not surprisingly, the risk was greater for the ground-foraging birds than for the non-ground-foraging birds (5.4% vs 1.4%). The same pattern was also seen when retrospectively analyzing data from more than 15,000 birds captured in 1991 Citation6. In total, 14% of the ticks were positive for B. burgdorferi s.l. spirochetes and almost twice as many nymphs than larvae (19.3% vs 10.1%) Citation4. This is probably explained by successive accumulation of spirochetes during feeding both as larvae and nymphs. The finding that also larvae were infected, again points to the fact that birds act as reservoir hosts. To exclude the possibility of transovarial transmission, birds infested with larvae were studied by comparing the proportion of birds with infected larvae among birds infested with one larva and the proportion of birds with one or more infected larvae among multiple infested birds. A higher prevalence of infection among ticks from multiple infested birds (21.4%) compared to single infested birds (5.5%) was found, showing that birds indeed were the source of infection. Also, a correlation between the proportion of birds infested with larvae and nymphs and the proportion of birds infested with infected ticks showed that birds were the reservoir hosts of the spirochetes. In addition, 3% of the ground-foraging birds were infested with infected ticks, compared to 0.6% of the non-ground-foraging birds. The percentage of birds with infected larvae among the larvae-infested birds was higher among ground-foraging species (34.0%) than among other bird species (7.8%). This, again, suggests a more central role in the ecology of B. burgdorferi s.l. for the former group of birds. The birds’ reservoir competence, determined as the efficiency of spirochete transmission to larvae with regard to spirochete numbers and infection prevalence, was also evaluated. When analyzing spirochete load and infection prevalence for larvae collected from the same bird, these parameters were found to positively correlate for 56 larvae from 25 birds. However, an experimental infection where larvae would be allowed to molt to nymphs and subsequently were allowed to feed on new hosts would fully determine the role of birds as reservoir hosts as proposed by Richter et al. Citation45. Due to the size of the sample material, this was unfortunately not possible. Furthermore, in order to know if the two groups of birds (ground-foraging and non-ground-foraging birds) differed in reservoir competence, larvae from individual birds were studied. There were no differences between the two groups regarding the mean value of spirochete numbers in the larvae: 135 (95% CI 21–862) and 23 (95% CI 2–318), respectively. Also, there was no difference in infection prevalence between the two groups of larvae: 61% (95% CI 46–77%) and 77% (95% CI 50–100%), respectively. Together, this suggests that the two groups of birds are equally competent for infecting larvae and their importance as reservoir hosts is rather decided by their foraging behavior. The Borrelia species were determined for 88 of the 160 positive samples. The overall dominance of B. garinii and total lack of B. afzelii among larvae was in agreement with other studies focusing on host association of B. burgdorferi s.l. spirochetes Citation3. This finding yet again indicates the important role of birds in the ecology of B. garinii.
To study the clinical relevance of the B. garinii spirochetes spread by passerine birds, 47 isolates from ticks infesting birds and 11 clinical isolates of the same species from erythema migrans (EM) patients Citation46 were compared, using the amplicons generated from the rrs (16S)–rrl (23S) intergenic spacer (IGS) Citation8Citation47. Two groups were defined with nine and two sub groups respectively (1:1–1:9 and 2:1–2:2) as shown in . Six subgroups (1:1, 1:3, 1:4, 1:9, 2:1, and 2:2) comprising 29 (62%) of the bird tick isolates were also found in the biopsies from EM patients. In addition, larvae were infected with three variants (1:1, 1:3, and 2:1) that also cause disease in humans. Passerine birds are therefore reservoir hosts of clinically important B. garinii spirochetes.
Table 1. Grouping of Borrelia garinii isolates from ticks infesting passerine birds and clinical samples [erythema migrans (EM)] based on the rrs (16S)–rrl (23S) intergenic spacer (IGS)
Dubska et al. examined the prevalence of various Borrelia spp. in I. ricinus ticks collected from passerine birds in Czech Republic. In agreement with our findings, great tits (Parus major), Eurasian blackbirds (Turdus merula), and song thrushes (Turdus philomelos) were found capable of transmitting B. garinii to feeding ticks. Furthermore, the two latter bird species were shown not only to be competent reservoir hosts but also suggested to play a central role in the ecology of B. garinii in Europe Citation48Citation49.
In conclusion, migratory passerine birds can have dual roles in the maintenance of B. burgdorferi s.l. spirochetes, both as long-range carriers of infected ticks and as reservoir hosts infecting larvae. Thus, their contribution to B. burgdorferi s.l. ecology and epidemiology is of great importance.
The marine infection cycle
Olsen et al. Citation5 were the first to isolate Borrelia spirochetes from Ixodes uriae. The ticks were collected from razorbills (Alca torda) on an isolated island in the Baltic Sea where no mammals were present. As seabirds were the only blood host available for the ticks, a marine enzootic infection cycle was suggested, where B. garinii circulating among colonial seabirds depend on I. uriae as the only vector Citation5. The distribution of this marine infection cycle was then studied since large seabird colonies are present globally. I. uriae were collected from seabirds at nine different locations worldwide. B. garinii spirochetes with identical flagellin B (flaB) genes were found in ticks from both the southern hemisphere (Campbell Island, New Zealand, and Crozet Islands) and the northern hemisphere (Egg and St. Lazaria Islands, USA) suggesting that seabirds can act as long-distance carriers of the infection (; 10). As the ticks only feed for a few days and the birds’ migration causes them to spend longer periods of time above or in open water, the spirochetes are probably transported as a latent infection in the birds, rather than by infected ticks. A phylogenetic analysis of I. uriae from both the southern and the northern hemisphere also suggests separate reproduction cycles of the ticks Citation50. It has been shown that a latent B. burgdorferi s.s. infection can be reactivated when the birds are subjected to stress as in the case of seasonal migration Citation51. This could further aid in the global dispersal of B. burgdorferi s.l. infected ticks. B. garinii is by far the most prevalent species isolated from seabirds and I. uriae, even though occasionally also B. lusitaniae and B. burgdorferi s.s. have been found Citation52. As discussed earlier, B. garinii is one of the most prevalent species found among I. ricinus and I. persulcatus in Europe and Asia. However, the marine infection cycle increases the habitat to include also the North Pacific Ocean (Egg and St. Lazaria Islands outside Alaska, USA) and the North Atlantic Ocean (Gull Island, Canada). This suggests B. garinii is present on both the West and the East coasts of North America Citation10Citation53.
Fig. 1. Global distribution of Borrelia garinii in the marine infection cycle. Seabird colonies where B. garinii spirochetes are known to be present are indicated with a circle. The seabird colonies are: 1. Hornöya Island, Norway Citation9; 2. Faroe Island, Denmark Citation61; 3. Flatey Island, Iceland Citation10; 4. Commander Island, Russia Citation8; 5. Malgrundet Island, Sweden Citation62; 6. Bonden Island, Sweden Citation5; 7. Campbell Island, New Zealand Citation10; 8. Crozet Islands Citation10; 9. Egg and St. Lazari Islands, USA Citation10; 10. Gull Island, Canada Citation53.
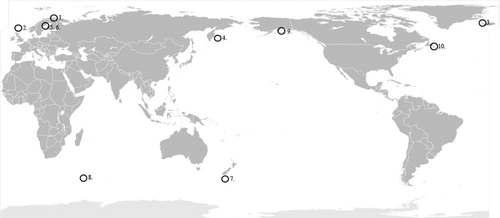
Surprisingly, B. garinii have also been isolated from I. uriae found in seabird colonies located in extreme climate zones such as the Arctic and the subAntarctic regions as well as the Bering Sea littoral Citation8Citation9Citation10. Seabird colonies where B. garinii infected I. uriae or birds have been found are indicated in . When comparing seabird-associated B. garinii strains from Arctic Norway (Hornöya Island) with other isolates collected from seabird colonies on Faroe Islands, identical IGS were found Citation9. Also, close to identical IGS were found when comparing the Arctic B. garinii isolates to those isolated from migrating passerine birds and LB patients in southern Sweden. This supports the theory of birds as global carriers and disseminators of B. garinii, also with potential impact on human health. This will be discussed more in detail later in this review article.
Aside from the marine infection cycle, other enzootic cycles involving avian hosts and specialized tick species have been studied. The tick Ixodes dentatus has been described as vector for Borrelia spp. It is to a large extent ornitophagous and has been proposed a potential factor in maintenance and dispersal of Borrelia spp. Citation54. Also, B. garinii and B. burgdorferi s.s. have been found in Ixodes lividus, which is almost exclusively associated with Sand Martins (Riparia riparia) Citation55.
The seabird tick I. uriae
Ixodes uriae is also known as the ‘seabird tick’ (). It is strictly localized to seabird colonies where it completes the entire life cycle by feeding on various seabirds Citation56Citation31. It is wide ranging in the choice of seabird host and has been reported to feed on more than 50 different colonial seabird species Citation56Citation57. I. uriae can be found all around the world, both on the southern and the northern hemisphere Citation58. It is also present in very demanding climates not typically associated with ticks, such as the Arctic and subAntarctic Citation9Citation10Citation58. This tick can withstand temperatures as low as –30°C, which is necessary for survival in these often very harsh environments. In milder climates, the tick has a 3-year lifespan and feeds once per year. However, in colder climates (Arctic and Barents Sea littoral), the tick's life cycle can be as long as 7 years Citation31Citation59. I. uriae are often very abundant in these seabird colonies and to keep the appropriate humidity, the ticks tend to stay together in clusters Citation60. Therefore, virtually under every stone and in every crack, it is possible to find great numbers of ticks in all developmental stages and both sexes () and as a consequence, many birds become heavily infested.
Prevalence and densities of B. garinii in ticks and seabirds
Many seabird colonies are very crowded, and spread of infection among birds should therefore be easily facilitated. Different studies suggest that as many as 30% of the nymphs and adults in seabird colonies are positive for B. garinii Citation8Citation10. A rather homogeneous B. garinii population could be expected in these colonies since high numbers of ticks and birds thrive on often relatively limited areas. Surprisingly, great heterogeneity among the B. garinii spirochetes has been observed in different studies, suggesting a frequent contact with other distant geographical locations Citation8Citation10. Today, colonies worldwide are known to harbor B. garini-infected I. uriae or seabirds. Some of the B. garinii isolates from different colonies also share high homology, based on sequencing of flaB or IGS, even though the seabird colonies are separated by thousands of kilometers (; ).
Table 2. Borrelia garinii rrs (16S)–rrl (23S) intergenic spacer (IGS) sequence identity within and between strain collections of different geographical and biological origins
When DNA extracts from 299 I. uriae collected from a colony with tufted puffins (Fratercula cirrhata) on the Commander Islands, Russia (; ), were screened by qPCR for presence of B. burgdorferi s.l. spirochetes, 99 (33.1%) were found positive. By partial sequencing of the IGS amplicon, all positive samples were identified as B. garinii. The infection prevalence was equally distributed among the different development stages of ticks. As expected, the bacterial densities dramatically increased after each blood meal. When nymphal ticks had completed their feeding, mean bacterial numbers per specimen had increased from 16 (95% CI 5–49) to 893 (95% 144—5,546). A similar increase from 209 (95% CI 54–807) to 2,489 (95% CI 535–11561) mean spirochetes per adult tick could also be observed. The molting process had a negative influence on bacterial numbers, and therefore an oscillating pattern of the spirochete population appeared when different development stages of the tick were compared (; 8). Interestingly, even though the infection prevalence was high among the ticks in the colony and the tufted puffins are by far the most dominating seabird species present, none of 86 blood samples collect from adult birds of this species were positive for B. burgdorferi s.l. spirochetes, as shown by qPCR analysis Citation8. As discussed earlier, some studies suggest that birds are poor reservoir hosts for B. burgdorferi s.l. spirochetes, whereas others show that Borrelia infection in birds can persist for months Citation51Citation41. B. garinii have been isolated earlier from Atlantic puffins (Fratercula arctica), a close relative to the tufted puffin Citation61. The relatively high prevalence of infected ticks of all developmental stages and the total absence of infected adult tufted puffins in the colony on Commander Islands suggest that ticks may constitute the actual reservoir of B. garinii Citation8. Possibly, newly hatched birds staying in their burrows for weeks could be transiently infected and subsequently infect new ticks. In this way, the pool of infected ticks could be maintained even though the infection of the birds wanes over time. This could be clarified by studying blood samples and ticks collected from very young birds.
Fig. 4. The spirochete population found in different stages of Ixodes uriae collected within one seabird colony on Commander Islands, Russia. Mean numbers of spirochetes per tick increase dramatically after each feeding, but the subsequent molting has a negative effect on the cell count. Mean and asymmetric confidence intervals are antilogs of log10-transformed data from positive samples.
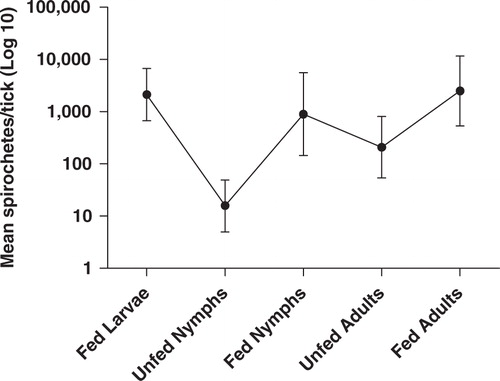
Interaction between the terrestrial and the marine infection cycle
Borrelia garinii spirochetes are found among rodents and passerine birds as well as among seabirds, suggesting that there has to be some overlap between the marine and the terrestrial infection cycles. The marine infection cycle is restricted to different seabirds and the open sea. I. uriae ticks are very wide ranging in their choice of seabird host, but the ticks are seldom found outside seabird colonies. Therefore, an interaction with the terrestrial infection cycle has to take place within the seabird colony. Rodents searching for food and resting migratory passerine birds are possible candidates mediating contact with I. uriae or I. ricinus. The frequency by which I. uriae feed on rodents and other birds is however not known. On some islands in the Baltic Sea, where the I. uriae and I. ricinus habitats overlap and seabirds as well as migrating passerine birds nest side by side, host cross-over may be possible. Furthermore, B. garinii isolates with identical IGS have been found in both I. ricinus and I. uriae collected from the Swedish islands Norrbyskär and Bonden, separated only by 25 km Citation62.
To further study the global ecology of this species, the IGS of 77 B. garinii isolates of diverse biological and geographical origins were compared by Comstedt et al. Citation8, where 20 different genetic variants were found. The seabird-associated strains as a group, isolates from seabirds or I. uriae, represented 32% of the sample collection, but comprised 50% of the IGS types. This suggests that B. garinii associated with seabirds constitute a more diverse group of spirochetes than the terrestrial group. Indeed, the 20 IGS types grouped into six phylogenetic clusters, all including B. garinii circulating among seabirds. Five of the clusters also involved isolates from migrating passerine birds. Furthermore, B. garinii isolated from clinical samples were found in three of the clusters. Strains originating from one seabird colony on Commander Islands in far eastern Russia also did not group together. Instead, some of them were more closely related to B. garinii isolated from European LB patients or passerine birds. One of the most complex genetic variant contained B. garinii isolates from I. ricinus-infesting migrating birds in Sweden, questing I. ricinus from Lithuania, as well as both skin and cerebrospinal fluid isolates from LB patients. Other genetic variants identified represented B. garinii from I. uriae and I. ricinus as well as isolates from migrating passerine birds. Because the B. garinii isolates did not necessarily cluster according to geography, biological hosts, or tick vectors, this again supports the theory of a frequent exchange of strains between the marine and the terrestrial infection cycles Citation8. The range of IGS identity between as well as within different B. garinii strain collections is summarized in . Even though some collections are limited in size, the overall pattern suggests extensive sequence variation among the strains, but also occasionally high sequence identity (IGS identities above 99% between two or more strains representing different collections are indicated in bold numbers in ).
In summary, the current view suggests two reasons why the marine infection cycle is not an immediate threat to humans. Firstly the geographical barrier, as the seabird colonies are often located in coastal areas or on islands where human population density is low, the risk of acquiring an infection is very limited. Secondly the biological barrier, implying that I. uriae prefer to feed on different seabirds rather than mammals including humans Citation56Citation63Citation64. We therefore conclude that the marine infection cycle instead influences the global spread and the heterogeneity among B. garinii strains. However, the exchange of strains with the terrestrial infection cycle as suggested in this review article overcomes the two described barriers, and new B. garinii strains are introduced in environments where also humans are at risk of acquiring the infection.
Conflict of interest and funding
SB was funded by the Swedish Research Council for medicine and health and the Swedish council of Forestry and Agricultural Research, Formas.
Acknowledgements
Pär Comstedt's studies were supported by Formas, grant 23.0161. The Swedish Polar Research Secretariat is acknowledged for logistic support during several expeditions.
References
- Stanek G, Strle F. Lyme borreliosis. Lancet. 2003; 362: 1639–47.
- Kurtenbach K, Hanincová K, Tsao J, Margos G, Fish D, Ogden N. Fundamental processes in the evolutionary ecology of Lyme borreliosis. Nat Rev Microbiol. 2006; 4: 660–9.
- Kurtenbach K, De Michelis S, Etti S, Schäfer SM, Sewell HS, Brade V, et al.. Host association of Borrelia burgdorferi sensu lato-the key role of host complement. Trends Microbiol. 2002; 10: 74–79.
- Comstedt P, Bergström S, Olsen B, Garpmo U, Marjavaara L, Mejlon H, et al.. Migratory passerine birds as reservoirs of Lyme borreliosis in Europe. Emerg Infect Dis. 2006; 12: 1087–95.
- Olsen B, Jaenson TG, Noppa L, Bunikis J, Bergström S. A. Lyme borreliosis cycle in seabirds and Ixodes uriae ticks. Nature. 1993; 362: 340–2.
- Olsen B, Jaenson TG, Bergström S. Prevalence of Borrelia burgdorferi sensu lato-infected ticks on migrating birds. Appl Environ Microbiol. 1995; 61: 3082–7.
- Dietrich M, Gómez-Díaz E, McCoy KD. Worldwide distribution and diversity of seabird ticks: implications for the ecology and epidemiology of tick-borne pathogens. Vector Borne Zoonotic Dis. 2011; 11: 453–70.
- Comstedt P, Asokliene L, Eliasson I, Olsen B, Wallensten A, Bunikis J, et al.. Complex population structure of Lyme borreliosis group spirochete Borrelia garinii in subarctic Eurasia. PLoS One ; : e. 2009; 4: 5841.
- Larsson C, Comstedt P, Olsen B, Bergström S. First record of Lyme disease Borrelia in the Arctic. Vector Borne Zoonotic Dis. 2007; 7: 453–6.
- Olsen B, Duffy DC, Jaenson TG, Gylfe Å Bonnedahl J, Bergström S. Transhemispheric exchange of Lyme disease spirochetes by seabirds. J Clin Microbiol. 1995; 33: 3270–4.
- Kurtenbach K, De Michelis S, Sewell HS, Etti S, Schäfer SM, Holmes E, et al.. The key roles of selection and migration in the ecology of Lyme borreliosis. Int J Med Microbiol. 2002; 33: 152–4.
- Margos G, Vollmer SA, Cornet M, Garnier M, Fingerle V, Wilske B, et al.. A new Borrelia species defined by multilocus sequence analysis of housekeeping genes. Appl Environ Microbiol. 2009; 75: 5410–16.
- Will G, Jauris-Heipke S, Schwab E, Busch U, Rössler D, Soutschek E, et al.. Sequence analysis of ospA genes shows homogeneity within Borrelia burgdorferi sensu stricto and Borrelia afzelii strains but reveals major subgroups within the Borrelia garinii species. Med Microbiol Immunol. 1995; 184: 73–80.
- Wilske B. Diagnosis of Lyme borreliosis in Europe. Vector Borne Zoonotic Dis. 2003; 3: 215–27.
- Olsen B, Munster VJ, Wallensten A, Waldenstrom J, Osterhaus AD, Fouchier RA. Global patterns of influenza a virus in wild birds. Science. 2006; 312: 384–8.
- Hubalek Z. An annotated checklist of pathogenic microorganisms associated with migratory birds. J Wildl Dis. 2004; 40: 639–59.
- De Marco MA, Foni GE, Campitelli L, Raffini E, Di Trani L, Delogu M, et al.. Circulation of influenza viruses in wild waterfowl wintering in Italy during the –99 period: evidence of virus shedding and seroconversion in wild ducks. Avian Dis ; 47. 1993; 2003: 861–6.
- Ogg JE, Ryder RA, Smith Jr HL. Isolation of Vibrio cholerae from aquatic birds in Colorado and Utah. Appl Environ Microbiol. 1989; 55: 95–99.
- Palmgren H, Sellin M, Bergström S, Olsen B. Enteropathogenic bacteria in migrating birds arriving in Sweden. Scand J Infect Dis. 1997; 29: 565–8.
- Woolhouse ME. Gowtage-Sequeria S. Host range and emerging and reemerging pathogens. Emerg Infect Dis. 2005; 11: 1842–7.
- Buck JD. A note on the experimental uptake and clearance of Candida albicans in a young captive gull (Larus sp.). Mycopathologia. 1986; 94: 59–61.
- Hubalek Z. Pathogenic microorganisms associated with free-living birds (review). Acta Sci Nat Brno. 1994; 28: 1–74.
- Waldenström J, Lundkvist A, Falk KI, Garpmo U, Bergström S, Lindegren G, et al.. Migrating birds and tickborne encephalitis virus. Emerg Infect Dis. 2007; 13: 1215–18.
- Bjöersdorff A, Bergström S, Massung RF, Haemig PD, Olsen B. Ehrlichia-infected ticks on migrating birds. Emerg Infect Dis. 2001; 7: 877–9.
- Alekseev AN, Semenov AV, Dubinina HV. Evidence of Babesia microti infection in multi-infected Ixodes persulcatus ticks in Russia. Exp Appl Acarol. 2003; 29: 345–53.
- Somov GP, Soldatov GM. On the role of birds in circulating the pathogen of tick typhus in nature. Zh Mikrobiol Epidemiol Immunobiol. 1964; 41: 126–9.
- Syrucek L, Raska K. Q fever in domestic and wild birds. Bull World Health Organ. 1956; 15: 329–37.
- Sonenshine DE. Biology of ticks. Oxford University Press. New York, 1991
- Piesman J, Oliver JR, Sinsky RJ. Growth kinetics of the Lyme disease spirochete (Borrelia burgdorferi) in vector ticks (Ixodes dammini). Am J Trop Med Hyg. 1990; 42: 352–7.
- Shih CM, Telford III SR, Spielman A. Effect of ambient temperature on competence of deer ticks as hosts for Lyme disease spirochetes. J Clin Microbiol. 1995; 33: 958–61.
- Sonenshine DE. Biology of ticks. New York: Oxford university press. 1993.
- Randolph S, Rogers D. Crimean-Congo hemorrhagic fever. Netherlands: Springer. 2007.
- Anderson JF, Magnarelli LA. Avian and mammalian hosts for spirochete-infected ticks and insects in a Lyme disease focus in Connecticut. Yale J Biol Med. 1984; 57: 627–41.
- Anderson JF, Johnson RC, Magnarelli LA, Hyde FW. Involvement of birds in the epidemiology of the Lyme disease agent Borrelia burgdorferi. Infect Immun. 1986; 51: 394–6.
- Hanincova K, Taragelova V, Koci J, Schafer SM, Hails R, Ullmann AJ, et al.. Association of Borrelia garinii and B. valaisiana with songbirds in Slovakia. Appl Environ Microbiol. 2003; 69: 2825–30.
- Marie-Angele P, Lommano E, Humair PF, Douet V, Rais O, Schaad M, et al.. Prevalence of Borrelia burgdorferi sensu lato in ticks collected from migratory birds in Switzerland. Appl Environ Microbiol. 2006; 72: 976–9.
- Barbour AG. Isolation and cultivation of Lyme disease spirochetes. Yale J Biol Med. 1984; 57: 521–5.
- Welty JC, Baptista L. The life of birds. 4 Sub ed. New York: Harcourt Brace College Publishers. 1997.
- Hubalek Z, Halouzka J, Heroldova M. Growth temperature ranges of Borrelia burgdorferi sensu lato strains. J Med Microbiol 1998; 47: 929–32..
- Hanincova K, Schafer SM, Etti S, Sewell HS, Taragelova V, Ziak D, et al.. Association of Borrelia afzelii with rodents in Europe. Parasitology. 2003; 126: 11–20.
- Piesman J, Dolan MC, Schriefer ME, Burkot TR. Ability of experimentally infected chickens to infect ticks with the Lyme disease spirochete, Borrelia burgdorferi. Am J Trop Med Hyg. 1996; 54: 294–8.
- Isogai E, Tanaka S, BragaIII IS, Itakura C, Isogai H, Kimura K, et al.. Experimental Borrelia garinii infection of Japanese quail. Infect Immun. 1994; 62: 3580–2.
- Olsen B, Gylfe Å Bergström S. Canary finches (Serinus canaria) as an avian infection model for Lyme borreliosis. Microb Pathog. 1996; 20: 319–24.
- Ginsberg H, Buckley P, Balmforth M, Zhioua E, Mitra S, Buckley F. Reservoir competence of native North American birds for the Lyme disease spirochete, Borrelia burgdorfieri. J Med Entomol. 2005; 42: 445–9.
- Richter D, Spielman A, Komar N, Matuschka FR. Competence of American robins as reservoir hosts for Lyme disease spirochetes. Emerg Infect Dis. 2000; 6: 133–8.
- Bennet L. Erythema migrans in primary health care. Doctoral thesis, Lund University., Malmö, 2005.
- Bunikis J, Garpmo U, Tsao J, Berglund J, Fish D, Barbour AG. Sequence typing reveals extensive strain diversity of the Lyme borreliosis agents Borrelia burgdorferi in North America and Borrelia afzelii in Europe. Microbiology. 2004; 150: 1741–55.
- Dubska L, Literak I, Kocianova E, Taragelova V, Sychra O. Differential role of passerine birds in distribution of Borrelia spirochetes, based on data from ticks collected from birds during the postbreeding migration period in Central Europe. Appl Environ Microbiol. 2009; 75: 596–602.
- Dubska L, Literak I, Kocianova E, Taragelova V, Sverakova V, Sychra O, et al.. Synanthropic birds influence the distribution of Borrelia species: analysis of Ixodes ricinus ticks feeding on passerine birds. Appl Environ Microbiol. 2010; 77: 1115–17.
- Gylfe Å Yabuki M, Drotz M, Bergström S, Fukunaga M, Olsen B. Phylogeographic relationships of Ixodes uriae (Acari: Ixodidae) and their significance to transequatorial dispersal of Borrelia garinii. Hereditas. 2001; 134: 195–9.
- Gylfe Å Bergström S, Lundström J, Olsen B. Reactivation of Borrelia infection in birds. Nature. 2000; 403: 724–5.
- Duneau D, Boulinier T, Gómez-Díaz E, Petersen A, Tveraa T, Barrett R, et al.. Prevalence and diversity of Lyme borreliosis bacteria in marine birds. Infect Genet Evol. 2008; 8: 352–9.
- Smith Jr RP, Muzaffar SB, Lavers J, Lacombe EH, Cahill BK, Lubelczyk CB, et al.. Borrelia garinii in seabird ticks (Ixodes uriae), Atlantic Coast, North America. Emerg Infect Dis. 2006; 12: 1909–12.
- Hamer S, Hickling G, Sidge J, Rosen M, Walker E, Tsao J. Discovery of diverse Borrelia burgdorferi strains in a bird-tick cryptic cycle. Appl Environ Microbiol. 2011; 77: 1999–2007.
- Movila A, Gatewood A, Toderas I, Duca M, Papero M, Uspenskaia I, et al.. Prevalence of Borrelia burgdorferi sensu lato in Ixodes ricinus and I. lividus ticks collected from wild birds in the Republic of Moldova. Int J Med Microbiol. 2008; 298: 149–153.
- Arthur DR. British ticks. London: Butterworth. 1963.
- McCoy KD, Chapuis E, Tirard C, Boulinier T, Michalakis Y, Bohec CL, et al.. Recurrent evolution of host-specialized races in a globally distributed parasite. Proc Biol Sci. 2005; 272: 2389–95.
- Bergström S, Haemig PD, Olsen B. Distribution and abundance of the tick Ixodes uriae in a diverse subantarctic seabird community. J Parasitol. 1999; 85: 25–27.
- Steele GM, Davies CR, Jones LD, Nuttall PA, Rideout K. Life History of the seabird tick, Ixodes (ceratizodes) Uriae, at St. Abb's head, Scotland. Acarologia. 1990; 31: 125–130.
- Benoit J, Yoder J, Lopez-Martinez G, Elnitsky M, Lee R, Denlinger D. Habitat requirements of the seabird tick, Ixodes uriae (Acari: Ixodidae), from the Antarctic Peninsula in relation to water balance characteristics of eggs, nonfed and engorged stages. J Comp Physiol. 2007; 177: 205–15.
- Gylfe Å Olsen B, Strasevicius D, Marti Ras N, Weihe P, Noppa L, et al.. Isolation of Lyme disease Borrelia from puffins (Fratercula arctica) and seabird ticks (Ixodes uriae) on the Faeroe Islands. J Clin Microbiol. 1999; 37: 890–6.
- Bunikis J, Olsen B, Fingerle V, Bonnedahl J, Wilske B, Bergström S. Molecular polymorphism of the lyme disease agent Borrelia garinii in northern Europe is influenced by a novel enzootic Borrelia focus in the North Atlantic. J Clin Microbiol. 1996; 34: 364–8.
- Clifford CM. Arctic and tropical arboviruses. New York: Academic Press. 1979.
- Mehl R, Traavik T. The tick Ixodes uriae (Acari, Ixodides) in seabird colonies in Norway. Fauna Norv. 1983; 30: 94–107.
- Glöckner G, Lehmann R, Romualdi A, Pradella S, Schulte-Spechtel U, Schilhabel M, et al.. Comparative analysis of the Borrelia garinii genome. Nucleic Acids Res. 2004; 32: 6038–46.