Abstract
Introduction: To contribute to the understanding of multiresistant bacteria, a ‘One Health’ approach in estimating the rate of extended-spectrum beta-lactamase (ESBL)-producing Escherichia coli and getting insights into the transmission from clinical settings to the surrounding environment was employed by collecting fecal samples of dogs in a public area. Isolates were compared to those from samples of diseased dogs from a nearby small-animal clinic.
Materials and methods: One hundred fecal samples of dogs were collected on a single day in the public area of a veterinary faculty with a small-animal clinic and adjacent residential neighborhoods. All identified ESBL-producing strains were isolated by selective plating, genotypically analyzed by DNA microarray, polymerase chain reaction, sequence analysis, and pulsed-field gel electrophoresis and compared to 11 clinical ESBL/AmpC-producing E. coli isolated from diseased dogs treated in the small-animal clinic 2 months before and 2 months following the environmental sampling collection.
Results and discussion: Fourteen percent (14/100) of the extra-clinical samples harbored phenotypic ESBL/putative AmpC-producing E. coli with additional resistances against other antimicrobials. One ESBL-strain displayed an identical macrorestriction pattern to one clinical, another one to three clinical clonal ESBL-producing strains. The genotypic ESBL-determinants (blaCTX-M-1 and blaCTX-M-15) and detection rates (10%) in dog feces collected outside of the small-animal clinic are comparable to the rates and ESBL-types in the healthy human population in Germany and to clinical and non-clinical samples of humans and companion animals in Europe. The occurrence of identical strains detected both outside and inside the clinical setting suggests a connection between the small-animal clinic and the surrounding environment.
In conclusion, dog feces collected in proximity to veterinary facilities should be considered as a non-point infection source of zoonotic ESBL-producing E. coli for both animals and humans. The common sniffing behavior of dogs further urges hygienic measures on the part of dog-patient owners, who should be educated to remove their pet’s feces immediately and effectively.
Extended-spectrum beta-lactamase (ESBL) enzymes have become abundant. They are not only able to hydrolyze penicillins but also newer, third-generation cephalosporins and monobactams. Limitations in antimicrobial therapies result from a multidrug-resistant (MDR) phenotype present in these bacteria, which are often additionally resistant against fluoroquinolones, aminoglycosides, and other classes of antimicrobials (Citation1). ESBL-producing Escherichia coli in humans, livestock, wildlife, and in non-clinical (Citation2–Citation4) and clinical isolates of companion animals originating from soft tissue, enteric, or urinary tract infections (Citation5) are well documented. Limited data, however, are available concerning the rate of these bacteria in dog feces outside of clinical settings worldwide (Citation6) as well as regarding a possible transmission of multiresistant bacteria from veterinary clinics into the environment. Berlin is the city in Germany with the most dogs per inhabitant (Citation7). The dog often lives in close contact with its human companion and sometimes shares home and bed (Citation8, Citation9) and routinely leaves excrement on streets and fields. It is highly interesting to what extent canine feces may carry zoonotic, multiresistant E. coli and its implications for transmission pathways in public health. Also, the keeping of dogs has been recently identified as a putative risk factor for ESBL secretion in owners (Citation10). The aim of this study was to provide a ‘One Health’ approach in estimating the rate of ESBL-producing E. coli in dog feces outside of clinical settings. The samples were collected on a publicly accessible veterinary campus with small-animal clinic in Berlin to assess a possible link between the clinic and the surrounding environment, acknowledging, however, that the proof of a direct epidemiological link would be difficult to establish. A likely contamination of the environment surrounding small-animal veterinary hospitals with multiresistant bacteria was previously demonstrated by Ghosh et al. (Citation11). In addition, samples were collected from adjacent residential neighborhoods of the veterinary faculty. This study detected high rates of ESBL-producing E. coli outside the clinical setting and discovered clonal strains from extra-clinical dog feces and clinical dog isolates.
Material and methods
The samples were collected on a campus of the veterinary faculty and adjacent neighborhoods in the city of Berlin (Germany) within a 24-hour period in June 2013. The area (approximately 2.5 square kilometers) was divided into five equal sized districts. Individual fecal residues, presumably from dogs, were collected using 20 swabs from each district providing 100 total fecal samples. Data collection procedures called for there to be at least 10 meters of distance between the sampling sites and to collect only fresh appearing samples to avoid sampling the same animal more than once. Third-generation cephalosporin-resistant bacteria were isolated using cefotaxime (4 μg/ml)-containing CHROMagar™ plates and were confirmed as E. coli via classical biochemical methods including glucose fermentation (Kligler agar), lysin-indol-motility (LIM agar), and urea fermentation (Citation12). One putative ESBL-producing E. coli isolate per sample was further processed. Confirmatory testing (BD BBL® Sensi-Disc [Becton, Dickinson, Germany]) using the control strain K. pneumoniae ATCC®700603 analyzed the occurrence of not only ESBL-producing strains but also putative AmpC-producing strains (Citation13). Additional resistances to ampicillin, amoxicillin–clavulanic acid, gentamicin, kanamycin, doxycycline, sulfonamide–trimethoprim, chloramphenicol, nalidixic acid (BD BBL® Sensi-Disc [Becton, Dickinson, Germany]), and enrofloxacin (Bayer, Germany) were tested using agar disc diffusion testing according to the CLSI guidelines with the control strain E. coli ATCC®25922 (Citation13). Following DNA isolation, resistance genotyping of the strains was performed via DNA microarray (Alere technologies, Jena, Germany, Array strip® E. coli) (Citation14). Hybridization results for blaCTX-M-like, blaTEM-like, blaOXA-like, and plasmidic AmpC-genes like blaCMY-1 were verified via polymerase chain reaction (PCR) and sequencing as described previously (Citation15, Citation16). The phylogenetic group was determined using Structure 2.3.4 software based on the concatenated sequences of the seven housekeeping genes used for multilocus sequence typing (MLST) (University of Chicago [http://pritch.bsd.uchicago.edu/structure.html]). MLST determination was carried out as described previously (Citation17). Gene amplification and sequencing were performed using primers specified on the E. coli MLST website (University of Warwick [http://mlst.warwick.ac.uk/mlst/]). Sequences were analyzed with the software package Ridom SeqSphere 0.9.39 (Ridom website [http://www3.ridom.de/seqsphere]) and sequence types (STs) were computed automatically. Pulsed-field gel electrophoresis (PFGE) was used as recently described (Citation15) to detect clonally related extra-clinical ESBL-/putative AmpC-producing strains, to estimate the impact of double sampling and to compare the extra-clinical samples to 11 previously confirmed ESBL/putative AmpC-producing E. coli isolates from diseased dogs, which were collected in the small-animal clinic 2 months before and 2 months following the extra-clinical sampling time point. This time period was chosen in order to increase the odds of finding clinical strains with similar macrorestriction patterns compared to those isolated from the extra-clinical dog feces considering the low overall detection of ESBL-producing E. coli in the clinic. Samples collected from diseased dogs included wound tissue, feces, and urine.
Results
In an area of approximately 2.5 square kilometers, 16 of 100 extra-clinical fecal samples revealed cefotaxime-resistant E. coli, 12 of which were ESBL producers and four putative AmpC producers. PFGE detected clones of two ESBL-producing E. coli strains (which were removed from the analysis), resulting in 10 (10%) ESBL-producing, four (4%) putative AmpC-producing, and overall 14 (14%) non-clonal strains. At least one ESBL-producing E. coli was found in all five districts, with no differential repartition. Thirteen strains (including ESBL- and putative AmpC-producers) were resistant to three or more than three antimicrobial classes, thus showing a multiresistant phenotype. Resistance to ampicillin (14/14), amoxicillin–clavulanic acid (10/14), gentamicin (9/14), kanamycin (12/14), doxycycline (13/14), and sulfamethoxazole–trimethoprim (12/14) was common, but chloramphenicol (4/14), nalidixic acid (7/14) and enrofloxacin-resistance (5/14) also occurred (Fig. 1a). Resistance genotyping using DNA microarray accorded mostly with the phenotypic results (see Fig. 1a for details). All ESBL-producing strains (10/10) showed positive signals for the blaCTX-M-group-1 probe, which was confirmed by sequencing (blaCTX-M-1 [5/10], blaCTX-M-15 [5/10]). All putative AmpC-producing strains (4/4) were initially positive for the blaMOX-CMY-9 probe (a class C ESBL-precursor) and additionally harbored blaCTX-M-1, but CMY-1 could only be verified for one strain (IMT31346) with PCR. Other AmpC genes including blaFOX, blaMOX, and blaACT were negative (Citation16). Analysis also detected blaTEM-1 (7/14) and blaOXA-1 (5/14). Non-beta-lactam resistance genes were tetA/B (13/14), sul1/2 (13/14), strA/B (7/14), catA1/B3/B8 (9/14), as well as aadA4 (8/14), aac(6’)-II (5/14), dfrA7/17/19/21 (12/14), and qnrS (1/14) (Fig. 1a).
Fig 1. (a) Phenotypic and genotypic typing results of ESBL- and AmpC-producing E. coli isolates based on a dendrogram (>XbaI-generated PFGE profiles after exclusion of double clones). Software: BioNumerics 6.6, Applied Maths, Belgium, cluster analysis of dice similarity indices based on the unweighted pair group method with arithmetic mean (UPGMA). (b) Comparison of clones from clinical dog isolates and extra-clinical fecal samples.
CEF: cefotaxime; AMP: ampicillin; AMC: amoxicillin–clavulanic acid; GEN: gentamicin; KAN: kanamycin; DOX: doxycycline; SXT: sulfamethoxazole–trimethoprim; CAP: chloramphenicol; NAL: nalidixic acid; ENRO: enrofloxacin; R: resistant; I: intermediate.
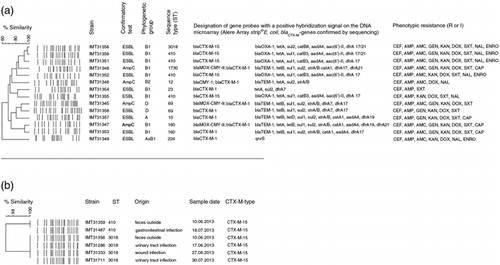
The 10 ESBL-producing strains belonged mostly to phylogenetic group B1 (7/10), three strains belonged to groups D, A, and hybrid group AxB1 (1/10 each). The putative AmpC-producers belonged to ECOR groups B1 (2/4), B2 (1/4), and D (1/4) (Fig. 1a). MLST revealed seven different STs (e.g. ST410 and ST10) among ESBL-producers and four different ones among putative AmpC-producing isolates (see Fig. 1a for details).
PFGE revealed one environmental strain (IMT31359), which showed the same macrorestriction pattern as one clinical isolate (IMT31487) and another one (IMT31356), which was identical to three clonal isolates (IMT31286, IMT31333, and IMT31711) from individual dogs treated in the small-animal clinic (Fig. 1b).
Discussion
A substantial number (14%) of the extra-clinical dog feces tested harbored ESBL/putative AmpC-producing E. coli with additional resistances to other antimicrobial classes. Despite their origin of different sampling districts, clones of two strains were removed from the analysis to reduce the bias of sampling the feces of the same dog. It is also possible, however, that the same clones occur in different animals. The appearance of identical clinical strains (IMT31286, IMT31333, and IMT31711) collected from different samples from different dogs at different time points underlines the success of certain clones in this clinical setting. Finding that same clone (IMT31356) outside the clinic supports the hypothesis of clinical-isolate transmission into the environment, possibly through one of the infected dogs or asymptomatically colonized animals. Interestingly, the corresponding clinical clones do not originate from gastrointestinal infections, but from urinary-tract and wound infections, demonstrating the clinical relevance of certain clones. Certain genetic resistance determinants found in the samples collected in this study, namely blaCTX-M-1 and blaCTX-M-15, have recently been reported to be highly prevalent types of ESBL-colonizers in the healthy community in Bavaria (Germany) (Citation18) and also in non-clinical and clinical samples from humans and companion animals in Europe (Citation2, Citation4, Citation5, Citation19). Besides these gene types, detected STs including ST410 and ST224 are typically ESBL-associated STs, which have been frequently found in human medicine (Citation5).
Although this pilot study faces epidemiological limitations due to a restricted sample size and non-identical sampling time procedures, the reservoir role of dog feces, which contain ESBL-producing E. coli, must not be underestimated.
The rate detected in this study reflects a slightly higher percentage of ESBL-/putative AmpC-producing E. coli than that of human isolates outside of hospital environments (3–10%) (Citation18, Citation20, Citation21). The results might be influenced by the presence of the small-animal clinic in this area, which treats patients with antibiotics, thus selecting for resistance genes and therefore giving one explanation for high carriage of resistant bacteria in dogs taken for a walk on the campus. In addition, previous studies discussed a wash out of multiresistant bacteria from human and veterinary hospitals into the environment (Citation11, Citation22, Citation23). However, dog owners visiting the clinic do not usually walk their dog as far as the adjoining areas where half the samples were collected, and other studies investigating healthy pets in Tunisia found comparable numbers of ESBL producers (Citation3). Regardless, it is worth investigating further the extent to which dog feces in other public urban areas of Berlin, in the adjacent neighborhoods of veterinary clinics, and cities worldwide, contain multiresistant E. coli.
Conclusion
To best of the authors’ knowledge, this is the first study estimating the rate of ESBL-/putative AmpC-producing E. coli, which have been isolated from environmental dog feces, and comparing these to isolates from a nearby small-animal clinic. As dogs often live close to humans and their zoonotic role as potential carriers of multiresistant strains should not be underestimated, their feces as a mechanism for transmission in environmental settings, particularly in close proximity to veterinary clinics, must be acknowledged and better understood. Dog owners in general but especially those of diseased dogs should be educated to remove the pet’s feces immediately as other dogs’ sniffing behavior might contribute to the zoonotic and environmental spread of ESBL-producing bacteria.
Despite the limits of this study’s generalizability, it follows an integrated, ‘One Health’ approach that might serve as a model for future cross-cutting collaborations as well as opportunities for expanded epidemiological research and next-generation sequencing applications in the field of multiresistant bacteria.
Conflict of interest and funding
The authors have not received any funding or benefits from industry or elsewhere to conduct this study.
Acknowledgements
We would like to thank the following for helping with the sampling and the analysis: K Oelgeschläger, A Schmidt, CA Bertram, B Gutjahr, J Kandzia, J Viernickel, O Wenske, S Wichert, S Winkler, S Woelke, L Niemann, and H Laube.
References
- Pitout JD. Extraintestinal pathogenic Escherichia coli: a combination of virulence with antibiotic resistance. Front Microbiol 2012; 3: 9.
- Hordijk J, Schoormans A, Kwakernaak M, Duim B, Broens E, Dierikx C, et al. High prevalence of fecal carriage of extended-spectrum beta-lactamase/AmpC-producing Enterobacteriaceae in cats and dogs. Front Microbiol 2013; 4: 274.
- Sallem RB, Gharsa H, Slama KB, Rojo-Bezares B, Estepa V, Porres-Osante N, et al. First detection of CTX-M-1, CMY-2, and QnrB19 resistance mechanisms in fecal Escherichia coli isolates from healthy pets in Tunisia. Vector Borne Zoonotic Dis 2013; 13: 98–102.
- Costa D, Poeta P, Brinas L, Saenz Y, Rodrigues J, Torres C. Detection of CTX-M-1 and TEM-52 beta-lactamases in Escherichia coli strains from healthy pets in Portugal. J Antimicrob Chemother 2004; 54: 960–1.
- Ewers C, Bethe A, Semmler T, Guenther S, Wieler LH. Extended-spectrum beta-lactamase-producing and AmpC-producing Escherichia coli from livestock and companion animals, and their putative impact on public health: a global perspective. Clin Microbiol Infect 2012; 18: 646–55.
- Procter TD, Pearl DL, Finley RL, Leonard EK, Janecko N, Reid-Smith RJ, et al. A cross-sectional study examining the prevalence and risk factors for anti-microbial-resistant generic Escherichia coli in domestic dogs that frequent dog parks in three cities in South-Western Ontario, Canada. Zoonoses Public Health 2014; 61: 250–9.
- Berlin-Brandenburg Statistisches Landesamt (2013). Statis-tisches Jahrbuch. Berlin: Kulturbuchverlag GmbH.
- Blouin DD. All in the family? Understanding the meaning of dogs and cats in the lives of American pet owners. PhD thesis, Indiana University, Bloomington, IN, 2008.
- Walther B, Hermes J, Cuny C, Wieler LH, Vincze S, Abou Elnaga Y, et al. Sharing more than friendship — nasal colonization with coagulase-positive staphylococci (CPS) and co-habitation aspects of dogs and their owners. PLoS One 2012; 7: e35197.
- Meyer E, Gastmeier P, Kola A, Schwab F. Pet animals and foreign travel are risk factors for colonisation with extended-spectrum beta-lactamase-producing Escherichia coli. Infection 2012; 40: 685–7.
- Ghosh A, KuKanich K, Brown CE, Zurek L. Resident cats in small animal veterinary hospitals carry multi-drug resistant enterococci and are likely involved cross-contamination of the hospital environment. Front Microbiol 2012; 3: 62.
- Winkle S. Mikrobiologische und serologische Diagnostik. Jena: VEB Gustav Fischer Verlag; 1979.
- CLSI (2008). Performance standards for antimicrobial disk and dilution susceptibility tests for bacteria isolated from animals. Approved standard. 3rd ed. Wayne, PA: CLSI.
- Bogaerts P, Hujer AM, Naas T, de Castro RR, Endimiani A, Nordmann P, et al. Multicenter evaluation of a new DNA microarray for rapid detection of clinically relevant bla genes from beta-lactam-resistant gram-negative bacteria. Antimicrob Agents Chemother 2011; 55: 4457–60.
- Ewers C, Grobbel M, Stamm I, Kopp PA, Diehl I, Semmler T, et al. Emergence of human pandemic 025:H4-5T131 CTX-M-15 extended-spectrum-beta-lactamase-producing Escherichia coli among companion animals. J Antimicrob Chemother 2010; 65: 651–60.
- Perez-Perez FJ, Hanson ND. Detection of plasmid-mediated AmpC beta-lactamase genes in clinical isolates by using multiplex PCR. J Clin Microbiol 2002; 40: 2153–62.
- Wirth T, Falush D, Lan R, Colles F, Mensa P, Wieler LH, et al. Sex and virulence in Escherichia coli: an evolutionary perspec-tive. Mol Microbiol 2006; 60: 1136–51.
- Valenza G, Nickel S, Pfeifer Y, Eller C, Krupa E, Lehner-Reindl V, et al. Extended-spectrum-beta-lactamase-producing Escherichia coli as intestinal colonizers in the German community. Antimicrob Agents Chemother 2014; 58: 1228–30.
- Franiek N, Orth D, Grif K, Ewers C, Wieler LH, Thalhammer JG, et al. ESBL-producing E. coli and EHEC in dogs and cats in the Tyrol as possible source of human infection. Berl Munch Tierarztl Wochenschr 2012; 125: 469–75.
- Valverde A, Coque TM, Sanchez-Moreno MP, Rollan A, Baquero F, Canton R. Dramatic increase in prevalence of fecal carriage of extended-spectrum beta-lactamase-producing Enterobacteriaceae during nonoutbreak situations in Spain. J Clin Microbiol 2004; 42: 4769–75.
- Stromdahl H, Tham J, Melander E, Walder M, Edquist PJ, Odenholt I. Prevalence of faecal ESBL carriage in the commu-nity and in a hospital setting in a county of Southern Sweden. Eur J Clin Microbiol Infect Dis 2011; 30: 1159–62.
- Wellington EM, Boxall AB, Cross P, Feil EJ, Gaze WH, Hawkey PM, et al. The role of the natural environment in the emergence of antibiotic resistance in gram-negative bacteria. Lancet Infect Dis 2013; 13: 155–65.
- Martinez JL. The role of natural environments in the evolution of resistance traits in pathogenic bacteria. Proc Biol Sci 2009; 276: 2521–30.