Abstract
Arthropod-borne viruses (arboviruses) may cause severe emerging and re-emerging infectious diseases, which pose a significant threat to human and animal health in the world today. These infectious diseases range from mild febrile illnesses, arthritis, and encephalitis to haemorrhagic fevers. It is postulated that certain environmental factors, vector competence, and host susceptibility have a major impact on the ecology of arboviral diseases. Presently, there is a great interest in the emergence of Alphaviruses because these viruses, including Chikungunya virus, O'nyong'nyong virus, Sindbis virus, Ross River virus, and Mayaro virus, have caused outbreaks in Africa, Asia, Australia, Europe, and America. Some of these viruses are more common in the tropics, whereas others are also found in temperate regions, but the actual factors driving Alphavirus emergence and re-emergence remain unresolved. Furthermore, little is known about the transmission dynamics, pathophysiology, genetic diversity, and evolution of circulating viral strains. In addition, the clinical presentation of Alphaviruses may be similar to other diseases such as dengue, malaria, and typhoid, hence leading to misdiagnosis. However, the typical presence of arthritis may distinguish between Alphaviruses and other differential diagnoses. The absence of validated diagnostic kits for Alphaviruses makes even routine surveillance less feasible. For that purpose, this review describes the occurrence, genetic diversity, clinical characteristics, and the mechanisms involving Alphaviruses causing arthritis in humans. This information may serve as a basis for better awareness and detection of Alphavirus-caused diseases during outbreaks and in establishing appropriate prevention and control measures.
Arthropod-borne viruses (arboviruses) are transmitted by haematophagus arthropods such as mosquitoes, ticks, midges, and sandflies (Citation1), and most of them belong to the Togaviridae, Bunyaviridae, Rhabdoviridae, Reoviridae, and Flaviviridae families (Citation2, Citation3). The main focus of this review is the group of mosquito-borne viruses of the Alphavirus genus in the Togaviridae family. Alphaviruses are single-stranded positive-sense RNA viruses with a genome length ranging from 11,000 to 12,000 nucleotides (Citation4). The genome codes for structural proteins are composed of the capsid proteins (C), envelope glycoproteins (E1, E2, E3, and 6K), and non-structural proteins (nsP1, nsP2, nsP3, and nsP4) (Citation5).
Alphaviruses associated with arthritis have been reported to cause outbreaks in most parts of the world, sometimes with severe impact on human health (Citation6). The past two decades have experienced epidemics of Alphaviruses of public health concern such as chikungunya virus (CHIKV), Sindbis virus (SINV), Ross River virus (RRV), Mayaro virus (MAYV), Barmah Forest virus (BFV), and O'nyong'nyong virus (ONNV), which has resulted in high morbidity in humans (Citation7–Citation9). Regardless of the evidence of circulation of these viruses in most parts of the world, and the mechanisms behind the emergence of Alphavirus disease, the actual burden of the disease in human populations is not known. This may be attributed to the lack of sero-epidemiological studies and reliable diagnostic tools. In addition, the role of animals, birds, and arthropod vectors in the transmission and dissemination of these viruses across diverse geographical areas is unclear (Citation10, Citation11). Emphasis has been put on other arboviruses that present more severe symptoms such as encephalitis and/or haemorrhagic fevers, which result in high mortality with little attention on Alphaviruses causing arthritis, leading to high morbidity and long-lasting symptoms (Citation9, Citation12–Citation14).
It is suggested that a number of demographic and societal changes have a key impact on the ecology of Alphaviruses and may be responsible for the emergence of these viruses in general. These demographic changes include global expansion of the population that results in uncontrolled urbanisation and leads to increased movement of humans, animals, and vectors, which may harbour viral pathogens. For example, chikungunya fever was introduced to Italy via a viraemic traveller from India (Citation15). Societal changes such as human encroachment in wildlife and animal sanctuaries in search of pasture and water during droughts, changes in land-use patterns, irrigation systems, and deforestation also have an impact (Citation16, Citation17). Demographic and societal factors may also have an influence on the transmission cycle of these viruses, which largely involve interaction between pathogen, vector, host, and the environment (Citation18). Climate change may also contribute to the emergence and re-emergence of Alphaviruses (Citation19). This is made possible by fluctuations in abiotic factors such as temperature, rainfall, and humidity, which may lead to a shift in the vectors dynamics (Citation19, Citation20).
According to Atkins (Citation21), viral genetic changes due to genetic reassortment, recombination, and mutations may result in more virulent strains that have increased potential to cause epidemics in immunologically naïve populations. A good example is the Western equine encephalitis virus, which is a result of recombination between viruses of the Eastern equine encephalitis virus and SINV (Citation22). This review will focus mainly on emerging and re-emerging Alphaviruses of public health importance with an inclination to CHIKV, ONNV, RRV, and SINV, which are known to cause arthritis in humans. We describe the epidemiology, clinical features, diagnosis, molecular biology, genetic diversity, and future inclination and/or trends in the emergence of these viruses.
Epidemiology of arthritic Alphaviruses
The epidemiology of Alphaviruses is not clearly understood due to the sporadic nature of the epidemics caused by these viruses (Citation13). Nonetheless, Alphaviruses have a wide geographical distribution across all continents (Citation14). In general, most of these Alphaviruses are known to be enzootic in nature and have the potential to disseminate to other regions (Citation23). That may be because of the adaptations of vectors in new ecological niches, air-travel, seaborne trade, and virus evolution among other factors.
Chikungunya virus
CHIKV was first isolated in 1952 from the blood of a febrile patient in Tanganyika, presently known as Tanzania and since then has spread to other regions such as southern, western, and central Africa (Citation24) (). CHIKV outbreaks have also been documented in India: Calcutta in 1963; Chennai, Pondicherry, and Vellore in 1964; Visakhapatnam, Rajahmundry, and Kakinada in 1965; Nagpur in 1965; and Barsi in 1973 (Citation25) (). Furthermore, CHIKV caused an outbreak in the Democratic Republic of the Congo between 1999 and 2000 with concomitant outbreaks in Indonesia (). The period 2004–2011 witnessed the epidemic of CHIKV in epic proportions. This outbreak was linked to transmission via the Aedes albopictus mosquito vector that has a wide geographical distribution (Citation13). The epidemic began in Kenya and eventually spread across the Indian Ocean to India and Southeast Asia, reaching Myanmar in 2010 (Citation26). In Kenya, CHIKV affected communities living in Lamu Island and Mombasa situated near the Indian Ocean (Citation27).
Affected islands in the Indian Ocean included Comoros, Mauritius, Seychelles, Madagascar, Mayotte, and Réunion involving hundreds of thousands of people (Citation28). From 2005 to 2006, the Réunion Island was hit by the CHIKV, accounting for approximately 265,000 clinical cases and 237 deaths (Citation28). The global toll of CHIKV infection is estimated to be approximately 2 million (Citation28) with India alone reaching an estimated 1.3 million cases (Citation28). Recent outbreaks of CHIKV have been identified in the Americas, mainly south and central (Citation29). In 2013, the first outbreak of CHIKV was discovered at St. Martin in the Caribbean, and by 2014, the virus had spread throughout the entire island into Latin America including Brazil (Citation30–Citation32). Reports from these outbreaks have estimated 1.6 million cases. In Chiapas, Mexico, in 2014, an outbreak occurred where 79% of all febrile illnesses with polyarthralgia were CHIKV positive (Citation33).
O'nyong'nyong virus
ONNV was originally isolated from anopheline mosquitoes and human serum during the 1959 epidemic that affected more than 2 million people in East Africa (Kenya, Uganda, Tanzania, Malawi, and Mozambique) (Citation21, Citation34, Citation35). After an absence of 35 years in East Africa, another epidemic occurred in southern Uganda (Citation36). A more recent epidemic (2002) was reported near the shores of Lake Wamala in the Mubende District, Central Uganda (Citation35). Antibodies against ONNV have been detected in humans in Nigeria, Ghana, and Sierra Leone (Citation37, Citation38) (). ONNV has also been isolated in samples from a febrile patient in Chad (Citation34) (). Evidence of ONNV causing an outbreak in Côte d'Ivoire in the 1980s has also been documented (Citation39) (). Although past studies have indicated that ONNV is restricted to Africa, a confirmed imported case of ONNV virus was reported in 2013 in Germany from a 60-year-old woman who had taken vacation in Kenya at the shores of Lake Victoria where outbreaks of the virus had been previously reported (Citation40). Humans may be the only natural host of ONNV, as other vertebrate reservoirs have not yet been identified (Citation41). ONNV includes three strains restricted to Africa, namely, SG650 from Uganda, 37,997 from Senegal, and Igbo Ora virus from Ivory Coast (Citation41).
Sindbis virus
SINV was first isolated from a group of 63 Culex mosquitoes in the Sindbis district, located north of Cairo, Egypt (Citation42). The signs and symptoms of this virus were not well known until 1961 when the virus was isolated from humans in Uganda presenting symptoms of the viral disease (Citation43). This virus has been linked to outbreaks of fever, rash, and arthralgia in South Africa in 1963 and 1974, which affected thousands of people (Citation44) and in Northern Europe in 1981–1982, 1988, 1995, 2002, and 2013, respectively (Citation45–Citation47). In Sweden, SINV causes Ockelbo disease; in Finland, Pogosta disease; and in Russia, Karelian fever (Citation48). Subsequent cases of SINV infection in South Africa have been reported between 1983–1984 and 2006–2010 (Citation44). Other cases and outbreaks of SINV have been documented to occur in Malaysia, Philippines, Papua New Guinea (PNG), Kenya, Australia, and China (Citation49–Citation51) (). A closely related virus to SINV is Babanki virus, which was first identified in Cameroon and known to cause similar signs and symptoms as SINV. This virus is transmitted by similar mosquito vectors of the genus Culex as demonstrated also for SINV and suggested to be a strain of SINV (Citation52, Citation53).
Ross River virus
RRV is endemic in Australia, PNG, and Pacific Islands (Citation54). It is the most common arboviral disease affecting humans in Australia. The virus was first detected in samples of Ochlerotatus vigilax mosquitoes trapped near Ross River, Queensland, Australia in 1963 (Citation55). In 1979 and 1980, RRV was responsible for a massive outbreak in the Western Pacific involving Fiji, New Caledonia, Samoa, and the Cook Islands (Citation56–Citation58).
Mayaro virus
MAYV is endemic in South America and was first isolated and identified in 1954 from patients presenting an acute febrile illness in Trinidad (Citation59, Citation60). Several Mayaro disease outbreaks of low magnitude have been reported to occur in northern Brazil and eastern Bolivia (Citation61, Citation62). In 1978, an outbreak of MAYV occurred in Pará, Brazil where 55 cases were confirmed (Citation63). In 2008, another outbreak of MAYV was reported in a rural community in Santa Barbara municipality, northern Brazil where about 36 cases were tested positive by serology (Citation64).
Transmission of arthritic viruses
A number of mosquito species have been associated as vectors in the transmission cycle of arthritic Alphaviruses (Citation65). For instance, CHIKV is transmitted mainly by Aedes species of mosquitoes. This virus is maintained in two cycles – an urban cycle by A. aegypti and A. albopictus mosquito vectors and a sylvatic cycle by A. furcifer, A. africanus mosquitoes, and wild primates (Citation66). CHIKV transmission cycle involves mosquitoes, which serve as arthropod vectors, and humans as vertebrate hosts and reservoir during epidemics, whereas monkeys, rodents, and birds may act as reservoirs of CHIKV during the interepidemic periods (Citation67).
ONNV is transmitted by anopheline mosquitoes: Anopheles funestus and A. gambiae, which are the primary vectors during epidemics, although a strain of this virus has been isolated from Mansonia uniformis mosquitoes (Citation35, Citation68). The actual reservoir host for ONNV has not been identified yet; however, humans can serve as amplification hosts during epidemics (Citation69).
SINV is transmitted by Culex, Aedes, and Culiseta mosquitoes, and the transmission cycle involves birds as reservoirs and mosquitoes as the viral vector (Citation49, Citation70). For instance, Culex torrentium has been suggested as the major vector in Sweden, and Aedes cinereus for transmitting the virus from birds to humans (Citation71). SINV has been isolated from several Culex and Aedes species of mosquitoes, for example, Culiseta morsitans, Culex pipens, C. torrentium, and Ae. cinereus (Citation47, Citation70, Citation72). The actual reservoirs and their role in the transmission and dissemination of these viruses are still uncertain. Furthermore, this virus has been isolated from isolated juvenile hooded crow (Corvus corone sardonius) (Citation42). Birds are most probably involved in the natural cycle of SINV, as an important reservoir, but spillover to other vertebrates may occur (Citation72–Citation74). Grouse and passerines are probable amplifying hosts for SINV (Citation48, Citation74, Citation75).
RRV is transmitted by a wide range of mosquito species that fall in the genera Culex, Aedes (Ochlerotatus), Anopheles, Coquillettidia, and Mansonia (Citation76). Aedes camptorhynchus and A. vigilax are the primary vectors of RRV in the southern and northern regions, whereas Culex annulirostris is the major vector in inland areas (Citation76, Citation77). The main reservoir hosts belong to family Macropodidae such as kangaroos and wallabies; though horses, brushtail possums, and flying foxes have been associated to play a role in the maintenance of RRV in nature (Citation76, Citation78–Citation80).
The transmission cycle of MAYV is similar to the sylvatic cycle of yellow fever virus (YFV) involving non-human primates, mainly monkeys as reservoirs of the virus (Citation59). Haemagogus species of mosquitoes are the primary vectors of MAYV, although A. aegypti species have also been demonstrated to be competent vectors as indicated by laboratory examinations (Citation81).
In general, the transmission of Alphaviruses encompasses diverse vectors and reservoirs with different biology. So far, only CHIKV seems to generate enough viraemia in humans to sustain transmission via human–mosquito transmission, similar to dengue and malaria. However, mutations may occur that can change the tropism of the virus, as for CHIKV that adapted to growth in a new vector, A. albopictus after the introduction of a single mutation in the E1 envelope glycoprotein gene (Citation82).
Clinical features of arthritic Alphaviruses
The definite clinical picture of Alphaviruses is intricate because most of these viruses present not only similar signs and symptoms as other infections like malaria and dengue (Citation83) but are also transmitted by related mosquito vectors. Even though, CHIKV in some respects shows similar clinical pictures as dengue virus, which may lead to misdiagnosis especially in areas where dengue is endemic (Citation84), there are some typical discrepancies, for example, the presence of joint pain and lack of thrombocytopenia in CHIKV (Citation85). Laboratory documentation is mandatory for etiological assessment. The most common symptoms of arthritic Alphaviruses are acute fever, skin rash, malaise, fatigue, myalgia, and arthralgia that may be severe (Citation12, Citation56, Citation86–Citation88). The infection typically causes symmetrical polyarthritis preferably in large joints in both arms and legs. It is difficult to investigate the proportion of subclinical and asymptomatic infections, but for CHIKV, it is estimated that a majority of infected persons present with symptoms (Citation89). In rare cases, infection because of these viruses may present severe symptoms such as haemorrhage and paraesthesia as with chikungunya fever (Citation90). However, ONNV infections may cause eye and chest pains (Citation91). In rare circumstances, RRV can cause splenomegaly, haematuria and glomerulonephritis, neck stiffness, and photophobia (Citation76, Citation92). Most of the emerging Alphaviruses have been associated to cause severe and long-lasting debilitating joint pain and fatigue (Citation14, Citation86, Citation88, Citation93, Citation94).
Diagnosis of arthritic Alphaviruses
The diagnosis of infections caused by Alphaviruses is mainly performed during epidemics and is faced with challenges due to serological cross-reactivity between Alphaviruses (Citation95). Laboratory documentation is mandatory for etiological assessment. Evidence of coinfection of CHIKV with other arboviruses such as dengue or yellow fever exists and has posed a major constraint in the diagnosis because the same mosquito species that is involved in maintaining CHIKV also maintains dengue viruses and YFV (Citation96, Citation97). Differential diagnosis has to be performed to rule out some diseases such as malaria and typhoid fever, which may present similar symptoms as Alphavirus diseases (Citation83). The periodic nature of outbreaks caused by these viruses poses a challenge in the development of effective diagnostic tools and interventions (Citation13). Virus isolation has been useful in defining viral agents in serum and vectors. Alphaviruses are fast-growing viruses, and if kept under appropriate conditions, they induce cytopathic effects within the first 1–3 days post-infection in contrast to other arboviruses. Serological techniques that use solid-phase antibody-binding assays, such as enzyme-linked immunosorbent assay (ELISA), have provided a diagnostic platform for identification of these viral pathogens (Citation98). However, these techniques require validation due to lack of specificity and sensitivity (Citation99, Citation100). Plaque reduction assay is the gold standard for antibody detection especially towards viruses that belong to a similar genus, in this case Alphaviruses. Reverse transcription (RT)-polymerase chain reaction (PCR) is used for detection of RNA virus in clinical samples or for epidemiological surveys. RT-PCR is an excellent molecular diagnostic method for arbovirus detection with high specificity and sensitivity, enabling the identification of viruses in minute samples (Citation101, Citation102). The next-generation sequencing (NGS) technologies such as 454, SOLiD, and Illumina have allowed for genetic characterisation through genome sequencing. However, NGS has not proven superior to other techniques in the diagnosis of viruses (Citation98). A combination of serology and PCR may be the most suitable means for accurate diagnosis of arthritic Alphaviruses, especially during the first 2 weeks of the disease in order to accurately detect acute and convalescent disease cases. Use of both techniques will enhance the sensitivity and specificity while maintaining a fast turnaround time in diagnosis of Alphavirus infections.
Genetic diversity and phylogeny of arthritic Alphaviruses
Alphaviruses have a wide geographical distribution in the world. This is probably due to the erratic nature of outbreaks caused by these viruses creating a challenge in monitoring of emerging Alphaviruses, their risk, and understanding of the epidemic and maintenance transmission patterns and processes of molecular evolution. Therefore, genetic studies have been performed on a number of Alphaviruses such as Venezuelan and Eastern equine encephalitis virus and CHIKV in order to understand their mechanisms behind their emergence (Citation103).
Genetic diversity of Alphaviruses is an important aspect in the identification of circulating virus strains in diverse geographical regions and how these strains evolve over time. By selection analysis, the amino acid sequences of circulating virus strains can be studied comprehensively to determine whether the selection pressures, which are as a result of mutations, can lead to more virulent strains that can cause severe forms of disease in immunologically naïve populations. For instance, virulent strains of SINV have been demonstrated to infect neurons in mice hence spreading to the central nervous system causing encephalomyelitis. One amino acid substitution (histidine to glutamine) in the SINV-E2 gene at position 55 has been linked to increased virulence (Citation104) and another change (serine for arginine) at E2 position 114 has been linked to decreased virulence (Citation105, Citation106).
Genetic studies indicate the presence of three distinct CHIKV lineages circulating in Asia and Africa (Citation4), namely, Asian, Central/East African, and West African, which are based on their main geographical locations, for example, the West African genotypes comprise isolates from Nigeria and Senegal (). It is believed that the CHIKV outbreaks experienced from 2013 to 2015 in diverse areas in the Caribbean were caused by the Asian genotype strain of the virus, whereas from 2005 to 2006, outbreak in the Réunion Island was caused by the East/Central/South African genotype (Citation107, Citation108). However, in September 2014, genetic analyses of positive samples of cases taken during the recent outbreak of CHIKV experienced in east-central Brazil indicate that this epidemic was caused by the East/Central/South African and not the Asian genotype, indicating that both genotypes are circulating in the Americas (Citation31, Citation109).
Fig. 2 Whole genome phylogeny of Alphaviruses causing arthritis in humans. The tree illustrates the genetic clustering of available whole genomic sequences of CHIKV, ONNV, MAYV, RRV, and SINV extracted from GenBank. The evolutionary history was inferred by using the maximum likelihood method based on the Tamura–Nei model (Citation116). Evolutionary analyses were conducted in MEGA6 (Citation117).
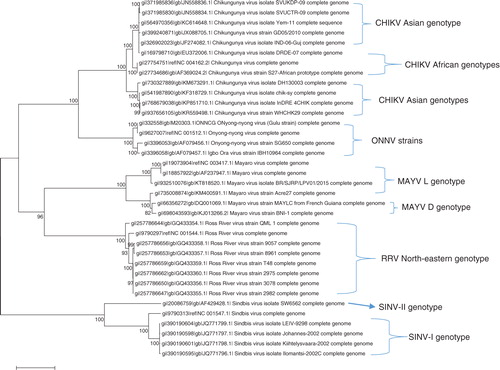
Three major strains of ONNV have been isolated from humans and mosquito species of A. furcifer. These strains are restricted to the African continent including the original Gulu strain from Uganda (Citation41). The nucleotide sequences of these strains have been published in GenBank. Igbo Ora virus, which is endemic in Ivory Coast, was initially thought to be related to ONNV by complement fixation test, and molecular analyses of the sequences confirm that the virus is a strain of ONNV (Citation41) ().
The close relationship between the South African and Northern European SINV strains suggests that migratory birds may have carried the virus over long distances. Avian hosts may advance the spread of the viruses via migratory routes across different continents, and this leads to the expansion of the virus population and increasing the chances for genetic drift (Citation10, Citation49, Citation110). SINV fall in to five distinct genotypes based on the structural glycoprotein E2, with varying geographical distribution, for example, SINV-I circulates in Europe, Middle East, and Africa; SINV-II genotype is found in Australia and Malaysia; SINV-III in India and Philippines; SINV-IV in China and Azerbaijan; and SINV-V in New Zealand (Citation49) ().
RRV has a number of strains circulating in particular regions of the Australian continent (Citation76). These strains have been shown to vary according to their level of virulence in mice and also in terms of their antigens (Citation111–Citation114). Analysis of RRV isolates from Australia and Pacific Islands that have genotypes restricted to specific geographical sites indicates the possibility of the virus being amplified by hosts, such as kangaroos and wallabies, restricted to specific geographical areas (Citation76). RRV has different genotypes circulating mainly in western, north-eastern, and south-eastern parts of Australia (). However, there is a likelihood that some of the RRV stains of the virus are being disseminated by viraemic travellers and/or animals to other geographical regions or even across the globe. Genetic analysis of MAYV sequences has confirmed the presence of two circulating genotypes, D and L, based on the glycoprotein genes and the 3′ non-coding region. The genotype D is distributed in Trinidad, Brazil, French Guiana, Surinam, Peru, and Bolivia, whereas L type is limited to north-central regions of Brazil (Citation115) ().
The presence of diverse Alphavirus genotypes around the globe may signify their restriction to specific geographical locations, vectors, and amplifying hosts. This review clearly points out additional factors such as changes in the host range of vertebrate hosts, ecological, demographic, and societal factors, which may influence genetic diversity of arthritic Alphaviruses.
Future inclination of arthritic Alphaviruses
In general, Alphaviruses have a relatively unknown ecology and epidemiology (Citation13), which make it difficult to predict future outbreaks. A number of factors have been suggested to contribute to the increase in prevalence of these viruses. This includes climate change, increased urbanisation, international travel and trade, change in land-use patterns mainly for agricultural use, deforestation combined with changes in vector biology, and containment and viral adaptation of new ecological niches (Citation16). These factors, combined with the spontaneous nature of these viruses, could serve as a means to provide an in-depth understanding of the emergence and re-emergence of potential Alphaviruses in human populations. In Australia, it is hypothesised that a change in land-use practices and climate change may amplify the range and activity of Alphaviruses as well as other mosquito-borne viruses in the future (Citation118).
The circulation of Alphaviruses in nature has not been fully explored. Information about possible animal reservoirs and their actual role in the maintenance, transmission, and dissemination of these viruses during the interepidemic periods is uncertain and needs to be investigated further irrespective of the long periods between epidemics. The presence of a wide range of possible viral hosts including wildlife also needs to be explored. A few studies have demonstrated the presence of antibodies against Alphaviruses, for example, the detection of antibodies against CHIKV in serum sampled from non-human primates and antibodies against SINV in passerine species of birds (Citation24, Citation119). There is evidence of a few strains of SINV that have been distributed to South Africa from Sweden, most likely by passerine migratory birds (Citation12).
As most Alphaviruses are transmitted by mosquito vectors, there is a high possibility of propagation in diverse regions leading to outbreaks. The global spread of Alphaviruses may be attributed to the presence of diverse mosquito vectors; a wide range of reservoirs that amplifies these viruses in nature; the transmission of chikungunya by both sylvatic and urban cycles, which involve diverse Aedes species, non-human primates, and humans; and their ability to create new genetic variants by recombination. In addition, relocation of viraemic vertebrates as drivers of dissemination to geographical areas where the environment is compatible with endemisation has also contributed to wide distribution of these viruses. An example is SINV transmission, which is linked to the movement of migratory birds from South Africa to Europe and vice versa (Citation119).
Currently, there is a lack of vaccines for prevention and antiviral treatment of Alphavirus diseases (Citation13). The lack of vaccines and efficient treatment is possibly attributed to the fact that these outbreaks are sporadic and most of the viral diseases are self-limiting. For CHIKV, one of the most important Alphavirus, vaccines studies are initiated, but candidates need to be evaluated further (Citation120, Citation121). Meanwhile, prevention using other means is important. For instance, the cases of CHIKV in the Réunion Island began to rise in December 2005, and by May 2006, the disease had waned off (Citation13). The CHIKV outbreak response plan initiated by the citizens of Réunion Island is one of the strategies that made it possible for the disease to be managed and controlled (Citation122). This involved mass campaigns where reports of the suspect cases were brought to light, creation of a website where all matters concerning the disease were channelled, and community sensitisation and mobilisation through education. Regular surveillance systems should be put in place in endemic areas inclined towards these viruses to enable early detection including quantification of the burden of these diseases and prediction of future outbreaks before they arise. Therefore, there is a need for development of rapid and accurate (with high degree of sensitivity and specificity) diagnostic kits specific to Alphaviruses, which are cost-effective. This will assist in reducing the time and energy involved in performing potential differential diagnoses to rule out the presence or absence of arbovirus and parasitic pathogens in clinical samples.
Conclusions
The presence of diverse arthropod vectors and reservoir hosts (known and unknown) plays an important role in the circulation, transmission, and dissemination of emerging Alphaviruses across diverse geographical regions and over great distances. Ecological factors influence the transmission dynamics of these viruses by providing an environment for the virus, vector, host, and reservoir to thrive. The identification of an accurate case definition, diagnosis, and treatment of Alphaviruses remains a challenge due to the similar signs and symptoms they present with other arbovirus and parasitic diseases. A case in point is the uncertainty around a clinical distinction between the symptoms for chikungunya and dengue. The periodic nature of their epidemics and the relatively long periods between epidemics make it difficult for therapeutic options to be used. Whether Alphaviruses are maintained in reservoir hosts between epidemics needs to be investigated to guide the detection of these viruses, for appropriate prevention and control measures to be in place before they cause new outbreaks.
Conflict of interest and funding
The authors declare no conflict of interest.
Acknowledgements
This research was funded by the Swedish International Development Agency (grant no. SWE-2011-016) and the Swedish Research Council Formas (grant no. 221-2014-1556).
References
- Pfeffer M, Dobler G. Review emergence of zoonotic arboviruses by animal trade and migration. Parasites & Vectors. 2010; 3: 35. [PubMed Abstract] [PubMed CentralFull Text].
- Ulloa A, Langevin SA, Mendez-Sanchez J, Arredondo-Jimenez JI, Raetz JL, Powers AM, etal. Serologic survey of domestic animals for zoonotic arbovirus infections in the Lacandón Forest region of Chiapas, Mexico. Vector Borne Zoonotic Dis. 2003; 3: 3–9. [PubMed Abstract].
- Hubálek Z. Mosquito-borne viruses in Europe. Parasitol Res. 2008; 103: 29–43.
- Powers AM, Logue CH. Changing patterns of chikungunya virus: re-emergence of a zoonotic arbovirus. J Gen Virol. 2007; 88: 2363–77. [PubMed Abstract].
- Leung JY-S, Ng MM-L, Chu JJH. Replication of alphaviruses: a review on the entry process of alphaviruses into cells. Adv Virol. 2011; 2011: 249640. [PubMed Abstract] [PubMed CentralFull Text].
- Gould EA, Higgs S. Impact of climate change and other factors on emerging arbovirus diseases. Trans Roy Soc Trop Med Hyg. 2009; 103: 109–21. [PubMed Abstract] [PubMed CentralFull Text].
- Meltzer E. Arboviruses and viral hemorrhagic fevers (VHF). Infect Dis Clin North Am. 2012; 26: 479–96. [PubMed Abstract].
- Gould E, Coutard B, Malet H, Morin B, Jamal S, Weaver S, etal. Understanding the alphaviruses: recent research on important emerging pathogens and progress towards their control. Antiviral Res. 2010; 87: 111–24. [PubMed Abstract].
- Rulli NE, Melton J, Wilmes A, Ewart G, Mahalingam S. The molecular and cellular aspects of arthritis due to alphavirus infections. Ann N Y Acad Sci. 2007; 1102: 96–108. [PubMed Abstract].
- Weaver S, Rico-Hesse R, Scott T. Genetic diversity and slow rates of evolution in New World alphaviruses. Genetic diversity of RNA viruses. 1992. pp. 99–117.
- Martina BE, Osterhaus AD. 23. Wildlife and the risk of vector-borne viral diseases. Emerg Pests Vector Borne Dis Eur. 2007; 1: 411.
- Laine M, Luukkainen R, Toivanen A. Sindbis viruses and other alphaviruses as cause of human arthritic disease. J Intern Med. 2004; 256: 457–71. [PubMed Abstract].
- Suhrbier A, Jaffar-Bandjee M-C, Gasque P. Arthritogenic alphaviruses – an overview. Nat Rev Rheumatol. 2012; 8: 420–9. [PubMed Abstract].
- Toivanen A. Alphaviruses: an emerging cause of arthritis?. Curr Opin Rheumatol. 2008; 20: 486–90. [PubMed Abstract].
- Rezza G, Nicoletti L, Angelini R, Romi R, Finarelli A, Panning M, etal. Infection with chikungunya virus in Italy: an outbreak in a temperate region. Lancet. 2007; 370: 1840–6. [PubMed Abstract].
- Gubler DJ. The global emergence/resurgence of arboviral diseases as public health problems. Arch Med Res. 2002; 33: 330–42. [PubMed Abstract].
- Wilcox BA, Gubler DJ. Disease ecology and the global emergence of zoonotic pathogens. Environ Health Prev Med. 2005; 10: 263–72. [PubMed Abstract] [PubMed CentralFull Text].
- Russell RC. Mosquito-borne disease and climate change in Australia: time for a reality check. Aust J Entomol. 2009; 48: 1–7.
- Semenza JC, Menne B. Climate change and infectious diseases in Europe. Lancet Infect Dis. 2009; 9: 365–75. [PubMed Abstract].
- Gubler DJ, Reiter P, Ebi KL, Yap W, Nasci R, Patz JA. Climate variability and change in the United States: potential impacts on vector-and rodent-borne diseases. Environ Health Perspect. 2001; 109(Suppl 2): 223. [PubMed Abstract] [PubMed CentralFull Text].
- Atkins GJ. The pathogenesis of alphaviruses. ISRN Virol. 2012; 2013: 861912.
- Go YY, Balasuriya UB, Lee C-K. Zoonotic encephalitides caused by arboviruses: transmission and epidemiology of alphaviruses and flaviviruses. Clin Exp Vaccine Res. 2014; 3: 58–77. [PubMed Abstract] [PubMed CentralFull Text].
- Weaver SC, Reisen WK. Present and future arboviral threats. Antiviral Res. 2010; 85: 328–45. [PubMed Abstract] [PubMed CentralFull Text].
- Chhabra M, Mittal V, Bhattacharya D, Rana U, Lal S. Chikungunya fever: a re-emerging viral infection. Indian J Med Microbiol. 2008; 26: 5. [PubMed Abstract].
- Arankalle VA, Shrivastava S, Cherian S, Gunjikar RS, Walimbe AM, Jadhav SM, etal. Genetic divergence of chikungunya viruses in India (1963–2006) with special reference to the 2005–2006 explosive epidemic. J Gen Virol. 2007; 88: 1967–76. [PubMed Abstract].
- Singh P, Chhabra M, Mittal V, Sharma P, Rizvi MA, Chauhan LS, etal. Current research and clinical trials for a vaccine against chikungunya virus. Vaccine Dev Ther. 2013; 3: 35–46.
- Renault P, Balleydier E, D'Ortenzio E, Bâville M, Filleul L. Epidemiology of chikungunya infection on Reunion Island, Mayotte, and neighboring countries. Méd Mal Infect. 2012; 42: 93–101.
- Charrel RN, de Lamballerie X, Raoult D. Chikungunya outbreaks-the globalization of vectorborne diseases. N Engl J Med. 2007; 356: 769. [PubMed Abstract].
- Weaver SC, Forrester NL. Chikungunya: evolutionary history and recent epidemic spread. Antiviral Res. 2015; 120: 32–9. [PubMed Abstract].
- Van Bortel W, Dorleans F, Rosine J, Blateau A, Rousset D, Matheus S, etal. Chikungunya outbreak in the Caribbean region, December 2013 to March 2014, and the significance for Europe. Euro Surveill. 2014; 19: 20759. [PubMed Abstract].
- Leparc-Goffart I, Nougairede A, Cassadou S, Prat C, De Lamballerie X. Chikungunya in the Americas. Lancet. 2014; 383: 514. [PubMed Abstract].
- Nunes MR, Faria NR, de Vasconcelos JM, Golding N, Kraemer MU, de Oliveira LF, etal. Emergence and potential for spread of chikungunya virus in Brazil. BMC Med. 2015; 13: 102. [PubMed Abstract] [PubMed CentralFull Text].
- Kautz TF, Díaz-González EE, Erasmus JH, Malo-García IR, Langsjoen RM, Patterson EI, etal. Chikungunya virus as cause of febrile illness outbreak, Chiapas, Mexico, 2014. Emerg Infect Dis. 2015; 21: 2070–3. [PubMed Abstract] [PubMed CentralFull Text].
- Bessaud M, Peyrefitte CN, Pastorino BA, Gravier P, Tock F, Boete F, etal. O'nyong-nyong virus, Chad. Emerg Infect Dis. 2006; 12: 1248. [PubMed Abstract] [PubMed CentralFull Text].
- Vanlandingham DL, Hong C, Klingler K, Tsetsarkin K, McElroy KL, Powers AM, etal. Differential infectivities of O'nyong-nyong and chikungunya virus isolates in Anopheles gambiae and Aedes aegypti mosquitoes. Am J Trop Med Hyg. 2005; 72: 616–21. [PubMed Abstract].
- Lanciotti RS, Ludwig ML, Rwaguma EB, Lutwama JJ, Kram TM, Karabatsos N, etal. Emergence of epidemic O'nyong-nyong fever in Uganda after a 35-year absence: genetic characterization of the virus. Virology. 1998; 252: 258–68. [PubMed Abstract].
- Guyer B. Serological survey for arboviruses in Igbo-Ora, western Nigeria. Ann Trop Med Parasitol. 1972; 66: 243. [PubMed Abstract].
- Woodruff A. The dangerous virus infections. Virus Dis. 1979; 109
- Lhuillier M, Cunin P, Mazzariol M, Monteny N, Cordellier R, Bouchite B. Epidémie rurale à virus «Igbo Ora»(avec transmission inter-humaine) en Côte-d'Ivoire en 1984–1985. Bull Soc Pathol Exotique. 1988; 81: 386–95.
- Tappe D, Kapaun A, Emmerich P, de Mendonca Campos R, Cadar D, Günther S, etal. O'nyong-nyong virus infection imported to Europe from Kenya by a traveler. Emerg Infect Dis. 2014; 20: 1766. [PubMed Abstract] [PubMed CentralFull Text].
- Powers AM, Brault AC, Tesh RB, Weaver SC. Re-emergence of chikungunya and O'nyong-nyong viruses: evidence for distinct geographical lineages and distant evolutionary relationships. J Gen Virol. 2000; 81: 471–9. [PubMed Abstract].
- Taylor R, Hurlbut H, Work T, Kingston J, Frothingham T. Sindbis virus: a newly recognized arthropod-transmitted virus. Am J Trop Med Hyg. 1955; 4: 844–62. [PubMed Abstract].
- Woodall J, Williams M, Ellice J. Sindbis infection in man. East Afr Virus Res Inst Rep . 1962; 12: 17.
- Storm N, Weyer J, Markotter W, Kemp A, Leman P, Dermaux-Msimang V, etal. Human cases of Sindbis fever in South Africa, 2006–2010. Epidemiol Infect. 2014; 142: 234–8. [PubMed Abstract].
- Kurkela S, Manni T, Myllynen J, Vaheri A, Vapalahti O. Clinical and laboratory manifestations of Sindbis virus infection: prospective study, Finland, 2002–2003. J Infect Dis. 2005; 191: 1820–9. [PubMed Abstract].
- Lundström JO. Mosquito-borne viruses in Western Europe: a review. J Vector Ecol. 1999; 24: 1–39.
- Bergqvist J, Forsman O, Larsson P, Näslund J, Lilja T, Engdahl C, etal. Detection and isolation of Sindbis virus from mosquitoes captured during an outbreak in Sweden, 2013. Vector Borne Zoonotic Dis. 2015; 15: 133–40. [PubMed Abstract].
- Brummer-Korvenkontio M, Vapalahti O, Kuusisto P, Saikku P, Manni T, Koskela P, etal. Epidemiology of Sindbis virus infections in Finland 1981–96: possible factors explaining a peculiar disease pattern. Epidemiol Infect. 2002; 129: 335–45. [PubMed Abstract] [PubMed CentralFull Text].
- Lundström JO, Pfeffer M. Phylogeographic structure and evolutionary history of Sindbis virus. Vector Borne Zoonotic Dis. 2010; 10: 889–907.
- Crabtree M, Sang R, Lutomiah J, Richardson J, Miller B. Arbovirus surveillance of mosquitoes collected at sites of active Rift Valley fever virus transmission: Kenya, 2006–2007. J Med Entomol. 2009; 46: 961–4. [PubMed Abstract].
- Miller BR, Nasci RS, Godsey MS, Savage HM, Lutwama JJ, Lanciotti RS, etal. First field evidence for natural vertical transmission of West Nile virus in Culex univittatus complex mosquitoes from Rift Valley province, Kenya. Am J Trop Med Hyg. 2000; 62: 240–6. [PubMed Abstract].
- Karabatsos N. International catalogue of arthropod-borne viruses. 1985; San Antonio, TX: American Society for Tropical Medicine and Hygiene. 3.
- Ochieng C, Lutomiah J, Makio A, Koka H, Chepkorir E, Yalwala S, etal. Mosquito-borne arbovirus surveillance at selected sites in diverse ecological zones of Kenya; 2007–2012. Virol J. 2013; 10: 140. [PubMed Abstract] [PubMed CentralFull Text].
- Westhorpe C. Ross River virus. Geodate. 2014; 27: 4.
- Doherty R, Whitehead R, Gorman B, O'gower A. The isolation of a third group A arbovirus in Australia, with preliminary observations on its relationship to epidemic polyarthritis. Aust J Sci. 1963; 26: 183–4.
- Harley D, Sleigh A, Ritchie S. Ross River virus transmission, infection, and disease: a cross-disciplinary review. Clin Microbiol Rev. 2001; 14: 909–32. [PubMed Abstract] [PubMed CentralFull Text].
- Aaskov J, Mataika J, Lawrence G, Rabukawaqa V, Tucker M, Miles J, etal. An epidemic of Ross River virus infection in Fiji, 1979. Am J Trop Med Hyg. 1981; 30: 1053–9. [PubMed Abstract].
- Rosen L, Gubler DJ, Bennett PH. Epidemic polyarthritis (Ross River) virus infection in the Cook Islands. Am J Trop Med Hyg. 1981; 30: 1294–302. [PubMed Abstract].
- Mourão MPG, Bastos Mde S, de Figueiredo RP, Gimaque JBL, dos Santos Galusso E, Kramer VM, etal. Mayaro fever in the city of Manaus, Brazil, 2007–2008. Vector Borne Zoonotic Dis. 2012; 12: 42–6.
- Anderson CR, Downs WG, Wattley GH, Ahin NW, Reese AA. Mayaro virus: a new human disease agent. II. Isolation from blood of patients in Trinidad, BW I. Am J Trop Med Hyg. 1957; 6: 1012–16. [PubMed Abstract].
- Causey OR, Maroja OM. Mayaro virus: a new human disease agent. III. Investigation of an epidemic of acute febrile illness on the river Guama in Pará, Brazil, and isolation of Mayaro virus as causative agent. Am J Trop Med Hyg. 1957; 6: 1017. [PubMed Abstract].
- Schaeffer M, Gajdusek DC, Lema AB, Eichenwald H. Epidemic jungle fevers among Okinawan Colonists in the Bolivian rain forest I. Epidemiology. Am J Trop Med Hyg. 1959; 8: 372–96. [PubMed Abstract].
- Pinheiro FP, Freitas RB, Travassos dRJ, Gabbay YB, Mello WA, LeDuc JW. An outbreak of Mayaro virus disease in Belterra, Brazil. I. Clinical and virological findings. Am J Trop Med Hyg. 1981; 30: 674–81. [PubMed Abstract].
- Azevedo RS, Silva EV, Carvalho VL, Rodrigues SG, Neto JPN, Monteiro HA, etal. Mayaro fever virus, Brazilian Amazon. Emerg Infect Dis. 2009; 15: 1830. [PubMed Abstract] [PubMed CentralFull Text].
- Weaver SC, Frolov IV. Mahy BWJ, Meulen V. Togaviruses. Topley and Wilson's microbiology and microbial infections. Virology, vol. 2 . 2005; Salisbury, United Kingdom: IRL Press. 1010–24.
- Volk SM, Chen R, Tsetsarkin KA, Adams AP, Garcia TI, Sall AA, etal. Genome-scale phylogenetic analyses of chikungunya virus reveal independent emergences of recent epidemics and various evolutionary rates. J Virol. 2010; 84: 6497–504. [PubMed Abstract] [PubMed CentralFull Text].
- Caglioti C, Lalle E, Castilletti C, Carletti F, Capobianchi MR, Bordi L. Chikungunya virus infection: an overview. New Microbiol. 2013; 36: 211–27. [PubMed Abstract].
- Lutwama JJ, Kayondo J, Savage HM, Burkot TR, Miller BR. Epidemic O'nyong-nyong fever in southcentral Uganda, 1996–1997: entomologic studies in Bbaale village, Rakai District. Am J Trop Med Hyg. 1999; 61: 158–62. [PubMed Abstract].
- Seymour RL, Rossi SL, Bergren NA, Plante KS, Weaver SC. The role of innate versus adaptive immune responses in a mouse model of O'nyong-nyong virus infection. Am J Trop Med Hyg. 2013; 88: 1170–9. [PubMed Abstract] [PubMed CentralFull Text].
- Lundström JO, Niklasson B, Francy DB. Swedish Culex torrentium and C. pipiens (Diptera: Culicidae) as experimental vectors of Ockelbo virus. J Med Entomol. 1990; 27: 561–3.
- Turell MJ, Lundström JO, Niklasson B. Transmission of Ockelbo Virus by Aedes cinereus, A. communis, and A. excrucians (Diptera: Culicidae) Collected in an Enzootic Area in Central Sweden. J Med Entomol. 1990; 27: 266–8. [PubMed Abstract].
- Francy DB, Jaenson T, Lundström JO, Schildt E-B, Espmark A, Henriksson B, etal. Ecologic studies of mosquitoes and birds as hosts of Ockelbo virus in Sweden and isolation of Inkoo and Batai viruses from mosquitoes. Am J Trop Med Hyg. 1989; 41: 355–63. [PubMed Abstract].
- McIntosh B, Dickinson D, McGillivray G. Ecological studies on Sindbis and West Nile viruses in South Africa. V. The response of birds to inoculation of virus. S Afr J Med Sci. 1969; 34: 77. [PubMed Abstract].
- Lundström JO, Lindström KM, Olsen B, Dufva R, Krakower DS. Prevalence of Sindbis virus neutralizing antibodies among Swedish passerines indicates that thrushes are the main amplifying hosts. J Med Entomol. 2001; 38: 289–97.
- Lundström JO, Turell MJ, Niklasson B. Antibodies to Ockelbo virus in three orders of birds (Anseriformes, Galliformes and Passeriformes) in Sweden. J Wildlife Dis. 1992; 28: 144–7.
- Russell RC. Ross River virus: ecology and distribution. Ann Rev Entomol. 2002; 47: 1–31.
- Tompkins DM, Slaney D. Exploring the potential for Ross River virus emergence in New Zealand. Vector Borne Zoonotic Dis. 2014; 14: 141–8. [PubMed Abstract].
- Mair T, Timoney P. Ross River virus. EVE Man 08-046 Mair v2:Layout 1 14/08/2009 13:10. 159–162. www.bellequine.co.uk.
- Kay BH, Boyd AM, Ryan PA, Hall RA. Mosquito feeding patterns and natural infection of vertebrates with Ross River and Barmah Forest viruses in Brisbane, Australia. Am J Trop Med Hyg. 2007; 76: 417–23. [PubMed Abstract].
- Claflin SB, Webb CE. Ross River virus: many vectors and unusual hosts make for an unpredictable pathogen. PLoS Pathog. 2015; 11: e1005070. [PubMed Abstract] [PubMed CentralFull Text].
- Halsey ES, Siles C, Guevara C, Vilcarromero S, Jhonston EJ, Ramal C, etal. Mayaro virus infection, Amazon basin region, Peru, 2010–2013. Emerg Infect Dis. 2013; 19: 1839. [PubMed Abstract] [PubMed CentralFull Text].
- Tsetsarkin KA, Vanlandingham DL, McGee CE, Higgs S. A single mutation in chikungunya virus affects vector specificity and epidemic potential. PLoS Pathog. 2007; 3: e201. [PubMed Abstract] [PubMed CentralFull Text].
- Stoler J, al Dashti R, Anto F, Fobil JN, Awandare GA. Deconstructing “malaria”: West Africa as the next front for dengue fever surveillance and control. Acta Trop. 2014; 134: 58–65. [PubMed Abstract].
- Carey DE. Chikungunya and dengue: a case of mistaken identity?. J Hist Med Allied Sci. 1971; 26: 243–62. [PubMed Abstract].
- Lee VJ, Chow A, Zheng X, Carrasco LR, Cook AR, Lye DC, etal. Simple clinical and laboratory predictors of Chikungunya versus dengue infections in adults. PLoS Negl Trop Dis. 2012; 6: e1876. doi: http://dx.doi.org/10.1371/journal.pntd.0001786.
- Kurkela S, Helve T, Vaheri A, Vapalahti O. Arthritis and arthralgia three years after Sindbis virus infection: clinical follow-up of a cohort of 49 patients. Scand J Infect Dis. 2008; 40: 167–73. [PubMed Abstract].
- Borgherini G, Poubeau P, Staikowsky F, Lory M, Le Moullec N, Becquart JP, etal. Outbreak of chikungunya on Reunion Island: early clinical and laboratory features in 157 adult patients. Clin Infect Dis. 2007; 44: 1401–7. [PubMed Abstract].
- Condon RJ, Rouse IL. Acute symptoms and sequelae of Ross River virus infection in South-Western Australia: a follow-up study. Clin Diagn Virol. 1995; 3: 273–84. [PubMed Abstract].
- Brouard C, Bernillon P, Quatresous I, Pillonel J, Assal A, De Valk H, etal. Estimated risk of Chikungunya viremic blood donation during an epidemic on Reunion Island in the Indian Ocean, 2005 to 2007. Transfusion. 2008; 48: 1333–41. [PubMed Abstract].
- Thiberville S-D, Moyen N, Dupuis-Maguiraga L, Nougairede A, Gould EA, Roques P, etal. Chikungunya fever: epidemiology, clinical syndrome, pathogenesis and therapy. Antiviral Res. 2013; 99: 345–70. [PubMed Abstract].
- Posey DL, O'rourke T, Roehrig JT, Lanciotti RS, Weinberg M, Maloney S. O'nyong-nyong fever in West Africa. Am J Trop Med Hyg. 2005; 73: 32. [PubMed Abstract].
- Jardine A, Speldewinde P, Lindsay MD, Cook A, Johansen CA, Weinstein P. Is there an association between dryland salinity and Ross River virus disease in southwestern Australia?. EcoHealth. 2008; 5: 58–68. [PubMed Abstract].
- Simon F, Parola P, Grandadam M, Fourcade S, Oliver M, Brouqui P, etal. Chikungunya infection: an emerging rheumatism among travelers returned from Indian Ocean islands. Report of 47 cases. Medicine. 2007; 86: 123–37. [PubMed Abstract].
- Schilte C, Staikowsky F, Couderc T, Madec Y, Carpentier F, Kassab S, etal. Chikungunya virus-associated long-term arthralgia: a 36-month prospective longitudinal study. PLoS Negl Trop Dis. 2013; 7: e2137. [PubMed Abstract] [PubMed CentralFull Text].
- Greiser-Wilke I, Moennig V, Kaaden O, Shope R. Detection of alphaviruses in a genus-specific antigen capture enzyme immunoassay using monoclonal antibodies. J Clin Microbiol. 1991; 29: 131–7. [PubMed Abstract] [PubMed CentralFull Text].
- Pialoux G, Gaüzère B-A, Jauréguiberry S, Strobel M. Chikungunya, an epidemic arbovirosis. Lancet Infect Dis. 2007; 7: 319–27. [PubMed Abstract].
- Van den Hurk AF, Hall-Mendelin S, Pyke AT, Frentiu FD, McElroy K, Day A, etal. Impact of Wolbachia on infection with chikungunya and yellow fever viruses in the mosquito vector Aedes aegypti. PLoS Neglected Tropical Diseases. 2012; 6: e1892. doi: http://dx.doi.org/10.1371/journal.pntd.0001892 [PubMed Abstract] [PubMed CentralFull Text].
- Hall RA, Blitvich BJ, Johansen CA, Blacksell SD. Advances in arbovirus surveillance, detection and diagnosis. BioMed Res Int. 2012; 2012: 512969.
- Blackburn N, Besselaar T, Gibson G. Antigenic relationship between chikungunya virus strains and o'nyong nyong virus using monoclonal antibodies. Res Virol. 1995; 146: 69–73. [PubMed Abstract].
- Powers A, Roehrig JT. Alphaviruses. Diagnostic virology protocols. 2011. pp. 17–38.
- Kong YY, Thay CH, Tin TC, Devi S. Rapid detection, serotyping and quantitation of dengue viruses by TaqMan real-time one-step RT-PCR. J Virol Methods. 2006; 138: 123–30. [PubMed Abstract].
- Sánchez-Seco MP, Rosario D, Quiroz E, Guzmán G, Tenorio A. A generic nested-RT-PCR followed by sequencing for detection and identification of members of the alphavirus genus. J Virol Methods. 2001; 95: 153–61.
- Weaver SC, Winegar R, Manger ID, Forrester NL. Alphaviruses: population genetics and determinants of emergence. Antiviral Res. 2012; 94: 242–57. [PubMed Abstract] [PubMed CentralFull Text].
- Tucker PC, Strauss E, Kuhn R, Strauss J, Griffin D. Viral determinants of age-dependent virulence of Sindbis virus for mice. J Virol. 1993; 67: 4605–10. [PubMed Abstract] [PubMed CentralFull Text].
- Griffin DE. A review of alphavirus replication in neurons. Neurosci Biobehav Rev. 1998; 22: 721–3. [PubMed Abstract].
- Davis NL, Fuller FJ, Dougherty WG, Olmsted RA, Johnston RE. A single nucleotide change in the E2 glycoprotein gene of Sindbis virus affects penetration rate in cell culture and virulence in neonatal mice. Proc Natl Acad Sci. 1986; 83: 6771–5. [PubMed Abstract] [PubMed CentralFull Text].
- Lanciotti RS, Valadere AM. Transcontinental movement of Asian genotype chikungunya virus. Emerg Infect Dis. 2014; 20: 1400. [PubMed Abstract] [PubMed CentralFull Text].
- Laras K, Sukri NC, Larasati RP, Bangs MJ, Kosim R, Wandra T, etal. Tracking the re-emergence of epidemic chikungunya virus in Indonesia. Trans Roy Soc Trop Med Hyg. 2005; 99: 128–41. [PubMed Abstract].
- Teixeira MG, Andrade AM, Maria da Conceição NC, Castro JS, Oliveira FL, Goes CS, etal. East/Central/South African Genotype Chikungunya Virus, Brazil, 2014. Emerg Infect Dis. 2015; 21: 906. [PubMed Abstract] [PubMed CentralFull Text].
- Shirako Y, Niklasson B, Dalrymple JM, Strauss EG, Strauss JH. Structure of the Ockelbo virus genome and its relationship to other Sindbis viruses. Virology. 1991; 182: 753–64. [PubMed Abstract].
- Faragher S, Hutchison C, Dalgarno L. Analysis of Ross River virus genomic RNA using HaeIII digests of single-stranded cDNA to infected-cell RNA and virion RNA. Virology. 1985; 141: 248–56. [PubMed Abstract].
- Sammels LM, Coelen RJ, Lindsay MD, Mackenzie JS. Geographic distribution and evolution of Ross River virus in Australia and the Pacific Islands. Virology. 1995; 212: 20–9. [PubMed Abstract].
- Wells P, Mikunda A, Doggett S, Cloonan M, Russell R, Weir R, etal. Studies of the infectivity of selected strains of Ross River virus in Aedes vigilax and Culex annulirostris collected at coastal and inland sites of New South Wales. Arbovirus Res Aust. 1997; 7: 322–5.
- Woodroofe G, Marshall ID, Taylor WP. Antigenically distinct strains of Ross River virus from north Queensland and coastal New South Wales. Aust J Exp Biol Med Sci. 1977; 55: 79–87. [PubMed Abstract].
- Powers AM, Aguilar PV, Chandler LJ, Brault AC, Meakins TA, Watts D, etal. Genetic relationships among Mayaro and Una viruses suggest distinct patterns of transmission. Am J Trop Med Hyg. 2006; 75: 461–9. [PubMed Abstract].
- Tamura K, Nei M. Estimation of the number of nucleotide substitutions in the control region of mitochondrial DNA in humans and chimpanzees. Mol Biol Evol. 1993; 10: 512–26. [PubMed Abstract].
- Tamura K, Stecher G, Peterson D, Filipski A, Kumar S. MEGA6: molecular evolutionary genetics analysis version 6.0. Mol Biol Evol. 2013; 30: 2725–9. [PubMed Abstract] [PubMed CentralFull Text].
- McMichael AJ, Woodruff RE. Climate change and infectious diseases 14. Soc Ecol Infect Dis. 2011; 378: 378–407.
- Kurkela S, Rätti O, Huhtamo E, Uzcátegui NY, Nuorti JP, Laakkonen J, etal. Sindbis virus infection in resident birds, migratory birds, and humans, Finland. Emerg Infect Dis. 2008; 14: 41. [PubMed Abstract] [PubMed CentralFull Text].
- Chang L-J, Dowd KA, Mendoza FH, Saunders JG, Sitar S, Plummer SH, etal. Safety and tolerability of chikungunya virus-like particle vaccine in healthy adults: a phase 1 dose-escalation trial. Lancet. 2014; 384: 2046–52. [PubMed Abstract].
- Rudd PA, Raphael AP, Yamada M, Nufer KL, Gardner J, Le TT, etal. Effective cutaneous vaccination using an inactivated chikungunya virus vaccine delivered by Foroderm. Vaccine. 2015; 33: 5172–80. [PubMed Abstract].
- Gaüzère B-A, Mausole J-H, Simon F. Citizens’ actions in response to chikungunya outbreaks, Réunion Island. Emerging Infectious Diseases. 2006; 21 doi: http://dx.doi.org/10.3201/eid2105.141385.