Abstract
Background
Campylobacter jejuni is the leading bacterial food-borne pathogen within the European Union (EU), and poultry meat is the primary route for transmission to humans.
Material and methods
This study examined the impact of partial depopulation (thinning), season, and farm performance (economic, hygiene, and biosecurity) on Campylobacter prevalence in Irish broilers over a 13-month period. Ten caecal samples were taken per flock, for a total of 211 flocks from 23 farms during the duration of the study. Campylobacter was isolated and enumerated according to modified published ISO methods for veterinary samples. Biosecurity was evaluated through a questionnaire based on risk factors for Campylobacter identified in previous studies. Hygiene compliance was assessed from audit records taken over the course of 1 year. All information relating to biosecurity and hygiene was obtained directly from the processing company. This was done to ensure farmers were unaware they were being monitored for Campylobacter prevalence and prevent changes to their behaviour.
Results and discussion
Farms with high performance were found to have significantly lower Campylobacter prevalence at first depopulation compared with low-performance farms across all seasons (P≤0.01). Peak Campylobacter levels were observed during the summer season at first thin in both the high- and low-performance groups. Campylobacter prevalence was found to increase to ≥85% in both high- and low-performance farms across all seasons at final depopulation, suggesting that Campylobacter was introduced during the first depopulation. On low-performance farms, four biosecurity interventions were found to significantly reduce the odds of a flock being Campylobacter positive (physical step-over barrier OR=0.17, house-specific footwear OR=0.13, absence of water body within 0.5 km OR=0.13, two or more broiler houses on a farm OR=0.16), compared with farms without these interventions. For high-performance farms, no single biosecurity intervention was identified as significant as this group had full compliance with multiple factors. High-performance farms had significantly better feed conversion ratios compared with low-performance farms (1.61 v 1.67 (P≤0.01)). No differences in flock mortality rates were observed (P≥0.05). This highlights the impact of season, biosecurity, partial depopulation, and farm performance on Campylobacter prevalence in Irish broilers.
There are approximately 9.4 million cases of campylobacteriosis per annum within the European Union (EU) causing an estimated 0.35 million quality adjusted life years (QALYs) and costing €2.4 billion each year (Citation1). In 2014, the European Food Safety Authority (EFSA) and European Centre for Disease Control (ECDC) reported 236,851 confirmed human campylobacteriosis cases within the EU. Campylobacter was the most commonly detected bacterial food-borne pathogen in humans with the primary source being broiler meat (Citation2). Irish broiler batches and carcasses had the fourth and second highest prevalences of Campylobacter contamination, respectively, within the EU (Citation3, Citation4). This is of particular concern as, in 2014, the farm gate value of the Irish broiler industry was approximately €133 million (Citation5). Given the high levels of Campylobacter contamination reported on Irish poultry carcasses, there is a need for integrated interventions in the poultry production chain to reduce the public health risk (Citation6).
Previous studies have demonstrated that strictly adhering to on-farm biosecurity practices can reduce Campylobacter prevalence by up to 50% at first slaughter (Citation7–Citation9). However, Katsma et al. (Citation8) have suggested that it is difficult to identify specific control measures that consistently reduce Campylobacter prevalence, making it difficult to recommend the specific investments needed. Denmark had the lowest Campylobacter prevalence in broiler flocks (11%) in the EU in 2013, which it controls with a strict on-farm biosecurity and hygiene programme (Citation2). Previous research has shown interventions at farm level, such as fly screens, house-specific boots/clothing, physical step-over barriers, and regular reminders to farmers to focus on biosecurity, can reduce on-farm Campylobacter prevalence (Citation7, Citation9) (Citation10). Another potential impediment to Campylobacter control on Irish broiler farms is the common practice of partial depopulation or ‘thinning’. It has been suggested that difficulties in maintaining biosecurity during thinning may allow for Campylobacter introduction (Citation11–Citation15).
The objectives of this study were to compare: 1) biosecurity measures on broiler farms within an integrated broiler processing company in the Republic of Ireland, 2) the differences in Campylobacter prevalence between farms with high hygiene and economic performance versus farms with low performance, and 3) the differences in Campylobacter prevalence between flocks at first and final thin.
Materials and methods
Farm selection
Growing farms, contracted to a large integrated broiler processor in the Republic of Ireland, were considered for inclusion in the study. The economic performance of each farm was assessed based on the gross income per thousand birds for 2013–2014. Farms in the top and bottom 10% (n=129) of economic performers were then evaluated for hygiene performance. Farm hygiene audit records for 2013–2014 (January–January) from all of the selected farms were analysed in order to identify farms with the highest and lowest levels of compliance with hygiene and disinfection practices. Twelve farms, which had full compliance with hygiene audits over the previous year and were in the top 10% of economic performers, were selected for inclusion in this study and classified as ‘high-performance’ farms. Twelve farms that had at least one hygiene non-compliance in at least three broiler cycles over the course of the previous 12 months and were in the bottom 10% of economic performers were also selected and classified as ‘low-performance’ farms. One of these low-performance farms subsequently withdrew from the study.
Biosecurity assessment
Each farm was assessed for compliance with 22 recognised biosecurity criteria by completion of a questionnaire by farm auditors employed by the processor. Multiple factors, such as the presence or absence of step-over barriers, concrete aprons at the front of houses, the use of house-specific footwear, and proximity to other livestock, were assessed in the questionnaire (Citation7, Citation9) (Citation10, Citation16) (Citation17) (). Based on this assessment, each farm was awarded an overall biosecurity score ranging from 0 (no compliance with any biosecurity criteria) to 22 (compliance with all biosecurity criteria), and a total biosecurity score for each performance group was calculated.
Table 1 Odds ratios and 95% confidence intervals (CIs) for Campylobacter positivity on farms with and without specific biosecurity criteria at first thin
All audit and questionnaire information to evaluate farm compliance/structural biosecurity was sourced directly from the processing company and caeca were collected directly from the slaughter plant. This minimised the risk of farmers consciously changing management practices that could have introduced bias into the study.
Campylobacter isolation and enumeration
In total, 123 flocks at first thin (bird age 33–35 days) and 88 flocks at final thin (age 41–42 days) were monitored over the course of 13 months from May 2014 to May 2015. Ten caecal samples were collected immediately after slaughter from birds in each flock. A 1 g sample of caecal contents was aseptically removed from each of the 10 birds per batch and was serially diluted and plated onto modified charcoal deoxycholate agar (mCCDA) (Oxoid, Hampshire, United Kingdom), according to a previously published protocol for caecal samples and based on the ISO enumeration method (Citation13). Plates were then incubated microaerobically (5–10% O2; 10% CO2) for 48 hours at 37°C, colonies counted, and the log values calculated. Isolates were confirmed as Campylobacter and speciated using a previously published PCR method (Citation18). Mean Campylobacter concentrations and within-flock prevalence were calculated based on 10 caecal samples per flock.
Evaluation of flock performance indicators (feed conversion ratio and percentage of bird mortality)
Data records containing feed conversion ratios (FCR), the percentage of bird mortality, and economic performance (gross income per thousand birds) were collected from the processing plant for the last six flocks raised on each selected farm over the course of 1 year.
Temperature
All farms were within 50 km of an Irish Meteorological Service weather monitoring station. The data for mean maximum and minimum recorded temperatures were obtained from this station for each month of the sampling period.
Statistical analysis
Campylobacter prevalence was calculated for each day in the high- and low-performance groups using the equation:
[(total number positive samples from all farms in each group÷total numbers of samples from all farms in each group)×(100) per day].
Wald 95% confidence intervals (95% CIs) were constructed for Campylobacter prevalence and mean Campylobacter concentrations. Campylobacter prevalence was calculated on a daily basis for both high- and low-performance groups at both their first and final slaughter points. A Mann-Whitney (MW) (Citation19) test was then performed to compare Campylobacter prevalences between: 1) high- versus low-performance farms at first thin, 2) high- versus low-performance farms at final thin, 3) first versus final thin in high-performance farms and 4) first versus final thin in low-performance farms. The MW test was also used to compare total biosecurity scores between high- and low-performance farms. The MW U (MWU-statistic) was used to determine the probability that a random chosen sample taken on any given day from a high-performance farm would have lower Campylobacter prevalence than a sample obtained from a low-performance farm. The MWU was also used to compare total biosecurity scores from high- and low-performance farms. Ninety-five percent CIs were also constructed for probabilities obtained using the MWU-statistic using the following formula:
where
where
n 1=the number of observations in group 1, n 2=the number of observations in group 2, θ=U/n 1 n 2 (the probability), U=MWU-statistic; Q 1=θ/(2−θ) and Q 2=(2 θ 2)/(1+θ) (Citation20).
An odds ratio (OR) according to Jewell (Citation21), was used to calculate the relative odds that farms meeting the biosecurity criteria were Campylobacter positive compared to farms that did not meet the criteria, within each of the high- and low-performance sample populations (). Ninety-five percent CIs were also constructed for all ORs calculated. In cases in which all farms met the biosecurity criteria, an OR could not be calculated (Citation21).
A one-way ANOVA was used to compare mean FCR and percentage mortality rates between high- and low-performance farms.
Finally, a two-tailed t-test assuming unequal variance was used to test for significant differences in mean Campylobacter concentrations between high- and low-performance farms at both first and final slaughter. For all comparisons, P values<0.05 were considered statistically significant.
Results
Biosecurity assessment
The high-performance farm group had a significantly higher total biosecurity score (S=205) (P<0.05) compared with the low-performance farm group (S=154).The probability that a farm randomly selected from the high-performance group would have a higher total biosecurity score than a farm randomly selected in the low-performance group was 76% (95% CI: 55–96%).
Four biosecurity criteria were significantly (P<0.05) associated with reduced odds of Campylobacter positivity on low-performance farms: 1) presence of a physical step-over barrier (OR=0.17), 2) use of house-specific footwear (OR=0.13), 3) two or fewer broiler houses on farm (OR=0.16), and 4) absence of a water body within 0.5 km of the farm (OR=0.13). In the high-performance farms, no single biosecurity criterion was found to significantly reduce the odds of a farm being Campylobacter positive. However, on these farms, full compliance with several biosecurity criteria was observed ().
Campylobacter prevalence in high- and low-performance farms at first thin
A statistically significant difference (P<0.01) in daily Campylobacter prevalences was found between high- and low-performance farms at first thin. There was a 64% (95% CI: 53–76%) probability that a sample from the high-performance group would have a lower Campylobacter prevalence than a sample from the low-performance group on any given day.
There was a statistically significant difference in Campylobacter prevalence between high- and low-performance farms (P<0.01) across all seasons at first thin. Campylobacter prevalence on high-performance farms was 22% (95% CI: 16–28%) in May 2014, 53% (95% CI: 45–61%) in summer [June–August], 37% (95% CI: 30–44%) in autumn [September–November], 6% (95% CI: 9–12%) in winter [December–February], and 16% (95% CI: 9–23%) in spring 2015 [March–May], compared with 45% (95% CI: 32–58%), 92% (95% CI: 87–96%), 70% (95% CI: 63–77%), 29% (95% CI: 18–39%), and 38% (95% CI: 30–47%), respectively, in low-performance farms at first thin (a).
Fig. 1 Comparisons of Campylobacter prevalence between high- and low-performance farms at a first and b final thin.
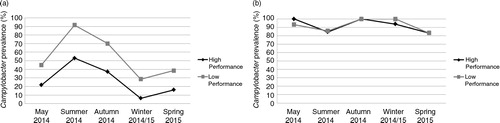
A statistically significant difference was also found in the mean Campylobacter concentrations of positive samples (P≤0.01) between high [7.5 (95% CI: 7.3–7.7) log10 CFU/g] and low [8.3 (95% CI: 8.2–8.4) log10 CFU/g] performance farms at first thin.
Campylobacter prevalence in high- and low-performance farms at final thin
There was no statistically significant difference (P>0.05) in Campylobacter prevalence between high- and low-performance farms at final thin. In addition, no significant differences were found in mean Campylobacter concentrations between high-performance [8.4 (95% CI: 8.3–8.5) log10 CFU/g] and low-performance [8.3 (95% CI: 8.2–8.4) log10 CFU/g] farms at final thin. At final thin mean Campylobacter prevalence increased to ≥85% in both groups of farms across all seasons (b).
Differences in Campylobacter prevalence between first and final thin
Campylobacter prevalence significantly (P<0.01) increased at final thin when compared with first thin in high-performance farms across all seasons. The probability of a high-performance farm having a lower Campylobacter prevalence on any given day at first thin compared with final thin was 83% (95% CI: 74–92%). In high-performance farms, Campylobacter prevalences ranged from 22% (95% CI: 16–28%) in May 2014, 53% (95% CI: 45–61%) in summer, 37% (95% CI: 30–44%) in autumn, 6% (95% CI: 1–12%) in winter, and 16% (95% CI: 9–23%) in spring 2015 at first thin to ≥85% across all seasons at final thin (a).
Fig. 2 Comparisons of Campylobacter prevalence between first and final thin in a high- and b low-performance farms.
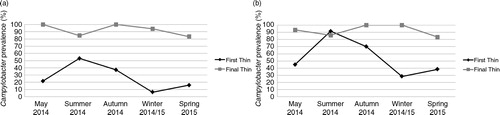
A similar effect was observed in low-performance farms: the probability that Campylobacter prevalence would be lower at first thin than final thin on any given day was 69% (95% CI: 57–81%). Campylobacter prevalence ranged from 45% (95% CI: 32–58%) in May 2014, 92% (95% CI: 87–96%) in summer, 70% (95% CI: 63–77%) in autumn, 29% in winter (95% CI: 19–39%), and 38% (95% CI: 30–47%) in spring 2015 at first thin to ≥85% across all seasons at final thin (b).
Mean overall Campylobacter concentrations in positive samples were significantly higher (P<0.05) at final thin [8.4 (95% CI: 8.3–8.5) log10 CFU/g] than at first thin [7.5 (95% CI: 7.3–7.7) log10 CFU/g] in samples from high-performance but not low-performance farms.
Economic performance, feed conversion ratio, and percentage of bird mortality
There was a significant difference (P<0.01) in economic performance and FCR between high- and low-performance farms but no significant difference (P>0.05) in the percentage of bird mortality. The mean difference in gross income per thousand birds between high- and low-performance farms was €107 (95% CI: €94–122). The annual average FCR (kg feed consumed per kg of bird weight produced) was significantly lower in high-performance (P=1.61; 95% CI: 1.61–1.63) than in low-performance (P=1.67; 95% CI: 1.65–1.67) farms. The average farm FCRs ranged between 1.59 and 1.70.
Temperature
Mean temperatures varied greatly with season. Highest temperatures were observed in July 2014. The highest average maximum (20°C) and minimum (10°C) temperatures were recorded in July 2014. The lowest average maximum (7.2°C) and minimum (1.1°C) temperatures were both recorded in February 2015 ().
Discussion
Campylobacter in Irish poultry meat has a high socio-economic cost in terms of QALYs, health care expenditure costs, and lost workdays. The EFSA state that 50–80% of human campylobacteriosis cases may be attributed to the broiler reservoir as a whole (Citation22). The disease burden of campylobacteriosis is estimated to cost the EU 0.35 million disability adjusted life years (DALYs) per year with total annual costs of approximately €2.4 billion (Citation1). In 2014, Campylobacter was the most commonly reported bacterial food-borne pathogen in Europe with 236,851 confirmed cases, however, these figures are considered vastly under-reported (Citation2). This highlights the importance of reducing the burden of disease in poultry through good on-farm hygiene and biosecurity which, in turn, will reduce incidence in humans giving both economic and human health benefits.
Farms within the high-performance group had a significantly higher total overall biosecurity score than those in the low-performance group. In some cases, the OR for Campylobacter positivity could not be calculated because all farms within the performance groups complied with the particular criterion. However, although a ratio could not be calculated, the finding that high-performance farms had full compliance with certain criteria identified as significant in the low-performance group (physical step-over barriers, and house-specific footwear) may explain the lower Campylobacter prevalences observed in high-performance farms.
Four biosecurity criteria (house-specific footwear, physical step-over barrier, absence of water body within 0.5 km, and two or more broiler houses on a farm) were associated with reduced odds of Campylobacter in the low-performance group. A previous study by Hald et al. (Citation23) similarly found that a physical step-over barrier was the single most important biosecurity measure for the production of Campylobacter free flocks. In addition, other studies have also highlighted that the use of house-specific footwear can lower the risk of Campylobacter colonisation of broiler poultry (Citation16, Citation24). A previous study by Humphrey et al. (Citation25) noted that the proper use of foot dip could delay colonisation with Campylobacter by 2–3 weeks as compared with farms that did not use foot dips, suggesting that contaminated footwear is an important source for Campylobacter colonisation of broilers. High standards of biosecurity implemented on farm can reduce the risk of Campylobacter introduction and transmission to and between flocks. Water has also been identified as a potential reservoir for Campylobacter (Citation26) whilst a higher number of broiler houses on farms has also been identified as an increased risk factor for Campylobacter (Citation27).
Campylobacter prevalence in high-performance farms was between 20–40% lower at first thin than in low-performance farms throughout the year. High-performance farms were selected based on full compliance with farm hygiene audits, which may explain why Campylobacter prevalence was lower in this group. A previous study in the United Kingdom by Gibbens et al. (Citation7) found that hygiene interventions such as twice weekly replenishment of foot dip, a hygiene protocol for entering/exiting broiler houses, and the use of a standardised method of cleaning and disinfecting houses prior to restocking reduced the risk of Campylobacter colonisation by over 50%. Previous studies found a carry-over of clones between consecutive flocks within broiler houses, which demonstrates the potential impact of ineffective hygiene and biosecurity measures (Citation28, Citation29).
There was a seasonal impact on the prevalence of Campylobacter in Irish broilers with a peak observed during the summer season at first thin. The highest mean maximum daily temperatures (18–20°C) were recorded during the month of July, which corresponded to the highest Campylobacter prevalences in both high- and low-performance farms at first thin. This corresponds with the EFSA baseline survey stating that July to September is the highest risk quarter of the year for Campylobacter colonisation of broilers (Citation22). A study in Danish broilers found that even with strict compliance with biosecurity practices, Campylobacter prevalences in excess of 70% were observed in August (Citation30). The higher Campylobacter flock prevalences observed during warmer months may be due to increases in insect activity, cattle grazing, and outside temperatures aiding Campylobacter survival and transmission (Citation9, Citation30) (Citation31). The higher seasonal occurrence of Campylobacter in Irish broilers during summer months highlights an important risk to public health as previous research has linked seasonal poultry Campylobacter peaks with peaks in human campylobacteriosis cases (Citation32).
At final depopulation 85% or more of flocks were Campylobacter positive irrespective of farm performance. It has been suggested that the practice of thinning and/or increased bird age may result in increased Campylobacter prevalence. In Ireland, the practice of thinning involves catching teams and equipment repeatedly entering the broiler house without consistent adherence to hygiene practices. The teams visit multiple farms each day and use equipment, such as forklifts, trucks, and catching crates, which are difficult to adequately disinfect between farms (Citation33). These practices may not only be helping to introduce Campylobacter from the surrounding environment into the broiler houses but also to carry Campylobacter from infected farms to non-infected farms. Previous research also demonstrated an association between the strains isolated from catching equipment immediately prior to thinning and the strains found in flocks at the time of final depopulation (Citation33). It has also been shown that efforts to implement additional biosecurity measures during thinning failed to prevent colonisation by Campylobacter (Citation34). The study identified transport crates as particularly problematic, noting that genotypes of campylobacters isolated from those crates were subsequently found in flocks colonised after thinning. Bird age has also been identified as a risk factor for the colonisation of broilers with Campylobacter (Citation16, Citation27) (Citation35); it is possible that the higher prevalence observed in birds at final thin was influenced by bird age. The higher prevalence of Campylobacter in broilers at final depopulation increases the risk of contamination of carcasses during processing (Citation13). Such batches could be considered high risk and appropriate strategies applied to mitigate this risk. A risk analysis by Havelaar et al. (Citation36) states that, at present, the reduction and prevention of faecal leakage during slaughter, and the separation of contaminated and non-contaminated flocks were the most effective methods for reducing Campylobacter contamination on carcasses. Currently, the Campylobacter status of flocks presented for slaughter is unknown in Ireland.
In conclusion, season, hygiene, and biosecurity practices are important influences on the epidemiology of Campylobacter on broiler farms. In particular, the use of physical step-over barriers, the presence of no more than two houses on a farm, the absence of a water body within 0.5 km, and the use of house-specific footwear may help to decrease Campylobacter prevalence. As thinning was identified as a significant risk factor for Campylobacter introduction, this practice should, if possible, be discontinued. Furthermore, in the absence of testing to determine the Campylobacter status of flocks, batches at final thin should be considered high risk and appropriate risk mitigation measures applied.
Conflict of interest and funding
The authors have not received any funding or benefits from industry or elsewhere to conduct this study.
Acknowledgements
The authors gratefully acknowledge the Food Institutional Research Measure (FIRM) programme administered by the Irish Department of Agriculture, Food and Marine for funding this study (Research grant number 11SF328). The authors all farmers and stakeholders who participated in this study.
References
- EFSA Panel on Biological Hazards (BIOHAZ). Scientific opinion on Campylobacter in broiler meat production: control options and performance objectives and/or targets at different stages of the food chain. EFSA J. 2011; 9: 2105.
- European Food Safety Authority and European Centre for Disease Prevention and Control. Trends and sources of zoonoses, zoonotic agents and food-borne outbreaks in 2014. EFSA J. 2015; 13: 4329.
- European Food Safety Association. Scientific opinion on quantification of the risk posed by broiler meat to human campylobacteriosis in the EU. EFSA J. 2010; 8: 1–89.
- Health Service Executive. Health protection surveillance centre annual report 2011. 2011; Dublin: Health Service Executive. 1–163.
- Central Statistics Office. Poultry income taken by the Irish state for 2014: agricultural output, input and income. Available from: http://www.cso.ie/px/pxeirestat/Database/eirestat/AgriculturalOutputInputandIncome/AgriculturalOutputInputandIncome_statbank.asp?SP=AgriculturalOutput,InputandIncome&Planguage=0 [cited 6 January 2016]..
- Food Safety Authority of Ireland. Recommendations for a practical control programme for Campylobacter in the poultry production and slaughter chain. Report of the Scientific Committee of the Food Safety Authority of Ireland. 2011; 1–44. Available from: https://www.fsai.ie/recommendationsforapracticalcontrolprogrammeforcampylobacterinthepoultryproduction-andslaughterchain.html [cited 6 February 2016].
- Gibbens JC, Pascoe SJS, Evans SJ, Davies RH, Sayers AR. A trial of biosecurity as a means to control Campylobacter infection of broiler chickens. Prev Vet Med. 2001; 48: 85–99.
- Katsma WEA, De Koeijer AA, Jacobs-Reitsma WF, Mangen MJJ, Wagenaar JA. Assessing interventions to reduce the risk of Campylobacter prevalence in broilers. Risk Anal. 2007; 27: 863–76.
- Newell DG, Elvers KT, Dopfer D, Hansson I, Jones P, James S, etal. Biosecurity-based interventions and strategies to reduce Campylobacter spp. on poultry farms. Appl Environ Microbiol. 2011; 77: 8605–14.
- Hald B, Sommer HM, Skovgård H. Use of fly screens to reduce Campylobacter spp. introduction in broiler houses. Emerg Infect Dis. 2007; 13: 1951–3.
- Hue O, Le Bouquin S, Laisney MJ, Allain V, Lalande F, Petetin I, etal. Prevalence of and risk factors for Campylobacter spp. contamination of broiler chicken carcasses at the slaughterhouse. Food Microbiol. 2010; 27: 992–9.
- Sommer H, Heuer O, Sørensen A, Madsen M. Analysis of factors important for the occurrence of Campylobacter in Danish broiler flocks. Prev Vet Med. 2013; 111: 100–11.
- Koolman L, Whyte P, Bolton DJ. An investigation of broiler caecal Campylobacter counts at first and second thinning. J Appl Microbiol. 2014; 117: 876–81.
- Hald B, Rattenborg E, Madsen M. Role of batch depletion of broiler houses on the occurrence of Campylobacter spp. in chicken flocks. Lett Appl Microbiol. 2001; 32: 253–6.
- Patriarchi A, Fox A, Maunsell B, Fanning S, Bolton D. Molecular characterization and environmental mapping of Campylobacter isolates in a subset of intensive poultry flocks in Ireland. Foodborne Pathog Dis. 2011; 8: 99–108.
- Evans SJ, Sayers AR. A longitudinal study of Campylobacter infection of broiler flocks in Great Britain. Prev Vet Med. 2000; 46: 209–23.
- Bouwknegt M, van de Giessen A, Dam-Deisz WDC, Havelaar AH, Nagelkerke NJD, Henken AM. Risk factors for the presence of Campylobacter spp. in Dutch broiler flocks. Prev Vet Med. 2004; 62: 35–49.
- Wang G, Clark CG, Taylor TM, Pucknell C, Barton C, Price L, etal. Colony multiplex PCR assay for identification and differentiation of Campylobacter jejuni, C. coli, C. lari, C. upsaliensis, and C. fetus subsp. Fetus J Clin Microbiol. 2002; 40: 4744–7. [PubMed Abstract].
- Altman DG, Chatfield C, Zidek J. Comparing groups – continuous data. Practical statistics for medical research . 1990; Florida: Chapman & Hall/CRC. 194–6. 1st ed.
- Hanley A, McNeil J. The meaning and use of the area under a receiver operating characteristic (ROC) curve. Radiology. 1982; 143: 29–36.
- Jewell NP, Chatfield C, Tanner M, Zidek J. Estimation and interference for measures of association. Statistics for epidemiology . 2003; Florida: Chapman & Hall/CRC. 81–4. 1st ed.
- European Food Safety Authority. Analysis of the baseline survey on the prevalence of Campylobacter in broiler batches and of Campylobacter and Salmonella on broiler carcasses, in the EU, 2008. Part B: analysis of factors associated with Campylobacter colonisation of broiler batches and with Campylobacter contamination of broiler carcasses; and investigation of the culture method diagnostic characteristics used to analyse broiler carcass samples. EFSA J. 2010; 8: 1522.
- Hald B, Wedderkopp A, Madsen M. Thermophilic Campylobacter spp. in Danish broiler production: a cross-sectional survey and a retrospective analysis of risk factors for occurrence in broiler flocks. Avian Pathol. 2000; 29: 123–31.
- van de Giessen AW, Tilburg JJ, Ritmeester WS, van der Plas J. Reduction of Campylobacter infections in broiler flocks by application of hygiene measures. Epidemiol Infect. 1998; 121: 57–66.
- Humphrey TJ, Henley A, Lanning DG. The colonization of broiler chickens with Campylobacter jejuni: some epidemiological investigations. Epidemiol Infect. 1993; 110: 601–7.
- Devane ML, Nicol C, Ball A, Klena JD, Scholes P, Hudson JA, etal. The occurrence of Campylobacter subtypes in environmental reservoirs and potential transmission routes. J Appl Microbiol. 2005; 98: 980–90.
- McDowell SWJ, Menzies FD, McBride SH, Oza AN, McKenna JP, Gordon AW, etal. Campylobacter spp. in conventional broiler flocks in Northern Ireland: epidemiology and risk factors. Prev Vet Med. 2008; 84: 261–76.
- Damjanova I, Jakab M, Farkas T, Mészáros J, Galántai Z, Turcsányi I, etal. From farm to fork follow-up of thermotolerant campylobacters throughout the broiler production chain and in human cases in a Hungarian county during a ten-months period. Int J Food Microbiol. 2011; 150: 95–102.
- Agunos A, Waddell L, Léger D, Taboada E. A systematic review characterizing on-farm sources of Campylobacter spp. for broiler chickens. PLoS One. 2014; 9: e104905.
- Hald B, Skovgård H, Bang DD, Pedersen K, Dybdahl J, Jespersen JB, etal. Flies and Campylobacter infection of broiler flocks. Emerg Infect Dis. 2004; 10: 1490–2.
- Ellis-Iversen J, Jorgensen F, Bull S. Risk factors for Campylobacter colonisation during rearing of broiler flocks in Great Britain. Prev Vet Med. 2009; 89: 178–84.
- Stanley K, Jones K. Cattle and sheep farms as reservoirs of Campylobacter . J Appl Microbiol. 2003; 94: 104S–13S.
- Allen VM, Weaver H, Ridley AM, Harris JA, Sharma M, Emery J, etal. Sources and spread of thermophilic Campylobacter spp. during partial depopulation of Broiler Chicken Flocks. J Food Prot. 2008; 71: 264–70. [PubMed Abstract].
- Ridley A, Morris V, Gittins J, Cawthraw S, Harris J, Edge S, etal. Potential sources of Campylobacter infection on chicken farms: contamination and control of broiler-harvesting equipment, vehicles and personnel. J Appl Microbiol. 2011; 111: 233–44.
- Lawes JR, Vidal A, Clifton-Hadley FA, Sayers R, Rodgers J, Snow L, etal. Investigation of prevalence and risk factors for Campylobacter in broiler flocks at slaughter: results from a UK survey. Epidemiol Infect. 2012; 140: 1725–37.
- Havelaar AH, Mangen MJJ, de Koeijer AA, Bogaardt MJ, Evers EG, Jacobs-Reitsma WF, etal. Effectiveness and efficiency of controlling Campylobacter on broiler chicken meat. Risk Anal. 2007; 27: 831–44.