Abstract
Background: This study investigated the hepatoprotective effect and antioxidant properties of phloroacetophenone (2′,4′,6′-trihydroxyacetophenone – THA), an acetophenone derived from the plant Myrcia multiflora.
Material & Method: The free radical scavenging activity in vitro and induction of oxidative hepatic damage by carbon tetrachloride (CCl4) (0.5 ml/kg, i.p.) were tested in male Swiss mice (25±5 g).
Results: This compound exhibited in vitro antioxidant effects on FeCl2–ascorbate-induced lipid peroxidation (LPO) in mouse liver homogenate, scavenging hydroxyl and superoxide radicals, and 2,2-diphenyl-1-picrylhydrazyl. The in vivo assays showed that THA significantly (p<0.01) prevented the increases of hepatic LPO as measured by the levels of thiobarbituric acid-reactive substances, mitochondrial swelling. It also protected hepatocytes against protein carbonylation and oxidative DNA damage. Consistent with these observations, THA pre-treatment normalized the activities of antioxidant enzymes, such as catalase, glutathione peroxidase, and superoxide dismutase, and increased the levels of reduced glutathione (GSH) in CCl4-treated mice. In addition, THA treatment significantly prevented the elevation of serum enzymatic activities of alanine amino transferase, aspartate amino transferase, and lactate dehydrogenase, as well as histological alterations induced by CCl4. Silymarin (SIL) (24 mg/kg), a known hepatoprotective drug used for comparison, led to a significant decrease (p<0.01) in activities of theses enzymes in way very similar to that observed in pre-treatment with THA.
Conclusion: These results suggest that the protective effects are due to reduction of oxidative damage induced by CCl4 resulting from the antioxidant properties of THA.
Introduction
Reactive oxygen species (ROS) are causative factors of degenerative diseases, including some hepatopathies Citation1. The overproduction of ROS and therefore oxidative stress can be initiated by a variety of factors, including exposure to xenobiotics, such as acetaminophen and carbon tetrachloride (CCl4) Citation2. CCl4 has been extensively used to study liver injury induced by ROS in the mouse model, which is closely analogous to hepatotoxicity in humans. The hepatotoxic effect of CCl4 is thought to be initiated as a result of its reductive dehalogenation by cytochrome P450 2E1 to the highly reactive trichloromethyl radical (•CCl3), which is subsequently converted into a trichloromethyl peroxyl radical (•OOCCl3) in the presence of oxygen Citation3. Removal of hydrogen atoms from unsaturated fatty acids by such radicals generates carbon-centered lipid radicals. These lipid radicals quickly add molecular oxygen to form lipid peroxyl radicals, thereby initiating the process of lipid peroxidation (LPO). Unless neutralized by radical scavengers, these peroxyl radicals in turn abstract hydrogen atoms from other lipid molecules, thereby propagating the process of LPO Citation4. Also, •CCl3 can react with sulfhydryl groups, such as reduced glutathione (GSH) and protein thiols, which eventually leads to abnormal protein function, membrane LPO and, consequently, damage to mitochondria and nuclei, leading to impaired physiological functions of hepatocytes, and finally, to cell necrosis Citation5.
In this sense, the antioxidant action plays an important role in protecting against CCl4-induced liver injury. Protective effects of various natural and synthetic products against hepatotoxicity have been reported Citation6Citation7Citation8. Phenolic compounds or polyphenols, which consist of secondary vegetal metabolites, constitute a wide and complex array of phytochemicals that exhibit antioxidant action Citation9. Among the phenolic compounds, some acetophenones possess several biological properties Citation10. It has been reported by Mathiesen and collaborators Citation11 that phloroacetophenone (2′,4′,6′-trihydroxyacetophenone – THA; ) exercises its antioxidant activity in vitro by scavenging 2,2-diphenyl-1-picrylhydrazyl (DPPH), but no further investigations were carried out. THA is the aglycone part of a glycoside from Myrcia multiflora (family Myrtaceae), a plant popularly known as ‘insulin plant’ and used for diabetes in traditional medicine in South America. The literature cites several sources for obtaining phloroacetophenone derivatives, but the free form of acylphloroglucinol is rare Citation12Citation13Citation14. Isolated flavanone and flavonol glucosides have been reported to inhibit aldose reductase and α-glucosidase activities and to possess a potential for hypoglycemic activity in alloxan-induced diabetic animals Citation15Citation16. But only studies that describe the antioxidant effect and hepatoprotector of THA are available.
Here, we investigated both the in vitro and in vivo antioxidant activity and the potential protective effects of THA in CCl4-induced hepatotoxicity in mice. The protective activity of THA was compared with that of Silymarin (SIL), a natural antioxidant that has been used in clinical practice for the treatment of toxic liver disease Citation17. This study was carried out taking into consideration that THA possesses a beneficial activity as an antioxidant and hepatoprotective agent, although the mechanism for the activity remains to be elucidated.
Materials and methods
Chemicals
All chemicals were of the highest commercially available purity. THA monohydrate was from Fluka. All other chemicals were from Sigma-Aldrich Co.
Plant material and isolation of phloracetophenone glucoside
The leaves of cultivated M. multiflora were obtained from Albano Ferreira Martins, Ltd., São Paulo, Brazil. The dried leaves of M. multiflora were subjected to the methods of extraction and isolation of phloracetophenone glucoside according to Suksamraran and collaborators Citation18. The compound was isolated and identified by preparative TLC and analyzed by 1H NMR. IR showed that data were consistent with those reported for the 4,6-dihydroxy-2-O-(β-d-glucopyranosyl) acetophenone. Indeed, by the acidic hydrolysis of the compound mentioned above, it is possible to prepare the THA.
To obtain phloroacetophenone, 4,6-dihydroxy-2-O-(β-d-glucopyranosyl) acetophenone (200 mg) was treated with 3 N HCl in methanol (200 ml) at a reflux temperature of 100°C for 30 min Citation18Citation19Citation20. After neutralization by careful addition of 20% aqueous NaHCO3 and elimination of methanol under vacuum and controlled temperature, the phloracetophenone was extracted with CH2Cl2, followed by the evaporation of solvent and recrystallization with boiling water. This yielded crystals of colorless needles that were submitted to 1H NMR, IR, and TLC analyses with a synthetic standard of THA monohydrate. Analytical TLC was carried out on 0.2-mm plates of silica gel 60 F254 (Merck, Darmstadt). For separation and identification of compounds, the following mobile phases (a, b) and spray reagent (c) were used: (a) AcOet–H2CO2–HOAc–H20 (500:5:5:2); (b) CHCl3–(CH3)2CO–H2CO2 (150:33:17), and (c) vanillin/H2SO4 (10% vanillin in a 2:1 mixture of 99.5% ethanol and concentrated H2SO4), followed by heating for color development.
In vitro antioxidant activity
The free radical scavenging activity of THA was evaluated using the 2-diphenyl-1-picryl-hydrazyl-hydrate (DPPH) radical scavenger method and measured at 518 nm Citation21. Superoxide anion () was measured according to Robak and Gryglewski Citation22, using its generation through xanthine/xanthine oxidase and detected by nitro blue tetrazolium (NBT) reduction at 560 nm. The hydroxyl radical (•OH) was generated by the deoxyribose method and its reaction with thiobarbituric acid (TBA) was indirectly detected through the pink chromophore measured at 532 nm Citation23. LPO was assayed using the TBA method, as described by Chen and Tappel Citation24. THA antioxidant activities in vitro were evaluated in triplicates in the range 0.5 to 500 µg/ml and the results were expressed as IC50, which was the concentration (in µg/ml) of THA required to inhibit the generation rates of radicals by 50%. SIL, a mixture of four flavonolignans that possesses a recognized ROS scavenger activity as well as a hepatoprotective effect, was used as the antioxidant standard Citation17.
Animals
Male Swiss mice, supplied by the local Bioterio Central of the Federal University of Santa Catarina and weighing 25±5 g, were housed under controlled conditions (12-h light–dark cycle, 22±2°C, 60% air humidity), and had free access to standard laboratory chow and water. All animal procedures were conducted in accordance with legal requirements appropriate to the species (Guiding Principles for the Care and Use of Laboratory Animals, NIH publication #85.23, revised in 1985) and with the approval of the local Ethics Committee.
Hepatoprotective activity
Hepatotoxicity was induced by CCl4 (0.5 ml/kg body weight in olive oil, injected intraperitoneally) according to the method described by Carbonari et al. Citation7. THA was preliminarily evaluated at 3, 6, and 12 mg/kg body weight and the dose of 6 mg/kg was selected because it was the lowest dose that could normalize the hepatic enzymes aspartate amino transferase (AST), alanine amino transferase (ALT), and lactate dehydrogenase (LDH). Mice were randomly divided into five groups (n=6 each group) and administered orally by gavage during 7 days as follows:
Group I: Control group, treated with vehicle (saline) daily for 7 days.
Group II: Treated with vehicle (saline) daily for 7 days followed by CCl4 on day 7.
Group III: Treated with THA (6 mg/kg) daily for 7 days.
Group IV: Treated with THA (6 mg/kg) daily for 7 days followed by CCl4 on day 7.
Group V: Treated with SIL (24 mg/kg) daily for 7 days.
Group VI: Treated with SIL (24 mg/kg) daily for 7 days followed by CCl4 on day 7.
Determination of biochemical markers of hepatic injury
Blood was placed at room temperature for 1 h and then centrifuged at 1,000g for 10 min to obtain serum. The enzymatic activities of AST, ALT, and LDH were assayed spectrophotometrically according to Reitman and Frankel Citation25, Bergmeyer Citation26, and Allain et al. Citation27, using a commercial kit (LABTEST, Diagnostica S.A., Minas Gerais, Brazil).
Oxidative damage biomarkers
Peroxidation of hepatic tissue lipids, in vivo, was measured by the method of Okawa et al. Citation28. The amount of thiobarbituric acid-reactive substances (TBARS) was expressed as nmoles of TBARS formed per mg of protein and using a molar coefficient (ɛ) of 153 mmol/l per cm. Oxidative damage of proteins was quantified as carbonyl protein content according to the method of Levine et al. Citation29. This method is based on spectrophotometric detection of the end product of the reaction of 2,4-dinitrophenylhydrazine with protein carbonyl to form protein hydrazones detected at 370 nm, and the results were expressed as nmoles of carbonyl group per mg of protein, using ɛ = 22 mmol/l per cm. Liver GSH levels were measured by a spectrophotometric method Citation30 in acid hepatic homogenates combined with disodium hydrogen phosphate and DTNB solution, and the yellow chromophore formed was quantified at 412 nm using a molar coefficient (ɛ) of 14.1 mmol/l per cm. The results were expressed in µmol per mg of protein.
Detection of DNA damage
The extent of DNA fragmentation was assessed by the alkaline Comet assay proposed by Singh et al. Citation31. Liver samples (100 mg) were homogenized in 1,000 µl phosphate-buffered saline (PBS) (1:9) and 10 µl of this solution was added to 70 µl of 0.75% low-melting point agarose. The cell/agarose mixture was added to a fully frosted microscope slide coated with a layer of 300 µl of normal-melting agarose (1%). The slides were immersed into a cold and freshly made lysis solution [2.5 mM NaCl, 100 mM EDTA, 1% Triton X-100, 10% dimethyl sulfoxide (DMSO) and 10 mM Tris, pH 10] for a minimum of 2 h. Subsequently, the slides were incubated in freshly prepared alkaline buffer (300 mM NaOH and 1.0 mM EDTA, pH 13) for 30 min. DNA was subjected to electrophoresis for 30 min at 280 mA and 25 V, and then neutralized with 0.4 M Tris, pH 7.5. Finally, DNA was stained with ethidium bromide (2.0 µg/ml). Images of 100 randomly selected cells (50 cells from each of two replicate slides) were analyzed from each animal and comet image lengths (nuclear region + tail) were measured in arbitrary units. A DNA damage index (DI) was compiled according to tail size, from undamaged (0) to maximally damaged (400), resulting in a single DNA damage score for each animal, and consequently, for each group studied. Thus, the DI of each group ranged from 0 (completely undamaged) to 400 (maximum damage).
Antioxidant enzymes
Catalase (CAT) activity was determined by measuring at 240 nm the decrease of H2O2 in a freshly prepared 10 mM hydrogen peroxide solution; the results are expressed in mmol/min per mg protein and ɛ = 40 mmol/l per cm Citation32. Superoxide dismutase (SOD) activity in homogenates was determined by measuring the inhibition of the rate of autocatalytic adrenochrome formation and expressed in U SOD/mg protein Citation33. Glutathione peroxidase (GPx) activity was quantified by a coupled assay with glutathione reductase (GR) catalyzed oxidation of NADPH. Measurements were made at 340 nm and expressed in µmol/min per mg protein Citation34. Protein was measured by the method of Lowry et al. Citation35 using bovine serum albumin as standard.
Determination of mitochondrial swelling
Mitochondria were prepared from mouse liver according to the method of Aprille et al. Citation36. In brief, livers of mice were excised and homogenized in an isolation buffer (225 mM d-mannitol, 75 mM sucrose, 0.05 mM EDTA, 10 mM Tris–HCl pH 7.4) at 4°C. The homogenates were centrifuged at 600g for 5 min and supernatants were centrifuged at 8,800g for 10 min. The pellet was washed twice with the same buffer. Protein concentration was determined by using Coomassie Brilliant Blue Citation37.
Mitochondrial swelling was assessed by measuring the absorbance of their suspension at 540 nm Citation38. Liver mitochondria isolated from each group of mice were dissolved at 0.5 mg protein per ml in 3 ml of the assay buffer (125 mM sucrose, 50 mM KCl, 2 mM KH2PO4, 10 mM HEPES, 5 mM succinate). To the assay buffer was added 50 mmol/l of Ca2 + to initiate mitochondrial swelling and the absorbance (A) at 0.5, 1, 2, 3, 4, 5, and 6 min was measured. The rate of mitochondrial swelling was calculated by using following formula:
Histological analyses
The hepatic duct was chosen (adjacent to liver) to standardize the histological analyses and to avoid misinterpretations. Samples of the same liver lobes (8–15×3–4 mm) for all treatment were fixed in 10% of the same liver lobes for all treatments were fixed in 10% of formalin buffered with 0.1 M phosphate (pH 7.2). After fixation, samples were washed in tap water for 4 h and dehydrated through an increasing ethanol series, cleared, and embedded in 56–60% paraffin. Sections were cut at 5-µm thickness, stained with Cason's trichromic staining, and mounted in Eukit medium Citation39. Histological sections were photographed under a light microscope with a digital image capture system (Olympus, model Dx 41).
Statistical analysis
Data were expressed as means±SD. Statistical analysis was performed to compare treated groups with the respective control groups using one-sample Kolmogorov-Smirnov analysis of normality and two-way analysis of variance (ANOVA), followed by the Duncan's multiple range test where appropriate. Values of p<0.01 were considered statistically significant.
Results
In vitro antioxidant activity
A marked difference in DPPH radical scavenging capacity was found: the isomeric mix of flavonolignans SIL showed a higher DPPH scavenging capacity than THA (). Accordingly, SIL inhibited the generation of superoxide anion radicals to a greater extent than THA, but it is important to note that SIL concentration was about four-fold higher than that of THA. Curiously, no significant difference between SIL and THA was detected regarding both •OH scavenging capacity and protection against membrane LPO ().
Table 1. In vitro antioxidant activity of THA and Silymarin measured by scavenging of DPPH, and •OH radicals, and protection against lipid peroxidation (LPO) (levels of thiobarbituric acid reactive substances) in hepatic homogenates
Biochemical markers of hepatic injury
After 12 h since administration of CCl4 to mice, the levels of markers, such as ALT, AST, and LDH activities, were high in serum sample when compared with controls (). Oral administration of THA or SIL significantly restored levels of these hepatic markers, except for LDH activity.
Table 2. Effects of THA and Silymarin on CCl4-induced liver damage in mice
Oxidative damage biomarkers and DNA fragmentation
Pre-treatment of mice with THA or SIL had a remarkable antioxidant effect and prevented the LPO induced by CCl4 (82.7% and 83.3%, respectively) (A). In accordance with the results related to LPO, protein oxidation quantified as carbonyl protein content also showed a significant increase after CCl4 treatment (51.9%), which was significantly decreased by THA or SIL pre-treatment (35.8% and 31.2%, respectively) (B). In addition, fluorescence microscopy revealed that CCl4 caused remarkable DNA fragmentation (DI = 110.2±3.3) in hepatocyte homogenates when compared to the control group (DI = 49.0±1.0). On the other hand, DNA fragmentation in mice pre-treated with THA or SIL (DI = 51.0±2.0 and 45.0±4.0) was similar to that in control mice (C).
Glutathione (GSH) content and antioxidant enzymes
When administration of CCl4 to the mice resulted in approximately 60% depletion of hepatic GSH levels compared with controls (). Pre-treatment with THA or SIL was effective in protecting hepatocytes against such depletion. On the other hand, CCl4 treatment resulted in a significant (p<0.01) decrease of the hepatic enzymes CAT, SOD, and GPx when compared with the negative control group. THA or SIL pre-treatments prevented such decreases, which were within normal limits ().
Table 3. Effects of THA and Silymarin on GSH levels and antioxidant enzyme activities (CAT, SOD, and GPx) in livers of treated mice and of control mice
Mitochondrial swelling
Calcium-induced remarkable mitochondrial swelling in normal mice, which was greatly attenuated in mice pre-treated with CCl4 (46.9%) (), suggesting a decline in the sensitivity of mitochondria to swelling due to high calcium content. However, THA or SIL blocked such attenuation with inhibitory rates of 49.5% and 47.7%, respectively.
Histological analyses
Histological analyses revealed that in terms of morphology, livers subjected to all treatments generally showed normal structural integrity. The stromal and the parenchymal portions presented structural characteristics of healthy liver of mice. Intact hepatocytes plates were observed in fairly radial position in relation to the centrolobular vein with reticular fibers present on both sides. Plenty of capillary sinusoids of normal caliber and non-congestion were also observed. Hepatocytes were large and polyhedral, with round nuclei, abundant heterochromatin and nucleoli quite evident, all of which are characteristic of normal cells. However, when using Cason's staining, which can reveal distinct substrates with different colors, the CCl4 group (G) showed higher variation in color with two distinct populations of hepatocytes (separated by dashed lines). These cells exhibited a variety of substrates in the cytoplasm (as revealed by different staining), indicating that although initial morphological changes are not evident, there are indications of changes in hepatocyte metabolism. When the histological preparations are observed in a smaller magnification, the number of hepatocytes with different colors (and therefore with different metabolic activities) form groups quite limited under CCl4 treatment not clear (C, dashed lines). When comparing the other groups, the appearance in color (and thus in cell physiology) is very similar to each other (A–E, dotted lines), showing that the hepatoprotective treatments with THA (D) and SIL (E) were very effective against the hepatotoxic effects of CCl4.
Fig. 4. Histological analyses of liver in control mice (A), mice treated with THA (B), mice treated with CCl4 (C), mice pre-treated with THA followed by CCl4 (D), and mice pre-treated with Silymarin followed by CCl4 (E). Liver tissue was stained with trichromic Cason's staining (100×, bar = 100 µm). Photomicrographs F and G show histocytological details of normal and abnormal livers, respectively (Cason's staining 1000×, bar = 10 µm). Dashed lines separate the number of hepatocytes with different staining and arrows showed the nucleus and reticular fibers.
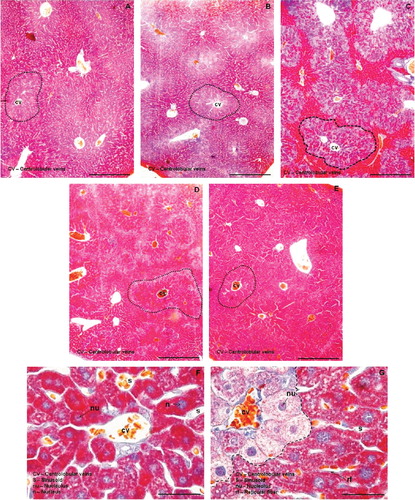
Discussion
We demonstrate that THA has a concentration-dependent scavenging capacity for the DPPH radical higher than that of SIL, which is attributable to its hydrogen-donating ability. DPPH possesses a proton-free radical with the characteristics of absorption that decreases significantly on exposure of proton radical scavengers Citation40.
In the present study, THA or SIL were able to scavenge in a concentration-dependent manner, measured by the xanthine/xanthine oxidase and NBT assays (). Although the superoxide anion (
) is a relatively weak oxidant, it reacts to form more potent ROS, such as the hydroxyl radical (•OH), which initiate LPO processes Citation41. Furthermore, superoxide anion (
) is also indirectly initiate the LPO as a result of H2O2 formation, creating precursors of •OH Citation41. Taking this into account, the results suggest that the antioxidant activity of THA is also related to its ability to scavenge the superoxide anion (
).
In the LPO assay, •OH was scavenged by co-incubation of mouse liver homogenate with different concentrations of THA or SIL, and both phenolic compounds showed substantial and similar protection against membrane LPO (). Furthermore, the evidence of •OH scavenging activity of THA was obtained through the deoxyribose system, which displayed an IC50 close to that of SIL, thereby explaining such a strong protection against LPO. This property probably relies on the presence of phenolic hydroxyls at the ortho and para positions Citation11. An early study that used this system to assess the biological activity of natural plant-derived biomolecules reported that molecules that can inhibit deoxyribose degradation are those that can chelate iron ions and render them inactive or poorly active for the Fenton reaction Citation42. In summary, the in vitro results suggest that THA, which effectively scavenges several ROS, behaves in a way similar to SIL. The broad activity range related to THA suggests that multiple and synergistic mechanisms are probably responsible for the antioxidant activity of this acetophenone-derived substance.
CCl4 is known to cause hepatic damage manifested in marked elevation in serum levels of LDH and amino transferase enzymes (AST and ALT), especially ALT, which is considered the primary and specific marker of liver injury Citation43. Accordingly, our results also showed a significant increase in the activities of AST, ALT, and LDH, thereby confirming the hepatocellular damage in CCl4-treated mice. A similar profile was observed by Hewawasam and collaborators Citation44 in adult Swiss mice treated with CCl4 (0.5 ml/kg in olive oil). After pre-treatment with THA, the activities of these enzymes were decreased to values similar to those of controls (). THA could also prevent the CCl4-induced increase in hepatic TBARS levels, suggesting that THA inhibits LPO and its propagation reactions (A). In addition, the histological analyses revealed typical toxicological effects on hepatocytes promoted by CCl4, as already described in the literature Citation45Citation46. THA alone increased LDH levels in relation to controls but this enhancement was significantly lower than that observed in CCl4 treatment.
CCl4 causes marked toxicity by increasing the liver LPO, as detected by enhanced levels of hepatic TBARS Citation47Citation48. As already mentioned, CCl4 hepatotoxicity depends on the reductive dehalogenation of CCl4 catalyzed by CYP450 in the hepatic endoplasmic reticulum, leading to the generation of an unstable complex of trichloromethyl peroxy radicals (CCl3•), which is reported to be a highly reactive species Citation5. This free radical attacks membrane lipids, causing their peroxidation, and may also covalently bind to lipids and proteins, thereby initiating deleterious processes that ultimately leads to cell damage. THA may afford cell protection by impairing CCl4-mediated LPO, thus preventing the generation of free radical derivatives, if the ameliorated TBARS levels and mitochondrial sensitivity to calcium are taken into consideration (A).
Besides the finding that CCl4 increased LPO, it also depleted intracellular GSH levels, indicating that GSH loss might result from the detoxification of CCl4 by GSH conjugation. In addition, the previous enhancement of carbonyl protein contents was significantly prevented in mice treated with THA or SIL, suggesting a further antioxidant protection against protein oxidation. Therefore, these combined results strongly suggest that acetophenone acts as an antioxidant.
The histopathological analysis provided complementary evidence that pre-treatment with THA or SIL attenuated the cytoplasmatic changes in mouse liver induced by CCl4 administration. The hepatocyte plate of CCl4-treated mice resulted in cytophysiological changes shown by Cason's trichrome staining, revealing different colors in the cytoplasm (). This might have been due to the formation of highly reactive radicals associated with the oxidative threat induced by CCl4. Cellular accumulation of lipid hydroperoxides can cause cytotoxicity associated with peroxidation of membrane phospholipids by lipid hydroperoxides, the basis for a related cellular damage Citation41. The cytophysiological changes coincided with increased levels of LPO (A), protein oxidation (B), and DNA fragmentation (C), while the administration of THA or SIL prevented such biochemical and histological alterations induced by CCl4. This effect could be attributable to the antioxidant activity of these two compounds, which significantly attenuated the oxidative threat and led to restoration of normal physiological functions.
In addition, the antioxidant enzymes CAT and GPx in mice pre-treated with THA or SIL and then treated with CCl4 had activities similar to those of controls (). Treatment of mice with CCl4 concurrently induces processes in acute injury and also in regeneration, in which injury events are predominantly expressed at early stages, although this regeneration process is latent Citation49. A possible reason why CAT and GPx activities were augmented after treatment with THA or SIL in the presence of CCl4 is the capacity of these compounds to counteract CCl4-induced massive ROS production Citation41. Therefore, we speculate here that THA plays a role during the early stages in CCl4-induced liver injury, decreasing LPO and protein carbonylation and, consequently, improving cellular antioxidant status, thereby preventing ALT, AST, and LDH leakage from liver.
The activity of GPx, an important antioxidant enzyme that takes part in the enzymatic neutralization of free radicals, was significantly decreased by CCl4 treatment and normalized in mice pre-treated with THA or SIL. This result strongly suggests that CCl4, probably through its conversion to , the active metabolite produced in the liver after oral administration, inhibits the activity of GPx. In accordance with the response found in GPx, CAT activity also showed decreased values after CCl4 treatment, and again the pre-treatment with THA was able to normalize its activity. Taking into account the diminished GSH content together with the persistent enhancement of TBARS levels found in livers of mice treated with CCl4, these combined results suggest that this organ was facing a severe oxidative insult. It also strongly suggests that this process was reversed after THA treatment because THA possesses some antioxidant properties that can guarantee GSH maintenance probably through ROS neutralization.
In contrast to the toxic activation of CCl4 via P450 2E1 biotransformation process, the detoxification pathway also involves GSH conjugation of the •CCl3, a P450 2E1-mediated CCl4 metabolite Citation50. A previous study on the mechanism of CCl4-induced hepatotoxicity showed that GSH plays a key role in detoxifying the toxic metabolites of CCl4 and that liver necrosis begins when GSH stores are markedly depleted Citation4. GSH levels are largely mediated by the activity of glutathione-S-transferase, generating adducts with the toxic metabolites of CCl4, and it has been suggested that one of the main causes of CCl4-induced liver injury is protein and LPO caused by its free radical derivatives Citation4. As already mentioned, the present results showed that pre-treatment with THA or SIL significantly inhibited LPO, protein carbonylation, and DNA damage and that it also inhibited CCl4-induced hepatic GSH depletion.
ROS inflict damage on several cellular structures and functional components, including DNA, even under physiological conditions Citation41. The present study showed a 52% enhancement of DNA fragmentation in mice exposed to CCl4 compared to the control group. However, DNA damage in liver was decreased in animals pre-treated with THA, suggesting that either the system of enzymatic repair was induced and/or an increase in antioxidant defenses in cell nuclei took place.
In conclusion, both the in vitro and the in vivo results indicate that THA can protect mouse liver from injury induced by CCl4 probably through its ROS scavenging ability. Nevertheless, further research must be carried out to verify the capacity of THA to block P450-mediated CCl4 bioactivation and also to elucidate the mechanisms regarding the hepatoprotective effect of THA at the molecular level.
Conflict of interest and funding
The authors have not received any funding or benefits from industry to conduct this study.
Acknowledgements
R.C. Pedrosa (Proc. 300718/2003-9) and D. Wilhelm Filho (Proc. 309438/2008-0) are recipients of research grants from the National Council for Scientific and Technological Development (CNPq), Brazil.
References
- Hensley K, Robinson KA, Gabbita SP, Salsman S, Floyd RA. Reactive oxygen species, cell signaling and cell injury. Free Radic Biol Med. 2000; 28: 1456–62.
- Ronsein GE, Guidi DB, Benassi JC, Wilhelm Filho D, Pedrosa RC. Cytoprotective effects of carvedilol against oxygen free radical generation in rat liver. Redox Rep. 2005; 10: 131–7.
- Goeptar AR, Scheerens H, Vermeulen NP. Oxygen and xenobiotic reductase activities of cytochrome P450. Crit Rev Toxicol. 1995; 25: 25–65.
- Recknagel RO, Glende EA, Britton RS. Free radical damage and lipid peroxidation. Hepatotoxicology. Meeks RG, Harrison SD, Bull RJCRC Press. Boca Raton FL, 1991; 401–36.
- Recknagel RO, Glende EA, Dolack JA, Walter RL. Mechanism of carbon tetrachloride toxicity. Pharmacol Ther. 1992; 43: 139–54.
- Halim AB, el-Ahmady O, Hassab-Allah S, Abdel-Galil F, Hafez Y, Darwish A. Biochemical effect of antioxidants on lipids and liver function in experimentally-induced liver damage. Ann Clin Biochem. 1997; 34: 656–63.
- Carbonari KA, Ferreira EA, Rebello JM, Felipe KB, Rossi MH, Felício JD, et al.. Free-radical scavenging by Ouratea parviflora in experimentally-induced liver injuries. Redox Rep. 2006; 11: 124–30.
- Lin H-M, Tseng H-C, Wang C-J, Lin J-J, Lo C-W, Chou F-P. Hepatoprotective effects of Solanum nigrum Linn extract against CCl4-induced oxidative damage in rats. Chem Biol Interact. 2008; 171: 283–93.
- Bravo L. Polyphenols: chemistry, dietary sources, metabolism and nutritional significance. Nutr Rev. 1998; 56: 17–33.
- Favier L, Tonn C, Guerreiro E, Rotelli A, Pelzer L. Anti-inflammatory activity of acetophenones from Ophryosporus axilliflorus. Planta Med. 1998; 64: 657–9.
- Mathiesen L, Malterud KE, Sund RB. Hydrogen bond formation as basis for radical scavenging activity: a structure-activity study of c-methylated dihydrochalcones from Myrica gale and structurally related acetophenones. Free Radic Biol Med. 1997; 22: 307–11.
- Lee KR, Hong SW, Kwak JH, Pyo S, Jee OP. Phenolic constituents from the aerial parts of Artemisia stolonifera. Arch Pharm Res. 1996; 19: 231–4.
- Dai Y, He XJ, Zhou GX, Kurihara H, Ye WC, Yao XS. Acylphloroglucinol glycosides from the fruits of Pyracantha fortuneana. J Asian Nat Prod Res. 2008; 10: 111–7.
- Chosson E, Chaboud A, Chulia AJ, Raynaud J. A phloroacetophenone glucoside from Rhododendron ferrugineum. Phytochemistry. 1998; 47: 87–8.
- Yoshikawa M, Shimada H, Nishida N, Li Y, Toguchida I, Yamahara J, Matsuda H. Antidiabetic principles of natural medicines. II. Aldose reductase and alpha-glucosidase inhibitors from Brazilian natural medicine, the leaves of Myrcia multiflora DC. (Myrtaceae): structures of myrciacitrins I and II and myrciaphenones A and B. Chem Pharm Bull. 1998; 46: 113–9.
- Matsuda H, Nishida N, Yoshikawa M. Antidiabetic principles of natural medicines. V. Aldose reductase inhibitors from Myrcia multiflora DC. 2: structures of myrciacitrins III, IV, and V. Chem Pharm Bull. 2002; 50: 429–31.
- Pradhan SC, Girish C. Hepatoprotective herbal drug, Silymarin from experimental pharmacology to clinical medicine. Indian J Med Res. 2006; 124: 491–504.
- Suksamraran A, Eiamong S, Piyachaturawat P, Byrne LT. A phloracetophenone glucoside with choleretic activity from Curcuma comosa. Phytochemistry. 1997; 45: 103–5.
- Brandão MGL, Nery CGC, Mamão MAS, Krettli AU. Two methoxylated flavone glycosides from Bidens pilosa. Phytochemistry. 1998; 48: 397–9.
- Zhao LM, Jin HS, Sun LP, Piao HR, Quan ZS. Synthesis and evaluation of antiplatelet activity of trihydroxychalcone derivatives. Bioorg Med Chem Lett. 2005; 15: 5027–9.
- Brand-Williams W, Cuvelier ME, Berset C. Use of free radical method to evaluate antioxidant activity. Leb Wiss Technol. 1995; 28: 25–30.
- Robak J, Gryglewski RJ. Flavonoids are scavengers of superoxide anions. Biochem Pharmacol. 1988; 37: 837–73.
- Halliwell B, Gutteridge JMC. Formation of thiobarbituric acid-reactive substances from deoxyribose in the presence of iron sits- the role of superoxide and hydroxyl radicals. FEBS Lett. 1981; 128: 347–52.
- Chen H, Tappel AL. Protection of multiple antioxidants against heme protein oxidation and lipid peroxidation induced by CBrCl3 in liver, lung, kidney, heart and spleen. J Agric Food Chem. 1996; 44: 854–8.
- Reitman S, Frankel S. A colorimetric method for the determination of serum glutamic oxalacetic and glutamine pyruvic transaminases. Am J Clin Pathol. 1957; 28: 56–63.
- Bergmeyer HU. Methods of enzymatic analysis. Verl Chem. 1983; 3: 118–25.
- Allain CC, Poon LC, Chan CSG, Richmond W, Fu PC. Enzymatic determination of total serum cholesterol. Clin Chem. 1974; 20: 470–5.
- Okawa H, Ohishi N, Yagi K. Assay for lipid peroxides in animal tissues by thiobarbituric acid reaction. Anal Biochem. 1979; 95: 351–8.
- Levine RL, Garland D, Oliver CN, Amici A, Climent AI, Lenz AG, et al.. Determination of carbonyl content in oxidatively modified proteins. Methods Enzymol. 1990; 186: 464–78.
- Anderson ME. Determination of glutathione and glutathione disulfide in biological samples. Methods Enzymol. 1985; 113: 548–55.
- Singh NP, McCoy MT, Tice RR, Schneider EL. A simple technique for quantification of low levels of DNA damage in individual cells. Exp Cell Res. 1988; 175: 184–91.
- Aebi H. Catalase in vitro. Methods Enzymol. 1984; 105: 121–6.
- Misra HP, Fridovich I. The role of superoxide anion in the autoxidation of epinephrine and a simple assay for superoxide dismutase. J Biol Chem. 1972; 247: 3170–5.
- Flohé L, Gunzler WA. Assays of glutathione peroxidase. Methods Enzymol. 1984; 105: 114–21.
- Lowry OH, Rosebrough NJ, Farr AL. Protein measurement with Folin phenol reagent. J Biol Chem. 1993; 193: 265–75.
- Aprille JR, Hom JA, Rulfs J. Liver and skeletal muscle mitochondrial function following burn injury. J Trauma. 1977; 17: 279–88.
- Bradford MM. A rapid and sensitive method for the quantitation of microgram quantities of protein utilizing the principle of protein–dye binding. Anal Chem. 1976; 72: 248–54.
- Tang XH, Gao J, Chen J, Xu LZ, Tang YH, Zhao XN, et al.. Mitochondrial modulation is involved in the hepatoprotection of Limonium sinense extract against liver damage in mice. J Ethnopharmacol. 2008; 120: 427–31.
- Cargnin-Ferreira E, Sarasquete C. Histofisiología de Moluscos Bivalvos Marinos [Histology of Marine Bivalve]. CSIC. Madrid, 2008
- Constantin M, Bromont C, Fickat R, Massingham R. Studies on the activity of bepridil as a scavenger of free radicals. Biochem Pharmacol. 1990; 40: 1615–22.
- Halliwell B, Gutteridge JMC. Free radicals in biology and medicine3rd ed. Oxford University Press. New York, 1999
- Halliwell B, Gutteridge JMC, Aruoma OI. The deoxyribose method: a simple “test tube” assay for determination of rate constants for reactions of hydroxyl radicals. Anal Biochem. 1987; 165: 215–9.
- Vozarova B, Stefan N, Lindsay RS, Saremi A, Pratley RE, Bogardus C, et al.. High alanine aminotransferase is associated with decreased hepatic insulin sensitivity and predicts the development of type 2 diabetes. Diabetes. 2002; 51: 1889–95.
- Hewawasam RP, Jayatilaka KAPW, Pthinrana C, Mudduwa LKB. Hepatoprotective effect of Epaltes divaricatta extract on tetrachloride induced hepatotoxicity in mice. Indian J Med Res. 2004; 120: 30–4.
- Friedman SL. The cellular basis of hepatic fibrosis: mechanisms and treatment strategies. N Engl J Med. 1993; 328: 1828–35.
- Muriel P, Moreno MG, Hernandez M, del C. Resolution of liver fibrosis in chronic CCl4 administration in the rat after discontinuation of treatment: effect of silymarin, silibinin, colchicine and trimethylcolchicinic acid. Basic Clin Pharmacol Toxicol. 2005; 96: 375–80.
- Poli G. Liver damage due to free radicals. Brit Med Bull. 1993; 49: 604–20.
- Noyan T, Kömüroglu U, Bayram I, Sekeroglu MR. Comparison of the effects of melatonin and pentoxifylline on carbon tetrachloride-induced liver toxicity in mice. Cell Biol Toxicol. 2006; 22: 381–91.
- Bhave SV, Donthamsetty S, Latendesse JR, Mehendale HM. Inhibition of cyclooxygenase-2 aggravates secretory phospholipase A2-mediated progression of acute live injury. Toxicol Appl Pharmacol. 2008; 228: 239–46.
- Williams AT, Burk RF. Carbon tetrachloride hepatotoxicity: an example of free radical-mediated injury. Semin Liver Dis. 1990; 10: 279–84.