Abstract
Allergic asthma is a disease characterized by persistent allergen-driven airway inflammation, remodeling, and airway hyperresponsiveness. CD4+ T-cells, especially T-helper type 2 cells, play a critical role in orchestrating the disease process through the release of the cytokines IL-4, IL-5, and IL-13. Allergen-specific immunotherapy (SIT) is currently the only treatment with a long-term effect via modifying the natural course of allergy by interfering with the underlying immunological mechanisms. However, although SIT is effective in allergic rhinitis and insect venom allergy, in allergic asthma it seldom results in complete alleviation of the symptoms. Improvement of SIT is needed to enhance its efficacy in asthmatic patients. Herein, the immunoregulatory mechanisms underlying the beneficial effects of SIT are discussed with the ultimate aim to improve its treatment efficacy.
Allergic asthma is recognized as a chronic inflammatory disease of the airways in which many types of cells play a role, in particular mast cells, eosinophils, B- and T-lymphocytes, and epithelial cells. It is characterized by the production of allergen-specific immunoglobulin (Ig)E antibodies, reversible airflow obstruction, airway hyperresponsiveness (AHR) to a wide variety of specific or non-specific stimuli, chronic airway inflammation, and airway remodeling Citation1. During the past several decades, the prevalence of allergic asthma is increasing dramatically (> 20% in every 10 years), especially in children Citation2. Approximately, it affects 10–15% of the population in most developed industrial countries Citation2. Both genetic and environmental factors are involved in allergic asthma. Genetic studies indicate that multiple genes are linked to the onset of this disease Citation3Citation4Citation5. However, the epidemic increase of asthma cannot be explained by genetic changes in the population. Changes in environment and lifestyle including isolation of houses which increases numbers of house dust mite, and childhood immunizations have contributed to the increase Citation6Citation7Citation8Citation9. In addition, epidemiological and clinical studies have suggested a link between the relative decreased exposure to bacteria or other pathogenic agents (such as helminthes) during the first years of life, and the increase in allergic asthma Citation10Citation11Citation12; this is referred to as the ‘hygiene hypothesis.’
Pathogenesis of asthma
The concepts of the pathogenesis of allergic asthma are illustrated in . The development of allergic asthma requires sensitization to an environmental allergen, which can occurs years before the onset of clinical symptoms Citation6Citation13. For allergic sensitization allergens are taken up and processed by professional antigen-presenting cells (APCs), such as dendritic cells (DCs) and presented to allergen-specific T-cells that subsequently differentiate into T-helper (Th) 2-type cells. Activation of Th2 cells leads to the production of inflammatory cytokines, such as interleukin 4 (IL-4) and IL-13, thereby stimulating allergen-specific B-cells to proliferate and switch to production of IgE Citation14Citation15. Then IgE binds to high-affinity IgE receptor FcɛRI on mast cells. Upon re-exposure, the allergen will bind to allergen-specific IgE on mast cells thereby cross-linking the IgE–FcɛRI complexes which subsequently cause the release of preformed mediators like histamine and several other inflammatory mediators Citation16Citation17. These mast cell-derived mediators cause the early asthmatic reaction which is characterized by airway smooth muscle constriction, extensive vascular leakage, mucus hypersecretion, enhanced airway responsiveness, and recruitment of inflammatory cells Citation17Citation18Citation19.
Fig. 1. Schematic representation of the pathophysiology of allergic asthma. In genetically predisposed individuals, primary exposure to an allergen leads to activation of Th2 lymphocytes and stimulation of IgE synthesis. Later on, exposure causes immediate release of the biologically active mediators (histamine, leukotrienes) via mast cell degranulation and further activation of Th2 cells with resulting eosinophil inflammation and AHR.
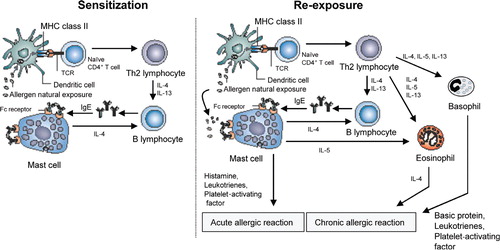
In approximately 50% of the asthmatic patients this early phase reaction is followed by the late phase reaction. This phase is characterized by significant involvement of infiltrated inflammatory cells, such as eosinophils, T lymphocytes and macrophages, and resident epithelial, endothelial and smooth muscle cells in promoting the chronic symptoms of airway inflammation. In addition, these cells may also be an important source of inflammatory mediators like chemokines, cytokines, and leukotrienes in asthma.
Asthma: a CD4+ T-helper type 2 (Th2)-mediated disease
CD4+ T-cells play a crucial role in controlling inflammation in allergic asthma. They are the predominant lymphocyte population that infiltrates the airways in asthmatics and are activated in these sites, expressing the surface activation markers class II histocompatibility antigen [HLA-DR], CD25 (IL-2R), and very late activation antigen-1 (VLA-1) Citation20Citation21. In addition to CD4+ T-cells, CD8+ T-cells, and γ/δ T-cells have been identified in the airways of allergic asthmatics Citation21. About 60% of the CD4+ T-cells in the airway of persons with asthma are invariant natural killer T (iNKT) cells Citation22Citation23Citation24Citation25. However, there is still controversy whether iNKT-cells can induce allergic asthma in the absence of CD4+ T-cells Citation26Citation27Citation28.
Human and murine CD4+ T-cells are divided into two broad functional subsets according to their profiles of cytokine secretion Citation29Citation30. The conditions under which CD4+ Th cells become activated during an immune response determine the development toward the Th1- or the Th2-phenotype Citation31. CD4+ Th1-type cells secrete predominantly IL-2 and interferon gamma (IFN-γ) and are particularly implicated in cell-mediated immune responses against invading intracellular pathogens, such as viruses Citation32Citation33. CD4+ Th2-type cells produce a different panel of cytokines including IL-4, IL-13, and IL-5 Citation34. IL-4 promotes the development of Th2 cells and together with other cytokines, promotes the growth of mast cells, basophils, and eosinophils Citation35Citation36. Furthermore, IL-4 and IL-13 are required for the induction of proliferation and isotype switching to IgE by B-cells that recognize the allergen Citation14Citation37. IL-5 secretion by Th2 cells is critical for eosinophil differentiation and maturation Citation38Citation39.
Over the years it became clear that the allergen-induced airway inflammation is orchestrated by activated Th2 cells. Th2-type lymphocytes play a critical role in the initiation, progression, and persistence of allergic asthma. Initially, a disturbed balance between Th1- and Th2-mediated immune responses has been postulated to underlie aberrant Th2 reactions to ‘innocuous’ environmental antigens. Indeed, allergen-specific T-cell clones isolated from the blood of allergic individuals express a Th2 cytokine profile secreting IL-4, IL-5, and minimal IFN-γ and IL-2, whereas those clones from non-atopic individuals displayed a Th1 profile Citation40. Furthermore, allergic asthma is associated with the expression of IL-3, IL-4, and IL-5 in bronchoalveolar cells, strongly supporting Th2 activation Citation41. Moreover, it has been shown that when antigen-specific Th2 cells activated with inhaled antigen, Th2 cells-induced airway eosinophilia, mucus hypersecretion, and AHR after short-exposure to antigen, while Th1 cells resulted in a neutrophil-predominant inflammatory response without mucus production or AHR Citation42Citation43, indicating that Th1 cells alone do not produce any of the characteristic features of asthma. In human studies, CD4+ T-cells producing IL-4, IL-5, and IL-13 have been identified in bronchoalveolar lavage fluid and airway biopsies from asthmatics patients. These cytokines are secreted in the airways of patients with mild or asymptomatic disease Citation41. Furthermore, it has been shown that CD4+ Th2 lymphocytes are increased in the airways of asthmatic patients after antigen challenge Citation21Citation44Citation45.
Allergen-specific immunotherapy (SIT)
Current pharmacologic therapies for allergic asthma, such as bronchodilators and inhaled corticosteroids, are effective in reducing and preventing symptom development, but do not reverse the progression of or cure this disease. SIT is unique in that it not only reduces symptoms, but also induces long-lasting disease remission. SIT has been shown to improve allergic dysfunction and to re-direct the immune system away from the allergic response.
SIT was first introduced at St. Mary's Hospital London, at the end of the nineteenth century and many of the basic principles described remain valid today. In 1911, Noon carried out the first study of active immunization to prevent allergy against grass pollen using s.c. injection of a distilled water extract of the pollen of timothy grass, Phleum pretense Citation46. In 1918, it was generally accepted that hay fever, asthma, and anaphylaxis were the result from antibodies produced after exposure to sensitizing antigen Citation47.
By 1961, a new technique was developed to allow in vitro measurements of histamine release from cells in whole blood in the presence of specific allergen before and after treatment with ragweed extract Citation48. The findings demonstrated that after SIT, histamine release was completely abolished in a few patients and was reduced in others. Five years later, a double-blind study performed by Lichtenstein and Osler Citation49 demonstrated that the treatment with crude ragweed extract or the major allergen of ragweed ‘Amb a 1’ resulted in reduction of cellular sensitivity in some patients. Treated patients showed little correlation between cellular sensitivity and symptom scores. This finding was accompanied with a rise in blocking antibodies. After these results, the term immunotherapy has been used to describe the process because it greatly deals with complex immunologic changes.
Specific immunotherapy (SIT) for allergic asthma
SIT for the management of allergic asthma continues to be a matter for discussion Citation50Citation51Citation52Citation53. Abramson and colleagues Citation51Citation54Citation55 performed a meta-analysis of all trials published over the past 50 years of the twentieth century examining the impact of SIT in patients with allergic asthma. Overall, SIT was efficacious of decreasing asthma medication use, reducing bronchial hyperresponsiveness, and improving asthma symptom scores. While there was no consistent effect on lung function, SIT significantly reduced the airways response to inhalation of specific allergen, with some reduction in non-specific AHR as well.
Mechanisms involved in specific immunotherapy (SIT)
The potential mechanisms that have been proposed to explain the beneficial effects of SIT are illustrated in .
Fig. 2. Schematic representation of the potential immune deviation leading to the beneficial effects of SIT. Allergen SIT results in both a shift in allergen-specific T-cells from Th2 to Th0/Th1 responses and the generation of IL-10 and TGF-β producing T regulatory (Treg) cells. Allergen-specific Th1 immune responses protect against the development of allergic disorders by inducing the production of IFN-γ, which inhibits the development of Th2 cells. The regulatory cytokines IL-10 and TGF-β induce switching of B cell responses in favor of IgG4 antibodies and IgA antibodies, respectively, and suppress IgE production. IL-10 and TGF-β directly or indirectly suppress effector cells of allergic inflammation such as mast cells and eosinophils thereby preventing release of mediators and late-phase inflammation. Sold gray arrows represent immune response pathway to natural exposure; dotted arrows represent immune response pathway to IT; blocked lines represent inhibition.
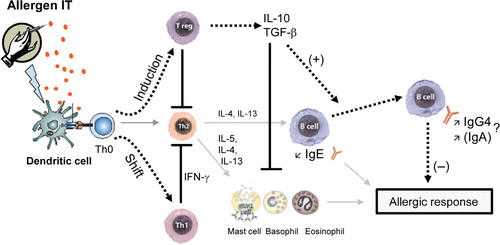
To explain the immunological mechanisms underlying the clinical improvement, intensive research has concentrated upon the specific antibody response in serum with respect to class and subclass distribution Citation56. Studies showed that the allergen-specific IgE levels rise temporarily during initial phase of SIT, but fall back to pre-treatment levels during maintenance therapy Citation57. Subsequently, it was demonstrated that SIT also induces allergen-specific IgG (mostly IgG1 and IgG4) and in few reported cases IgA levels Citation58Citation59Citation60. These findings led to the hypotheses that these IgG antibodies contribute to the immunosuppressive effects of SIT by blocking IgE-facilitated antigen presentation Citation61Citation62 and that such antibodies act as blocking antibodies by engaging low-affinity Fc receptors for Ig (e.g. FcγRII) expressed by B lymphocytes, basophils, and mast cells Citation60. In fact, a substantial number of studies demonstrated increased specific IgG4 levels together with clinical improvement Citation63Citation64. However, the role of IgG (and IgA) in SIT has been questioned and it is not clear whether the significant increase in IgG levels has a causal role in alleviating symptoms or simply represents a bystander effect, occurring as a consequence of high allergen exposure. In Fcγ knockout mouse model of allergic asthma we found that bronchoalveolar lavage (BAL) eosinophilia, Th2 cytokines, and serum ovalbumin (OVA)-specific IgE levels were strongly suppressed by SIT, demonstrating that IgG Fcγ receptors have no major role in the beneficial effects of SIT (unpublished data). Moreover, results demonstrated that the suppression of allergen-induced airway inflammation by SIT is not B-cell dependent, ruling out a critical role for B-cells, IgGs, and IgA in SIT in the mouse model of allergic asthma.
Thus, in the context of allergic diseases, including asthma, most research indicates that during SIT the increase of IgG is an epiphenomenon, however, the role of allergen-specific antibodies, as putative ‘blocking factors(s),’ in SIT providing protection against allergic disease appears unlikely.
T-cell tolerance
The generation of an effective immune response involves antigen-specific T-cell expansion and differentiation of effector function. T-cell activation requires at least two distinct signals, including signaling via the antigen-specific T-cell receptor and a co-stimulatory pathway Citation31. Antigen stimulation of T-cells can lead either to a positive immune response, characterized by proliferation, differentiation, clonal expansion, and effector function, or in the absence of an appropriate co-stimulatory signal to a state of long-lasting unresponsiveness, termed anergy Citation65. The most important co-stimulatory molecules involved are CD28/CD152 (CTLA-4)–CD80/CD86 (B7-1/-2) and CD40-CD154 (CD40 ligand) Citation66Citation67Citation68. Anergy induction can down-regulate both cellular and humoral immune responses. The induction of T-cell non-responsiveness or anergy as the mechanism of SIT is supported by the observed diminution of allergen-specific T-cell proliferation and cytokine production Citation69, although in some instances IL-2 was able to restore these functions Citation70. However, the usually increased IgG production during SIT cannot be explained by the induction of T-cell anergy Citation71Citation72. Various methods for anergy induction have been described. Stimulation of T-cells with high doses of antigen in the absence of appropriate co-stimulation or in the presence of IL-10 has been found to induce a profound form of anergy Citation73Citation74. Under these conditions anergic T-cells showed limited peripheral expansion and a significant amount of death, with a residual subset of cells remaining that were unresponsive to antigen restimulation both in vivo and in vitro. It has been suggested that T-cells rendered anergic in vivo and in vitro become regulatory T-cells that can regulate other T-cell responses Citation75Citation76.
Immune deviation toward T-helper type 1 (Th1) responses
In the late 1980s, a favorable explanation was based on the Th1/Th2 dichotomy in specific immune reactions Citation29. Mechanistic studies demonstrated that SIT alters the balance of cytokines released from Th-lymphocytes with a shift from Th2-cells in association with allergic inflammation toward Th1-cells that release IFN-γ, which inhibits Th2-cells Citation77Citation78Citation79. Early studies showed that allergen-specific T-cell clones shift from IL-4 to IFN-γ production after SIT Citation77Citation80. However, the effects of SIT on Th1 cytokine secretion are less consistent Citation81. In humans, several clinical trials demonstrated a reduction of the allergen-specific Th2 response, whereas the Th1 response remained undetectable Citation78Citation82 and in a separate study, SIT diminished both allergen-specific Th2 and Th1 responses Citation83. In experimental allergic asthma models some studies have confirmed that the relation between presence of Th1-associated cytokines and amelioration of disease, but others have not found such effects Citation84. In recent years, the concept of immune deviation toward Th1 response by SIT lost favor with the alternative observation on regulatory T-cells.
Adaptive regulatory T (Treg) cells are taking the center stage as crucial immunoregulatory cells that may offer an explanation for many of the observations that occur during SIT that cannot be adequately explained by the Th2 to Th1 shift Citation85Citation86Citation87.
Regulatory T-cells
Several subsets of regulatory T-cells with distinct phenotypes and mechanism of actions have been identified. These include: Citation1 naturally occurring CD4+CD25+Foxp3+ T-cells (nTregs) that inhibit immune responses through cell-to-cell contact, Citation2 CD4+Foxp3+ iTregs induced in the periphery, in contrast to nTregs not generated in the thymus; the capacity of these cells to suppress the proliferation of naïve T-cells requires cell–cell contact, and Citation3 cells induced in the periphery following antigen exposure (aTreg cells). aTreg cells are subdivided into type 1 regulatory T (Tr1) cells which secrete high levels of IL-10 and low to moderate levels of transforming growth factor (TGF)-β and type 3 regulatory T (Th3) cells which secrete TGF-β.
Natural CD4+CD25+Foxp3+ T regulatory cells
The natural CD4+CD25+Foxp3+ regulatory T (nTreg) cells are generated in the thymus and represent 5–10% of the CD4+ T lymphocytes both in mice and humans. These cells are thought to perform a specialized role in controlling both the innate and the adaptive immune response Citation88Citation89. Depletion of nTreg cells leads to the spontaneous development of various autoimmune diseases Citation90Citation91Citation92Citation93Citation94Citation95Citation96. Although the mechanism of immune regulation mediated by nTreg cells is not well understood, these cells can both directly suppress responding T-cells and down-modulate APC function in vitro Citation97Citation98. nTreg cells may also convert CD4+ Th cell into regulatory cells expressing IL-10 and/or TGF-β in culture systems Citation99Citation100. It has been shown that nTreg cells induce infectious tolerance in vivo by catalyzing the formation of IL-10 producing regulatory T-cells Citation101.
Adaptive T regulatory cells
In recent years, it has been demonstrated that SIT is associated with the induction of aTreg cells. The aTreg cells are generated in the periphery from naïve T-cells after encountering antigens presented by tolerogenic DCs. In studies of SIT for bee venom anaphylaxis, Akdis et al. Citation85 were the first to provide evidence for a role of IL-10 and Tr1 cells in the beneficial effects of SIT. They observed that the therapy-induced increase in secretion of IL-10 in T-cell cultures along with decreased allergen-driven proliferation and decreased production of Th2 and Th1 cytokines. Moreover, in clinical trials, SIT has been shown to increase the production of IL-10 by APCs, including B cells, monocytes, and macrophages Citation61Citation87Citation102. Findings were extended by SIT studies with airborne allergens that showed increases in IL-10 and TGF-β producing antigen-specific Treg cells in the blood and airway tissue Citation61Citation85Citation86Citation87. Induction of IL-10 producing Tr1 cells has been shown to be associated with successful SIT Citation85Citation86. Animal studies confirmed the suppressive role of IL-10 during allergic inflammatory diseases Citation84Citation103Citation104Citation105Citation106. In human studies, the regulatory cytokine IL-10 has been shown to increase the production of IgG4 while preventing IL-4-mediated class switching to IgE Citation107. Furthermore, IL-10 down-regulate IgE-dependent activation of basophils and mast cells, and decrease survival and activation of eosinophils Citation108. In addition, IL-10 (and TGF-β) might act on APCs. IL-10 down-regulates Major Histocompatability Complex (MHC) class II expression on monocytes and reduces their antigen presentation capacity Citation109. Moreover, IL-10 down-regulates the CD80 expression on DCs and macrophages Citation110Citation111. IL-10 may therefore block the APC dependent CD28–CD80 interaction and subsequent co-stimulatory signaling in T-cells Citation112.
The other immunoregulatory cytokine associated with regulatory T-cells is TGF-β. Studies have provided evidence for increases in the amount of TGF-β-driven allergen-specific IgA following SIT, indicating other antibody classes than IgG might contribute to clinical efficacy Citation87. Moreover, TGF-β has been shown to induce the expression of IL-10 by T-cells Citation113. Literature data suggest that early increase in IL-10 Citation85 and sustained TGF-β production may be required for successful SIT to induce stable tolerance to non-pathogenic environmental antigens Citation114.
Role of dendritic cells (DCs) in generation of adaptive regulatory T (aTreg) cells
Although there is suggestive evidence that SIT achieves beneficial effectiveness by the induction of aTreg cells, the mechanism by which SIT induces aTreg cells is not completely understood. Over the past decade, it has become clear that DCs play a critical role in the generation of all aTreg cell subsets Citation115 and regulation of immune responses to a variety of antigens Citation116. The ability of DCs to induce immunity or tolerance depends on their maturation state. Tolerogenic DCs are semi-mature cells with increased expression of MHC II and CD86 but low levels of expression of CD40 and absence of the pro-inflammatory cytokines IL-6 and TNF-α Citation117. It has been shown that aTreg cells induced by immature DCs are characterized by high levels of IL-10 cytokine secretion Citation118Citation119. The nuclear factor-κB (NF-κB) protein Re1B activity is critically required for DCs maturation Citation120. Re1B-deficient bone marrow-derived DCs (BMDCs) or BMDCs in which Re1B activity is inhibited have the potential to induce antigen-specific immune tolerance in vivo Citation121. Inhibition of NF-κB signaling by various drugs Citation122Citation123Citation124 induces DCs to acquire tolerogenic properties that favor the induction of Tr1-like cells in vitro and tolerance in mouse models of transplantation and autoimmune diseases Citation125Citation126Citation127.
Besides this, recent findings demonstrate that DCs induce development of aTreg cells by several mechanisms including high expression of the tryptophan-catabolizing enzyme indoleamine 2,3-dioxygenase (IDO) Citation128Citation129. IDO expressing DCs inhibit T-cell proliferation in vitro and promote tolerance in vivo Citation130Citation131, including maternal tolerance during pregnancy Citation132, control of allograft rejection Citation133, and protection against autoimmunity Citation134. A number of studies have also demonstrated that IDO is implicated in tolerance induction by regulatory T-cells expressing cytotoxic T-lymphocyte antigen-4 (CTLA-4) Citation135Citation136. Moreover, IDO may directly mediate inhibition of T-cells proliferation by tryptophan depletion or downstream tryptophan metabolites Citation137Citation138. Recently, we showed that inhibition of IDO throughout SIT abrogated the efficacy of SIT on the reduction of BAL eosinophil numbers and AHR to methacholine, but no changes in serum OVA-specific IgE levels were observed Citation139. In addition, we demonstrated that inhibition of IDO throughout the aerosol challenge period did not interfere with tolerance induction by SIT. These findings reveal that IDO plays a role only during induction of tolerance by SIT and that activity of IDO is irrelevant for the effects of SIT thereafter. Moreover, considering the conceptual possibilities that either tryptophan depletion or downstream tryptophan metabolites mediated-IDO immunoregulation we showed that formation of tryptophan metabolites, rather than tryptophan depletion, is the mechanism by which SIT induces tolerance to the induction of airway manifestations of asthma.
Improvement of specific immunotherapy (SIT)
The goal of SIT is the transformation of an allergic individual into one who can tolerate allergen exposure by converting deleterious allergic immune response into protective responses. Despite the impressive efficacy of allergen (injection) SIT for treatment of allergic rhinitis and insect venom allergy, its efficacy in allergic asthma remains controversial Citation51. Furthermore, it has been reported that s.c. administration of allergens can induce severe systemic reactions due to cross-linking of allergen-specific IgE on mast cells Citation140Citation141. Thus, for development of a more effective and safe form of SIT, more insight into the underlying immunological mechanisms of allergen IT is considered necessary to improve efficacy, in particular in asthmatic patients. There is growing evidence that the positive effects of SIT are associated with aTreg cells (Tr1 and Th3) and the immunosuppressive cytokines that they produce. The mechanisms by which these cells are induced by SIT are still not fully understood. Well-described pathways to induce aTreg cells are antigen presentation to T-cell by immature DCs or the presence of IL-10 or TGF-β in the local microenvironment. In this regard, combination of SIT with administration of compounds that inhibit DCs maturation, such as the biologically active form of vitamin D3 (1α,25-dihydroxyvitamin D3) or glucocorticoids, might be novel immunotherapeutic strategies to improve SIT for better treatment of allergic diseases, including allergic asthma. Interestingly, in a mouse model of allergen immunotherapy, we recently demonstrated that co-administration of 1α,25-dihydroxyvitamin D3 effectively suppressed AHR to methacholine and potentiated the reduction of serum allergen-specific IgE levels and BAL eosinophilia in the suboptimal SIT regime matched by a reduction in Th2 cytokines, IL-5, and IL-13 Citation142. We further observed that in vitro antigen-induced productions of Th2-cytokines are decreased in lung-draining lymph node cells after this combination SIT. Moreover, data demonstrates that the immunoregulatory cytokines IL-10 and TGF-β are involved in tolerance to allergen-induced airway manifestation of asthma since the suppressive effects of combined SIT are completely abrogated by blocking of the IL-10R and neutralizing of TGF-β by using mAbs at the time of antigen inhalation challenge.
Conclusion
Peripheral (naturally occurring and adaptive) T- cell tolerance is the key immunological mechanism in healthy immune response to self- and non-infectious non-self-antigen. Changes in the balance between allergen-specific Treg and Th2, and/or Th1 cells are very crucial in the development and also treatment of allergic diseases. Understanding of the immunological mechanisms that lead to tolerance induction by SIT, , in particular the role of regulatory T-cells in allergen-specific peripheral tolerance may lead to more rational and safer approaches that could result in prevention and cure of allergic diseases, including asthma.
Fig. 3. Hypothetical scheme of the proposed mechanisms contributing to the induction of tolerance by SIT. (A) Natural exposure to an allergen leads to activation of Th2 cells. (B) nTreg cells are generated in thymus, but can also develop from conventional CD4+ T-cells by specific condition or signals. nTreg cells induce IDO expression on dendritic cell (IDO+DC). This is partially mediated via the interaction of CTLA-4 expressed on nTreg and CD80 (B7) expressed on DC. (C) IDO catalyzes the initial and rate-limiting step of tryptophan degradation. (D) IDO expressing DC induces development of Treg after allergen IT by a mechanism at present not completely defined. (E) 1,25(OH)2D3 inhibits transcription factor NF-κB thereby inhibiting maturation of DC and resulting in tolerogenic DC, which direct the induction of Treg cells. Treg cells suppressed Th2 responses and effector cells by the release of immunoregulatory cytokines IL-10 and TGF-β. (F) The role of allergen-specific IgA is unclear. Dotted arrows represent immune response pathway to SIT; blocked lines represent inhibition.
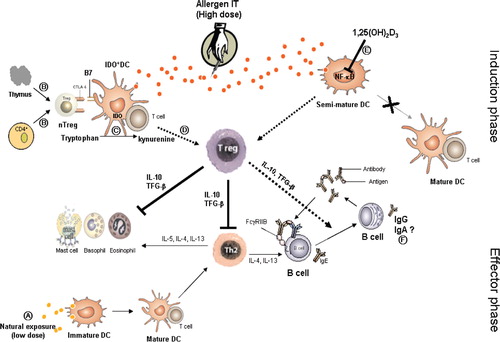
Conflict of interest and funding
Y.A.T was supported by a grant 965.02 from the Libyan Ministry of Higher Education
Acknowledgements
This study was partly financially supported by the Utrecht Institute for Pharmaceutical Sciences, Utrecht University, Utrecht, The Netherlands.
References
- Maddox L, Schwartz DA. The pathophysiology of asthma. Annu Rev Med. 2002; 53: 477–98.
- EUROP WHO. Environmental hazards trigger childhood allergic disorders. Fact sheet EURO/01/3. April 2003.
- Bosse Y, Hudson TJ. Toward a comprehensive set of asthma susceptibility genes. Annu Rev Med. 2007; 58: 171–84.
- Carroll W. Asthma genetics: pitfalls and triumphs. Paediatr Respir Rev. 2005; 6: 68–74.
- Cookson WO. Asthma genetics. Chest. 2002; 121: S7S–13.
- Holt PG, Thomas WR. Sensitization to airborne environmental allergens: unresolved issues. Nat Immunol. 2005; 6: 957–60.
- Perry TT, Wood RA, Matsui EC, Curtin-Brosnan J, Rand C, Eggleston PA. Room-specific characteristics of suburban homes as predictors of indoor allergen concentrations. Ann Allergy Asthma Immunol. 2006; 97: 628–35.
- Trasande L, Thurston GD. The role of air pollution in asthma and other pediatric morbidities. J Allergy Clin Immunol. 2005; 115: 689–99.
- von Mutius E. The environmental predictors of allergic disease. J Allergy Clin Immunol. 2000; 105: 9–19.
- Matricardi PM, Rosmini F, Riondino S, Fortini M, Ferrigno L, Rapicetta M, et al.. Exposure to foodborne and orofecal microbes versus airborne viruses in relation to atopy and allergic asthma: epidemiological study. BMJ. 2000; 320: 412–7.
- Tantisira KG, Weiss ST. Childhood infections and asthma: at the crossroads of the hygiene and Barker hypotheses. Respir Res. 2001; 2: 324–7.
- Strachan DP. Family size, infection and atopy: the first decade of the “hygiene hypothesis”. Thorax. 2000; 55: S2–10.
- Noertjojo K, Dimich-Ward H, Obata H, Manfreda J, Chan-Yeung M. Exposure and sensitization to cat dander: asthma and asthma-like symptoms among adults. J Allergy Clin Immunol. 1999; 103: 60–5.
- Del Prete G, Maggi E, Parronchi P, Chretien I, Tiri A, Macchia D, et al.. IL-4 is an essential factor for the IgE synthesis induced in vitro by human T cell clones and their supernatants. J Immunol. 1988; 140: 4193–8.
- Van der Pouw Kraan TC, Van der Zee JS, Boeije LC, De Groot ER, Stapel SO, Aarden LA. The role of IL-13 in IgE synthesis by allergic asthma patients. Clin Exp Immunol. 1998; 111: 129–35.
- Hart PH. Regulation of the inflammatory response in asthma by mast cell products. Immunol Cell Biol. 2001; 79: 149–53.
- Durham SR. Mechanisms of mucosal inflammation in the nose and lungs. Clin Exp Allergy. 1998; 28: 11–6.
- Charlesworth EN. Late-phase inflammation: influence on morbidity. J Allergy Clin Immunol. 1996; 98: S291–7.
- Bradding P, Holgate ST. Immunopathology and human mast cell cytokines. Crit Rev Oncol Hematol. 1999; 31: 119–33.
- Corrigan CJ, Hartnell A, Kay AB. T lymphocyte activation in acute severe asthma. Lancet. 1988; 1: 1129–32.
- Walker C, Bode E, Boer L, Hansel TT, Blaser K, Virchow JCJr. Allergic and nonallergic asthmatics have distinct patterns of T-cell activation and cytokine production in peripheral blood and bronchoalveolar lavage. Am Rev Respir Dis. 1992; 146: 109–15.
- Akbari O, Faul JL, Hoyte EG, Berry GJ, Wahlstrom J, Kronenberg M, et al.. CD4+ invariant T-cell-receptor+ natural killer T cells in bronchial asthma. N Engl J Med. 2006; 354: 1117–29.
- Akbari O, Stock P, Meyer E, Kronenberg M, Sidobre S, Nakayama T, et al.. Essential role of NKT cells producing IL-4 and IL-13 in the development of allergen-induced airway hyperreactivity. Nat Med. 2003; 9: 582–8.
- Bilenki L, Yang J, Fan Y, Wang S, Yang X. Natural killer T cells contribute to airway eosinophilic inflammation induced by ragweed through enhanced IL-4 and eotaxin production. Eur J Immunol. 2004; 34: 345–54.
- Lisbonne M, Diem S, de Castro Keller A, Lefort J, Araujo LM, Hachem P, et al.. Cutting edge: invariant V alpha 14 NKT cells are required for allergen-induced airway inflammation and hyperreactivity in an experimental asthma model. J Immunol. 2003; 171: 1637–41.
- Das J, Eynott P, Jupp R, Bothwell A, Van Kaer L, Shi Y, et al.. Natural killer T cells and CD8(+) T cells are dispensable for T cell-dependent allergic airway inflammation. Nat Med. 2006; 12: 1345–6.
- Mutalithas K, Croudace J, Guillen C, Siddiqui S, Thickett D, Wardlaw A, et al.. Bronchoalveolar lavage invariant natural killer T cells are not increased in asthma. J Allergy Clin Immunol. 2007; 119: 1274–6.
- Vijayanand P, Seumois G, Pickard C, Powell RM, Angco G, Sammut D, et al.. Invariant natural killer T cells in asthma and chronic obstructive pulmonary disease. N Engl J Med. 2007; 356: 1410–22.
- Mosmann TR, Coffman RL. TH1 and TH2 cells: different patterns of lymphokine secretion lead to different functional properties. Annu Rev Immunol. 1989; 7: 145–73.
- Wierenga EA, Snoek M, Jansen HM, Bos JD, van Lier RA, Kapsenberg ML. Human atopen-specific types 1 and 2 T helper cell clones. J Immunol. 1991; 147: 2942–9.
- Kapsenberg ML, Hilkens CM, Wierenga EA, Kalinski P. The role of antigen-presenting cells in the regulation of allergen-specific T cell responses. Curr Opin Immunol. 1998; 10: 607–13.
- Pinto RA, Arredondo SM, Bono MR, Gaggero AA, Diaz PV. T helper 1/T helper 2 cytokine imbalance in respiratory syncytial virus infection is associated with increased endogenous plasma cortisol. Pediatrics. 2006; 117: e878–86.
- Johnson PA, Conway MA, Daly J, Nicolson C, Robertson J, Mills KH. Plasmid DNA encoding influenza virus haemagglutinin induces Th1 cells and protection against respiratory infection despite its limited ability to generate antibody responses. J Gen Virol. 2000; 81: 1737–45.
- Mosmann TR, Cherwinski H, Bond MW, Giedlin MA, Coffman RL. Two types of murine helper T cell clone. I. Definition according to profiles of lymphokine activities and secreted proteins. J Immunol. 1986; 136: 2348–57.
- Swain SL, Weinberg AD, English M, Huston G. IL-4 directs the development of Th2-like helper effectors. J Immunol. 1990; 145: 3796–806.
- Coyle AJ, Le Gros G, Bertrand C, Tsuyuki S, Heusser CH, Kopf M, et al.. Interleukin-4 is required for the induction of lung Th2 mucosal immunity. Am J Respir Cell Mol Biol. 1995; 13: 54–9.
- Barner M, Mohrs M, Brombacher F, Kopf M. Differences between IL-4R alpha-deficient and IL-4-deficient mice reveal a role for IL-13 in the regulation of Th2 responses. Curr Biol. 1998; 8: 669–72.
- Coffman RL, Seymour BW, Hudak S, Jackson J, Rennick D. Antibody to interleukin-5 inhibits helminth-induced eosinophilia in mice. Science. 1989; 245: 308–10.
- Stafford S, Lowell C, Sur S, Alam R. Lyn tyrosine kinase is important for IL-5-stimulated eosinophil differentiation. J Immunol. 2002; 168: 1978–83.
- Kapsenberg ML, Jansen HM, Bos JD, Wierenga EA. Role of type 1 and type 2 T helper cells in allergic diseases. Curr Opin Immunol. 1992; 4: 788–93.
- Robinson DS, Hamid Q, Ying S, Tsicopoulos A, Barkans J, Bentley AM, et al.. Predominant TH2-like bronchoalveolar T-lymphocyte population in atopic asthma. N Engl J Med. 1992; 326: 298–304.
- Cohn L, Homer RJ, Marinov A, Rankin J, Bottomly K. Induction of airway mucus production by T helper 2 (Th2) cells: a critical role for interleukin 4 in cell recruitment but not mucus production. J Exp Med. 1997; 186: 1737–47.
- Cohn L, Tepper JS, Bottomly K. IL-4-independent induction of airway hyperresponsiveness by Th2, but not Th1, cells. J Immunol. 1998; 161: 3813–6.
- Azzawi M, Bradley B, Jeffery PK, Frew AJ, Wardlaw AJ, Knowles G, et al.. Identification of activated T lymphocytes and eosinophils in bronchial biopsies in stable atopic asthma. Am Rev Respir Dis. 1990; 142: 1407–13.
- Corrigan CJ, Kay AB. CD4 T-lymphocyte activation in acute severe asthma. Relationship to disease severity and atopic status. Am Rev Respir Dis. 1990; 141: 970–7.
- Noon L. Prophylactic inoculation against hay fever. Lancet. 1911; 2: 1572–3.
- Cooke R. Hay fever and asthma: the uses and limitations of desensitization. NY Med J. 1918; 107: 577–83.
- Arsdel PJV, Middleton EJ. the effect of hyposensitization on the in vitro histamine release by specific antigen. J Allergy. 1961; 32: 348–56.
- Lichtenstein LM, Osler AG. Studies on the mechanisms of hypersensitivity phenomena. XII. An in vitro study of the reaction between ragweed pollen antigen, allergic human serum and ragweed-sensitive human leukocytes. J Immunol. 1966; 96: 169–79.
- Bousquet J, Michel FB. Specific immunotherapy in asthma: is it effective?. J Allergy Clin Immunol. 1994; 94: 1–11.
- Abramson MJ, Puy RM, Weiner JM. Is allergen immunotherapy effective in asthma? A meta-analysis of randomized controlled trials. Am J Respir Crit Care Med. 1995; 151: 969–74.
- Bousquet J. Pro: immunotherapy is clinically indicated in the management of allergic asthma. Am J Respir Crit Care Med. 2001; 164: 2139–40. (Discussion 41–2).
- Adkinson NF Jr . Con: immunotherapy is not clinically indicated in the management of allergic asthma. Am J Respir Crit Care Med. 2001; 164: 2140–1. (Discussion 1–2).
- Abramson M, Puy R, Weiner J. Immunotherapy in asthma: an updated systematic review. Allergy. 1999; 54: 1022–41.
- Abramson MJ , Puy RM , Weiner JM . Allergen immunotherapy for asthma. Cochrane Database Syst Rev. 2000; CD001186.
- Djurup R. The subclass nature and clinical significance of the IgG antibody response in patients undergoing allergen-specific immunotherapy. Allergy. 1985; 40: 469–86.
- Creticos PS, Van Metre TE, Mardiney MR, Rosenberg GL, Norman PS, Adkinson NFJr. Dose response of IgE and IgG antibodies during ragweed immunotherapy. J Allergy Clin Immunol. 1984; 73: 94–104.
- Gehlhar K, Schlaak M, Becker W, Bufe A. Monitoring allergen immunotherapy of pollen-allergic patients: the ratio of allergen-specific IgG4 to IgG1 correlates with clinical outcome. Clin Exp Allergy. 1999; 29: 497–506.
- Jakobsen CG, Bodtger U, Poulsen LK, Roggen EL. Vaccination for birch pollen allergy: comparison of the affinities of specific immunoglobulins E, G1 and G4 measured by surface plasmon resonance. Clin Exp Allergy. 2005; 35: 193–8.
- Wachholz PA, Durham SR. Induction of ‘blocking’ IgG antibodies during immunotherapy. Clin Exp Allergy. 2003; 33: 1171–4.
- Nouri-Aria KT, Wachholz PA, Francis JN, Jacobson MR, Walker SM, Wilcock LK, et al.. Grass pollen immunotherapy induces mucosal and peripheral IL-10 responses and blocking IgG activity. J Immunol. 2004; 172: 3252–9.
- van Neerven RJ, Wikborg T, Lund G, Jacobsen B, Brinch-Nielsen A, Arnved J, et al.. Blocking antibodies induced by specific allergy vaccination prevent the activation of CD4+ T cells by inhibiting serum-IgE-facilitated allergen presentation. J Immunol. 1999; 163: 2944–52.
- Flicker S, Valenta R. Renaissance of the blocking antibody concept in type I allergy. Int Arch Allergy Immunol. 2003; 132: 13–24.
- Wachholz PA, Durham SR. Mechanisms of immunotherapy: IgG revisited. Curr Opin Allergy Clin Immunol. 2004; 4: 313–8.
- Gudmundsdottir H, Turka LA. T cell costimulatory blockade: new therapies for transplant rejection. J Am Soc Nephrol. 1999; 10: 1356–65.
- Gao JX, Madrenas J, Zeng W, Cameron MJ, Zhang Z, Wang JJ, et al.. CD40-deficient dendritic cells producing interleukin-10, but not interleukin-12, induce T-cell hyporesponsiveness in vitro and prevent acute allograft rejection. Immunology. 1999; 98: 159–70.
- Kobata T, Azuma M, Yagita H, Okumura K. Role of costimulatory molecules in autoimmunity. Rev Immunogenet. 2000; 2: 74–80.
- Wells AD, Walsh MC, Bluestone JA, Turka LA. Signaling through CD28 and CTLA-4 controls two distinct forms of T cell anergy. J Clin Invest. 2001; 108: 895–903.
- Ebner C, Siemann U, Bohle B, Willheim M, Wiedermann U, Schenk S, et al.. Immunological changes during specific immunotherapy of grass pollen allergy: reduced lymphoproliferative responses to allergen and shift from TH2 to TH1 in T-cell clones specific for Phl a major grass pollen allergen. Clin Exp Allergy. 1997; 27: 1007–15.
- Moverare R, Elfman L, Bjornsson E, Stalenheim G. Cytokine production by peripheral blood mononuclear cells following birch-pollen immunotherapy. Immunol Lett. 2000; 73: 51–6.
- Bahceciler NN, Isik U, Barlan IB, Basaran MM. Efficacy of sublingual immunotherapy in children with asthma and rhinitis: a double-blind, placebo-controlled study. Pediatr Pulmonol. 2001; 32: 49–55.
- Pajno GB, Barberio G, De Luca F, Morabito L, Parmiani S. Prevention of new sensitizations in asthmatic children monosensitized to house dust mite by specific immunotherapy. A six-year follow-up study. Clin Exp Allergy. 2001; 31: 1392–7.
- Jenkins MK, Schwartz RH. Antigen presentation by chemically modified splenocytes induces antigen-specific T cell unresponsiveness in vitro and in vivo. J Exp Med. 1987; 165: 302–19.
- Schwartz RH. Models of T cell anergy: is there a common molecular mechanism?. J Exp Med. 1996; 184: 1–8.
- Buer J, Lanoue A, Franzke A, Garcia C, von Boehmer H, Sarukhan A. Interleukin 10 secretion and impaired effector function of major histocompatibility complex class II-restricted T cells anergized in vivo. J Exp Med. 1998; 187: 177–83.
- Taams LS, van Rensen AJ, Poelen MC, van Els CA, Besseling AC, Wagenaar JP, et al.. Anergic T cells actively suppress T cell responses via the antigen-presenting cell. Eur J Immunol. 1998; 28: 2902–12.
- Jutel M, Pichler WJ, Skrbic D, Urwyler A, Dahinden C, Muller UR. Bee venom immunotherapy results in decrease of IL-4 and IL-5 and increase of IFN-gamma secretion in specific allergen-stimulated T cell cultures. J Immunol. 1995; 154: 4187–94.
- Secrist H, Chelen CJ, Wen Y, Marshall JD, Umetsu DT. Allergen immunotherapy decreases interleukin 4 production in CD4+ T cells from allergic individuals. J Exp Med. 1993; 178: 2123–30.
- Varney VA, Hamid QA, Gaga M, Ying S, Jacobson M, Frew AJ, et al.. Influence of grass pollen immunotherapy on cellular infiltration and cytokine mRNA expression during allergen-induced late-phase cutaneous responses. J Clin Invest. 1993; 92: 644–51.
- McHugh SM, Deighton J, Stewart AG, Lachmann PJ, Ewan PW. Bee venom immunotherapy induces a shift in cytokine responses from a TH-2 to a TH-1 dominant pattern: comparison of rush and conventional immunotherapy. Clin Exp Allergy. 1995; 25: 828–38.
- Kowalski ML, Jutel M. Mechanisms of specific immunotherapy of allergic diseases. Allergy. 1998; 53: 485–92.
- O'Brien RM, Byron KA, Varigos GA, Thomas WR. House dust mite immunotherapy results in a decrease in Der p 2-specific IFN-gamma and IL-4 expression by circulating T lymphocytes. Clin Exp Allergy. 1997; 27: 46–51.
- Akdis CA, Akdis M, Blesken T, Wymann D, Alkan SS, Muller U, et al.. Epitope-specific T cell tolerance to phospholipase A2 in bee venom immunotherapy and recovery by IL-2 and IL-15 in vitro. J Clin Invest. 1996; 98: 1676–83.
- Vissers JL, van Esch BC, Hofman GA, Kapsenberg ML, Weller FR, van Oosterhout AJ. Allergen immunotherapy induces a suppressive memory response mediated by IL-10 in a mouse asthma model. J Allergy Clin Immunol. 2004; 113: 1204–10.
- Akdis CA, Blesken T, Akdis M, Wuthrich B, Blaser K. Role of interleukin 10 in specific immunotherapy. J Clin Invest. 1998; 102: 98–106.
- Francis JN, Till SJ, Durham SR. Induction of IL-10 + CD4 + CD25+ T cells by grass pollen immunotherapy. J Allergy Clin Immunol. 2003; 111: 1255–61.
- Jutel M, Akdis M, Budak F, Aebischer-Casaulta C, Wrzyszcz M, Blaser K, et al.. IL-10 and TGF-beta cooperate in the regulatory T cell response to mucosal allergens in normal immunity and specific immunotherapy. Eur J Immunol. 2003; 33: 1205–14.
- Maloy KJ, Salaun L, Cahill R, Dougan G, Saunders NJ, Powrie F. CD4 + CD25+ T(R) cells suppress innate immune pathology through cytokine-dependent mechanisms. J Exp Med. 2003; 197: 111–9.
- Sakaguchi S, Sakaguchi N, Shimizu J, Yamazaki S, Sakihama T, Itoh M, et al.. Immunologic tolerance maintained by CD25+ CD4+ regulatory T cells: their common role in controlling autoimmunity, tumor immunity, and transplantation tolerance. Immunol Rev. 2001; 182: 18–32.
- Suri-Payer E, Amar AZ, Thornton AM, Shevach EM. CD4 + CD25+ T cells inhibit both the induction and effector function of autoreactive T cells and represent a unique lineage of immunoregulatory cells. J Immunol. 1998; 160: 1212–8.
- Sakaguchi S, Sakaguchi N, Asano M, Itoh M, Toda M. Immunologic self-tolerance maintained by activated T cells expressing IL-2 receptor alpha-chains (CD25). Breakdown of a single mechanism of self-tolerance causes various autoimmune diseases. J Immunol. 1995; 155: 1151–64.
- Brunkow ME, Jeffery EW, Hjerrild KA, Paeper B, Clark LB, Yasayko SA, et al.. Disruption of a new forkhead/winged-helix protein, scurfin, results in the fatal lymphoproliferative disorder of the scurfy mouse. Nat Genet. 2001; 27: 68–73.
- Fontenot JD, Gavin MA, Rudensky AY. Foxp3 programs the development and function of CD4 + CD25+ regulatory T cells. Nat Immunol. 2003; 4: 330–6.
- Khattri R, Cox T, Yasayko SA, Ramsdell F. An essential role for Scurfin in CD4 + CD25+ T regulatory cells. Nat Immunol. 2003; 4: 337–42.
- Wildin RS, Ramsdell F, Peake J, Faravelli F, Casanova JL, Buist N, et al.. X-linked neonatal diabetes mellitus, enteropathy and endocrinopathy syndrome is the human equivalent of mouse scurfy. Nat Genet. 2001; 27: 18–20.
- Ziegler SF. FOXP3: of mice and men. Annu Rev Immunol. 2006; 24: 209–26.
- Cederbom L, Hall H, Ivars F. CD4 + CD25+ regulatory T cells down-regulate co-stimulatory molecules on antigen-presenting cells. Eur J Immunol. 2000; 30: 1538–43.
- Thornton AM, Shevach EM. CD4 + CD25+ immunoregulatory T cells suppress polyclonal T cell activation in vitro by inhibiting interleukin 2 production. J Exp Med. 1998; 188: 287–96.
- Jonuleit H, Schmitt E, Kakirman H, Stassen M, Knop J, Enk AH. Infectious tolerance: human CD25(+) regulatory T cells convey suppressor activity to conventional CD4(+) T helper cells. J Exp Med. 2002; 196: 255–60.
- Zheng SG, Wang JH, Gray JD, Soucier H, Horwitz DA. Natural and induced CD4 + CD25+ cells educate CD4 + CD25-cells to develop suppressive activity: the role of IL-2, TGF-beta, and IL-10. J Immunol. 2004; 172: 5213–21.
- Mekala DJ, Alli RS, Geiger TL. IL-10-dependent infectious tolerance after the treatment of experimental allergic encephalomyelitis with redirected CD4 + CD25+ T lymphocytes. Proc Natl Acad Sci USA. 2005; 102: 11817–22.
- Akdis CA, Blaser K. Role of IL-10 in allergen-specific immunotherapy and normal response to allergens. Microbes Infect. 2001; 3: 891–8.
- Grunig G, Corry DB, Leach MW, Seymour BW, Kurup VP, Rennick DM. Interleukin-10 is a natural suppressor of cytokine production and inflammation in a murine model of allergic bronchopulmonary aspergillosis. J Exp Med. 1997; 185: 1089–99.
- Zuany-Amorim C, Haile S, Leduc D, Dumarey C, Huerre M, Vargaftig BB, et al.. Interleukin-10 inhibits antigen-induced cellular recruitment into the airways of sensitized mice. J Clin Invest. 1995; 95: 2644–51.
- Hagenbaugh A, Sharma S, Dubinett SM, Wei SH, Aranda R, Cheroutre H, et al.. Altered immune responses in interleukin 10 transgenic mice. J Exp Med. 1997; 185: 2101–10.
- Van Oosterhout AJ, Van Esch B, Hofman G, Hofstra CL, Van Ark I, Nijkamp FP, et al.. Allergen immunotherapy inhibits airway eosinophilia and hyperresponsiveness associated with decreased IL-4 production by lymphocytes in a murine model of allergic asthma. Am J Respir Cell Mol Biol. 1998; 19: 622–8.
- Jeannin P, Lecoanet S, Delneste Y, Gauchat JF, Bonnefoy JY. IgE versus IgG4 production can be differentially regulated by IL-10. J Immunol. 1998; 160: 3555–61.
- Royer B, Varadaradjalou S, Saas P, Guillosson JJ, Kantelip JP, Arock M. Inhibition of IgE-induced activation of human mast cells by IL-10. Clin Exp Allergy. 2001; 31: 694–704.
- de Waal Malefyt R, Haanen J, Spits H, Roncarolo MG, te Velde A, Figdor C, et al.. Interleukin 10 (IL-10) and viral IL-10 strongly reduce antigen-specific human T cell proliferation by diminishing the antigen-presenting capacity of monocytes via downregulation of class II major histocompatibility complex expression. J Exp Med. 1991; 174: 915–24.
- Ding L, Linsley PS, Huang LY, Germain RN, Shevach EM. IL-10 inhibits macrophage costimulatory activity by selectively inhibiting the up-regulation of B7 expression. J Immunol. 1993; 151: 1224–34.
- Kawamura T, Furue M. Comparative analysis of B7-1 and B7-2 expression in Langerhans cells: differential regulation by T helper type 1 and T helper type 2 cytokines. Eur J Immunol. 1995; 25: 1913–7.
- Schandene L, Alonso-Vega C, Willems F, Gerard C, Delvaux A, Velu T, et al.. B7/CD28-dependent IL-5 production by human resting T cells is inhibited by IL-10. J Immunol. 1994; 152: 4368–74.
- Kitani A, Fuss I, Nakamura K, et al.. Transforming growth factor (TGF)-beta1-producing regulatory T cells induce Smad-mediated interleukin 10 secretion that facilitates coordinated immunoregulatory activity and amelioration of TGF-beta1-mediated fibrosis. J Exp Med. 2003; 198: 1179–88.
- Blaser K, Akdis CA. Interleukin-10, T regulatory cells and specific allergy treatment. Clin Exp Allergy. 2004; 34: 328–31.
- Kapsenberg ML. Dendritic-cell control of pathogen-driven T-cell polarization. Nat Rev Immunol. 2003; 3: 984–93.
- Banchereau J, Steinman RM. Dendritic cells and the control of immunity. Nature. 1998; 392: 245–52.
- Lutz MB, Schuler G. Immature, semi-mature and fully mature dendritic cells: which signals induce tolerance or immunity?. Trends Immunol. 2002; 23: 445–9.
- Dhodapkar MV, Steinman RM, Krasovsky J, Munz C, Bhardwaj N. Antigen-specific inhibition of effector T cell function in humans after injection of immature dendritic cells. J Exp Med. 2001; 193: 233–8.
- Jonuleit H, Schmitt E, Steinbrink K, Enk AH. Dendritic cells as a tool to induce anergic and regulatory T cells. Trends Immunol. 2001; 22: 394–400.
- Burkly L, Hession C, Ogata L, Reilly C, Marconi LA, Olson D, et al.. Expression of relB is required for the development of thymic medulla and dendritic cells. Nature. 1995; 373: 531–6.
- Martin E, O'Sullivan B, Low P, Thomas R. Antigen-specific suppression of a primed immune response by dendritic cells mediated by regulatory T cells secreting interleukin-10. Immunity. 2003; 18: 155–67.
- Adorini L, Penna G, Giarratana N, Roncari A, Amuchastegui S, Daniel KC, et al.. Dendritic cells as key targets for immunomodulation by Vitamin D receptor ligands. J Steroid Biochem Mol Biol. 2004; 89–90: 437–41.
- de Jong EC, Vieira PL, Kalinski P, Kapsenberg ML. Corticosteroids inhibit the production of inflammatory mediators in immature monocyte-derived DC and induce the development of tolerogenic DC3. J Leukoc Biol. 1999; 66: 201–4.
- Lee JI, Ganster RW, Geller DA, Burckart GJ, Thomson AW, Lu L. Cyclosporine A inhibits the expression of costimulatory molecules on in vitro-generated dendritic cells: association with reduced nuclear translocation of nuclear factor kappa B. Transplantation. 1999; 68: 1255–63.
- Dong X, Craig T, Xing N, Bachman LA, Paya CV, Weih F, et al.. Direct transcriptional regulation of RelB by 1alpha,25-dihydroxyvitamin D3 and its analogs: physiologic and therapeutic implications for dendritic cell function. J Biol Chem. 2003; 278: 49378–85.
- Gregori S, Casorati M, Amuchastegui S, Smiroldo S, Davalli AM, Adorini L. Regulatory T cells induced by 1 alpha,25-dihydroxyvitamin D3 and mycophenolate mofetil treatment mediate transplantation tolerance. J Immunol. 2001; 167: 1945–53.
- Gregori S, Giarratana N, Smiroldo S, Uskokovic M, Adorini L. A 1alpha,25-dihydroxyvitamin D(3) analog enhances regulatory T-cells and arrests autoimmune diabetes in NOD mice. Diabetes. 2002; 51: 1367–74.
- Belladonna ML, Grohmann U, Guidetti P, Volpi C, Bianchi R, Fioretti MC, et al.. Kynurenine pathway enzymes in dendritic cells initiate tolerogenesis in the absence of functional IDO. J Immunol. 2006; 177: 130–7.
- Fallarino F, Grohmann U, You S, McGrath BC, Cavener DR, Vacca C, et al.. The combined effects of tryptophan starvation and tryptophan catabolites down-regulate T cell receptor zeta-chain and induce a regulatory phenotype in naive T cells. J Immunol. 2006; 176: 6752–61.
- Grohmann U, Fallarino F, Bianchi R, Belladonna ML, Vacca C, Orabona C, et al.. IL-6 inhibits the tolerogenic function of CD8 alpha+ dendritic cells expressing indoleamine 2,3-dioxygenase. J Immunol. 2001; 167: 708–14.
- Munn DH, Sharma MD, Lee JR, Jhaver KG, Johnson TS, Keskin DB, et al.. Potential regulatory function of human dendritic cells expressing indoleamine 2,3-dioxygenase. Science. 2002; 297: 1867–70.
- Munn DH, Zhou M, Attwood JT, Bondarev I, Conway SJ, Marshall B, et al.. Prevention of allogeneic fetal rejection by tryptophan catabolism. Science. 1998; 281: 1191–3.
- Grohmann U, Orabona C, Fallarino F, Vacca C, Calcinaro F, Falorni A, et al.. CTLA-4-Ig regulates tryptophan catabolism in vivo. Nat Immunol. 2002; 3: 1097–101.
- Grohmann U, Fallarino F, Bianchi R, Orabona C, Vacca C, Fioretti MC, et al.. A defect in tryptophan catabolism impairs tolerance in nonobese diabetic mice. J Exp Med. 2003; 198: 153–60.
- Fallarino F, Grohmann U, Hwang KW, Orabona C, Vacca C, Bianchi R, et al.. Modulation of tryptophan catabolism by regulatory T cells. Nat Immunol. 2003; 4: 1206–12.
- Orabona C, Grohmann U, Belladonna ML, Fallarino F, Vacca C, Bianchi R, et al.. CD28 induces immunostimulatory signals in dendritic cells via CD80 and CD86. Nat Immunol. 2004; 5: 1134–42.
- Frumento G, Rotondo R, Tonetti M, Damonte G, Benatti U, Ferrara GB. Tryptophan-derived catabolites are responsible for inhibition of T and natural killer cell proliferation induced by indoleamine 2,3-dioxygenase. J Exp Med. 2002; 196: 459–68.
- Terness P, Bauer TM, Rose L, Dufter C, Watzlik A, Simon H, et al.. Inhibition of allogeneic T cell proliferation by indoleamine 2,3-dioxygenase-expressing dendritic cells: mediation of suppression by tryptophan metabolites. J Exp Med. 2002; 196: 447–57.
- Taher YA, Piavaux BJA, Gras R, Esch BCAMv, Hofman GA, Bloksma N, et al.. Indoleamine 2,3-dioxygenase-dependent tryptophan metabolites contribute to tolerance induction during allergen immunotherapy in a mouse model. J Allergy Clin Immunol. 2008; 121: 983–91.
- Bousquet J, Hejjaoui A, Dhivert H, Clauzel AM, Michel FB. Immunotherapy with a standardized Dermatophagoides pteronyssinus extract. Systemic reactions during the rush protocol in patients suffering from asthma. J Allergy Clin Immunol. 1989; 83: 797–802.
- Greineder DK. Risk management in allergen immunotherapy. J Allergy Clin Immunol. 1996; 98: S330–4.
- Taher YA, van Esch BC, Hofman G, Henricks PAJ, Van Oosterhout AJ. 1alpha,25-dihydroxyvitamin D3 potentiates the beneficial effects of allergen immunotherapy in a mouse model of allergic asthma: role for IL-10 and TGF-beta. J Immunol. 2008; 180: 5211–21.