Abstract
Background
The mechanisms of smoking tobacco leading to chronic obstructive pulmonary disease (COPD) are beginning to be understood. However, conclusions about the role of blood or lung oxidative stress markers were disparate.
Aims
To investigate the oxidative stress in blood or lung associated with tobacco smoke and to evaluate its effect on pulmonary function data and its relation with physical activity.
Methods
It is a case-control study. Fifty-four male-smokers of more than five pack-years (PY) and aged 40–60 years were included (29 Non-COPD, 16 COPD). Physical activity score was determined. Blood sample levels of malondialdehyde (MDA), protein-cys-SH (PSH), and Glutathione (GSH) were measured. Fractional exhaled nitric oxide (FeNO) and plethysmographic measurements were performed. Correlation coefficients (r) evaluated the association between oxidative stress markers and independent variables (plethysmographic data and physical activity score).
Results
Non-COPD (48±6 years) and COPD (49±5 years) groups had similar tobacco consumption patterns, that is, 27±14 PY versus 30±19 PY, respectively. Compared to the Non-COPD group, the COPD group had significantly lower levels of GSH and PSH, that is, mean±SE were 40±6 versus 25±5 µg/mL and 54±10 versus 26±5 µg/g of hemoglobin, respectively. However, MDA level and FeNO values were similar. In the COPD group, none of the oxidative stress markers was significantly correlated with plethysmographic data or physical activity score. In the Non-COPD group, GSH was significantly correlated with physical activity score (r=0.47) and PSH was significantly correlated with total lung capacity (TLC) (r=−0.50), residual volume (r=0.41), and physical activity score (r=0.62). FeNO was significantly correlated with TLC of the COPD group (r=−0.48).
Conclusion
Compared to the Non-COPD group, the COPD group had a marked decrease in blood antioxidant markers (GSH and PSH) but similar blood oxidant (MDA) or lung (FeNO) burden.
Chronic obstructive pulmonary disease (COPD) continues to cause heavy health and economic burden around the world (Citation1) and in North Africa, in particular (Citation2). This disease defines a group of chronic inflammatory pulmonary disorders characterized by airflow limitation that is not fully reversible (Citation3, Citation4). It is accompanied by systemic manifestations causing serious impact on the quality of life and survival of patients, including dyspnea, nutritional depletion, and skeletal muscle dysfunction, which contribute to exercise intolerance (Citation5). The clinical and functional manifestations of COPD result from lung injury occurring through various mechanisms, including chronic inflammation, oxidative stress, protease/antiprotease imbalance, and apoptosis (Citation6). Chronic inflammation is usually the result of tobacco smoke (80–90% of cases); other factors, particularly occupational exposures, may play a role (Citation3). Oxidative stress is central in the pathogenesis of COPD, because smoking is associated with increased oxidative stress in the lungs (Citation7, Citation8).
The term ‘oxidative stress’ describes the consequences of an imbalance between oxidant–antioxidant balance, with the outcome being favorable to oxidants (Citation7). The highly free radicals mostly represent oxidants. The last can be centered on oxygen (reactive oxygen species (ROS), such as malondialdehyde (MDA)) or nitrogen (nitrogen reactive species (NRS), such as nitric-oxide (NO)). MDA and NO are toxic products associated with lipid peroxidation and react with protein and deoxyribonucleic-acid (Citation9, Citation10). Biological systems are exposed to pollution, cigarette smoke and generate (production by phagocytes and mitochondria) continuously oxidizing (Citation10). To oppose their potential deleterious effects, living organisms, and lung in particular, have developed a sophisticated antioxidant system comprising several molecules, especially glutathione (GSH) and protein-cys-SH (PSH) (Citation6–Citation8, Citation11) (Citation12). GSH is one of the most effective enzymatic antioxidant and its activity appears to be an important feature in determining oxidative damage (Citation9, Citation13). PSH possesses antioxidant properties which play an important role in protecting biological systems against the oxidative stress (Citation14).
In practice, oxidative stress can be assessed and monitored through the determination of the levels of biomarkers in different biological samples, especially serum and exhaled-breath-condensate (EBC) (Citation9). In a recent study (Citation15) aiming to evaluate the difference in the burden of oxidative stress in patients with COPD, smokers, and non-smokers by measuring oxidative stress markers in the EBC samples, authors found significantly higher levels of 8-isoprostane and H2O2 in the COPD and smokers groups than in the non-smokers group. However, levels were similar between smokers and COPD subjects (Citation15). In addition, MDA levels were similar between the three groups and there was no correlation between oxidative stress markers levels and pulmonary function data (Citation15). The authors concluded that even if respiratory function tests are within normal limits, oxidant burden in smokers’ lungs is equivalent to that in COPD patients (Citation15). What about oxidative stress in smokers and COPD blood? Previous studies have demonstrated that smokers have higher levels of oxidative stress biomarkers compared to healthy non-smokers (Citation16, Citation17). In COPD patients, data are controversial: higher levels of MDA and lower levels of PSH and GSH (Citation18–Citation20), higher level of GSH in COPD patients when compared with either healthy non-smokers or smokers free from COPD (Citation21, Citation22). Some plausible explanations of the oxidative stress results discordance could be subjects’ different physical activity status (Citation23, Citation24) or socioeconomic levels (SELs) (Citation25, Citation26).
The present study aims to investigate the blood and lung oxidative stress markers of smokers free from COPD (Non-COPD) and with COPD, and to evaluate its effect with pulmonary function data and its relation with physical activity score.
Populations and methods
Type of study
The present case-control study was performed over an 8-month period (April to November 2013) in Farhat HACHED Hospital (Sousse, Tunisia). The city lies on the Gulf of Hammamet on the Mediterranean Sea and has 173,047 inhabitants (year 2010). In a recent local population-based prevalence survey (Citation2), a history of current or past smoking was found in 47.4 and 74.3% of men. In addition, 7.8% of the residents aged 40 years or over had COPD, and this was more common in males than in females (Citation2).
The study was conducted in accordance with the Declaration of Helsinki. Participants provided written consent and the study protocol was approved by the ethics committee of the hospital (approval number 2204/2013).
Sample size
The null hypothesis (Citation27) was H 0 : m 1 =m 2 and the alternative hypothesis was H a : m 1 =m 2+d, where d is the difference between two means and n 1 and n 2 are the sample sizes for the Non-COPD and COPD smokers groups, such N=n 1+n 2. The total sample size was estimated using the following formula (Citation27): N=[(r+1)(Z α/2+Z 1-β ) 2 σ 2 ]/r d 2. Z α is the normal deviate at a level of significance of 1.96 (5% level of significance), Z 1-β is the normal deviate at 1-β% power with β% of type II error (0.67 at 75% statistical power); ‘r’ equal to n1/n2 is the ratio of sample size required for two groups (r=0.5 gives the sample size distribution as 1:2 for two groups). ‘r’ was considered because of the unequal sample sizes for various reasons, such as higher prevalence of smokers free from COPD compared to smokers with COPD (among 661 higher smokers more than 20 pack-years (PY, i.e., one PY equals one pack a day for 1 year), only 16% had COPD (Citation2)). σ and d are the pooled standard deviation (SD) and difference of MDA in EBC means of two groups of smokers (Non-COPD and COPD). These two values were obtained from a previous study based on a similar hypothesis (Citation15) in which the researchers found that the mean MDA (µmol/l) in two groups of smokers (COPD versus Non-COPD) were 0.08 and 0.07 and common SD was 0.015. The total sample size for the study was 45 smokers (29 Non-COPD and 16 COPD).
Population
COPD patients were recruited from the pulmonary department of the local hospital. Non-COPD smokers were selected by convenience sampling from the staff of the local faculty of medicine or hospital, as well as from acquaintances of people involved in the study.
Only male smokers aged 40–60 years without a history of atopy and free from asthma, allergies, pulmonary tuberculosis, or recent respiratory tract infection were included. Imperfect performance of the respiratory maneuvers was applied as an exclusion criterion. Only smokers of more than 5 PY were included. The smoker must have stopped smoking for at least 6 h (Citation15, Citation28) and was asked to fast (no eating or drinking) for at least 10 h before coming to the hospital. Subjects were asked to not participate in strenuous activity for 1 h prior to the fractional exhaled nitric oxide (FeNO) measurements (Citation29).
Smokers presenting an acute response in the first-second-forced-expiratory-volume (FEV1) and/or forced vital capacity (FVC) to inhaled bronchodilator of more than 20% of initial values were excluded. Smokers were divided into two groups, taking into account the post-bronchodilator FEV1/FVC ratio. Smokers with a post-bronchodilator FEV1/FVC ratio >0.70 were considered as free from COPD and qualified as smokers Non-COPD group (Non-COPD) (Citation3, Citation4). Those with a post-bronchodilator FEV1/FVC ratio ≤0.70 were considered as smokers COPD patient group (Citation3, Citation4).
Subjects with COPD were clinically stable, with no worsening of symptoms, need of increase in medication, emergency care, or hospitalization within the previous 4 weeks. Duration of the COPD and medical treatments were recorded. COPD subjects treated with inhaled corticosteroids (budesonide 200–400 µg/d, fluticasone 500–1,000 µg/d), inhaled β-adrenergic agonists, theophylline oral or inhaled N-acetylcysteine or vitamin C and/or E were excluded.
Collected data
Smokers were evaluated for 1 day. The study protocol was in the following order: fasting blood sample (MDA, PSH, and GSH levels); response to medical questionnaire (Citation30) (schooling level (SL) and SEL, cigarette consumption, sputum, cough, dyspnea, chest pain, whistling, medical and surgical histories, and medication use) and physical activity questionnaire (Citation31) (household, sporting, leisure, and physical activity scores); and anthropometric data measurement (age, weight, height, body mass index (BMI)); FeNO assessment and plethysmographic data measurement (FVC, FEV1, FEV1/FVC, mid maximal expiratory flow (MMEF), slow vital capacity (SVC), thoracic gas volume (TGV), residual volume (RV), and total lung capacity (TLC)).
Clinical, SL, SEL, tobacco use, and sports activity evaluations
Two groups (Yes/No) of smokers were defined according the presence or the lack of cough, sputum-production, dyspnea, chest pain, and whistling (Citation30).
Two SLs were defined (Citation32): low (illiterate, primary education); and high (secondary and university education).
Two SELs were defined (Citation32): unfavorable (unskilled worker, jobless); and favorable (skilled worker, farm owner, manager).
Active smoking was assessed by a series of questions about past and current consumption. Cigarette use was evaluated in PY.
A translated version of the Voorrips et al. (Citation31) physical activity questionnaire was filled out by each smoker, and household, sporting, and leisure activities were evaluated to yield a total physical activity score (Citation31). It is a validated physical activity questionnaire for young and elderly apparently healthy people (Citation31). The questionnaire provides a reliable and valid method for classifying subjects into categories of high, medium, and low physical activity (Citation31). According to the total physical activity score, two groups were defined (non-active (score<9.4); and active (score≥9.4)).
Physical examination
Anthropometric data were verified, measured, or calculated: age (year), height (m), weight (kg), BMI (kg/m2). Height (±0.01 m) was measured with a height gauge (standing stadiometer type DETECTO®) with shoes removed, heels joined, and back straight. Weight (±1 kg) was measured with a digital scale (OHAUS, Florhman Park, NJ, USA), and BMI (weight/height2) was calculated. Depending on BMI, the smokers were classified as non-obese (BMI<30) and obese (BMI≥30) (Citation33).
Blood sample
Venous blood was collected into dry and heparin tubes. Blood samples were immediately centrifuged at 3,000 rpm for 10 min, divided into plasma, serum, and red blood cells, and then stored at −80°C. MDA level was estimated based on the method using thiobarbituric acid (Citation34). Two colors (red–pink) were quantified by spectrophotometer at 535 nm (SPEROL 1,500, Analytic Jena). PSH level was determined by subtracting the non-protein thiols from total thiols (Citation35). GSH level was determined based on the color complex formation of non-protein sulfydryl groups, which were separated by deproteinization with trichloroacetic acid (Citation35). High level of MDA and low levels of GSH and PSH are signs of a significant oxidative stress (Citation36).
FeNO measurement
The FeNO (ppb) was measured by Medisoft HypAir FeNO method using an electrochemical analyzer (Medisoft, Sorinnes (Dinant), Belgium). International recommendations were respected (Citation37). The instrument was calibrated and used according to the manufacturer's instructions and worked in conjunction with a personal computer. The software supplied by the manufacturer provided both audio and visual feedback allowing the participant to maintain a constant exhaled breath flow rate (Citation37).
The online method, with constant flow rate was used (Citation37). After a full unforced exhalation outside the mouthpiece, a maximal inspiration was performed through an absorber to ensure NO-free air. The subject then performed a controlled exhalation using flow control at an exhalation pressure of 4–10 cmH2O for at least 10 sec, during which time sample collection and gas analysis was performed. Nasal contamination is presented by closure of the velum by using five cmH2O oral back-pressures. A nose clip was not used. The subjects maintained the required flow rate of 50 mL/sec with the aid of visual feedback from the computer screen. Three acceptable measurements were taken within a 15-min period (Citation37). The mean of the three values was used (Citation37).
Lung function measurements and applied definition
The spirometric measurements were performed with a body plethysmograph (ZAN 500 Body II Meβgreräte GmbH, Germany), carefully following international recommendations (Citation38, Citation39).
The plethysmographic technique followed these steps: 1) the procedure was explained to smokers in detail; 2) the plethysmograph door was closed and time was allotted for thermal transients to stabilize, and patients to relax; 3) smokers were instructed to attach the mouthpiece and breathe quietly until they achieved stable end-expiration, 4) when smokers were at or near TGV, the shutter was closed at end-expiration for 2–3 sec, and they were instructed to perform a series of gentle pants at a frequency of 0.5–1.0 Hz; 5) after a series of 3–5 technically satisfactory pants, maneuvers were recorded; 6) the shutter opened and patients performed an expiratory reserve volume maneuver followed by a SVC maneuver; 7) with regards to repeatability, at least three TGV values that agreed within 5% were obtained and the mean value reported.
The FVC maneuver had three distinct phases: maximal inspiration, a blast of exhalation, and continued complete exhalation to the end of testing. Smokers were verbally encouraged to continue exhaling air at the end of the maneuver to obtain optimal effort. The criterion for end of testing was a volume–time curve showing no change in volume (25 mL) for 1 sec, despite the patient's effort to exhale for at least 6 sec. Repeatability was acceptable when the difference between the largest and the next largest FVC was <150 mL, and the difference between the largest and next largest FEV1 was <150 mL. Plethysmographic parameters were measured or calculated before/post-bronchodilator inhalation of 400 µg Salbutamol® via a large volume spacer (Citation40) and compared with local predicted values (Citation41).
Lung hyperinflation was defined as an abnormal increase in RV higher than the upper limit of normal (Citation42). COPD diagnosis was retained when the post-bronchodilator FEV1/FVC ratio was lower than 0.70 (Citation3, Citation4).
Statistical analysis
Variables distributions were normal.
Smokers’ characteristics and lung function data of the two groups were expressed as mean±SD and/or 95% confidence interval (95% CI).
Biological markers were expressed as mean±standard error (SE) and 95% CI.
Student t-test was used to compare means of quantitative data (physical scores, anthropometric, lung function data, and oxidative stress markers), and chi-square test was used to compare qualitative data (obesity and socioeconomic status, clinical and pathological data).
Pearson product–moment correlation coefficients (r) evaluated the associations between oxidative stress markers and independent variables such as lung function data expressed as percentage of predicted values (FEV1, TLC, and RV), FeNO, and physical activity score. In addition, correlation coefficients were used to evaluate the associations between FeNO and the above lung function data and physical activity score.
All mathematical computations and statistical procedures were performed using Statistica statistical software (Statistica Kernel version 6; Stat Software. France). Significance was set at the 0.05 level.
Results
Characteristics of the two groups
presents the characteristics of the two groups. There were no statistically significant differences between their ages or the amounts of tobacco they used. Compared to Non-COPD group, the COPD one had significantly:
Lower height, weight, and BMI. However, the two groups contain statistically similar percentages of obese smokers.
Lower percentage of subjects having a high SL.
Lower household, sporting, leisure, and physical activity scores.
Higher percentages of ‘sputum-producers’ and dyspnea smokers.
Table 1 Clinical characteristics of the two groups: smokers’ Non-COPD and COPD
FeNO and plethysmographic data
presents the FeNO and the plethysmographic data of the two groups. Compared to Non-COPD group, the COPD group had significantly lower FEV1 (L,%), FVC (L), FEV1/FVC ratio (absolute value), MMEF (L,%), and SVC (L). However, no significant difference was found between the two groups’ static volumes (TLC, RV, or TGV) or FeNO data.
Table 2 Fractional exhaled nitric oxide (FeNO) and plethysmographic data of the two groups: smokers’ Non-COPD and COPD
The percentage of smokers with lung-hyperinflation was similar between the COPD and the Non-COPD groups, 4 (25%) versus 4 (14%), respectively, p=0.36.
presents the correlations between FeNO and lung function data and physical activity score in the two groups. FeNO was significantly correlated only with TLC of the COPD group.
Table 3 Correlation coefficient between fractional exhaled nitric oxide (FeNO) and lung function data and physical activity score in the two groups: smokers’ Non-COPD (n=29) and COPD (n=16)
Oxidative stress markers levels
presents the levels of oxidative stress markers in the two groups. Compared to the Non-COPD group, the COPD group had significantly lower levels of GSH and PSH, mean±SE, were 40.46±5.56 versus 24.67±5.41 µg/mL and 53.59±10.44 versus 25.72±5.03 µg/g of hemoglobin, respectively. However, MDA level was similar for the two groups, means±SE were 15.79±0.94 versus 12.73±1.49 µmol/L, respectively.
Fig 1. Oxidative stress levels of the two groups: smokers Non-COPD (Non-COPD) and smokers COPD patients (COPD). a) Malondialdehyde (MDA) level measured in 26 Non-COPD and 15 COPD smokers. b) Glutathione (GSH) level measured in 24 Non-COPD and 15 COPD smokers. c) Protein sulfhydryl (PSH) level measured in 24 Non-COPD and 16 COPD.
Data are shown as box-and-whisker plots illustrating the mean (), ±standard-error (), and 95% confidence interval (). p (t-test): comparison of levels of oxidative stress markers between the two groups.
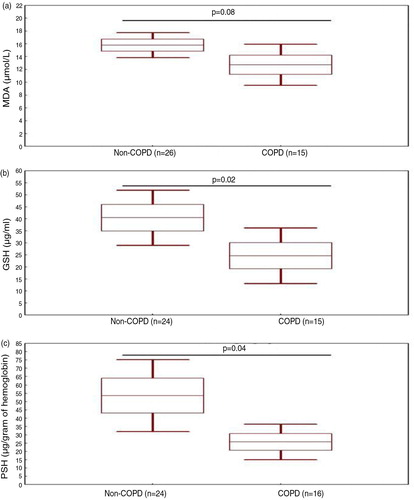
presents the levels of oxidative stress markers in the two groups divided according to the obesity status. Comparison of the non-obese versus obese smokers for the same smokers group revealed a significant statistical difference only for the GSH of the COPD group (it was significantly higher in the obese subgroup compared to the non-obese group). Comparison of the Non-COPD versus COPD smokers for the same obesity status revealed that the COPD obese subgroup has a significantly lower MDA level when compared to the Non-COPD obese subgroup. In addition, the COPD non-obese subgroup has significantly lower levels of PSH and GSH when compared with the Non-COPD non-obese subgroup.
Table 4 Oxidative stress markers of the two groups (smokers’ Non-COPD and COPD) divided according to the obesity status
presents the correlations between oxidative stress markers and lung function data and physical activity score in the two groups. In the COPD group, none of the oxidative stress markers was significantly correlated with lung function data or physical activity score. However, in the Non-COPD group, GSH was significantly correlated with physical activity score (r=0.47), and PSH was significantly correlated with TLC, RV, and physical activity score (respectively, r=−0.50, r=0.41 and r=0.62).
Table 5 Correlation coefficient between oxidative stress markers and lung function data and physical activity score in the two groups: smokers’ Non-COPD (n=29) and COPD (n=16)
Discussion
We compared two age- and amount of tobacco used-matched groups of smokers of more than 5 PY: 29 Non-COPD and 16 COPD. Compared to the Non-COPD group, the COPD group had significantly lower levels of GSH and PSH. However, MDA level and FeNO values were similar for the two groups. Therefore, the null hypothesis, that there is no difference between the MDA levels of the two groups is retained. In the COPD group, none of the oxidative stress markers was significantly correlated with lung function data or physical activity score. However, in the Non-COPD group, GSH was significantly correlated with physical activity score and PSH was significantly correlated with TLC, RV, and physical activity score. FeNO was significantly correlated only with TLC of the COPD group.
Study limitations
Convenience sampling is a statistical method of drawing representative data by selecting people because of the ease of their volunteering (Citation43). Its advantages are the availability and the quickness with which data can be gathered (Citation43). Its disadvantages are the risk that the sample might not represent the population as a whole, and it might be biased by volunteers (Citation43).
In the present study, we have compared oxidative stress levels of Non-COPD smokers with these of COPD smokers. Other authors have compared COPD subjects with both healthy smokers and healthy non-smokers (Citation15) or have compared smokers’ subjects with both passive smokers and non-smokers without tobacco smoke exposure (Citation9). However, taking three groups in a single study seems to have limited precedence in literature and raises some substantive questions such as whether the prevalence of COPD in the three groups is comparable.
Occupational exposure is a risk factor for COPD (Citation3). For that reason, socioeconomic evaluation, based on the job of the smoker, was done and the SELs of the two groups was compared (). There was no significant difference between the two groups SELs, therefore, we can speculate that they were controlled (matched) for occupational exposures.
The present calculated sample size (n=45 smokers: 29 Non-COPD and 16 COPD) seems to be satisfactory. It is closer to that of Inonu et al. (Citation15) (n=51 smokers: 26 Non-COPD and 25 COPD) but smaller to that of Hanta et al. (Citation44) (n=101 smokers: 30 Non-COPD and 71 COPD) or to that of Arja et al. (Citation19) (n=386 smokers: 150 Non-COPD and 236 COPD).
Cigarette smoke is a complex mixture of chemical compounds and smoking is associated with increased oxidative stress in the lungs (Citation9). There are many studies in literature reporting an increase in lipid peroxidation and in the release of oxygen radicals, decreased antioxidant capacity, and an imbalance of oxidant/antioxidant status in smokers (6–12, 15).
Oxidative stress can be assessed and monitored through the determination of the levels of biomarkers in different biological samples, such as serum, urine, bronchoalveolar-lavage-fluid, induced sputum, bronchial biopsy, and EBC (Citation9, Citation15). In the present study, the choice was made for blood sample, which is a little bit invasive method, and FeNO. However, it was desirable to add collection of EBC, suggested as a simple, non-invasive, and easily repeatable procedure. In addition, samples from the lower respiratory tract for monitoring airway inflammation and oxidative stress can be obtained with this technique (Citation9, Citation15).
Several oxidative stress markers are measurable in lung or in blood. In the present study, two oxidative stress markers were evaluated, one in blood (MDA) and one in lung (FeNO); and two blood anti-oxidant markers (GSH and PSH) were measured. Some authors have opted for other oxidative stress markers such as 8-Isoprostane and hydrogen peroxide (Citation15) or 8-hydroxydeoxyguanosine, nitrite/nitrate, vitamin C, superoxide dismutase, and GSH peroxidase (Citation9).
Correlations between oxidative stress markers and initial lung function parameters were analyzed. Three plethysmographic parameters, expressed as percentage of predicted values were chosen (): FEV1, TLC, and RV. FEV1 is a sensitive index for proximal airways, highly reproducible (Citation38). This index selection is the most widely used among the indices of forced expiration to study the variations of lung obstruction after bronchodilator use (Citation40). TLC and RV are two volumes often used in practice to evaluate lung hyperinflation, a pattern frequently seen in smokers (Citation42). Lung hyperinflation, a major functional consequence of COPD, is well correlated with dyspnea (Citation45). In addition, correlations between FeNO values and the above plethysmographic parameters were also evaluated. In a recent study, done on Tunisian healthy non-smoking adult males, FeNO values were not correlated with plethysmographic data (Citation29) and to the best of our knowledge, no study has evaluated the correlation between smokers’ FeNO and lung volumes, such as TLC or RV.
The present study presented three limitations. The first one was about diurnal variations of some analyzed markers (Citation15). As stated by Inonu et al. (Citation15) repeated measurements at different hours of the day could improve the reliability of the results. As in other studies (Citation9, Citation15), repeated measurements were not used in the present one, but all blood samples were taken at the same time of day to avoid possible differences due to diurnal changes. The second limitation was about females’ non-inclusion. As in other studies (Citation9, Citation15), the study groups were more homogeneous in order to exclude changes caused by sex differences (Citation15). The last limitation was about inclusion of obese smokers. Obesity induces systemic oxidative stress in part through increased production of ROS in adipose tissue (Citation46). It is hypothesized that the lung serves as a target organ for this oxidative stress (Citation46). This is manifested as increased oxidation of airway NO into nitrate and NRS and hence reduction of NO bioavailability and exhaled NO levels (Citation46). In the present study (), 12/49 (27%) smokers (10 Non-COPD and 2 COPD) were obese. First, this percentage was similar to that described in Tunisian general population (Citation47) (prevalence of obesity in the total population was 28%) and the present study sample could be a ‘representative sample’ of Tunisian population. Second, the present study COPD sample was also representative of COPD population where obesity prevalence is around 18% (Citation48). In a recent local study (Citation42), it was found that 13% of the 366 COPD smokers have obesity. Third, the two groups of Non-COPD and COPD contain statistically similar percentages of obese smokers. At least, when we have looked for correlation between oxidative stress markers and lung function data, the last were expressed as percentage predicted values and so adjusted to anthropometric data, especially weight. In addition, data show, paradoxically, that obese smokers have a statistically higher level of anti-oxidant marker (GSH) when compared with non-obese smokers.
Oxidative stress markers: FeNO and MDA
NO is one of the major ROS in cigarette smoke (Citation49). In the present study, FeNO levels were similar in the two groups. Assessment of FeNO in patients with COPD provides seemingly conflicting results: it has been reported to range from low (Citation50) to elevated (Citation51) values when compared to those of healthy subjects, with several studies reporting no difference between these groups (Citation52–Citation55). Maziak et al. (Citation56) showed that COPD patients, particularly those with unstable disease, have higher levels of FeNO than smokers with chronic bronchitis. These results were supported by Kanazawa et al. (Citation54) who found higher FeNO levels in COPD patients than in healthy controls. However, some other authors (Citation52, Citation53) (Citation55) reported no differences in FeNO levels between stable COPD patients and healthy controls. In addition, FeNO was not associated with the severity of COPD (Citation57). This variability may in part be explained by the heterogeneous nature of the disease, with multiple factors influencing FeNO. At the alveolar level, NO rapidly combines with reduced hemoglobin and is therefore scavenged by pulmonary capillary blood (Citation51). In the presence of an altered ventilation–perfusion mismatch in COPD, this scavenging will take place less efficiently (Citation51). Destruction of alveolar epithelial cells in more severe COPD may influence NO production in the airways, and the development of cor pulmonale in severe COPD may reduce FeNO, presumably as a reflection of endothelial injury. Furthermore, smoking (current or former) influences the composition of the airway inflammation and thus FeNO formation (Citation58).
MDA is the most mutagenic product of lipid peroxidation and can be determined in serum, bronchoalveolar lavage fluid, and tissues (Citation10). Lipid peroxidation describes the oxidation reactions between ROS and polyunsaturated fatty acids, it reflects the degradation of lipids by oxidative stress inducing changes in the structure and the permeability of lung membrane and causing a loss of selectivity of ion exchange (Citation44). In the present study, MDA levels were similar between the two smokers’ groups. Literature data about MDA in smokers are controversial. Morrison et al. (Citation59) have shown that lipid peroxidation products in plasma and bronchoalveolar lavage measured as thiobarbituric acid reactive substance were higher in smokers compared to non-smokers. This result was confirmed by other authors (Citation9, Citation60). Hanta et al. (Citation44) showed that the plasma concentration of MDA was higher in smokers Non-COPD and COPD patients than in healthy non-smokers. This result was recently confirmed by Arja et al. (Citation19) who showed that COPD patients had higher MDA levels compared to smokers Non-COPD, 3.23±0.57 and 1.46±0.14 nmol/mL, respectively.
Anti-oxidative stress markers: GSH and PSH
Cigarette smoke affects antioxidant defense of the lungs (Citation61). GSH is one of the most effective enzymatic endogenous antioxidants and its activity appears to be an important feature in determining oxidative damage (Citation9, Citation13). In the present study, compared to the Non-COPD group, the COPD group had a significantly lower level of GSH. This result is in agreement with other studies (Citation19, Citation62) (Citation63) but in contrast with other studies showing an increase (Citation21) or no changes (Citation22) in the GSH concentration in COPD. Other studies have shown that smokers have low plasma antioxidant defenses (Citation59). Smoking plays a key role in lung diseases such as COPD, and cigarette smoke contains millions of oxygen free radicals (Citation64), so it may be the cause of the decrease in the content of GSH. This is confirmed by studies showing a reduction of GSH in plasma after exposure to cigarette smoke (Citation65). Additional studies are necessary to disclose the exact mechanisms primarily responsible. The decline in GSH levels may reflect a decrease in antioxidant capacity of lung tissue in patients with COPD as the GSH redox cycle is a fundamental element of the antioxidant defense system (Citation66). The oxidant–antioxidant balance is affected by the decrease in antioxidant mechanisms. Indeed, deficiencies in antioxidant enzymatic and non-enzymatic systems have been described in patients with COPD (Citation67). This depletion can be explained by the increased oxidants produced by activated circulating neutrophils in COPD (Citation68), directly and indirectly through the recruitment and activation of inflammatory cells by cigarette smoke (Citation69). Thus, additional studies are needed to investigate the regulation of GSH levels in the lungs of smokers and patients with COPD to design therapy antioxidant GSH.
PSH possesses antioxidant properties which play an important role in protecting biological systems against the oxidative stress (Citation14). PSH can take part in the protection of cells against free radicals and at the same time they are involved in the regulation of cellular homeostasis’ (Citation70). These proteins appear to be targets for ROS. Their oxidation leads to the formation of disulfide bonds between SH groups, which decrease their content (Citation71). Compared to the Non-COPD group, the COPD group had significantly lower level of PSH. This result is consistent with those of other studies (Citation65, Citation72). Protein damages can often be more important than those of lipids in oxidative stress conditions (Citation73). It is well known that oxidative damage of proteins results in decreased levels of PSH (Citation74). The decline of PSH is a marker of oxidative stress. According to Nagler et al. (Citation75) cigarette smoke is the cause of this PSH depletion.
Correlation between oxidative stress markers and plethysmographic data or physical activity score
In the present study, FeNO was negatively and significantly correlated only with TLC of the COPD group (). Dweik et al. (Citation76) found that TLC increased linearly with increased air trapping as measured by elevated ratio of RV to TLC. The independent association of elevated FeNO with increased TLC is a novel finding and suggests that there is an inflammatory component affecting lung mechanics that is separate from the air trapping mechanism in asthma. However, this hypothesis did not explain the present study result in COPD. Doruk et al. (Citation9) did not detect any correlation between NO levels and pulmonary function test data and they hypothesized that their result could be associated with reversible decrease in NO levels. Indeed, in many studies it was reported that NO levels decrease in smokers. This reduction is reversible and NO levels increase after smoking cessation (Citation9). Cigarette smoke may inhibit NO production by multiple mechanisms. Cigarette smoking causes a transient decrease in exhaled NO that returns to baseline levels within 15 min. This transient effect is consistent with the known inhibition of NO-synthase activity by NO which is present in cigarette smoke in high concentrations (Citation77). However, this transient decrease would not seem to explain the lower levels of exhaled NO in cigarette smokers that were observed after abstinence for at least 8 h. This more sustained reduction would be more consistent with the reduction of the transcription of the inducible form of NO-synthase, the family of enzymes responsible for the NO production.
As in the study of Doruk et al. (Citation9), no correlation was found between MDA levels and plethysmographic data (). It is known that MDA is not the most powerful marker of pulmonary oxidative stress (Citation9). There are other different lipid peroxidation products such as the 8-Isoprostane. The last is the most widely studied marker of systemic and pulmonary oxidative stress (Citation78), and as one of the mediators of the reversible component of obstruction in patients with COPD (Citation79); and lipid peroxidation was obviously increased in patients with COPD especially at severe stage of this disease (Citation72). As found by Doruk et al. (Citation9), no correlation was found between GSH levels and plethysmographic data ().
PSH was correlated with TLC of the COPD group and with the RV of the Non-COPD group (). The correlation between PSH and lung volumes can suggest that oxidative stress and lung hyperinflation are positively related. Indeed, Garcia-Rio et al. (Citation80) showed that in stable patients with COPD, dynamic hyperinflation is related to the level of airway oxidative stress.
Physical activity score was significantly correlated with the GSH and the PSH levels of the Non-COPD group (). In the present study, the Non-COPD group was more active than the COPD group (). It has recently been suggested that regular exercise reduces lung function decline and risk of COPD among active smokers; and one plausible mechanism involved in this effect was decrease in oxidative stress markers and/or increase of antioxidant markers (Citation24). As other authors (Citation24), we can hypothesize that regular aerobic physical training attenuates the development of pulmonary disease induced by cigarette smoke exposure.
Future recommendations
In smokers free from COPD, the associations determined between physical activity score and GSH and/or PSH, or between PSH and lung volumes suggested that these biomarkers might be used in early diagnosis of airway disease. It would be very interesting to investigate the oxidative stress in the blood associated with tobacco smoke and to evaluate its effect with a 6-min-walk-test (Citation81) and its relation with physical activity.
In conclusion, compared to smokers free from COPD, those with COPD had a marked decrease in the blood antioxidant markers (GSH and PSH) but similar blood oxidant (MDA) or lung (FeNO) burden.
Conflict of interest and funding
The authors declare that there are no conflicts of interest.
Acknowledgements
Authors wish to thank Professor Dhouha Boukeri for her invaluable contribution in the improvement of the quality of the writing in the present paper.
References
- Burney P, Jithoo A, Kato B, Janson C, Mannino D, Nizankowska-Mogilnicka E. Chronic obstructive pulmonary disease mortality and prevalence: the associations with smoking and poverty – a BOLD analysis. Thorax. 2014; 69: 465–73. [PubMed Abstract] [PubMed CentralFull Text].
- Daldoul H, Denguezli M, Jithoo A, Gnatiuc L, Buist S, Burney P, etal. Prevalence of COPD and tobacco smoking in Tunisia – results from the BOLD study. Int J Environ Res Public Health. 2013; 10: 7257–71. [PubMed Abstract] [PubMed CentralFull Text].
- Vestbo J, Hurd SS, Agusti AG, Jones PW, Vogelmeier C, Anzueto A, etal. Global strategy for the diagnosis, management, and prevention of chronic obstructive pulmonary disease: GOLD executive summary. Am J Respir Crit Care Med. 2013; 187: 347–65. [PubMed Abstract].
- Ben Saad H, Ben Amor L, Ben Mdella S, Ghannouchi I, Ben Essghair M, Bougmiza I. The diagnosis of COPD is recommendation dependent. Tunis Med. 2014. (In press)..
- Ben Saad H, Hamadou R, Ben Cheikh I, Chouchene A, Rejeb N, Zbidi A, etal. Respiratory rehabilitation of chronic obstructive pulmonary disease patients: preliminary data of Tunisian experience. J Med Rehabil. 2008; 28: 138–47.
- MacNee W. Pathogenesis of chronic obstructive pulmonary disease. Proc Am Thorac Soc. 2005; 2: 258–66. [PubMed Abstract] [PubMed CentralFull Text].
- MacNee W, Rahman I. Is oxidative stress central to the pathogenesis of chronic obstructive pulmonary disease?. Trends Mol Med. 2001; 7: 55–62. [PubMed Abstract].
- Rahman I, MacNee W. Role of oxidants/antioxidants in smoking-induced lung diseases. Free Radic Biol Med. 1996; 21: 669–81. [PubMed Abstract].
- Doruk S, Ozyurt H, Inonu H, Erkorkmaz U, Saylan O, Seyfikli Z. Oxidative status in the lungs associated with tobacco smoke exposure. Clin Chem Lab Med. 2011; 49: 2007–12. [PubMed Abstract].
- Kanabrocki EL, Ryan MD, Murray D, Jacobs RW, Wang J, Hurder A, etal. Circadian variation in multiple sclerosis of oxidative stress marker of DNA damage, A potential cancer marker?. Clin Ter. 2006; 157: 117–22. [PubMed Abstract].
- MacNee W. Pulmonary and systemic oxidant/antioxidant imbalance in chronic obstructive pulmonary disease. Proc Am Thorac Soc. 2005; 2: 50–60. [PubMed Abstract].
- Macnee W, Rahman I. Oxidants and antioxidants as therapeutic targets in chronic obstructive pulmonary disease. Am J Respir Crit Care Med. 1999; 160: S58–65. [PubMed Abstract].
- Chow CK. Nutritional influence on cellular antioxidant defense systems. Am J Clin Nutr. 1979; 32: 1066–81. [PubMed Abstract].
- Abraham P, Wilfred G, Ramakrishna B. Oxidative damage to the hepatocellular proteins after chronic ethanol intake in the rat. Clin Chim Acta. 2002; 325: 117–25. [PubMed Abstract].
- Inonu H, Doruk S, Sahin S, Erkorkmaz U, Celik D, Celikel S, etal. Oxidative stress levels in exhaled breath condensate associated with COPD and smoking. Respir Care. 2012; 57: 413–19. [PubMed Abstract].
- Lodovici M, Casalini C, Cariaggi R, Michelucci L, Dolara P. Levels of 8-hydroxydeoxyguanosine as a marker of DNA damage in human leukocytes. Free Radic Biol Med. 2000; 28: 13–17. [PubMed Abstract].
- Morrow JD, Frei B, Longmire AW, Gaziano JM, Lynch SM, Shyr Y, etal. Increase in circulating products of lipid peroxidation (F2-isoprostanes) in smokers. Smoking as a cause of oxidative damage. N Eng J Med. 1995; 332: 1198–203.
- Mak JC. Pathogenesis of COPD. Part II. Oxidative-antioxidative imbalance. Int J Tuberc Lung Dis. 2008; 12: 368–74. [PubMed Abstract].
- Arja C, Surapaneni KM, Raya P, Adimoolam C, Balisetty B, Kanala KR. Oxidative stress and antioxidant enzyme activity in South Indian male smokers with chronic obstructive pulmonary disease. Respirology. 2013; 18: 1069–75. [PubMed Abstract].
- Cristovao C, Cristovao L, Nogueira F, Bicho M. Evaluation of the oxidant and antioxidant balance in the pathogenesis of chronic obstructive pulmonary disease. Rev Port Pneumol. 2013; 19: 70–5. [PubMed Abstract].
- Nadeem A, Raj HG, Chhabra SK. Increased oxidative stress and altered levels of antioxidants in chronic obstructive pulmonary disease. Inflammation. 2005; 29: 23–32. [PubMed Abstract].
- Andersson A, Ankerst J, Lindgren A, Larsson K, Hultberg B. Hyperhomocysteinemia and changed plasma thiol redox status in chronic obstructive pulmonary disease. Clin Chem Lab Med. 2001; 39: 229–33. [PubMed Abstract].
- Filaire E, Dupuis C, Galvaing G, Aubreton S, Laurent H, Richard R, etal. Lung cancer: what are the links with oxidative stress, physical activity and nutrition. Lung Cancer. 2013; 82: 383–9. [PubMed Abstract].
- Toledo AC, Magalhaes RM, Hizume DC, Vieira RP, Biselli PJ, Moriya HT, etal. Aerobic exercise attenuates pulmonary injury induced by exposure to cigarette smoke. Eur Respir J. 2012; 39: 254–64. [PubMed Abstract].
- Dutta A, Ray MR, Banerjee A. Systemic inflammatory changes and increased oxidative stress in rural Indian women cooking with biomass fuels. Toxicol Appl Pharmacol. 2012; 261: 255–62. [PubMed Abstract].
- Okubo H, Shaheen SO, Ntani G, Jameson KA, Syddall HE, Aihie Sayer A, etal. Processed meat consumption and lung function: modification by antioxidants and smoking. Eur Respir J. 2014; 43: 972–82. [PubMed Abstract].
- Suresh K, Chandrashekara S. Sample size estimation and power analysis for clinical research studies. J Hum Reprod Sci. 2012; 5: 7–13. [PubMed Abstract] [PubMed CentralFull Text].
- Miller MR, Crapo R, Hankinson J, Brusasco V, Burgos F, Casaburi R, etal. General considerations for lung function testing. Eur Respir J. 2005; 26: 153–61. [PubMed Abstract].
- Rouatbi S, Chouchene M, Sfaxi I, Ben Rejeb M, Tabka Z, Ben Saad H. Fraction of exhaled nitric oxide (FeNO) norms in healthy tunisian adults. BioMed Res Int. 2014. doi: http://dx.doi.org/10.1155/2014/269670. Available from: www.hindawi.com/journals/bmri/aip/269670 [cited 14 May 2014]..
- Ferris BG. Epidemiology standardization project (American Thoracic Society). Am Rev Respir Dis. 1978; 118: 1–120. [PubMed Abstract].
- Voorrips LE, Ravelli AC, Dongelmans PC, Deurenberg P, Van Staveren WA. A physical activity questionnaire for the elderly. Med Sci Sports Exerc. 1991; 23: 974–9. [PubMed Abstract].
- Ben Saad H, Tfifha M, Harrabi I, Tabka Z, Guenard H, Hayot M, etal. Factors influencing pulmonary function in Tunisian women aged 45 years and more. Rev Mal Respir. 2006; 23: 324–3800. [PubMed Abstract].
- Tsai AG, Wadden TA. In the clinic: obesity. Ann Intern Med. 2013; 159 ITC3-1–ITC3-15.
- Yagi K. A simple fluorometric assay for lipoperoxide in blood plasma. Biochem Med. 1976; 15: 212–16. [PubMed Abstract].
- Sedlak J, Lindsay RH. Estimation of total, protein-bound, and nonprotein sulfhydryl groups in tissue with Ellman's reagent. Anal Biochem. 1968; 25: 192–205. [PubMed Abstract].
- Waggiallah H, Alzohairy M. The effect of oxidative stress on human red cells glutathione peroxidase, glutathione reductase level, and prevalence of anemia among diabetics. N Am J Med Sci. 2011; 3: 344–7. [PubMed Abstract] [PubMed CentralFull Text].
- American Thoracic Society/European Respiratory Society. ATS/ERS recommendations for standardized procedures for the online and offline measurement of exhaled lower respiratory nitric oxide and nasal nitric oxide. Am J Respir Crit Care Med. 2005; 171: 912–30.
- Miller MR, Hankinson J, Brusasco V, Burgos F, Casaburi R, Coates A, etal. Standardisation of spirometry. Eur Respir J. 2005; 26: 319–38. [PubMed Abstract].
- Wanger J, Clausen JL, Coates A, Pedersen OF, Brusasco V, Burgos F, etal. Standardisation of the measurement of lung volumes. Eur Respir J. 2005; 26: 511–22. [PubMed Abstract].
- Ben Saad H, Prefaut C, Tabka Z, Zbidi A, Hayot M. The forgotten message from gold: FVC is a primary clinical outcome measure of bronchodilator reversibility in COPD. Pulm Pharmacol Ther. 2008; 21: 767–73. [PubMed Abstract].
- Ben Saad H, El Attar MN, Hadj Mabrouk K, Ben Abdelaziz A, Abdelghani A, Bousarssar M, etal. The recent multi-ethnic global lung initiative 2012 (GLI2012) reference values don't reflect contemporary adult's North African spirometry. Respir Med. 2013; 107: 2000–8. [PubMed Abstract].
- Ben Saad H, Ben Amor L, Ben Mdalla S, Ghannouchi I, Ben Essghair M, Sfaxi R, etal. The importance of lung volumes in the investigation of heavy smokers. Rev Mal Respir. 2014; 31: 29–40. [PubMed Abstract].
- Ben Saad H, Khemiss M, Nhari S, Ben Essghaier M, Rouatbi S. Pulmonary functions of narghile smokers compared to cigarette smokers: a case-control study. Libyan J Med. 2013; 8: 22650. [PubMed Abstract].
- Hanta I, Kocabas A, Canacankatan N, Kuleci S, Seydaoglu G. Oxidant-antioxidant balance in patients with COPD. Lung. 2006; 184: 51–5. [PubMed Abstract].
- Ben Saad H, Ben Attia Saafi R, Rouatbi S, Ben Mdella S, Garrouche A, etal. Which definition to use when defining airflow obstruction?. Rev Mal Respir. 2007; 24: 323–30. [PubMed Abstract].
- Sutherland TJ, Cowan JO, Young S, Goulding A, Grant AM, Williamson A, etal. The association between obesity and asthma: interactions between systemic and airway inflammation. Am J Respir Crit Care Med. 2008; 178: 469–75. [PubMed Abstract].
- Ghannem H, Hadj Fredj A. Eating habits and cardiovascular risk factors. Epidemiologic study of the Tunisian Sahel. Presse Med. 1999; 28: 1005–8. [PubMed Abstract].
- Franssen FM, O'Donnell DE, Goossens GH, Blaak EE, Schols AM. Obesity and the lung: 5. Obesity and COPD. Thorax. 2008; 63: 1110–17. [PubMed Abstract].
- Rahman I. The role of oxidative stress in the pathogenesis of COPD: implications for therapy. Treat Respir Med. 2005; 4: 175–200. [PubMed Abstract].
- Clini E, Bianchi L, Pagani M, Ambrosino N. Endogenous nitric oxide in patients with stable COPD: correlates with severity of disease. Thorax. 1998; 53: 881–3. [PubMed Abstract] [PubMed CentralFull Text].
- Ansarin K, Chatkin JM, Ferreira IM, Gutierrez CA, Zamel N, Chapman KR. Exhaled nitric oxide in chronic obstructive pulmonary disease: relationship to pulmonary function. Eur Respir J. 2001; 17: 934–8. [PubMed Abstract].
- Ziora D, Dworniczak S, Kaczmarczyk G, Jastrzebski D, Krzywiecki A, Kozielski J. Correlation of exhaled nitric oxide with nitrogen oxides and selected cytokines in induced sputum of chronic obstructive pulmonary disease patients. J Physiol Pharmacol. 2007; 58(Suppl 5): 791–9. [PubMed Abstract].
- Rutgers SR, Postma DS, ten Hacken NH, Kauffman HF, van Der Mark TW, Koeter GH, etal. Ongoing airway inflammation in patients with COPD who do not currently smoke. Thorax. 2000; 55: 12–18. [PubMed Abstract] [PubMed CentralFull Text].
- Kanazawa H, Shoji S, Yoshikawa T, Hirata K, Yoshikawa J. Increased production of endogenous nitric oxide in patients with bronchial asthma and chronic obstructive pulmonary disease. Clin Exp Allergy. 1998; 28: 1244–50. [PubMed Abstract].
- Delen FM, Sippel JM, Osborne ML, Law S, Thukkani N, Holden WE. Increased exhaled nitric oxide in chronic bronchitis: comparison with asthma and COPD. Chest. 2000; 117: 695–701. [PubMed Abstract].
- Maziak W, Loukides S, Culpitt S, Sullivan P, Kharitonov SA, Barnes PJ. Exhaled nitric oxide in chronic obstructive pulmonary disease. Am J Respir Crit Care Med. 1998; 157: 998–1002. [PubMed Abstract].
- Olin AC, Andelid K, Vikgren J, Rosengren A, Larsson S, Bake B, etal. Single breath N2-test and exhaled nitric oxide in men. Respir Med. 2006; 100: 1013–19. [PubMed Abstract].
- Dweik RA, Boggs PB, Erzurum SC, Irvin CG, Leigh MW, Lundberg JO, etal. An official ATS clinical practice guideline: interpretation of exhaled nitric oxide levels (FENO) for clinical applications. Am J Respir Crit Care Med. 2011; 184: 602–15. [PubMed Abstract].
- Morrison D, Rahman I, Lannan S, MacNee W. Epithelial permeability, inflammation, and oxidant stress in the air spaces of smokers. Am J Respir Crit Care Med. 1999; 159: 473–9. [PubMed Abstract].
- Bloomer RJ. Decreased blood antioxidant capacity and increased lipid peroxidation in young cigarette smokers compared to nonsmokers: impact of dietary intake. Nutr J. 2007; 6: 39. [PubMed Abstract] [PubMed CentralFull Text].
- Reddy S, Finkelstein EI, Wong PS, Phung A, Cross CE, van der Vliet A. Identification of glutathione modifications by cigarette smoke. Free Radic Biol Med. 2002; 33: 1490–8. [PubMed Abstract].
- Vina J, Servera E, Asensi M, Sastre J, Pallardo FV, Ferrero JA, etal. Exercise causes blood glutathione oxidation in chronic obstructive pulmonary disease: prevention by O2 therapy. J Appl Physiol (1985). 1996; 81: 2198–202.
- Calikoglu M, Unlu A, Tamer L, Ercan B, Bugdayci R, Atik U. The levels of serum vitamin C, malonyldialdehyde and erythrocyte reduced glutathione in chronic obstructive pulmonary disease and in healthy smokers. Clin Chem Lab Med. 2002; 40: 1028–31. [PubMed Abstract].
- Gan WQ, Man SF, Senthilselvan A, Sin DD. Association between chronic obstructive pulmonary disease and systemic inflammation: a systematic review and a meta-analysis. Thorax. 2004; 59: 574–80. [PubMed Abstract] [PubMed CentralFull Text].
- Gould NS, Min E, Gauthier S, Martin RJ, Day BJ. Lung glutathione adaptive responses to cigarette smoke exposure. Respir Res. 2011; 12: 133. [PubMed Abstract] [PubMed CentralFull Text].
- Jackson AA, Gibson NR, Lu Y, Jahoor F. Synthesis of erythrocyte glutathione in healthy adults consuming the safe amount of dietary protein. Am J Clin Nutr. 2004; 80: 101–7. [PubMed Abstract].
- Drost EM, Skwarski KM, Sauleda J, Soler N, Roca J, Agusti A, etal. Oxidative stress and airway inflammation in severe exacerbations of COPD. Thorax. 2005; 60: 293–300. [PubMed Abstract] [PubMed CentralFull Text].
- Rahman I, Morrison D, Donaldson K, MacNee W. Systemic oxidative stress in asthma, COPD, and smokers. Am J Respir Crit Care Med. 1996; 154: 1055–60. [PubMed Abstract].
- Woodruff PG, Koth LL, Yang YH, Rodriguez MW, Favoreto S, Dolganov GM, etal. A distinctive alveolar macrophage activation state induced by cigarette smoking. Am J Respir Crit Care Med. 2005; 172: 1383–92. [PubMed Abstract] [PubMed CentralFull Text].
- Droge W. Free radicals in the physiological control of cell function. Physiol Rev. 2002; 82: 47–95. [PubMed Abstract].
- Valko M, Rhodes CJ, Moncol J, Izakovic M, Mazur M. Free radicals, metals and antioxidants in oxidative stress-induced cancer. Chem Biol Interact. 2006; 160: 1–40. [PubMed Abstract].
- Ahmad A, Shameem M, Husain Q. Altered oxidant-antioxidant levels in the disease prognosis of chronic obstructive pulmonary disease. Int J Tuberc Lung Dis. 2013; 17: 1104–9. [PubMed Abstract].
- Reznick AZ, Packer L. Oxidative damage to proteins: spectrophotometric method for carbonyl assay. Methods Enzymol. 1994; 233: 357–63. [PubMed Abstract].
- Dalle-Donne I, Rossi R, Giustarini D, Milzani A, Colombo R. Protein carbonyl groups as biomarkers of oxidative stress. Clin Chim Acta. 2003; 329: 23–38. [PubMed Abstract].
- Nagler R, Lischinsky S, Diamond E, Drigues N, Klein I, Reznick AZ. Effect of cigarette smoke on salivary proteins and enzyme activities. Arch Biochem Biophys. 2000; 379: 229–36. [PubMed Abstract].
- Dweik RA, Sorkness RL, Wenzel S, Hammel J, Curran-Everett D, Comhair SA, etal. Use of exhaled nitric oxide measurement to identify a reactive, at-risk phenotype among patients with asthma. Am J Respir Crit Care Med. 2010; 181: 1033–41. [PubMed Abstract] [PubMed CentralFull Text].
- Robbins RA, Millatmal T, Lassi K, Rennard S, Daughton D. Smoking cessation is associated with an increase in exhaled nitric oxide. Chest. 1997; 112: 313–18. [PubMed Abstract].
- Montuschi P, Barnes PJ, Roberts LJ. Isoprostanes: markers and mediators of oxidative stress. FASEB J. 2004; 18: 1791–800. [PubMed Abstract].
- Rolin S, Masereel B, Dogne JM. Prostanoids as pharmacological targets in COPD and asthma. Eur J Pharmacol. 2006; 533: 89–100. [PubMed Abstract].
- Garcia-Rio F, Romero D, Lores V, Casitas R, Hernanz A, Galera R, etal. Dynamic hyperinflation, arterial blood oxygen, and airway oxidative stress in stable patients with COPD. Chest. 2011; 140: 961–9. [PubMed Abstract].
- Ben Saad H, Prefaut C, Tabka Z, Mtir AH, Chemit M, Hassaoune R, etal. 6-minute walk distance in healthy North Africans older than 40 years: influence of parity. Respir Med. 2009; 103: 74–84. [PubMed Abstract].