Abstract
Information on the diversity of marine fungi in polar environments is lacking, especially marine fungi colonizing wood. During visits to Tromsø and Longyearbyen, Norway, drift and trapped wood was collected to provide a preliminary account of lignicolous marine fungi in Arctic waters. Six marine fungi were recorded from 24 and 27 samples of wood from Tromsø and Longyearbyen, respectively. Among these, four marine fungi new to science were identified from wood collected at Longyearbyen. To shed light on the ecological role of this group of fungi in the Arctic, a physiological study of one of the collected fungi, Havispora longyearbyenensis, was conducted. H. longyearbyenensis grew at 4 °C, 10 °C, 15 °C and 20 °C in all salinities tested (0 0/00, 17 0/00, 34 0/00). However, growth was significantly reduced at 4 °C and 0 0/00 salinity. The optimal condition for growth of H. longyearbyenensis was at 20 °C in all salinities tested.
Marine fungi are an ecological group of organisms, represented by primarily filamentous ascomycetes, their anamorphs and yeasts (Pang & Mitchell Citation2005). Obligate marine fungi grow and reproduce on a range of lignocellulosic materials including wood, algae and marine grasses, thereby contributing to nutrient recycling in the ecosystems (Hyde et al. Citation1998). Marine fungi generally have appendages for dispersal (Hyde & Jones Citation1989) and produce wood-modifying enzymes (cellulases, laccases and Mn-dependent peroxidases) that degrade wood, producing a soft-rot type of decay (Bucher et al. Citation2004, Luo et al. Citation2005).
A significant volume of published work on the worldwide occurrence of marine fungi is available. Jones (Citation1993) categorized these fungi in three groups: tropical/subtropical, temperate/Arctic and cosmopolitan. Studies of marine fungi in polar environments are scant, except a few brief reports (Tubaki & Asano Citation1965, Pugh & Allsopp Citation1982, Kohlmeyer Citation1973, Pugh & Jones Citation1986). This article reports the diversity and species composition of marine fungi on drift and trapped wood collected at the shores of Tromsø and Longyearbyen, Norway.
Water temperature and salinity are important physical parameters affecting the growth of marine lignicolous fungi (Jones Citation2000), and sea temperature plays a significant role in determining geographical distribution of marine fungi (Jones Citation2000). The mycelial growth of a new marine fungus, Havispora longyearbyenensis, found in the wood collected for this study was examined various temperatures and salinities to elucidate the possible role of this group of fungi in this environment.
Materials and methods
Species composition and diversity study
Washed-up and trapped wood was collected at Tromsø (69°40'N, 18°56'E), mainland Norway, and Longyearbyen (78°13'N, 15°33'E), Spitsbergen, Svalbard, Norway, on 10 August 2006 and 12 August 2006, respectively. Wood samples were placed in plastic bags and transported to the laboratory in Hong Kong. Wood pieces were incubated in plastic boxes lined with moist tissue paper and observed for sporulating structures for a 3-month period. Fungi occurring on the wood surface or beneath the wood were picked up fine forceps, mounted in sterile seawater on a glass slide and observed on an Axioplan 2 imaging microscope (Carl Zeiss, Jena, Germany) and light micrographs were acquired by a ColorView 12 CCD camera (Olympus Soft Imaging System, Munster, Germany) using analy SIS v.3.2. Mycelial cultures were obtained from single spores on cornmeal seawater agar (CMAS, Oxoid, Basingstoke, Hampshire, UK) on full-strength seawater, supplemented with 0.5 g/L−1 penicillin G and 0.5 g/L−1 streptomycin sulfate, and kept at the City University Culture Collection.
Physiology study
Havispora longyearbyenensis CY5278 was subcultured on CMAS plates for 8 weeks and incubated at 15 °C as inoculum. Agar plugs (6mm in diameter) were cut from the growing edge of the fungal colonies on the CMAS plates using the wide end of a sterilized glass Pasteur pipette. Agar plugs were inoculated aseptically on the intersection point of two lines drawn on the bottom of CMAS plates. The fungus was inoculated on CMAS plates with three salinities (0 0/00, 17 0/00, 34 0/00). For each salinity, triplicate plates were incubated at 4°C, 10°C, 15°C, 20°C, 25°C, 30°C and 35°C for 125 days. Results were expressed as the colony diameter (in mm) of the fungus by calculating the mean colony diameter along the two perpendicular lines. Data were analysed using two-way ANOVA (Tukey’s test) using SIGMASTAT v3.0.
Results
Species diversity and composition
Twenty-four and 27 pieces of wood were collected at Tromsø and Longyearbyen, respectively. Six marine fungi were recorded at each location ().
Table 1. Species of lignicolous marine fungi found in wood samples collected on the shores of Tromsø and Longyearbyen, Norway.
Four of the marine taxa found in the samples from Longyearbyen were new to science (). These were: two ascomycetes, H. longyearbyenensis and Remispora spitsbergenensis, which were recently formally described (Pang et al. Citation2008 Citation2009); a new anamorphic taxon that is morphologically similar to Halenospora varia, but differs in having a hyaline conidial end cell; and a Lulworthia species new to science. The presence of only a few fruiting structures on the wood prevented proper circumscription of the Lulworthia species.
Fig. 1. Ascospores of marine lignicolous fungi identified from wood collected at Tromsø and Longyearbyen. (a) Amylocarpus encephaloides, (b) Ceriosporopsis tubulifera, (c) Lautosporopsis circumvestita, (d) Sablecola chinensis, (e) Havispora longyearbyenensis, (f) Remispora spitsbergenensis, (g) a new dermataceous hyphomycete, (h) a new Lulworthia species. Scale bars = 10 µm.
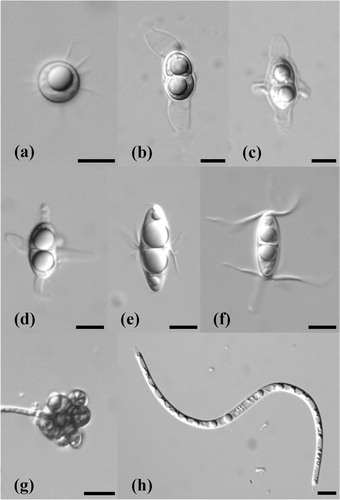
Physiology study
Havispora longyearbyenensis could grow between 4 °C and 20 °C in all salinities tested (0–34 0/00). The optimal for growth was at 20 °C in all salinities tested (). Overall, full-strength seawater (34 0/00) and half-strength seawater (17 0/00) supported better growth of H. longyearbyenensis. No significant difference was observed on growth at salinities of 17 0/00 and 34 0/00. At 4 °C, mycelial growth was slow and salinity did not affect growth significantly. No growth was observed at 25 °C and 30 °C for all salinities. Significantly better growth was recorded at 10 °C, 15 °C and 20 °C, compared to growth at 4 °C. No Phoma-pattern (an increased salinity optimum with increasing incubation temperature) was shown by the fungus (Lorenz & Molitoris Citation1992).
Discussion
The species composition of marine fungi collected at Tromsø () is similar to those reported at temperate locations and also Grytviken, South Georgia, in the Antarctic (Pugh & Jones Citation1986). Amylocarpus encephaloides (a), Ceriosporopsis tubulifera (b) and Lautosporopsis circumvestita (c) are common taxa, frequently reported in Denmark and Friday Harbour, San Juan County, WA, USA (Koch & Petersen Citation1996; Jones et al. Citation1998). Surprisingly, Sablecola chinensis (d), which has previously only been reported in southern China and Singapore, was isolated (Pang et al. Citation2004).
The species composition of lignicolous marine fungi from Longyearbyen was different from that from Tromsø. Only one species was identified in the samples from both Longyearbyen and Tromsø: Lulworthia sp. 1. Abundant driftwood, originating from Siberian and North American boreal forests, washes up at Spitsbergen (Alix Citation2005), and is evidently a suitable substratum for supporting growth of marine fungi. Four new marine fungi were discovered from only 27 wood samples collected over a 2-hour period in Longyearbyen, suggesting that more effort is required to study the diversity of marine lignicolous fungi in Longyearbyen and other parts of the Arctic. For future studies it should be noted that fungi may be present as fungal hyphae inside wood, making detection by the traditional method of identifying fruiting structures with a microscopic difficult (Pang & Mitchell Citation2005).
A number of factors—biological, chemical and physical—can affect the diversity and species composition of marine fungi, including the availability of substrata for colonization, the nature of the substratum, interference competition, water temperature and salinity. Temperature plays a major role in governing the geographical distribution of marine fungi (Jones Citation2000). In Arctic ecosystems, extreme conditions can be encountered in the form of low temperature (<10 °C during summer months) and varying salinity partly as a result of summer ice melt (Loeng Citation1991) and marine fungi must adapt to local physical and chemical conditions through changes in physiology (Raghukumar Citation2008).
The ability of H. longyearbyenensis to grow at a range of temperatures (4–20 °C) and salinities (0–34 0/00) indicates that it may be an indigenous marine lignicolous fungus in the Arctic. H. longyearbyenensis can be regarded as a psychrotolerant fungus as its growth in our experiment occurred between 4°C and 20 °C and no growth was observed at 25 °C respectively. Whether this fungus can grow at temperatures below 4 °C is a question for future research. Many other fungi have also been shown to be viable at low temperatures: soil fungi in tundra (Schadt et al. Citation2003) and cryptoendolithic fungi occurring inside the porosity of rocks (Onofri et al. Citation2004).
Mouzouras (Citation1986) studied the weight loss of Ochroma lagopus (balsa) by a cold-water marine fungus, Digitatispora marina. After 8 and 24 weeks incubation at 10 °C, 8.06% and 14.33% weight loss were noted, respectively, with only 1.06% and 4.94% weight loss at 22 °C. However, the opposite effect was noted for two warm-water species, Nia vibrissa and Halocyphina villosa, i.e., O. lagopus underwent a higher weight loss at 22 °C than at 10 °C. In a study of the effect of temperature on the growth of soil fungi isolated from Windmill Islands, continental Antarctica, the optimum temperature for all fungi was also 20 °C. However, these fungi were able to grow at 25 °C and 30 °C (Azmi & Seppelt Citation1997). In contrast, Kochkina et al. (Citation2007) reported that fungal species isolated from cryopegs and marine deposits at the Kolyma lowland, on the shores of the East Siberian Sea, exhibited better growth at 4 °C than at 26 °C.
Booth & Kenkel (Citation1986) divided marine fungi into six ecological groups based on temperature and salinity, and the Arctic group was regarded as cool-water homeothermic euryhaline. However, H. longyearbyenensis not only has a wide temperature growth range but also a wide salinity growth range, which is characteristics of the euryhalothermic groups.
Marine fungi produce wood-degrading enzymes that mineralize wood for nutrition (Luo et al. Citation2005). The growth of H. longyearbyenensis at low temperatures (4–20 °C) and at a range of salinities (0–34 0/00) in our experiment suggests that this species plays a role in the degradation of wood in the Arctic. Our study indicates that the ecological role of lignicolous marine fungi in the Arctic warrants further investigation.
Acknowledgements
We acknowledge the support of the Scholarship Enhancement Scheme grant no. 9368005 of the City University of Hong Kong. We thank: the Governor of Svalbard for allowing us to collect in Svalbard; Mr. Ian Gertz for the swift processing of our permit application; Dr. Steve Coulson (University Centre in Svalbard) for the provision of laboratory space and equipment; Prof. Gareth Jones for some essential references; Michael Chiang for help with statistics; and Eric Wong and Daniel Tsang for help during collection.
References
- Alix C. Deciphering the impact of change on the driftwood cycle: contribution to the study of human use of wood in the Arctic. Global and Planetary Change. 2005; 47: 83–98.
- Azmi O.R. Seppelt R.D. Fungi of the Windmill Islands, continental Antarctica. Effect of temperature, pH and culture media on the growth of selected microfungi. Polar Biology. 1997; 18: 128–134.
- Booth T. Kenkel N. Ecological studies of lignicolous marine fungi: a distribution model based on ordination and classification. The biology of marine fungi. Moss S.T. Cambridge University Press. Cambridge, 1986; 297–310.
- Bucher V.V.C. Hyde K.D. Pointing S.B. Reddy C.A. Production of wood decay enzymes, mass loss and lignin solubilization in wood by marine ascomycetes and their anamorphs. Fungal Diversity. 2004; 15: 1–14.
- Hyde K.D. Jones E.B.G. Observations on ascospore morphology in marine fungi and their attachment to surfaces. Botanica Marina. 1989; 32: 205–218.
- Hyde K.D. Jones E.B.G. Leano E. Pointing S.B. Poonyth A.D. Vrijmoed L.L.P. Role of fungi in marine ecosystems. Biodiversity and Conservation. 1998; 7: 1147–1161.
- Jones E.B.G. Tropical marine fungi. Aspects of tropical mycology. Isaac S. et al.. Cambridge University Press. Cambridge, 1993; 73–89.
- Jones E.B.G. Marine fungi: some factors influencing biodiversity. Fungal Diversity. 2000; 4: 53–73.
- Jones E.B.G. Vrijmoed L.L.P. Alias S.A. Intertidal marine fungi from San Juan Island and comments on temperate water species. Botanical Journal of Scotland. 1998; 50: 177–187.
- Koch J. Petersen K.R.L. A check list of higher marine fungi on wood from Danish coasts. Mycotaxon. 1996; 15: 397–414.
- Kochkina G.A. Ivanushkina N.E. Akimov V.N. Gilichinskii D.A. Ozerskaya S.M. Halo- and psychrotolerant Geomyces fungi from Arctic cryopegs and marine deposits. Microbiology. 2007; 76: 31–38.
- Kohlmeyer J. Spathulosporales, a new order and possible missing link between Laboulbeniales and Pyrenomycetes. Mycologia. 1973; 65: 614–647.
- Loeng H. Features of the physical oceanographic conditions of the Barents Sea. Polar Research. 1991; 10: 5–18.
- Lorenz R. Molitoris H.P. Combined influence of salinity and temperature (Phoma-pattern) on growth of marine fungi. Canadian Journal of Botany. 1992; 70: 2111–2115.
- Luo W. Vrijmoed L.L.P. Jones E.B.G. Screening of marine fungi for lignocellulose-degrading enzyme activities. Botanica Marina. 2005; 48: 379–386.
- Mouzouras R. Patterns of timber decay caused by marine fungi. The biology of marine fungi. Moss S.T. Cambridge University Press. Cambridge, 1986; 341–353.
- Onofri S. Selbmann L. Zucconi L. Pagano S. Antarctic microfungi as models for exobiology. Planetary and Space Science. 2004; 52: 229–237.
- Pang K.L. Mitchell J. I. Molecular approaches for assessing fungal diversity in marine substrata. Botanica Marina. 2005; 48: 332–347.
- Pang K.L. Chiang M.W.L. Vrijmoed L.L.P. Havispora longyearbyenensis gen. et sp. nov.: an Arctic marine fungus from Svalbard, Norway. Mycologia. 2008; 100: 291–295.
- Pang K.L. Chiang M.W.L. Vrijmoed L.L.P. Remispora spitsbergenensis sp. nov., a marine lignicolous ascomycete from Svalbard, Norway. Mycologia. 2009; 101: 531–534.
- Pang K.L. Jones E.B.G. Vrijmoed L.L.P. Two new marine fungi from China and Singapore, with the description of a new genus, Sablecola. Canadian Journal of Botany. 2004; 82: 485–490.
- Pugh G.J.F. Allsopp D. Microfungi in Signy Island, South Orkney Islands. British Antarctic Survey Bulletin. 1982; 57: 55–67.
- Pugh G.J.F. Jones E.B.G. Antarctic marine fungi: a preliminary account. The biology of marine fungi. Moss S.T. Cambridge University Press. Cambridge, 1986; 323–330.
- Raghukumar C. Marine fungal biotechnology: an ecological perspective. Fungal Diversity. 2008; 31: 19–35.
- Schadt C.W. Martin A.P. Lipson D.A. Schmidt SK. Seasonal dynamics of previously unknown fungal lineages in tundra soil. Science. 2003; 301: 1359–1361.
- Tubaki K. Asano I. Additional speices of fungi isolated from the Antarctic materials. JARE 1956–1962 Scientific Reports Series E. 1965; 27: 1–14.