Abstract
The pelagic copepods Calanus glacialis and C. finmarchicus are important components of Arctic marine ecosystems. Projected climate warming may influence the roles they play in the ecosystem. Arctic C. glacialis and boreal C. finmarchicus eggs were incubated at temperatures of 0, 2.5, 5, 7.5 and 10°C to investigate the effects of increasing temperature on egg development rate and hatching success. The effect of increasing temperature on median development time, described by B[ebreve]lehrádek's temperature function, was examined using a Bayesian approach. For the studied temperature range, we observed the increase of egg development rates with the increasing temperature, although there was no change in hatching success. Calanus finmarchicus eggs hatched significantly faster than C. glacialis above approximately 2°C; the difference was progressively larger at higher temperatures. This may indicate that the boreal species have physiological advantages in areas where ambient temperatures increase, which may lead to C. finmarchicus outcompeting the Arctic species in situations where timing is important, for example, in relation to spring bloom dynamics. Development time to hatching (DH) was evaluated using B[ebreve]lehrádek's model and a set of different assumptions. The models that best fitted our data were those with species-specific parameters: DH (h)=5940 (T+9.7)−1.63 for C. finmarchicus and DH (h)=14168 (T+14)−1.75 for C. glacialis.
Calanus species are among the key components of Arctic ecosystems on account of their high biomass and their role in food chains. They are predominant grazers and important prey items for other zooplankton species, fish and seabirds such as the little auk (Alle alle) (Karnovsky et al. Citation2003; Falk-Petersen et al. Citation2007). Calanus finmarchicus (Gunnerus 1770) is a boreal species that is widely distributed in the North Atlantic and European Arctic (Jaschnov Citation1970; Planque & Batten Citation2000; Weydmann et al. Citation2014). In the Barents and Norwegian seas, as well as in Disko Bay, it has a one-year life cycle, and spawns during or just after the spring phytoplankton bloom, which occurs in these areas between March and May (Niehoff et al. Citation2002; Madsen et al. Citation2008). Its sibling species Calanus glacialis (Jaschnov 1955), which is an Arctic species, spawns on shelves, along continental slopes and in fjords (Jaschnov Citation1970; Conover Citation1988; Weydmann et al. Citation2013). It uses its internal lipid reserves to develop gonads before the bloom, but requires energy from either ice-algae or phytoplankton blooms to maximize egg production rates (Hirche & Kattner Citation1993; Madsen et al. Citation2008; Søreide et al. Citation2010).
If the present trend of climate warming continues, current knowledge predicts this will have a substantial impact on the functioning of marine ecosystems in the Arctic (Wassmann Citation2011). Temperature affects every aspect of zooplankton life, from cellular processes to behaviour, development and fitness (Huntley & Lopez Citation1992; Clarke Citation2003), and warming beyond a species’ thermal tolerance could modify these aspects. For example, changes in development time can lead to a tipping point in the structure and functioning of Arctic marine ecosystems (Carstensen & Weydmann Citation2012). Increasing temperatures may especially influence the Arctic species C. glacialis, whose egg production rate was found to stabilize at 2.5°C (Kjellerup et al. Citation2012) and eventually decrease in temperatures above 5°C (Pasternak et al. Citation2013). At the same time, the presence and biomass of this species in the Nordic seas was observed to decrease with ambient temperatures above the critical threshold of ca. 6°C (Carstensen et al. Citation2012). Additionally, a positive balance between ingestion and respiration was found to cease above the same (5°C) temperature threshold (Alcaraz et al. 2013). None of the above effects was observed in parallel studies concerning the boreal C. finmarchicus at these temperatures (Carstensen et al. Citation2012; Pasternak et al. Citation2013).
The early development of Calanus species has been studied in different aspects, such as the influence of temperature (McLaren et al. Citation1969; Corkett et al. Citation1986); both temperature and food availability (Campbell et al. Citation2001; Cook et al. Citation2007) and pyrene exposure and temperature (Grenvald et al. Citation2013), although the authors did not focus on the problem of egg developmental time and hatching success under extreme temperatures. Development rates are routinely described by B[ebreve]lehrádek's (Citation1935) temperature function: DH=a (T – α) b , where DH (development time to hatching) is the egg development time to hatching, T is the temperature and a, b and α are fitted parameters defining the properties of species response curves.
The aim of this study was to investigate the effects of temperature on Arctic C. glacialis and boreal C. finmarchicus egg development rate and hatching success, in order to examine the implications of a warming Arctic scenario. Another goal was to examine the correctness of B[ebreve]lehrádek's temperature function parameters, known from earlier studies, when used for the experimentally obtained data set over a relatively large temperature range.
Materials and methods
Zooplankton collection and experiments
Zooplankton were collected from vertical tows with a Multi Plankton Sampler (Hydro-Bios; Kiel, Germany) or a WP-2 type net (Hydro-Bios) using a large volume cod end bucket (both nets with 0.180 mm mesh size) during the cruise of the RV Jan Mayen (currently RV Helmer Hanssen) to the north-west Barents and north-east Greenland seas in June 2009 (four sampling stations). In April–May 2010 we used a WP-2 net (mesh size 0.180 mm) in Hornsund, a fjord located at the south-western tip of Spitsbergen. In 2009, the mean water temperatures at the zooplankton sampling depths ranged from 1.28°C (station ATP 3; 77° 08.6′N 28° 11.0′E; 190 m) to 2.66°C (station ATP 6; 79° 56.0′N 14° 43.1′E; 123 m), whereas in 2010 in Hornsund it was 0.3°C. The mean chlorophyll a concentrations in the 0–100 m water layer in 2009, ranged from 0.89 (station ATP 7; 76° 29.7′N 28° 02.0′E; 142 m) to 1.17 mg m−3 at station ATP 6. In 2010 the samples were collected from 50 m to the surface at two stations, and chlorophyll a was not measured.
The experiments in 2009 and 2010 were performed aboard the RV Jan Mayen and at the Polish Polar Station in Hornsund, respectively, as a series of incubations at temperatures of 0°C, 2.5°C, 5°C, 7.5°C and 10°C. Females of Calanus glacialis and C. finmarchicus, sorted out from the zooplankton samples at the given stations, were transferred simultaneously to 250 ml beakers filled with the filtered seawater. The beakers were equipped with suspended inserts, which had mesh on the bottom, allowing the eggs to pass through, while keeping the females away from them and were reared in temperatures close to ambient (in 2009 T=2.5°C, in 2010 T=0°C) for egg shedding. In 2009 we collected eggs from 69 females of C. finmarchicus and 196 of C. glacialis, while in 2010 it was 19 and 32, respectively. Usually five females were placed in one beaker; they were not fed and kept until eggs were produced. The healthy-looking eggs were collected from the rearing beakers with females every 12 h, transported gently with a pipette to separate Petri dishes and incubated in laboratory coolers (type CHL 1B) at the experimental temperatures. To reduce the effects of females or in situ environmental conditions on the results, the eggs collected from groups of females from the same station were mixed and split into separate dishes (25 eggs per dish for C. finmarchicus and 30 eggs per dish for C. glacialis), which were set at all experimental temperatures. In total, we used 54 Petri dishes of 60 ml volume for C. finmarchicus (14 in 2009 and 40 in 2010) and 104 for C. glacialis (31 in 2009 and 73 in 2010); they were equally distributed among temperatures and treated as replicates in the following statistical analyses. The time “0” in our experiment is understood as the beginning of the egg incubation period, not as the exact time of egg release by females. The state of the reared eggs and the number of nauplii and unhatched eggs were checked daily, if possible, every 12 h. Incubations were continued until all the eggs hatched or showed signs of decay, which usually took around a week (168 h).
Statistical analysis
To properly evaluate all sources of uncertainty when applying B[ebreve]lehrádek's temperature function to the experimentally obtained data set, we decided to employ Bayesian statistics (Brooks Citation2003; Clark Citation2005). As a programming tool, we chose WinBUGS software, which is commonly used in ecological studies (Lunn et al. Citation2000; Kery Citation2010; Lunn et al. Citation2012).
To assess hatching success in the experimental temperatures, a Bayesian linear model with three main factors—year (Y), species (S) and temperature (T)—and their interactions—year–species interaction (YS), temperature–species interaction (TS), temperature–year interaction (TY)—was performed. The dependent variable was defined as logit(P), P=(K+1)/(N+2), where P is the mean value of hatching success probability, K is the number of hatched nauplii, and N is the total egg number. In total 10 000 samples of the joint posterior distribution from the Reversible Jump Markov Chain Monte Carlo (RJ MCMC) simulation in three independent chains was generated in WinBUGS software (Lunn et al. Citation2006; Lunn et al. Citation2008). In addition, a Brooks–Gelman–Rubin (B–G–R) test of chain convergence was successfully performed (Brooks & Gelman Citation1998).
Differences in egg development times between species and experimental temperatures were calculated for median hatching time, which was defined as the time at which half of the eggs had hatched. All 141 time-to-hatching log-median data were divided into 10 species-temperature cells (two species, five experimental temperatures), and the Jarque–Bera test of normality (Jarque & Bera Citation1987) was performed for each cell. In six cells, the p-value of the test was below or near 0.05, and six clear outliers were removed. Repeated Jarque–Bera tests for the remaining 135 data values resulted in p-values of approximately 0.1 and above.
For each model configuration, a number of models NM=2NP were tested in WinBUGS. NP represents the number of free (estimated) parameters in a particular configuration. For each parameter P, two cases were tested: models with common values of this parameter for both species (symbol P) and models with different values for each species C. finmarchicus and C. glacialis (symbol PP). For example, there are 2NP=4 models for NP=2 estimated parameters a and b, with symbols: aa–bb, a–bb, aa–b and a–b.
To compare different models and select the most plausible model, the Deviance Information Criterion, DIC(m), was estimated using WinBUGS (Spiegelhalter et al. Citation2002) for each model m of NM number of models, following the calculation of “penalized” likelihood L(m)=exp{−0.5·DIC(m)}. Posterior probability of model m equals:
Evidence in favour of model m against all other NM–1 models was calculated as follows (Jeffreys Citation1961):
Jeffreys’ qualitative scale for evidence (where evidence ≤0: bad model quality, >0 and <1: not substantial, ≥1 and <2: substantial, ≥2 and <3: strong, ≥3 and <4: very strong, ≥4: decisive evidence) was also adopted (see Lunn et al. Citation2012, chapter 8). The decisive level of evidence of a certain model indicates that all remaining models can be rejected from further analysis.
To compare the results between species with other published data B[ebreve]lehrádek's (Citation1935) temperature function DH=a (T – α)
b
was used, where DH is median time to hatching in hours, T is temperature in Celsius degrees, and a, b and α are fitted parameters. The parameters in B[ebreve]lehrádek's function define properties of species response curves to temperature: a describes differences in slope, b is associated with differences in monotonic curvelinearity within natural temperature ranges and α is a species’ “biological zero” temperature (McLaren et al. 1969). A nonlinear regression was performed using a log transformed expression: ; parameter a was “recovered” by exponentiation.
The fit of B[ebreve]lehrádek's temperature function to the experimental data was performed in three configurations: (i) with forced b=−2.05 as suggested by McLaren et al. (1969), which resulted in four models: a-(−2.05)-α, aa-(−2.05)-α, a-(−2.05)-αα, and aa-(−2.05)-αα; (ii) with a common parameter b estimated from data in four models: a–b–α, aa–b–α, a–b–αα, and aa–b–αα; (iii) with all parameters free, to determine the function that best fitted our data in eight models: a–b–α, aa–b–α, a–b–αα, aa–b–αα, a–bb–α, aa–bb–α, a–bb–αα, and aa–bb–αα. In each configuration, the most probable model was selected for further evaluation by the DIC described above. The ratio of hatching times between species as a function of temperature was also estimated for the model results that gave the best model fit.
Due to a very strong correlation among the estimated B[ebreve]lehrádek's model parameters, which resulted in substantial autocorrelations in most of the MCMC chains, in each of the total 12 simulations, the following calculation regime was applied: 20 000 initial iterations followed by one million main iterations recorded every 100 iterations (the “thin” value was 100), in three independent chains. The B–G–R test of convergence was always performed.
Results
The total number of 1261 eggs of Calanus finmarchicus (501 in 2009 and 760 in 2010) and 3323 eggs of C. glacialis (1477 in 2009 and 1846 in 2010) were selected from the mixed egg pool and used for the following incubation experiments (). Hatching occurred at all experimental temperatures with a considerably high success (). The proportion of hatched nauplii for C. finmarchicus ranged from 74.5% at 5°C in 2010 to 95.4% in the highest temperature in 2009, whereas in C. glacialis it ranged from 71.3% at 10°C in 2009 to 92.6% at 2.5°C in 2010 (). According to the Bayesian comparison of the linear models with three main factors and the interactions between them, which was performed using RJ MCMC, the most probable, at almost a decisive level of evidence (evidence=3.9, ), was the null model with no parameters. According to this analysis, the hatching success was the same at all temperatures, and for both years and species (see ).
Fig. 1 Relationship between the mean hatching success (%) and time (hours) in C. finmarchicus and C. glacialis in 2009 and 2010.
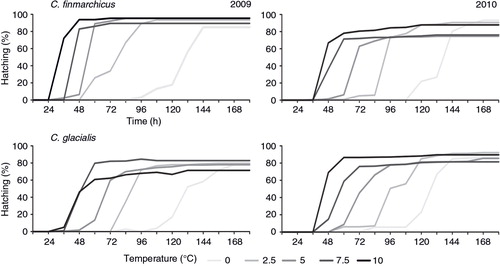
Table 1 Number of eggs and hatching success of Calanus finmarchicus and C. glacialis in the experimental temperatures.
Table 2 Bayesian linear model of hatching success, with three main factors and interactions performed by Reversible Jump Markov Chain Monte Carlo. All remaining models had a zero posterior probability.
In the first configuration of the tested B[ebreve]lehrádek's models, with forced b=−2.05, DH (S)=a(S) [T – α(S)]−2.05, a reasonable fit was achieved only by the model type aa-(−2.05)-αα (). According to this model, the experimental results were fitted to the following hatching time functions: DH (h)=28420 (T+13)−2.05 for C. finmarchicus and DH (h)=45838 (T+16.9)−2.05 for C. glacialis. Similarly, in the second configuration DH (S)=a(S) [T – α(S)] b with the value of a common parameter b estimated as b=−1.73 based on the data from our experiments, only the model type aa–b–αα was qualified as decisive (). In this case, the developmental functions were DH (h)=8629 (T+10.5)−1.73 for C. finmarchicus and DH (h)=12977 (T+13.7)−1.73 for C. glacialis. Model aa–b–αα was much more probable than model aa-(−2.05)-αα, with the decisive evidence criterion value above 4.5 (difference in DIC is 10.5 in favour of the model with common parameter b; see ).
Table 3 The comparison of B[ebreve]lehrádek's models: with a common parameter b (forced b=−2.05 and estimated b=−1.73), with evidence >0 according to Jeffreys’ qualitative scale for evidence; and with different configurations of fitted parameters a, b and α. The best model is in boldface.
The comparison of B[ebreve]lehrádek's models with different fitted parameters a, b and α resulted in the highest satisfactory evidence () only for the model with all free parameters aa–bb–αα: DH (S)=a(S) [T – α(S)] b(S). According to this model, the experimental results were best fitted to the following hatching time functions: DH (h)=5940 (T+9.7)−1.63 for C. finmarchicus and DH (h)=14168 (T+14)−1.75 for C. glacialis (). The ratio of hatching time between species based on the model aa−bb–αα () showed the higher rates for the Arctic species in the lowest experimental temperatures, i.e., from 0 to ca. 1°C. With the increasing temperature, C. finmarchicus eggs hatched faster; taking the±95% Bayesian credibility interval into account, the difference between species was evident above 2.6°C. These results are supported by the evidence of differences in hatching times between species calculated for temperature intervals (), which confirmed that for temperatures between 0 and 0.7°C C. glacialis egg hatching time was shorter, whereas C. finmarchicus eggs hatched substantially faster in temperatures above 2.0°C; however, the evidence was decisive only above 3.6°C.
Fig. 2 Relationship between median hatching time and temperature based on the best model aa–bb–αα, with ±95% Bayesian credibility interval shown by dotted lines, and the comparison of the obtained hatching rates with other studies. Whiskers indicate ranges between replicates.
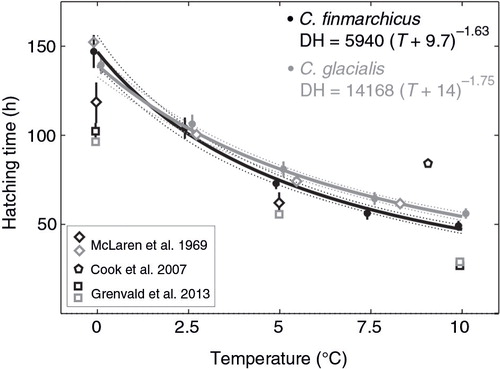
Fig. 3 Ratio of hatching time (HT) for C. glacialis (Cg) to HT for C. finmarchicus (Cf) based on the best model aa–bb–αα, with ±95% Bayesian credibility interval.
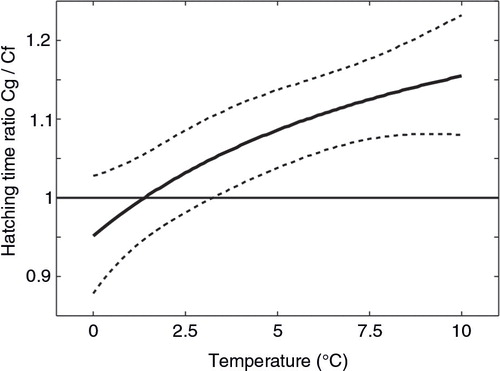
Table 4 Evidence of differences in hatching times between species—Calanus finmarchicus (Cf) and C. glacialis (Cg)—at the increasing temperatures based on the best model aa–bb–αα.
Discussion
The egg development times for both of the sibling copepod species Calanus finmarchicus and C. glacialis, which co-occur in the Arctic, was shorter with increasing temperature but did not lead to decreased hatching success, even at the maximum experimental temperature. Hatching was faster in higher temperatures, similar to the results of earlier studies. The hatching rates in C. glacialis obtained by McLaren et al. (Citation1969) were mostly within the ±95% Bayesian credibility interval of this study (); hence, they do not differ substantially. However, Grenvald et al. (Citation2013), in the control of their pyrene exposure experiments, measured earlier median hatching times for both species. In the case of C. finmarchicus (Marschall & Orr 1953 cited in McLaren et al. Citation1969), our observations of median hatching time resulted in slightly higher but comparable values, and this discrepancy might be due to small differences in experimental temperatures because the time “0” in our experiment was understood as the beginning of the egg incubation period, not as the exact time of egg shedding by females. Other factors that might have influenced our results were: the temperature in which females were maintained to shed eggs (2.5°C in 2009), which could accelerate egg development especially at lower temperatures, and the lower number of C. finmarchicus females and, consequently, eggs, than of C. glacialis.
Interestingly, we did not notice substantial differences in egg development rates and hatching success between Calanus females collected from different ambient conditions (open sea in 2009, fjord in 2010). In addition, the ranges in hatching times and success did not differ substantially between replicates (see the whiskers in ). Therefore, we believe that the locations of the sampling stations, sea temperature, and in situ chlorophyll concentrations did not have any substantial effect on the studied processes. However, since the number of eggs produced at each station varied, similarly as measured chlorophyll a concentrations, the results of egg-hatching experiments could have been biased towards stations where egg production rates were higher due to higher food availability (Hirche & Kattner Citation1993).
Kjellerup et al. (Citation2012), who examined the effects of temperature and food for C. finmarchicus and C. glacialis during three phases of the phytoplankton spring bloom in Disko Bay (western Greenland), found that C. glacialis sampled before the bloom, and incubated with excess food, exhibited high specific egg production at temperatures between 0 and 2.5°C, whereas egg production for C. finmarchicus more than tripled between 2.5 and 5°C. The authors suggested that a future warmer ocean would reduce the advantage of early spawning by C. glacialis and that C. finmarchicus would become increasingly prevalent. In our experiments, the egg development time of Arctic C. glacialis eggs was substantially longer than that of boreal C. finmarchicus above 2.0°C and on the decisive level of evidence above 3.6°C. Grenvald et al. (Citation2013) also demonstrated faster median hatching times in C. glacialis at lower temperatures (0°C) and in C. finmarchicus at higher temperatures (10°C); the authors explained this result by the different origin and specific temperature tolerance of each species.
The observed retarding of C. glacialis egg development rate at higher temperatures in comparison to C. finmarchicus, may have crucial and accumulating effects on the subsequent developmental stages. The reproduction and growth of C. glacialis is connected with the timing of ice algal and phytoplankton blooms, therefore the change in the current primary production regime due to global warming, coupled with higher sea temperatures, may lead to a mismatch between the timing of blooms and the reproductive cycle of this key Arctic grazer, while C. finmarchicus, which develops faster in higher temperatures, may take advantage of this situation.
In comparison to other developmental stages, eggs are particularly vulnerable to predation because they do not have the ability to escape, and reduced hatching times could lead to reduced predation rates and higher population abundances. Lower egg production rates with increasing temperatures (Kjellerup et al. Citation2012; Pasternak et al. Citation2013), coupled with longer egg development could eventually lead to decreasing numbers of the Arctic species and shift zooplankton community structure towards a smaller zooplankton size spectrum that is predominated by C. finmarchicus, with lower energy content per individual (Falk-Petersen et al. Citation2007). Such changes in the zooplankton community may have consequences for both terrestrial and marine lipid-driven Arctic ecosystems (Falk-Petersen et al. Citation2007; Stempniewicz et al. Citation2007; Zwolicki et al. Citation2013).
Calanus glacialis was reported to exhibit a tipping point at an ambient temperature of 5–6°C in its oceanic distribution (Carstensen et al. Citation2012), as well as in the rate of metabolic processes (Alcaraz et al. Citation2013). CitationPasternak et al. (2013) measured the egg production of both species, at the same experimental temperatures as used in this study and found that the egg production rates of C. finmarchicus increased with temperature over the entire tested range, but for C. glacialis the rates increased only in the range of 0–5°C and decreased with further temperature increases. Our experiments support the above findings, indicating that the temperature range of 5–6°C can be suggested as a threshold value for the Arctic C. glacialis in yet another biological characteristic that depends on temperature, the egg development time.
The above-mentioned studies and our results identifying the potential temperature tipping point are based mainly on Calanus individuals collected in the Svalbard area, whereas some of the identified differences between Calanus populations, e.g., by single nucleotide polymorphism in C. finmarchicus from the North Atlantic (Unal & Bucklin Citation2010), may have an effect on the results obtained for individuals of populations from different locations. It is not unlikely that these population differences could be the reason for lower median hatching times obtained for C. glacialis and C. finmarchicus from western Greenland (Grenvald et al. Citation2013).
From the tested models of B[ebreve]lehrádek's temperature function, with different combination of parameters, the model with three different parameters for each species proved to be the most accurate. Interestingly, parameter α, which is regarded as the species’ “biological zero” temperature (McLaren et al. Citation1969), was 3–4°C lower for the Arctic sibling in all versions of the model that we used. The commonly used model with a forced parameter b=−2.05 (e.g., Corkett et al. Citation1986; Campbell et al. Citation2001), which was postulated by McLaren et al. (Citation1969) to be applied to all copepod species, gave the worst fit to our experimental data, especially for C. finmarchicus. We propose new, species-specific values for parameter b in B[ebreve]lehrádek's temperature function for C. finmarchicus and C. glacialis egg hatching time, and we highlight the need to verify the parameters of important biological functions models. This issue is especially important due to the possibility of differences in temperature adaptations among populations of a single species over their distribution ranges, especially between regions where ambient temperatures are notably different. It is also worth noting that the use of Bayesian methods allowed us to not only to compare different models describing limited experimental data but also select the best-supported model based on its statistical confidence. The methods also allowed us to yield a more accurate estimation of the critical value of an important biological variable.
Acknowledgements
This research was funded by the Arctic Tipping Points project, contract no. 226248 of the Framework Program 7 of the European Union, the Polish State Committee for Scientific Research grant no. N N304 119834 and the Ministry of Science and Higher Education grant no. 1313/7. PR UE/2010/7.
References
- Alcaraz M., Felipe J., Grote U., Arashkevich E., Nikishina A. Life in a warming ocean: thermal thresholds and metabolic balance of Arctic zooplankton. Journal of Plankton Research. 2013; 36: 3–10.
- B[ebreve]lehrádek J. Temperature and living matter. 1935; Berlin: Borntraeger. (Protoplasma-Monographien.).
- Brooks S.P. Bayesian computation: a statistical revolution. Philosophical Transactions of the Royal Society A. 2003; 361: 2681–2697.
- Brooks S.P., Gelman A. Alternative methods for monitoring convergence of iterative simulations. Journal of Computational and Graphical Statistics. 1998; 7: 434–455.
- Campbell R.G., Wagner M.M., Teegarden G.J., Boudreau C.A., Durbin E.G. Growth and development rates of the copepod Calanus finmarchicus reared in the laboratory. Marine Ecology Progress Series. 2001; 221: 161–183.
- Carstensen J., Weydmann A. Tipping points in the Arctic: eyeballing or statistical significance?. Ambio. 2012; 41: 34–43.
- Carstensen J., Weydmann A., Olszewska A., Kwasniewski S. Effects of environmental conditions on the biomass of Calanus spp. in the Nordic seas. Journal of Plankton Research. 2012; 34: 951–966.
- Clark J.S. Why environmental scientists are becoming Bayesians. Ecology Letters. 2005; 8: 2–14.
- Clarke A. Costs and consequences of evolutionary temperature adaptation. Trends in Ecology and Evolution. 2003; 18: 573–581.
- Conover R.J. Comparative life histories in the genera Calanus and Neocalanus in high latitudes of the Northern Hemisphere. Hydrobiologia. 1988; 167/168: 127–142.
- Cook K.B., Bunker A., Hay S., Hirst A.G., Speirs D.C. Naupliar development times and survival of the copepods Calanus helgolandicus and Calanus finmarchicus in relation to food and temperature. Journal of Plankton Research. 2007; 29: 757–767.
- Corkett C.J., McLaren A., Sevigny J.M. The rearing of the marine calanoid copepods Calanus finmarchicus (Gunnerus), C. glacialis Jaschnov and C. hyperboreus Kroyer with comment on the equiproportional rule. Syllogeus. 1986; 58: 539–546.
- Falk-Petersen S., Timofeev S., Pavlov V., Sargent J.R. Ørbæk J.B., etal. Climate variability and possible effects on Arctic food chains: the role of Calanus. Arctic–alpine ecosystems and people in a changing environment. 2007; Berlin: Springer. 147–166.
- Grenvald J.C., Nielsen T.G., Hjorth M. Effects of pyrene exposure and temperature on early development of two co-existing Arctic copepods. Ecotoxicology. 2013; 22: 184–198.
- Hirche H.J., Kattner G. Egg production and lipid content of Calanus glacialis in spring: indication of a food-dependent and food-independent reproductive mode. Marine Biology. 1993; 117: 615–622.
- Huntley M.E., Lopez M.D.G. Temperature-dependent production of marine copepods: a global synthesis. American Naturalist. 1992; 140: 201–242.
- Jarque C.M., Bera A.K. A test for normality of observations and regression residuals. International Statistical Review. 1987; 55: 163–172.
- Jaschnov W.A. Distribution of Calanus species in the seas of the Northern Hemisphere. Internationale Revue gesamten Hydrobiologie. 1970; 55: 197–212.
- Jeffreys H. Theory of probability. 1961; 3rd edn, London: Oxford University Press.
- Karnovsky N.J., Weslawski J.M., Kwasniewski S., Walkusz W., Beszczynska-Moeller A. Foraging behavior of little auks in heterogeneous environment. Marine Ecology Progress Series. 2003; 253: 289–303.
- Kery M. Introduction to WinBUGS for ecologists: a Bayesian approach to regression, ANOVA, mixed models and related analyses. 2010; Amsterdam: Academic Press.
- Kjellerup S., Dunweber M., Swalethorp R., Nielsen T.G., Moller E.F., Markager S., Hansen B.W. Effects of a future warmer ocean on the so-existing copepods Calanus finmarchicus and C. glacialis in Disko Bay, western Greenland. Marine Ecology Progress Series. 2012; 447: 87–108.
- Lunn D.J., Best N., Whittaker J.C. Generic reversible jump MCMC using graphical models. Statistics and Computing. 2008; 19: 395–408.
- Lunn D.J., Jackson Ch., Best N., Thomas A., Spiegelhalter D. The BUGS book: a practical introduction to Bayesian analysis. 2012; Boca Raton, FL: CRC Press.
- Lunn D.J., Thomas A., Best N., Spiegelhalter D. WinBUGS—a Bayesian modelling framework: concepts, structure, and extensibility. Statistics and Computing. 2000; 10: 325–337.
- Lunn D.J., Whittaker J.C., Best N. A Bayesian toolkit for genetic association studies. Genetic Epidemiology. 2006; 30: 231–247.
- Madsen S.J., Nielsen T.G., Tervo O.M., Söderkvist J. On the importance of feeding for egg reproduction of Calanus finmarchicus and C. glacialis during the Arctic spring. Marine Ecology Progress Series. 2008; 353: 177–190.
- McLaren I., Corkett C.J., Zillioux E.J. Temperature adaptations of copepod eggs from the Arctic to the tropics. Biological Bulletin. 1969; 137: 486–493.
- Niehoff B., Madsen S.D., Hansen B.W., Nielsen T.G. Reproductive cycles of three dominant Calanus species in Disko Bay, West Greenland. Marine Biology. 2002; 140: 567–576.
- Pasternak A.F., Arashkevich E.G., Grote U., Nikishina A.B., Solovyev K.A. Different effects of increased water temperature on egg production of Calanus finmarchicus and C. glacialis. Oceanology. 2013; 53: 547–553.
- Planque B., Batten S.D. Calanus finmarchicus in the North Atlantic: the year of Calanus in the context of interdecadal change. ICES Journal of Marine Science. 2000; 57: 1528–1535.
- Søreide J.E., Leu E., Berge J., Graeve M., Falk-Petersen S. Timing of blooms, algal food quality and Calanus glacialis reproduction and growth in a changing Arctic. Global Change Biology. 2010; 16: 3154–3163.
- Spiegelhalter D.J., Best N.G., Carlin B.P., Van der Linde A. Bayesian measures of model complexity and fit (with discussion). Journal of the Royal Statistical Society B. 2002; 64: 583–639.
- Stempniewicz L., Blachowiak-Samolyk K., Weslawski J.M. Impact of climate change on zooplankton communities, seabird populations and Arctic ecosystem—a scenario. Deep-Sea Research Part II. 2007; 54: 2934–2945.
- Unal E., Bucklin A. Basin-scale population genetic structure of the planktonic copepod Calanus finmarchicus in the North Atlantic Ocean. Progress in Oceanography. 2010; 87: 175–185.
- Wassmann P. Arctic marine ecosystems in an era of rapid climate change. Progress in Oceanography. 2011; 90: 1–17.
- Weydmann A., Carstensen J., Goszczko I., Dmoch K., Olszewska A., Kwasniewski S. Shift towards the dominance of Boreal species in the Arctic: inter-annual and spatial zooplankton variability in the West Spitsbergen Current. Marine Ecology Progress Series. 2014; 501: 41–52.
- Weydmann A., Søreide J.E., Kwasniewski S., Leu E., Falk-Petersen S., Berge J. Ice-related seasonality in zooplankton community composition in a High Arctic fjord. Journal of Plankton Research. 2013; 35: 831–842.
- Zwolicki A., Zmudczyńska-Skarbek K.M., Iliszko L., Stempniewicz L. Guano deposition and nutrient enrichment in the vicinity of planktivorous and piscivorous seabird colonies in Spitsbergen. Polar Biology. 2013; 36: 363–372.