Abstract
The white-chinned petrel (Procellaria aequinoctialis) is the seabird species most frequently killed by fisheries in the Southern Ocean and is listed by the International Union for the Conservation of Nature and Natural Resources as globally vulnerable. It breeds around the sub-Antarctic, but genetic data identified two subspecies: P. a. aequinoctialis from islands in the Atlantic and Indian Oceans and P. a. steadi from the New Zealand sub-Antarctic islands. We identify the region of origin of birds killed by two long-line fisheries based on differences in the mitochondrial gene cytochrome b. All 113 birds killed off South Africa had the haplotype of P. a. aequinoctialis, whereas all the 60 birds from New Zealand had P. a. steadi haplotypes. The two subspecies of white-chinned petrels thus appear to disperse to different regions irrespective of their age, which accords with the tracking data of adult birds. Our finding has significant implications for managing the bycatch of this species by regional fisheries.
To access the supplementary material for this article, please see the supplementary file under Article Tools, online.
Caught on longlines or colliding with trawl cables, many seabirds are killed at sea (Croxall et al. Citation2012). As a result, most albatrosses and several large petrels are listed as threatened (ACAP Citation2009; BirdLife International Citation2016). The white-chinned petrel (Procellaria aequinoctialis) is the seabird species killed most frequently in Southern Ocean fisheries, with peak estimates of more than 50 000 birds killed annually (Ryan et al. Citation2012). It is confined to the Southern Ocean, with a circumpolar breeding distribution at sub-Antarctic islands (). Accurate population estimates are complicated by their burrow-nesting behaviour (Brooke Citation2004), but there is evidence that populations are decreasing (Phillips et al. Citation2006; Barbraud et al. Citation2009) and the species is listed as vulnerable, with the main threat being accidental mortality on fishing gear (BirdLife International Citation2016). Mitigation measures have been developed (Robertson et al. Citation2006) and national action plans implemented to reduce bycatch (Croxall et al. Citation2012). However, in order to assess the impact of fisheries on different island populations, we need to know where birds killed by specific fisheries breed, because differences in dispersal and foraging areas among island populations may affect their susceptibility to fishery bycatch (Berrow et al. Citation2000; Catard et al. Citation2000). Genetic markers can be useful to identify regions or colonies most at risk (e.g., Abbott et al. Citation2006).
Fig. 1 The main at-sea distribution and breeding locations of white-chinned petrels: Procellaria aequinoctialis aequinoctialis (circles) and P. a. steadi (squares).
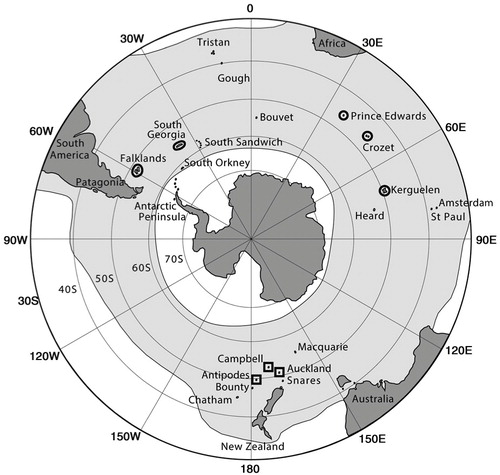
White-chinned petrels traditionally have been regarded as monotypic with few, if any, morphological differences among populations. Techow et al. (Citation2009) recognized two subspecies based on partial cytochrome b sequences: the nominate race from islands in the Atlantic and Indian Ocean sectors of the Southern Ocean, and P. a. steadi from the New Zealand sub-Antarctic islands (). Reasonably accurate population estimates are available for all breeding colonies of the nominate race (Ryan et al. Citation2012), but estimates of P. a. steadi are more crude. Best estimates suggest that they are much less abundant, comprising perhaps 20–25% of the nominate population (). Given this uneven distribution of breeding numbers, it is important to identify the colonies of origin for birds killed in different regional fisheries.
Table 1 Population estimates for white-chinned petrel breeding colonies (Brooke Citation2004; Barbraud et al. Citation2008; ACAP Citation2009; Barbraud et al. Citation2009; Martin et al. Citation2009; Ryan et al. Citation2012; BirdLife International 2016) and the number from each population used in this study. Numbers in parentheses are adult birds caught off breeding islands during the breeding season. See Techow et al. (Citation2009) for additional details.
Tracking and banding studies have found no evidence of movement of white-chinned petrels between colonies (Marchant & Higgins Citation1990; Hockey et al. Citation2005; Phillips et al. Citation2006; Péron et al. Citation2010) but relatively few individuals and colonies have been studied. The year-round movements of adults from four colonies have been tracked with light-logging geolocators. During the non-breeding season—the austral winter, from May to September—adults from South Georgia mainly occur off the east coast of South America (although some venture around Cape Horn to the west coast [Phillips et al. Citation2006]), whereas those from the Prince Edward Islands and Kerguelen occur off southern Africa (Péron et al. Citation2010; PGR unpubl. data) and those from New Zealand's Antipodes Islands visit the west coast of South America (D. Thompson, pers. comm.). However, these studies have only tracked adult birds. Genetic markers allow insights into the movements of all age classes, which is important given that non-breeders comprise more than half of the total white-chinned petrel population (Barbraud et al. Citation2008). In this study, we use the sequence differences in cytochrome b to assess whether the two subspecies of white-chinned petrels disperse to different foraging areas when not breeding.
Materials and methods
Blood and muscle tissue samples were collected from white-chinned petrels at their breeding islands and from birds killed by fishing vessels. Birds from breeding islands are summarized in , with full details in Techow et al. (Citation2009). Samples also were collected from birds of unknown provenance killed away from breeding colonies: 113 caught on longlines off South Africa in winter (May–October; 111 on tuna [Thunnus spp.] longlines and two on hake [Merluccius spp.] longlines), and 60 caught on New Zealand ling (Genypterus blacodes) longlines off southern and south-eastern New Zealand in early summer (November–December). Birds were sexed based on examination of gonads, and birds killed off South Africa were aged as juveniles (age 0–1 year), immature (maximum ca. 5 years old) or adult (>5 years) based on a combination of gonad condition, moult and bill structure (Marchant & Higgins Citation1990). Birds killed by long-line vessels were kept frozen until tissue samples could be collected and stored in 96% ethanol. Genomic DNA was extracted using an overnight Proteinase K digestion in extraction buffer (Fermentas; 10 mM Tris-HCl [pH 7.5], 10 mM MgCl2, 0.1 mg/ml BSA), followed by a phenol-chloroform extraction (Ausubel et al. Citation1989; Sambrook et al. Citation1989).
Cytochrome b sequencing and data analysis
A subset of 25 bycatch birds, selected at random, was sequenced for the cytochrome b gene; 11 from South Africa and 14 from New Zealand. PCR conditions are described by Techow et al. (Citation2009). Bands were excised from the agarose gel with a sterile blade and purified with the Promega Wizard® SV Gel and PCR Clean-up System. Of the resulting purified PCR products, 3 µl were electrophoresed on 2% agarose to determine the volume to be used as a template in a 10 µl BigDye® Terminator v3.1 Cycle Sequencing Kit (Applied Biosystems). Sequencing reaction products were purified using Centri-Sep Spin columns (Princeton Separations) and resolved by electrophoresis on an ABI 3130 Genetic Analyser. Haplotypes were compared with those identified previously from birds of known provenance (Techow et al. Citation2009). An unrooted Bayesian Inference tree was inferred with MrBayes version. 3.1.2 software. Default settings were used unless otherwise indicated (Huelsenbeck & Ronquist Citation2001), with haplotypes identified by Techow et al. (Citation2009) used to identify associations between bycatch haplotypes and those of known provenance. This tree was constructed with 13 million generations being computed with a burn-in of 13 000 trees in five independent runs sampling every 500th generation. Four Markov Chain Monte Carlo chains were run for each analysis, and Tracer version 1.4 software was used to test whether runs converged. The evolutionary model chosen was HKY+G, because it had the best fit to the data using MODELTEST version 3.06 software (Posada & Crandall Citation1998).
Restriction enzyme analysis of a SNP
The remaining 148 white-chinned petrels of unknown origin (102 from South Africa and 46 from New Zealand) were subjected to a restriction enzyme digest after amplifying the same fragment of the cytochrome b gene. Three fixed mutational differences were found between the two subspecies of white-chinned petrel (n=83 birds; Techow et al. Citation2009), allowing the two populations to be segregated with the restriction enzyme Csp6I (supplied by Fermentas). Cytochrome b genes from the New Zealand population P. a. steadi have two Csp6l sites resulting in three fragments of 311, 173 and 114 bp, whereas P. a. aequinoctialis from islands in the Atlantic and Indian Ocean have only one Csp6l site resulting in two fragments of 484 and 114 bp. PCR amplification of cytochrome b was as described by Techow et al. (Citation2009). PCR products were electrophoresed on 2% agarose gels. Size of products was determined according to a DNA ladder (Promega 100 bp DNA ladder). Digest conditions were as follows: five units of Csp6I, 1× digestion buffer B, 5 µl of PCR product in a total volume of 14.5 µl. Digests were incubated at 37°C for two hours and inactivated by incubating at 65°C for 20 min. Products were electrophoresed on 3% agarose. Each agarose gel contained a control sample of known origin.
Results
Twenty-five individuals of unknown provenance killed at sea off South Africa and New Zealand had 658 bp of cytochrome b sequenced (GenBank accession numbers: EU053404–EU053425), revealing five additional haplotypes to the 17 identified by Techow et al. (Citation2009) from 83 birds of known origin (Supplementary Table S1). The novel haplotypes nested with their hypothesized respective regional groupings; all birds from South African fisheries grouped with haplotypes from nominate birds, whereas the New Zealand bycatch grouped with P. a. steadi (). One individual (haplotype wcpB19), caught off New Zealand, contained two mutations indicative of P. a. steadi but had only one cutting site with the SNP, as would be expected for the nominate subspecies. It raises the possibility that some individuals assigned to P. a. aequinoctialis using the SNP could be P. a. steadi. Despite this, the SNP method found that all birds sampled off New Zealand (n=46) were identified as P. a. steadi and all those caught off South Africa (n=102) as P. a. aequinoctialis. There was no significant deviation from a 50:50 sex ratio in samples from either South Africa (χ 2=0.46, df=1, P=0.49) or New Zealand (χ 2=1.35, P=0.25), and roughly 64% of birds killed off South Africa were juvenile or immature birds (n=113).
Fig. 2 Unrooted haplotype tree constructed using Bayesian Inference haplotypes for the two subspecies of white-chinned petrels in relation to spectacled (Procellaria conspicillata, sp1-3) and grey petrel (P. cinerea), adapted from Techow et al. (Citation2009). Probability values are given indicating support for the topology. Haplotypes found in bycatch are represented in boldface; wcpB18-19 from the New Zealand long-line fishery and wcpB20-22 from South Africa.
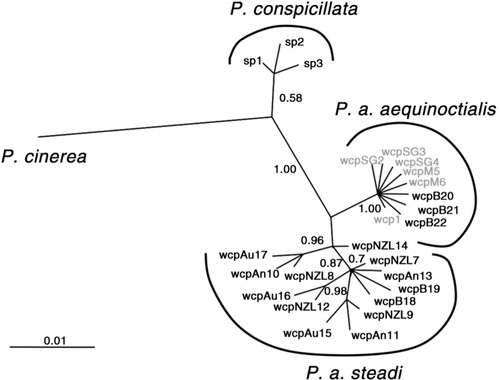
Discussion
Most procellariiformes are philopatric to their natal and breeding sites (Warham Citation1990), which is conducive to the evolution of population differentiation among colonies over time. Among albatrosses, species that feed primarily in shelf waters are more strongly structured genetically than are those with oceanic foraging ranges (Abbott & Double Citation2003; Burg & Croxall Citation2001, Citation2004; Friesen et al. Citation2007). Breeding white-chinned petrels range widely, foraging in both oceanic and coastal shelf waters (Weimerskirch et al. Citation1999; Catard et al. Citation2000; Delord et al. Citation2010) but mainly disperse to shelf waters when not breeding (Phillips et al. Citation2006; Péron et al. Citation2010). As a result, we might expect them to demonstrate some genetic structure. Cytochrome b analysis has found two distinct regional populations, one from New Zealand and another in the Atlantic and Indian Oceans (Techow et al. Citation2009). This conclusion was supported by the larger samples of birds included in our study, which identified a few additional haplotypes to those reported by Techow et al. (Citation2009).
Tracking studies using geolocators show that adult white-chinned petrels from South Georgia, the Prince Edward Islands, Kerguelen and the Antipodes Islands disperse to largely discrete non-breeding areas (Phillips et al. Citation2006; Péron et al. Citation2010; D. Thompson, pers. comm.; PGR unpubl. data). Sample sizes in these studies are modest, but the limited individual variation detected suggests that adults from different breeding islands tend to have different non-breeding sites, at least at a regional level, even within subspecies. Nominate adults from South Georgia spend the non-breeding season off South America (Phillips et al. Citation2006), whereas those from the Prince Edward Islands and Kerguelen spend the winter off southern Africa (Péron et al. Citation2010; PGR unpubl. data). However, little is known about the dispersal patterns of juvenile and immature white-chinned petrels. Only three birds banded as chicks have been reported away from their breeding islands: all were from the Crozets and were recovered as immatures off southern Africa (Marchant and Higgins Citation1990; Hockey et al. Citation2005), the same region where non-breeding adults from the southern Indian Ocean colonies spend the austral winter (Péron et al. Citation2010). Our results from the fishery bycatch birds support this contention; there was no evidence of immatures from New Zealand visiting the waters off South Africa, where immatures accounted for almost two-thirds of the birds sampled. The ages of birds sampled off New Zealand were not recorded, but it is likely that a substantial proportion were immatures, as adults comprise only about half of the population of white-chinned petrels (Barbraud et al. Citation2008). As a result, the absence of birds from the Atlantic or Indian Ocean colonies from samples collected off New Zealand suggests little interchange of pre-breeding birds between these regions (although most New Zealand samples were collected in summer, when older immature birds might be expected to return to the vicinity of their breeding islands).
Knowledge of at-sea ranges is crucial for the management of colonies at risk. Our results suggest that little, if any, mixing of the two subspecies occurs at the two non-breeding locations sampled. The impact of a given fishery is therefore largely confined to nearby breeding colonies. Most birds killed off South Africa probably originate from Indian Ocean colonies (Prince Edwards, Crozet and Kerguelen), whereas those killed off New Zealand probably come from the New Zealand sub-Antarctic islands. This is of concern for these regional breeding populations, given that the largest population breeds at South Georgia (Martin et al. Citation2009). The population in the southern Indian Ocean may be threatened given high levels of mortality off South Africa and Namibia (Ryan et al. Citation2012), and the New Zealand population of P. a. steadi requires strict conservation, because although it comprises only 20% of the global population, it is genetically the most diverse (Techow et al. Citation2009). Extending our study to white-chinned petrels killed off South America would be extremely interesting, particularly given the apparent overlap between New Zealand and South Georgia birds off the west coast of South America (Phillips et al. Citation2006; D. Thompson, pers. comm.).
Confidence in subspecies/colony assignments could be increased and discriminatory power enhanced with additional markers. Because neither of the two fixed differences in cytochrome b sequences between the two subspecies were recognized by restriction enzymes, the potential ambiguity in SNP results caused by one P. a. steadi haplotype could be resolved with mismatch primers (FitzSimmons et al. Citation1997). However, the level of discrimination offered by cytochrome b is too coarse to segregate between specific island populations. Other tools that could be used to attempt to segregate populations include morphometric differences or biogeochemical markers such as stable isotope ratios (Gómez-Díaz & González-Solis Citation2007). Such additional markers are needed to segregate between nominate white-chinned petrels from the large South Georgia population and those from breeding colonies in the southern Indian Ocean.
Supplementary Material
Download PDF (86.5 KB)Acknowledgements
Permission to collect samples from breeding islands was granted by the relevant authorities; we thank Pete McClelland, Deon Nel, Richard Phillips, Erica Sommer and Henri Weimerskirch for assistance with collecting samples. Fishery observers kindly collected birds killed by fishing vessels. Funding and logistical support was obtained from the South African National Antarctic Programme, the South African National Research Foundation and the University of Cape Town.
Notes
To access the supplementary material for this article, please see the supplementary file under Article Tools, online.
References
- Abbott C., Double M. Phylogeography of shy and white-capped albatrosses inferred from mitochondrial DNA sequences: implications for population history and taxonomy. Molecular Ecology. 2003; 12: 2747–2758. [PubMed Abstract].
- Abbott C., Double M.C., Gales R., Baker G.B., Lashko A., Robertson C.J.R., Ryan P.G. Molecular provenance analysis for shy and white-capped albatrosses killed by fisheries interactions in Australia, New Zealand, and South Africa. Conservation Genetics. 2006; 7: 531–542.
- ACAP (Agreement on the Conservation of Albatrosses and Petrels) 2009. Species assessment: white-chinned petrel Procellaria aequinoctialis. Accessed on the internet at http://www.acap.aq/acap-species on 26 May 2016.
- Ausubel F., Brent R., Kingston R. Current protocols in molecular biology. 1989; New York: Wiley.
- Barbraud C., Delord K., Marteau C., Weimerskirch H. Estimates of population size of white-chinned petrels and grey petrels at Kerguelen Islands and sensitivity to fisheries. Animal Conservation. 2009; 12: 258–265.
- Barbraud C., Marteau C., Ridoux V., Delord K., Weimerskirch H. Demographic response of a population if white-chinned petrels Procellaria aequinoctialis to climate and longline fishery bycatch. Journal of Applied Ecology. 2008; 45: 1460–1467.
- Berrow S., Wood A., Prince P.A. Foraging location and range of white-chinned petrels Procellaria aequinoctialis breeding in the South Atlantic. Journal of Avian Biology. 2000; 31: 303–311.
- BirdLife International 2016. Threatened birds of the world. Accessed on the internet at http//www.birdlife.org on 26 May 2016.
- Brooke M. Albatrosses and petrels across the world. 2004; Oxford: Oxford University Press.
- Burg T., Croxall J.P. Global relationships amongst black–browed and grey-headed albatrosses: analysis of population structure using mitochondrial DNA and microsatellites. Molecular Ecology. 2001; 10: 2647–2660. [PubMed Abstract].
- Burg T., Croxall J.P. Global population structure and taxonomy of the wandering albatross species complex. Molecular Ecology. 2004; 10: 2345–2355.
- Catard A., Weimerskirch H., Cherel Y. Exploitation of distant Antarctic waters and close shelf-break waters by white-chinned petrels rearing chicks. Marine Ecology Progress Series. 2000; 194: 249–261.
- Croxall J.P., Butchert S.H.M., Lascelles B., Stattersfield A.J., Sullivan B., Symes A., Taylor P. Seabird conservation status, threats and priority actions: a global assessment. Bird Conservation International. 2012; 22: 1–34.
- Delord K., Cotté C., Péron C., Marteau C., Pruvost P., Gasco N., Duhamel G., Cherel Y., Weimerskirch H. At-sea distribution and diet of an endangered top predator: relationship between white-chinned petrels and commercial longline fisheries. Endangered Species Research. 2010; 13: 1–16.
- FitzSimmons N.N., Limpus C.J., Norman J.A., Goldizen A.R., Miller J.D., Moritz C. Philopatry of male marine turtles inferred from mitochondrial DNA markers. Proceedings of the National Academy of Science of the United States of America. 1997; 94: 8912–8917.
- Friesen V.L., Burg T.M., McCoy K.D. Mechanisms of population differentiation in seabirds. Molecular Ecology. 2007; 16: 1765–1785. [PubMed Abstract].
- Gómez-Díaz E., González-Solis J. Geographic assignment of seabirds to their origin: combining morphologic, genetic, and biogeochemical analyses. Ecological Applications. 2007; 17: 1484–1498.
- Hockey P.A.R., Dean W.R.J., Ryan P.G. Roberts’ birds of Southern Africa. 2005; Cape Town: Trustees of the John Voelcker Bird Book. 7th edn.
- Huelsenbeck J., Ronquist F. MrBayes: Bayesian inferences of phylogeny. Bioinformatics. 2001; 17: 754–755. [PubMed Abstract].
- Marchant S., Higgins P.J. Handbook of Australian, New Zealand and Antarctic birds. Vol. 1. 1990; Melbourne: Oxford University Press.
- Martin A.R., Poncet S., Barbraud C., Foster E., Fretwell P., Rothery P. The white-chinned petrel (Procellaria aequinoctialis) on South Georgia: population size, distribution and global significance. Polar Biology. 2009; 32: 655–661.
- Péron C., Delord K., Phillips R.A., Charbonnier Y., Marteau C., Louzao M., Weimerskirch H. Seasonal variation in oceanographic habitat and behaviour of white-chinned petrels Procellaria aequinoctialis from Kerguelen Island. Marine Ecology Progress Series. 2010; 416: 267–284.
- Phillips R.A., Silk J.R.D., Croxall J.P., Afanasyev V. Year-round distribution of white-chinned petrels from South Georgia: relationships with oceanography and fisheries. Biological Conservation. 2006; 129: 336–347.
- Posada D., Crandall K. MODELTEST: testing the model of DNA substitution. Bioinformatics. 1998; 14: 817–818. [PubMed Abstract].
- Robertson G., McNeill M., Smith N., Wienecke B., Candy S., Olivier F. Fast-sinking (integrated weight) longlines reduce mortality of white-chinned petrels (Procellaria aequinoctialis) and sooty shearwaters (Puffinus griseus) in demersal longline fisheries. Biological Conservation. 2006; 132: 458–471.
- Ryan P.G., Dilley B.J., Jones M.G.W. The distribution and abundance of white-chinned petrels (Procellaria aequinoctialis) breeding at the sub-Antarctic Prince Edward Islands. Polar Biology. 2012; 35: 1851–1859.
- Sambrook J., Fritsch E., Maniatis T. Molecular cloning: a laboratory manual. 1989; New York: Cold Spring Harbor Laboratory Press. 2nd edn.
- Techow N., Ryan P.G., O'Ryan C. Phylogeography and taxonomy of white-chinned and spectacled petrels. Molecular Phylogenetics & Evolution. 2009; 52: 25–33. [PubMed Abstract] [PubMed CentralFull Text].
- Warham J. The petrels—their ecology and breeding systems. 1990; Sydney: Academic Press.
- Weimerskirch H., Catard A., Prince P.A., Cherel Y., Croxall J.P. Foraging white-chinned petrels Procellaria aequinoctialis: at risk from the tropics to Antarctica. Biological Conservation. 1999; 87: 273–275.