Abstract
Eight potential aflatoxin-sequestering agents (SAs) were tested for their ability to adsorb aflatoxin B1 (AfB1) and aflatoxin G1 (AfG1) in vitro. They belong to main SA classes: silicate minerals (calcium, magnesium and sodium bentonites, kaolinite, zeolite and clinoptinolite), activated carbon and yeast cell wall-derived. The AfB1 and AfG1 used in present work were extracted from a contaminated corn meal (82.21 mg/kg of AfB1 and 97.20 mg/kg of AfG1). Three single-concentration adsorption tests, consisting of a simply-water (W), a gastro-intestinal simulating monogastric model (MM) and a ruminant model (RM) were used. The methods differed for dilution media, incubation steps and pH condition in which they were conducted. In particular, one step (2h at 39°C) at pH 7 for W; two steps (4h at 39°C) at pH 2 and 7 for MM; and a pre-incubation in rumen fluid (pH 7 for 2h at 39°C) + two steps (4h at 39°C) at pH 2 and 7 for RM, characterized each method. The AfB1:SA ratio (g/g) and dilution factor (ng of incubated AfB1:mL of volume) were chosen (1:500,000 and 4.1, respectively) to reflect field conditions. The AfB1 and AfG1 recovered in controls were 92.3% and 104.9% in W and 89.5% and 101.5% in MM; while in RM were 65.2% and 81.9%; respectively. This supported the idea of intrinsic rumen fluid factors could be involved in sequestering of aflatoxins. In the present study, three SAs (activated carbon, Mg bentonite and Na bentonite) were very efficient to sequester the available AfB1, with a sequestering activity of over 99.0% with each method. The Ca bentonite and clinoptinolite were able to bind available AfB1 in MM and RM methods, while they appeared inefficient (available AfB1 sequestered less than 80%) when W was used. The adsorption ability of zeolite was confirmed only with the W method. Ineffective or limited sequestering activity were obtained with kaolinite and yeast cell wall-derived products with each method. The AfB1 and AfG1 sequestering efficiencies observed in the present work resulted very similar showing strong and positive correlation (P<0.001) within methods (r=0.79, r=0.96 and r=0.99, respectively for W, MM and RM methods). The two simulated gastrointestinal methods (MM and RM, respectively) gave similar results and could be considered useful for in vitro pre-screening of potential sequestering agents. However, the major practical and analytical implications related to rumen fluid method suggested that MM method should be used.
Introduction
Aflatoxins are hepatocarcinogenic molecules (CitationIARC, 2002) produced mainly by Aspergillus flavus and A. parasiticus either in field, storage or transport of many crops (CitationScheidegger and Payne, 2003). The primary aflatoxins produced by these fungi are aflatoxin B1(AfB1), aflatoxin B2 (AfB2), aflatoxin G1 (AfG1), and aflatoxin G2 (AfG2).
The AfB1, the most toxic and carcinogenic aflatoxin (CitationRoebuck and Maxuitenko, 1994), once ingested by dairy animals is rapidly absorbed in the gastro-intestinal tract, and rapidly appears in blood (CitationGallo et al., 2008) and in milk as aflatoxin M1 (AfM1), the principal AfB1 milk metabolite (CitationVeldman et al., 1992; CitationBattaccone et al., 2003; CitationMasoero et al., 2007). The AfB1 carry over (CO) rate into milk as AfM1 has been determined to range from 1% to 3% in lactating dairy cows and to be principally affected by milk yield (CitationDiaz et al., 2004; CitationVan Eijkeren et al., 2006; CitationMasoero et al., 2007), with a reported maximum value of about 6% (CitationVeldman et al., 1992).
These findings supported the European Union (EU) decision to fix limits for AfB1 in animal feeds at 20 µg/kg and in complete feedingstuffs for dairy animals at 5 µg/kg (CitationEuropean Commission, 2003). In milk, the EU set the AfM1 maximum permitted level at 0.050 µg/kg (CitationEuropean Commission, 2006).
A practical approach to control aflatoxicosis in livestock is the addition of aflatoxin-sequestering agents (SA) to diet (CitationCAST, 2003). These compounds are effective to sequester toxin molecules, such as aflatoxins, forming a complex in the gastrointestinal tract (CitationJouany, 2007; CitationMasoero et al., 2009) through electronic elementary charges, hydrogen bonds, Van der Waals bonds and physical capture (CitationPhillips et al., 1990; CitationDiaz and Smith, 2005; CitationYiannikouris et al., 2005; CitationPhillips et al., 2008). Besides, they are added to animal diet in small amount and are impurities-, flavour- and odours-free (CitationDiaz and Smith, 2005; CitationJouany, 2007).
A variety of SAs has been tested and currently traded. Some of the most commonly used SAs belong to three different groups: silicate materials or clay minerals (CitationPhillips et al., 1991; CitationRamos and Hernandez, 1996; CitationRao and Chopra, 2001; CitationDiaz et al., 2003; CitationJouany, 2007), yeast cell wall-based products (CitationKaraman et al., 2005; CitationYiannikouris et al., 2005) and activated carbons (CitationGalvano et al., 1996; CitationRao and Chopra, 2001; CitationDiaz et al., 2003; CitationDiaz and Smith, 2005).
Several in vitro adsorption tests have been proposed to pre-screen SAs before their use in vivo. Differences in the findings of in vitro studies are based upon the tested aflatoxins (crystalline pure AfB1 or extracted from natural contaminated feeds), the dilution factor (AfB1 concentration, w/v), the AfB1:SA ratio (w/w), the pH conditions and the media in which adsorption test was conducted (CitationRamos and Hermandez, 1996; CitationGrant and Phillips, 1998; CitationLedoux and Rottinghaus, 1999). Moreover, these methods often oversimplify the gastrointestinal conditions, such as pH, temperature and interaction with biological fluids (CitationLemke et al., 2001; CitationAvantaggio et al., 2003; CitationMoschini et al., 2008).
The objective of this work was to evaluate the efficacy of different typologies of SAs (mineral clays, activated carbon and yeast cell wallbased product) to adsorb AfB1 and AfG1 either in aqueous or in simulated monogastric/ruminant gastrointestinal fluids.
Materials and methods
Eight potential-aflatoxin SAs were evaluated for their capability to sequester aflatoxins in vitro. The SAs were: magnesium bentonite (Grupo Tolsa, Madrid, Spain); calcium bentonite (Tecnozoo, Padova, Italy); sodium bentonite (Amcol International Corp., Arlington Heights, IL, USA); kaolinite (Fluka 03584, Sigma-Aldrich Chemie Gmbh, Switzerland); zeolite (Fluka 96096, Sigma-Aldrich Chemie Gmbh, Switzerland); clinoptinolite (Tecno-zoo, Padova, Italy); yeast cell wall-based product (Mycosorb®, Alltech Italy, Bologna, Italy), and activated carbon (Acque Nymco, Milan, Italy). Only some of these products (i.e., magnesium bentonite, calcium bentonite, clinoptinolite and yeast cell wall-based product) are specifically marketed as mycotoxin SA by the producers.
Chemicals
Solvents used were of grade ACS-ISO. Acetonitrile, acetone, chloroform and methanol were HPLC grade (purity 99.5%, 99.0%, 99.0% and 99.9%; respectively) from Merck (Darmstadt, Germany). Purified water was obtained from a Milli-Q Gradient A10 water purification device (Millipore, Bedford, MA, USA). Phosphate-buffered saline (PBS) was prepared by dissolving 0.20 g KCl, 0.20 g KH2 PO4, 1.16 g anhydrous Na2 HPO4 and 8.00 g NaCl in 1 L of water. The pH was adjusted to 7.4 with NaOH (0.1 mol/L).
Preparation of aflatoxin test solution (solution A)
Aflatoxins (AfB1 and AfG1) were extracted from a contaminated corn meal inoculated with the Aspergillus flavus strain MPVP 2092 (Institute of Entomology and Plant Pathology, Università Cattolica del Sacro Cuore, Piacenza, Italy). The corn meal was incubated at 25°C and 0.99 water activity for 7 days, respecting the optimum mycotoxin production conditions (CitationGiorni et al., 2007). The final aflatoxin concentrations of the corn meal were: 82.21±0.01 mg/kg for AfB1 and 97.20±0.01 mg/kg for AfG1.
A stock solution (solution A) of AfB1 and AfG1 was prepared by extracting aflatoxins from 1 g of the contaminated corn meal using a water/methanol solution (80:20, v/v) in ratio of 1:100 (g/mL), at room temperature and in agitation (150 shake/min) for 120 min. The obtained solution A concentrations were 0.821 µg/mL for AfB1 and 0.974 µg/mL for AfG1, respectively.
Single concentration adsorption tests
A simply-water (W), a gastro-intestinal simulating monogastric model (MM) and a ruminant model (RM) were used to test the sequestering capacity of the different SAs. The W and MM were performed in agreement with CitationLemke et al. (2001), while the RM consisted of a pre-incubation with rumen fluid (CitationMoschini et al., 2008), followed by incubation steps applied for MM model.
The three methods differed for the dilution media, incubation steps and the pH conditions in which the adsorption tests were conducted:
W method: one step in deionized water at 39°C for 2h;
MM method : two steps at 39°C for 4h in two buffers (pH 2 and 7) to simulate gastrointestinal conditions of monogastri;
RM method : one step in rumen fluid (pH 7 for 2h at 39°C) followed by the two MM steps (pH 2 and 7 for 4h at 39°C), to simulate the ruminant digestible tract.
The pH 2 buffer used in the first step of MM method was prepared in agreement with CitationLemke et al. (2001) as follows: 1.250 g/L of pepsin enzyme, 0.500 g/L of citric acid monohydrate, 0.500 g/L of malic acid disodium salt, 0.050 mL/L of acetic acid and 0.042 mL/L of lactic acid. In the second step, the pH was increased up to 7 with sodium bicarbonate, followed by addition of pancreatine (20 mg/sample) and bile salts (70 mg/sample).
The RM method consisted of a pre-incubation in rumen fluid. The rumen fluid was collected from two fistulated cows housed at the CERZOO research and experimental centre (San Bonico, Piacenza, Italy) before the morning meal (08.00), filtered with a two layers cheesecloth, stored at 39°C under C02 and used within 1 h. The measured pH of the rumen fluid was 7.0±0.3. After this step, HCl 1M was used to reach pH 2 in test tubes and 50 mg/sample of pepsin was added to each sample. The third step of RM method was carried out by increasing pH up to 7 with sodium bicarbonate followed by the addition of pancreatine (20 mg/sample) and bile salts (70 mg/sample).
The AfB1:SA ratio used in the current study were designed to be about 1:500,000 (w/w), in agreement with AfB1:SA ratio used in our previous work (CitationMoschini et al., 2008). This ratio was chosen to reflect possible field conditions. In particular, dairy cows fed with an AfB1 contaminated diet near to the EU limit of 5 µg/kg (CitationEU, 2003) consumed, on average, 100–120 µg/head/day AfB1 in presence of a SA dose of about 50–60 g/head/day, as suggested by the producers for on farm utilization. A similar approach could be used for swine fed with a diet containing 1% of a SA with an AfB1 contamination of 20 µg/kg, representing the swine EU feedstuff limit (CitationEU, 2003). The dilution factor, considered as the ratio between the amount of incubated AfB1 (ng) and the total volume (mL) in which the absorption test was conducted, was similar for the three methods and equal to 4.1 (ng/mL), in agreement with CitationMoschini et al. (2008).
Incubation procedure
Single SAs were weighted (82.0±0.1 mg) in 100-mL test tubes (Pyrex®; Barloworld Scientific Ltd., Staffordshire, UK) for each method (W, MM and RM). Then, 0.200 mL of solution A and 40 mL of the three media, precisely deionized water (W), pH 2 buffer (MM) or rumen fluid (RM), were added to each test tube and gently shacked for 5 minutes every 15 minutes. Each single-concentration adsorption test was repeated twice.
Control samples (n=3) for each method (W, MM and RM, respectively) were prepared by adding 0.200 mL of solution A and 40 mL of specific media into 100-mL test tubes without the addition of the SA.
At the end of incubations for all samples, the supernatant was removed after centrifugation (3500 × g for 15 min at 4°C) and then the precipitate washed twice with 10 mL of water. The recovered supernatant (about 30 mL) as a whole was analyzed by HPLC. In particular for RM samples, to measure AfB1 and AfG1 sequestered by rumen fluid, the precipitate of the controls were treated in agreement with CitationMoschini et al. (2008) and analyzed for aflatoxin contents: the supernatant was removed and the precipitate was re-suspended in 10 mL chloroform before centrifugation. This step was repeated twice, and the recovered solution was analyzed by HPLC to measure the AfB1 and AfG1 contained in the rumen pellet of controls.
The sequestered aflatoxins (AfB1 and AfG1) by the SAs in each sample were calculated as percentage ratio between the aflatoxin quantity of supernatant and its amount in the control sample.
Animals
The ruminal fluid cow donors were fed on a diet formulated in agreement with dairy cow requirements (CitationNRC, 2001) and based on grass hay (700 g/kg), corn silage (200 g/kg) and concentrate (100 g/kg) on a dry matter basis. The research protocols and animal care were performed in accordance with the EC council directive guidelines regarding the use of animals for experimental and other scientific purposes (CitationEEC,1986).
Aflatoxin and SAs analysis and apparatus
Aflatoxins (AfB1 and AfG1) were analyzed in agreement with CitationMoschini et al. (2008). Briefly, 10 grams of dried contaminated corn meal were extracted with 100 mL of an acetone:water solution (85:15, v/v), shacked at 150 rpm for 45 min (Universal table Shaker 709) and filtered with Schleicher&Schuell 595.5 filter paper (Dassel, Germany). Elutions of 5 mL with 45 mL of bi-distilled water were performed and passed through an immuno-affinity column (Aflatoxin Easi-extract, Rhône diagnostics technologies, Glasgow, UK). The supernatant solution was passed through an immunoaffinity column previously washed with 20 mL of PBS. The column was than washed with 5 mL water and slowly eluted with 2.5 mL of methanol. The extract was dried under nitrogen, re-dissolved in 1 mL of an acetonitrile:water (25:75, v/v) solution and filtered (Millipore Corporation, Bedford, MA, USA, HV 0.45 µM) prior to HPLC analysis for the AfB1 and AfG1 contents.
Analysis was performed using an HPLC instrument consisting of a LC-200 pump (Perkin Elmer, Norwalk, CT, USA) an AS-2055 sampling system, a FP-1520 fluorescence detector (Jasco Corporation, Tokyo, Japan), and a UV derivatizer (UVE TM derivatizer, LC tech, Dorfen, Germany); the instrument was controlled by Borwin 1.5 software (Jasco). A Superspher RP-18 column (4 µm particle size, 125×4 mm i.d., Merck) was used at room temperature with a mobile phase of water: methanol:acetonitrile (64:23:13, v/v/v) at 1 mL/min. The AfB1 and AfG1 were detected after post-column photochemical derivatization to AfB2a and AfG2a, respectively. The detector was set at 365 nm excitation and 440 nm emission wavelengths.
The elemental components of the SAs were determined by a semi-quantitative X-ray fluorescence analysis using the scanning electron microscope Phillips XL 30 E-SEM (Phillips electron Optics B.V., Eindhoven, Netherlands) equipped with an energy-dispersive X-ray detector model Genesis (Edax Inc., Mc Kee Drive, Mahwah NY, USA) operating in low vacuum. The SA samples were assayed in duplicate according to the CitationAOAC (1990) to determine total ash content (procedure 942.05).
Statistical analysis
The in vitro data were analyzed using a complete randomized design and the general linear model of the SAS® (SAS Institute Inc., Cary, NC; version 9.1.3). A factorial arrangement was used and fixed effects of model included SA (n=8), method (n=3) and associated first order interaction. When data suggested the first order interaction, the SLICE option of LSMEANS statement was used to compare SAs within each tested method. The correlation between AfB1 and AfG1 sequestering efficiency was calculated with the Spearman rank correlation coefficient using PROC CORR of SAS (CitationSAS Institute Inc., 2001) within each method. Significance was declared at P<0.05.
Results and discussion
The most used SAs belong to the silicate mineral, the activated carbon and the yeast cell-wall product (CitationDiaz and Smith, 2005; CitationJouany, 2007) classes. The widest SA class is the silicate minerals, which could be grouped in phyllosilicate and tectosilicate sub-classes. The SAs belonging to phyllosilicate sub-class are montmorillonite/smectite, such as bentonite and kaolinite clays, while the tectosillicate sub-class includes zeolite and clinoptinolite clays (CitationDiaz and Smith, 2005).
In the present work, the tested clays had an ash content higher than 90% (). In particular for bentonite clays, the dominant cation characterized the type of bentonite, as confirmed by elementary chemical composition of magnesium bentonite (Mg 14.8%), calcium bentonite (Ca 14.8%) and sodium bentonite (Na 3.4%). Zeolite and kaolinite clays appeared different with respect to other clays having a similar Al and Si content (14.1% and 14.6% for zeolite and 18.6% and 21.0% for kaolinite, respectively). The high carbon content of activated carbon (89.6%) and yeast cell wall (61.4%) suggested an organic origin, even if the available analytical data can not exclude a possible clay presence, particularly for the yeast cell wall product.
Table 1 Elementary composition and total ash content (mean ± SD) of the tested adsorbents.
Single concentration adsorption tests
Before their use in animal diets, in vitro pre-screening tests of potential SAs are necessary to evaluate the sequestering efficiency of these materials in controlled experimental conditions. If a SA sequesters less than 80% of the available in vitro aflatoxins, it could be considered as ineffective and not tested in vivo (CitationLedoux and Rottinghaus, 1999; CitationDiaz and Smith, 2005).
Several lab methods have been proposed, but they differed for experimental conditions (CitationPhillips et al., 1988; CitationGalvano et al., 1996; CitationRamos and Hermandez, 1996; CitationGrant and Phillips, 1998; CitationLedoux and Rottinghaus, 1999; CitationLemke et al., 2001; CitationDiaz et al., 2003; CitationSpotti et al., 2005; CitationVekiru et al., 2007; CitationMoschini et al., 2008; CitationThieu and Petterson, 2008). These differences among methods could cause contrasting results for the same SA, when tested with different adsorption tests: CitationMoschini et al. (2008) reported the effect of AfB1:SA ratio (i.e., 1:5000; 1:50,000 and 1:500,000) on the sequestering performance of SAs. Also CitationVekiru et al. (2007) showed that adsorption abilities of a commercial HSCAS and a bentonite were influenced by the constituents and the pH of the incubation media (i.e. acetate buffer vs artificial or real gastro intestinal media). Similar discrepancies among methods were obtained by CitationLemke et al. (2001) testing a clinoptinolite in two single-concentration adsorption studies conducted in water media or in a gastro-intestinal model media. These authors emphasized the importance of using methods simulating the animals’ physiological conditions. More recently, CitationSpotti et al. (2005) and CitationMoschini et al. (2008) tested the SA efficiency using an in vitro method conducted in rumen fluid media, demonstrating a partial sequestering activity of rumen fluid against AfB1.
In the present work, the average AfB1 and AfG1 recovered in control samples in absence of any type of sequestering agents were 92.3% and 104.9% in W method (), in accordance to our previous results (CitationMoschini et al., 2008). Similar results were obtained in MM method (89.5% and 101.5%, respectively for AfB1 and AfG1), in agreement with CitationLemke et al. (2001). The acid condition (pH 2) in which the first step of MM method was conducted did not influence the amount of AfB1 recovered, showing the absence of the reaction of AfB1 to AfB2a (CitationVekiru et al., 2007). This could be related to the limited incubation time. The AfB1 presence observed in control samples of W and MM methods determinate an AfB1:SA ratio of about 1:500,000, as designed before experiments.
Table 2 Aflatoxin B1 (AfB1) and aflatoxin G1 (AfG1) recovered in supernatants, in rumen pellet and as total in water, simulating gastro-intestinal monogastric model and ruminant total tract model methods in control samples.
In RM method, the aflatoxin determine in the supernatant of the control after the centrifugation of the rumen fluid media were equal to 36.6% for the AfB1 and 55.6% for AfG1. The residual AfB1 extracted with chloroform from the precipitate was 28.6% and similar to AfG1 rumen pellet content, in agreement with previous published data (CitationSpotti et al., 2005; CitationMoschini et al., 2008). The total AfB1 and AfG1 recovered in control samples of RM method were 65.2% and 81.9%, respectively. Therefore, the AfB1:SA ratio for RM method was different with respect to other methods, being about 1:750,000. This supports the idea that some rumen fluid components such as chlorophyllin, bacteria and yeast cell wall could be effective in sequestering aflatoxins (CitationBreinholt et al., 1999; CitationPeltonen et al., 2000; CitationDiaz and Smith, 2005; CitationGratz et al., 2005; CitationOatley et al., 2005). In particular, CitationArimoto-Kobayashi et al. (1997) suggested that chlorophyllin structure could form a complex between porphyrin-like structure of chlorophyllin and the polycyclic structures of carcinogenic molecules, like AfB1. Also specific cell wall components in yeast (CitationDawson et al., 2001) and bacteria, i.e. lactobacilli, interacted with mycotoxins forming a stable complex (CitationGratz et al., 2005; CitationOatley et al., 2005; CitationNiderkom et al., 2009).
CitationUpadhaya et al. (2009) recently reported a loss of AfB1 ranging from 8 to 20% when in vitro incubated at different times (0, 3, 6, 9 and 12 h) with rumen fluids collected by goats and steers. These authors supposed that AfB1 was degraded by rumen microflora. CitationNiderkorn et al. (2007), testing more than 200 bacterial strains to verify their ability to bind and/or biotransform mycotoxins (i.e., deoxyvalenol, zearalenon and fumonisin B1 and B2), reported that most strains were capable to bind Fusarium toxins. In particular, these authors indicated Streptococcus spp. and Enterococcus spp. as the most effective genera, while only few strains (i.e., 8 Lactobacilli spp. and 3 Leiconostoc spp.) could be involved in the biotrasformation of zearalenone in α-zearalenol. However, these bacteria did not biotransform other studied mycotoxins as deoxynivalenol and fumonisins B1 and B2.
SA sequestering activity
Our results showed that three potential SAs (i.e., activated carbon, Mg bentonite and Na bentonite) exhibited strong AfB1-sequestering capacity, with values higher than 99.0% (), in all experimental condition and with no differences among methods ().
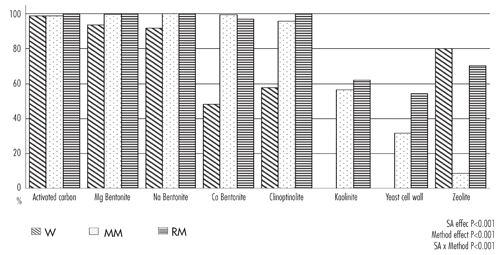
Table 3 Analysis of variance (ANOVA) of sequestering agents, method and first order interaction effects on the AfB1 and AfG1 sequestering efficiency data.
The high affinity of the activated carbon for AfB1 molecula confirmed the results obtained by other authors (CitationGalvano et al., 1996; CitationLemke et al., 2001; CitationRao and Chopra, 2001; CitationVekiru et al., 2007). The mechanism involved in the sequestering of aflatoxin could be the physical capture by the pore of activated carbon structure, even if it could depend by other factors, such as surface area, structure and dose of mycotoxins (CitationGalvano et al., 2001). However, the use of activated carbons as chemoprotective against aflatoxins produced variable results in vivo. CitationGalvano et al. (1996) reported that only one of the two tested activated carbons at 2.0% inclusion level in the animal diet resulted effective to reduce AfM1 excretion in lactating dairy cows, while CitationDiaz et al. (2004) did not observe a significant reduction of the AfM1 milk concentration when an activated carbon was added to the diet at 0.25% inclusion level.
Two bentonites (Mg and Na bentonites) were highly efficient to adsorb the available AfB1 in vitro. These results were in agreement with the ones by different authors (CitationDvorak, 1989; CitationRamos and Hermandez, 1996; CitationRao and Chopra, 2001; CitationDiaz et al., 2004; CitationMoschini et al., 2008), suggesting bentonite’s ability to adsorb AfB1 in several media, such as water, buffer solution, saline solution, swine stomach and bovine rumen fluids. More recently, CitationVeriku et al. (2007), testing 61 different bentonite materials, concluded that AfB1 was generally strongly bound by these clays, both in a pH 5 acetate buffer or in a pH 7 phosphate buffer. These results are consistent with data reported by CitationDiaz et al. (2003), comparing different SAs (i.e., Na and Ca bentonite, esterified glucomannan and activated carbons) at pH 3, 7 and 10. Moreover, CitationMoschini et al. (2008) reported that the AfB1-bentonite complex was very stable in the gastro-intestinal tract of lactating dairy cows, with an estimated release of the sequestered AfB1 lower than 5%.
CitationMasoero et al. (2009) testing the Mg bentonite in vivo on lactating dairy cows, measured a significant reduction in the AfM1 milk concentration (44%) and in the AfB1 carry over into milk (47%), when the SA was added at a level of 100 g/cow/day to an AfB1-contaminated diet causing an ingestion of 174 µg of AfB1/cow/diet.
In the present study, more than 97% of the available AfB1 was adsorbed by the Ca bentonite using MM and RM methods, while an inefficient sequestering activity was observed using the W method (i.e., 48%). Similar differences amog methods were observed with clinoptinolite clay, that could be considered effective to bind AfB1 if tested with MM and RM methods (96% and 100% sequestering activities, respectively), while it appeared inefficient in W media. In agreement with our observation, CitationLemke et al. (2001) reported that under MM conditions, a clinoptinolite showed a sequestering activity not observed in other methods. These authors concluded that the MM model was the most physiologically relevant method to pre-screen potential SAs.
Ineffective or limited sequestering activity was observed for kaolinite and yeast cell wall products in all methods. In particular, these products were not able to sequester AfB1 when tested in W media, while they showed poor sequestering activities both in MM and in RM (57% and 62% for kaolinite and 32% and 54% for yeast cell wall, respectively in MM and RM method) ().
The results obtained for yeast cell wall-derived products confirmed our previous data, in which a low in vitro efficiency was measured in all experimental conditions (CitationMoschini et al., 2008). In particular, the pH and the solvent type appeared to be critical in the reduction of the ability of β-d-glucans, a major component of the inner layer of the yeast cell wall, to complex mycotoxins (CitationYiannikouris et al., 2005). A previous report by CitationDawson et al. (2001) suggested that a pH of 4.0 was optimal for yeast cell wall product activity.
Differences among methods showed that the two gastro-intestinal model tests (MM and RM methods) gave higher (P<0.05) sequestering affinity than W method when Ca bentonite, clinoptinolite, kaolinite and yeast cell wall products were tested. This was not true for zeolite, which resulted efficient at binding AfB1 in W, sequestering about the 80% of the available AfB1, while it appeared inefficient using the MM method. Also CitationThieu et al. (2008), found that a zeolite product had a different capacity to adsorb AfB1 using a single concentration gastro intestinal method at pH 3 or pH 7 (80% and 20% sequestering activities, respectively).
Contrasting in vivo data were obtained adding zeolite to animal diet (CitationShariatmadari, 2008). CitationModirsanei et al. (2004) reported that zeolinite at 0.50% and 0.75% inclusion in the broiler chick diet did not reduce any adverse effects due to AfB1 ingestion (1.0 mg/kg of diet). On the contrary, CitationMiazzo et al. (2005) suggested that zeolinite is able to counteract same AfB1-depended toxic effects in growing broiler chicks ingesting 2.5 mg of AfB1/kg of diet. Similarly, CitationAbdel-Wahhab et al. (2002) reported improved haematological and biochemical parameters in rats fed with a contaminated AfB1(2.5 mg/kg) diet containing sorbent compound derived from a natural zeolite.
The AfB1 and AfG1 sequestering efficiencies observed in this experiment with the three methods resulted very similar (). This was confirmed by statistical analysis of the data: strong and positive correlations (P<0.001) were measured between AfB1 and AfG1 sequestering activity in MM and RM methods (r=0.96 and r=0.99, respectively). Less strong correlation was found for the W method (r=0.79; P<0.001).
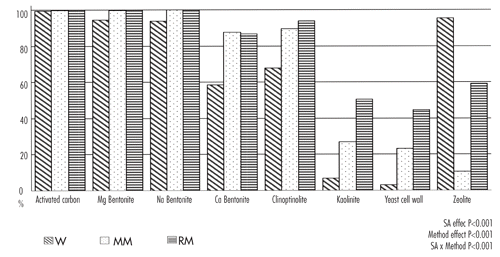
Despite some small differences between the chemical structure of the aflatoxin molecules (AfB1 and AfG1 belong to cyclopentenone and lactone series, respectively), these data seem to confirm suggestions of CitationPhillips et al. (2008) concerning the mechanism of aflatoxin absorption by clays: the process should be not site-specific because it involves the β-dicarbonyl system of AfB1 and AfG1 molecules, thought the chelation of metal ions at the surface and within the interlayer of silicate clays. The carbons of the β-dicarbonyl system have a partial positive charge and they could be attracted through an electron donor acceptor mechanism by the negatively charges of platelets of silicates. CitationPhillips et al. (2006) reported a good correlation between the magnitude of partial positive charges on carbons C11 and C1 of the β-dicarbonyl system and the strength of AF-SA complex.
Conclusions
Based on the results of the current paper, obtained with the in vitro pre-screening tests, three SAs products (activated carbon, Mg bentonite and Na bentonite) could be considered efficient at sequestering the available AfB1, resulting as promising agents for in vivo trials. The Ca bentonite and clinoptinolite products were able to adsorb available AfB1 in MM and RM model methods, while they appeared inefficient when the adsorbent test was performed in water media. The adsorption ability of zeolite was confirmed only by the W method.
The simulated gastrointestinal methods presented in the current paper (MM and RM, respectively) gave similar results and could be considered useful for in vitro pre-screening of potential sequestering agents. However, the major analytical complexity of the rumen fluid method supports the idea that the 2-steps MM method proposed by CitationLemke et al. (2001) should be used.
Acknowledgments:
This research was supported by the AFLARID, Ministry of Agricultural, Food and Forestry Policies (MiPAAF, Italy) project.
References
- Abdel-Wahhab M.A. Nada S.A. Khalil F.A. 2002 Physiological and toxicological responses in rats fed aflatoxin-contaminated diet with or without sorbent materials Anim. Feed Sci. Tech 97 209–219
- AOAC 1990 Official Methods of Analysis 15th ed. AOAC Washington, DC, USA
- Arimoto-Kobayashi S. Harada N. Tokunaga R. Odo J. Hayatsu H. 1997 Adsorption of mutagens to chlorophyllin-chitosan, an insoluble form of chlorophyllin Mutat. Res 381 243–249
- Avantaggio G. Havenaar R. Visconti A. 2003 Assessing the zearalenone-binding activity of adsorbent materials during passage through a dynamic in vitro gastrointestinal model Food Chem. Toxicol 41 1283–1290
- Battacone G. Nudda A. Cannas A. Borlino A.C. Bomboi G. Pulina G. 2003 Excretion of aflatoxin M1 in milk of dairy ewes treated with different doses of aflatoxin B1 J. Dairy Sci 86 2667–2675
- Breinholt V. Arbogast D. Loveland P. Pereira C. Dashwood R. Hendricks J. Bailey G. 1999 Chlorophyllin chemoprevention in trout initiated by aflatoxin B1 bath treatment: an evaluation of reduced bioavailability vs. target organ protective mechanisms Toxicol. Appl. Pharm 158 141–151
- CAST 2003 Mycotoxins: Risk in plant, animal and human system Ames ed. Iowa, USA
- Dawson K.A. Evans J. Kudupoje M. 2001 Understanding the adsorption characteristics of yeast cell wall preparations associated with mycotoxin binding Lyons T.P. Jacques K.A. Science and Technology in the Feed Industry Nottingham University Press Nottingham, UK 169–181
- Diaz D.E. Hagler W.M.J. Blackwelder J.T. Eve J.A. Hopkins B.A. Anderson K.L. Jones F.T. Whitlow L.W. 2004 Aflatoxin binders II: Reduction of aflatoxin M1 in milk by sequestering agents of cows consuming aflatoxin in feed Mycopathologia 157 233–241
- Diaz D.E. Hagler W.M.J. Hopkins B.A. Whitlow L.W. 2003 Aflatoxin Binders I: In vitro binding assay for aflatoxin B1 by several potential sequestering agents Mycopathologia 156 223–226
- Diaz D.E. Smith T.K. 2005 Mycotoxin sequestering agents: practical tools for the neutralisation of mycotoxins Diaz D.E. The Mycotoxin Blue Book Nottingham University Press Thrumpron, Nottingham, UK 323–339
- Dvorak M. 1989 Ability of bentonite and natural zoelite to absorb aflatoxin from liquid media Vet. Med-US 34 733–741
- European Commission 1986 Council Directive of 24 November 1986 on the approximation of laws, regulations and administrative provisions of the Member States regarding the protection of animals used for experimental and other scientific purposes, 86/609/EEC Official Journal, L 358 24 11 1986 1–28
- European Commission 2003 Commission Directive of 31 October 2003 amending Annex I to Directive 2002/32/EC of the European Parliament and of the Council on undesirable substances in animal feed (Text with EEA relevance), 2003/100/EC Official Journal, L285 31 10 2003 33–37
- European Commission Commission Regulation of 19 December 2006 setting maximum levels for certain contaminants in foodstuffs (Text with EEA relevance), 2006/1881/EC 2006 Official Journal, L364 19 12 2006 5–24
- Gallo A. Moschini M. Masoero F. 2008 Aflatoxins absorption in the gastro-intestinal tract and in the vaginal mucosa in lactating dairy cows Ital. J. Anim. Sci 7 53–63
- Galvano F. Pietri A. Fallico B. Bertuzzi T. Scirè S. Galvano M. Maggiore R. 1996 Activated carbons: in vitro affinity for aflatoxin B1 and relation of adsorption ability to physicochemical parameters J. Food Protect 59 545–550
- Galvano F. Piva A. Ritieni A. Galvano G. 2001 Dietary strategies to counteract the effects of mycotoxins: a review J. Food Protect 64 120–131
- Giorni P. Battilani P. Pietri A. Magan N. 2007 Effect of aw and CO2 level on Aspergillus flavus growth and aflatoxin production in high moisture maize post-harvest Int. J. Food Microbiol 122 109–113
- Grant P.G. Phillips T.D. 1998 Isothermal adsorption of aflatoxin B1 on HSCAS clay J. Agric. Food Chem 46 599–605
- Gratz S. Mykkanen H. El Nezami H. 2005 Aflatoxin B1 binding by a mixture of Lactobacillus and Propionibacterium: in vitro versus ex vivo J. Food Protect 68 2470–2474
- IARC 2002 World health organization international agency for research on cancer. Aflatoxins: B1, B2, G1, G2, M1. IARC monographs on the evaluation of carcinogenic risks to humans IARC ed. Lyon, France
- Jouany J.P. 2007 Methods for preventing, decontaminating and minimizing the toxicity of mycotoxins in feeds Anim. Feed Sci. Tech 137 342–362
- Karaman M. Basmacioglu H. Ortatatli M. Oguz H. 2005 Evaluation of the detoxifying effect of yeast glucomannan on aflatoxicosis in broilers as assessed by gross examination and histopathology Brit. Poultry Sci 46 394–400
- Ledoux D.R. Rottinghaus G.E. 1999 In vitro and in vivo testing of adsorbents for detoxifying mycotoxins in contaminated feedstuffs Lyons T.P. Jacques K.A. Biotechnology in the Feed Industry Nottingham University Press Nottingham, UK 369–379
- Lemke S.L. Ottinger S.E. Mayura K. Ake C.L. Pimpukdee K. Wang N. Phillips T.D. 2001 Development of a multi-tiered approach to the in vitro prescreening of claybased enterosorbents Anim. Feed Sci. Tech 93 17–29
- Masoero F. Gallo A. Diaz D. Piva G. Moschini M. 2009 Effects of the procedure of inclusion of a sequestering agent in the total mixed ration on proportional aflatoxin M1 excretion into milk of lactating dairy cows Anim. Feed Sci. Tech 150 34–45
- Masoero F. Gallo A. Moschini M. Piva G. Diaz D. 2007 Carryover of aflatoxin from feed to milk in dairy cows with low or high somatic cell counts Animal 9 1344–1350
- Miazzo R. Peralta M.F. Magnoli C. Salvano M. Ferrero S. Chiacchiera S.M. Carvalho E.C.Q. Rosa C.A.R. Dalcero A. 2005 Efficacy of Sodium Bentonite as a Detoxifier of Broiler Feed Contaminated with Aflatoxin and Fumonisin Poultry Sci 84 1–8
- Modirsanei M. Khosravi A.R. Kiaei S.M.M. Fard M.H.B. Gharagozloo M.J. Khazraeinia P. 2004 Efficacy of dietary natural zeolite and Saccharomyces cerevisiae in counteracting aflatoxicosis in broiler chicks J. Appl. Anim. Res 26 39–44
- Moschini M. Gallo A. Piva G. Masoero F. 2008 The effects of rumen fluid on the in vitro aflatoxin binding capacity of different sequestering agents and in vivo release of the sequestered toxin Anim. Feed Sci. Tech 147 292–309
- Niderkorn V. Morgavi D.P. Aboab B. Lemaire M. Boudra H. 2009 Cell wall component and mycotoxin moieties involved in the binding of fumonisin B1 and B2 by lactic acid bacteria J. Appl. Microbiol 106 977–985
- Niderkorn V. Morgavi D.P. Pujos E. Tissandier A. Boudra H. 2007 Screening of fermentative bacteria for their ability to bind and biotransform deoxynivalenol, zearalenone and fumonisins in an in vitro simulated corn silage model Food Addit. Contam 24 406–415
- NRC 2001 Nutrient requirements of dairy cattle 7th rev. ed. National Academy Press Washington, DC., USA
- Oatley J.T. Rarick M.D. GeunEog J. Linz L.E. 2000 Binding of aflatoxin B1 to bifidobacteria in vitro J. Food Protect 63 1133–1136
- Peltonen K.D. El Nezami H.S. Salminen S.J. Ahokas J.T. 2000 Binding of aflatoxin B1 by probiotic bacteria J. Sci. Food Agr 80 1942–1945
- Phillips T.D. Afriyie-Gyawu E. Wang J.S. Williams J. Huebner H. 2006 The potential of aflatoxin sequestering clay Barug D. Bhatnagar D. Van Egmond H.P. Van der Kamp J.W. Van Osenbruggen W.A. Visconti A. The mycotoxin factbook Wageningen Academic ed. Wageningen, The Netherlands 329–346
- Phillips T.D. Afriyie-Gyawu E. Williams J. Huebner H. Ankrah N.A. Ofori-Adjei D. Jolly P. Johnson N. Taylor J. Marroquin-Cardona A. Xu L. Tang L. Wang J.S. 2008 Reducing human exposure to aflatoxin through the use of clay: a review Food Addit. Contam 25 134–135
- Phillips T.D. Kubena L.F. Harvey R.B. Taylor D.R. Heidelbaugh N.D. 1988 Hydrated sodium calcium aluminosilicate: A high affinity sorbent for aflatoxin Poultry Sci 67 243–247
- Phillips T.D. Sarr A.B. Clement B.A. Kubena L.F. Harvey R.B. 1990 Prevention of aflatoxicosis in farm animals via selective chemisorption of aflatoxin Bray G.A. Ryan D.H. Mycotoxins, Cancer, and Health Louisiana State Univ. Press Baton Rouge, LA, USA 223–237
- Phillips T.D. Sarr A.B. Clement B.A. Kubena L.F. Harvey R.B. 1991 Prevention of aflatoxicosis in farm animals via selective chemisorption of aflatoxin Bray GA. Ryan D.H. Mycotoxins, Cancer, and Health Louisiana State University Press Baton Rouge, Louisiana, USA 223–237
- Ramos A.J. Hernandez E. 1996 In vitro aflatoxin adsorption by means of a montmorillonited silicate. A study of adsorption isotherms Anim. Feed Sci. Tech 62 263–269
- Rao S.B.N. Chopra S.C. 2001 Influence of sodium bentonite and activated charcoal on aflatoxin M1 excretion in milk of goats Small Ruminant Res 41 203–213
- Roebuck B.D. Maxuitenko Y.Y. 1994 Bio-chemical mechanism and biological implications of the toxicity of aflatoxins as related to aflatoxin carcinogenesis Eaton D.L. Groopman J. D. The Toxicology of Aflatoxins: Human Health, Veterinary, and Agricultural Significance Academic Press Inc San Diego, CA, USA 27–43
- SAS 2001 Statistical Analysis System Proprietary Software. Release 8.2 SAS Institute Inc Cary, NC, USA
- Scheidegger K.A. Payne G.A. 2003 Unlocking the secrets behind secondary metabolism: a review of Aspergillus flavus from pathogenicity to functional genomics J. Toxicol. Toxin. Rev 22 423–459
- Shariatmadari F. 2008 The application of zeolite in poultry production World Poultry Sci. J 64 76–84
- Spotti M. Fracchiolla M.L. Arioli F. Caloni F. Pompa G. 2005 Aflatoxin B1 binding to sorbents in bovine ruminal fluid Vet. Res. Commun 29 507–515
- Thieu N.Q. Pettersson H. 2008 In vitro evaluation of the capacity of zeolite and bentonite to adsorb aflatoxin B1 in simulated gastrointestinal fluids Mycotoxin Res 24 124–129
- Upadhaya SD. Sung HG. Lee CH. Lee SY. Kim SW. Cho KJ. Ha JK. 2009 Comparative study on the aflatoxin B1 degradation ability of rumen fluid from Holstein steers and Korean native goats J Vet. Sci 10 29–34
- Van Eijkeren J.C.H. Bakker M.I. Zeilmaker M.J. 2006 A simple steady-state model for carry-over of aflatoxins from feed to cow's milk Food Addit. Contam 23 833–838
- Veldman A. Meijs J.A.C. Borggreve G.J. Heeres van der Tol J.J. 1992 Carry-over of aflatoxin from cows' food to milk Anim. Prod 55 163–168
- Veriku E. Fruhauf S. Sahin M. Ottner F. Schatzmayr G. Krska R. 2007 Investigation of various adsorbents for their ability to bind aflatoxin B1 Mycotoxin Res 23 27–33
- Yiannikouris A. Bertin G. Jouany J.P. 2005 Reducing mycotoxin impact: the science behind Mycosorb®. Nutritional biotechnology in the feed and food industries 265–276 Proc. 21st Annual Symp. Bio-technology in the Feed Industry Lexington, KY, USA