Abstract
In this study, the cDNA sequence of the transferrin (Tf) gene in tongue sole (Cynoglossus semilaevis) (CsTf) was identified and characterised. The full length of CsTf cDNA was 2281 bp encoding 677 amino acids (aa). The mature CsTf protein was found to be made up of 656 aa and it consisted of two lobes (N- and C-lobes). Two- and three- dimensional (2-D and 3-D) structure analysis showed that CsTf possesses a similar structure (multi-α helix/β-sheet) to human Tf. Real-time polymerase chain reaction (PCR) analysis showed that CsTf was mainly expressed in the liver, and moderately expressed in the heart, spleen and kidney. Moreover, CsTf was up-regulated in different tissues under acute hypoxia [dissolved oxygen (DO)=0.8 mg/L]. Our results suggest that CsTf plays a role in fisH’s adaptation to hypoxic stress.
Introduction
Aquatic hypoxia has become a complex ecological phenomenon because of the increasing industrialisation and urbanisation of coastal zones (CitationWawrowski et al., 2011). Research has shown an increasing amount of genes that are down- or up-regulated under hypoxia. These genes are mainly involved in maintaining iron homeostasis, energy levels and muscle structure (CitationWulff et al., 2012).
Transferrin (Tf) is one of the members of a super-family of iron-binding proteins. It is expressed mainly in the liver and is also found in other tissues, including the brain, testes, ovary, spleen, mammary gland and kidney. Transferrin possesses the typical structure of the Tf family, which includes a N-terminal domain (N-lobe) and a C-terminal domain, connected by a short hinge region. Under hypoxia, mammalian Tf is up-regulated, thus demonstrating that it plays a role under hypoxic stress (CitationWenger et al., 1995). The exact molecular mechanism of hypoxic enhancement of Tf expression was elucidated by CitationRolfs et al. (1997). They found that the Tf promoter contained two hypoxia-inducible factor-1 (HIF-1) binding sites (HBSs), which could be bound by HIF-1 to confer oxygen regulation of Tf expression.
To date, most research on Tf has been carried out on several fishes, including Atlantic salmon (Salmo salar) (CitationKvingedal, 1994), Atlantic cod (Gadus morhua) (CitationDenovan-Wright et al., 1996), rainbow trout (Oncorhynchus mykiss) (CitationTange et al., 1997), mrigal carp (Cirrhinus mrigala) (CitationSahoo et al., 2009) and roughskin sculpin (Trachidermus fasciatus) (CitationLiu et al., 2012). The fish Tf gene is very similar to mammalian Tf and is mainly expressed in the liver or brain too. Fish Tf serves as a cadmium transport protein (CitationDe Smet et al., 2001) and activator of immune response of macrophages (CitationStafford et al., 2001; CitationJurecka et al., 2009). However, little is known regarding the response of Tf under hypoxia in fish.
The tongue sole (Cynoglossus semilaevis) is an important marine aquaculture species in China (CitationShao et al., 2010). Our previous study found that this species possesses specific adaptations to hypoxia (CitationWang et al., 2011a). However, there is limited information on its molecular responses under hypoxia. Therefore, in the current study, the Tf gene from tongue sole was cloned and characterised. Its structure and tissue distribution were determined, and the expression pattern under hypoxia was examined as well.
Materials and methods
Fish
The fishes used for the experiments were adult tongue sole (Cynoglossus semilaevis) with a mean length of 24.64±1.01 cm (±SD) and a mean weight of 77.44±8.21 g. Prior to the experiments, the fishes were acclimated on a 14-h light/10-h dark cycle at 21°C for 2 weeks.
Cloning the Cs Tf
Total RNA was extracted from the liver using TRIzol Reagent (Invitrogen, Carlsbad, CA, USA). After digesting with RNase-free DNase I (TaKaRa Bio Inc., Otsu, Japan) for 30 min at 37°C, total RNA was reverse transcripted into first-strand cDNA using SuperScript Reverse Transcriptase (Invitrogen) according to the manufacturer’s instructions.
The Japanese flounder (Paralichthys olivaceus) Tf sequence (GenBank accession no.: ACZ92269) was used to search the Expressed Sequence Tag (EST) database [National Center for Biotechnology Information (NCBI) (http://blast.ncbi.nlm.nih.gov/Blast.cgi)] using the BLASTP tool which identified one tongue sole EST (GenBank accession no.: GH229616). Further analysis showed that this EST shared a high identity with the middle sequence of Japanese flounder Tf. Specific primers (Tf-F1 and Tf-R1, listed in ) were designed according to the identified EST in order to clone this partial sequence from the liver tissue of tongue sole. After confirmation by sequencing, primers used for 3 and 5 rapid amplification of cDNA ends (RACE) were designed on the basis of the sequence obtained. The polymerase chain reaction (PCR) cycling program was: 1 cycle of 94°C for 5 min; 9 cycles of 94°C for 30 s, 64°C for 30 s, and 72°C for 90 s; 25 cycles of 94°C for 30 s, 62°C for 30 s, and 72°C for 90 s; 1 cycle of 72°C for 10 min. A 1225-bp 3 -RACE product was obtained and it contained the C-terminal of the transferrin (Tf) gene in Cynoglossus semilaevis (CsTf) coding region and 3 -prime untranslated region (UTR). A 395-bp 5 -RACE product was also obtained. It enclosed the 5 -UTR and N-terminal partial coding region. Primers used for the gene clone are listed in .
Table 1 Primers used for cloning and expression analysis of CsTf.
Sequence analysis
The deduced amino acids (aa) were predicted using the software at the ExPASy molecular biology server (http://www.expasy.org). Multiple sequence alignments were performed by CLUSTAL 1.8 (CitationClewley and Arnold, 1997) and shaded with Genedoc software (CitationNicholas et al., 1997). The signal peptide was predicted using the SignalP programme (http://www.cbs. dtu.dk/services/SignalP/) (CitationBendtsen et al., 2004), while the DISULFIND programme (http://disulfind.dsi.unifi.it/) was used to predict Disulfide bonds (http://disulfind.dsi.unifi.it/). The protein secondary structure was predicted foreseen using PSIPRED (http://bioinf.cs.ucl.ac.uk/psipred/). Phylogenetic trees were created by following the neighbour-joining (N-J) method using the MEGA programme (version 4.1), and were bootstrapped for 10,000 times.
Three-dimensional model of CsTf
We searched for the best template for CsTf using GeneSilico Metaserver (CitationKurowski and Bujnicki, 2003), Pcons.net (CitationWallner and Elofsson, 2005), and BLAST analysis. After determining the template, the three dimensional (3-D) structure of CsTf was constructed in the SWISS-MODEL server (http://expasy. org/swissmod/SWISS-MODEL.html) on the basis of the comparative modelling method. The model obtained was then validated by PROCHECK (CitationLaskoswki et al., 1993). We performed protein visualisation using the DeepView software.
Acute hypoxic stress
In total, 90 tongue soles were used for acute hypoxic stress. Groups of five fish were randomly assigned to the hypoxia group or control group. The control group was maintained under normoxic conditions (DO=6.2 mg/L). Acute hypoxic conditions (DO=0.8 mg/L) in the hypoxic stressed group were maintained by bubbled with nitrogen gas as previously described (CitationWang et al., 2011a, Citation2011b). During the experiments, the DO, pH and temperature were monitored using the YSI 556 multiprobe system (YSI Inc., Yellow Springs, OH, USA). The heart, liver, spleen, kidney, intestine, stomach, blood and gills from three fishes in each group were sampled after continuous exposure for 5, 30, 60, 90 and 120 min, and stored at −80°C until use.
Quantitative real-time polymerase chain reaction
The basal and hypoxic stressed expression patterns of CsTf were examined using the SYBR green-based real-time PCR method. RNA preparation and cDNA synthesis from different tissues were as previously described (CitationWang et al., 2011a, Citation2011b). The primers used for realtime PCR analysis were confirmed by the reverse transcription polymerase chain reaction (RT-PCR) and melting curve analysis on an ABI 7300 real-time PCR system (Applied Biosystems, Carlsbad, CA, USA). The real-time PCR was run in duplicate for each sample and carried out using a two-step method: 30 s at 95°C, followed by 40 cycles of 95°C for 5 s and 60°C for 31 s. Basal gene expression in normal tissue was measured relative to the gene expression of β-actin. The expression of CsTf under hypoxic stress was measured using the standard curve method and calculated using the following formula (CitationWang et al., 2011b):
Real-time PCR data were analysed using Origin 6.0. Results are expressed as mean±SD. The significance between the stressed and the control groups was evaluated using the Student’s t test, with P<0.05.
Results
Sequence analysis of CsTf
The CsTf cDNA sequence was 2281 bp, and it contained a 5 -UTR of 28 bp, an open reading frame of 2034 bp, and a 3 -UTR of 219 bp. There was one mRNA instability motif (ATTTA) and a polyadenylation signal (AATAAA) 16 bp upstream of the polyA tail (). The CsTf cDNA encoded 677 aa with a 21 aa signal peptide which was predicted using SignalP 3.0. The mature secreted CsTf was 656 aa, with a theoretical molecular mass of 74.90 kDa and an iso-electric point of 6.15. Similar to human Tf, CsTf also contained two lobes: the N-lobe comprising 23–326 residues and the C-lobe comprising 337–656 residues. The two lobes were connected by a short hinge region (327–336 residues). The secondary structure analysis showed that each lobe contained two subunits possessing a conserved /βstructure. Eight iron-binding residues (Asp71/385, Tyr 101/198/420/512 and His253/576) were predicted using ScanProsite. Four conserved Tf super-family motifs were also found in CsTf at the following residues: 101–110 (YYAVAVAKKE), 420–429 (YFAVAVIKRD), 198–213 (YDGAFRCLKEKGDVAF), and 512–528 (YTGALKCLADGVGDVAF) (CitationAnderson et al., 1989). Four conserved iron-binding sites in each lobe (Asp71, Tyr101, Tyr195 and His253 in the N-lobe; Asp385, Tyr420, Tyr512 and His576 in the C-lobe) and two anion sites in each lobe (Gly127 and Lys130 in the N-lobe; His444 and Arg449 in the C-lobe) remained conserved in CsTf (CitationFord, 2012). A total of 32 cysteines were found in CsTf. Analysis using DISULFIND showed that 24 cysteines might form 12 disulfide bonds, with six bonds in each lobe (). The CsTf shared 48.1% to 63.3% sequence identity with Tf from other vertebrates; the highest was with the Japanese flounder Tf (63.3%) and the lowest was with human Tf (48.1%). Analysing the sequence identity of the N-lobe and C-lobe of CsTf showed that these lobes shared a high identity with those in different species. The N-lobe of CsTf shared 65.6% identity with the N-lobe of Japanese medaka Tf, 66.5% with the N-lobe of Japanese flounder Tf, and 49.3% with the N-lobe of human Tf. The C-lobe of CsTf shared 58.5% identity with the C-lobe of Japanese medaka Tf, 59.4% with the C-lobe of Japanese flounder Tf, and 49.6% with the C-lobe of human Tf. The CsTf N-lobe shared 36% identity with its C-lobe.
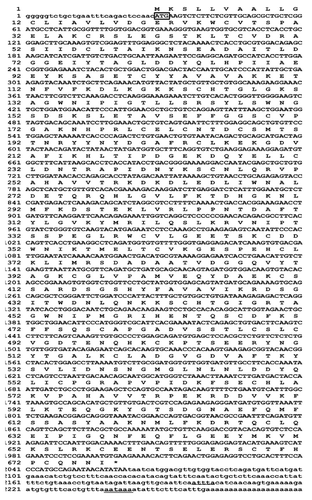
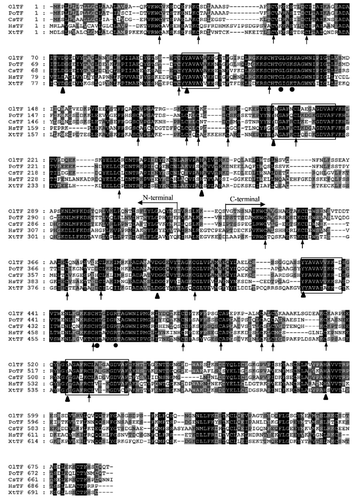
Phylogenetic analysis
To further analyse the evolutionary context of the CsTf gene, a phylogenetic tree was constructed with the MEGA4 programme using human lactotransferrin as an outgroup. The phylogenetic tree was divided into two main clades. One clade included Tf from mammals and the other from amphibians and fish. In the second clade, the amphibian Tf formed a separate cluster, and fish Tf formed a large cluster. In the latter, the fish in the same order formed a sub-cluster, e.g., fish (grass carp, common carp and Japanese crucian carp) in cyprinids were clustered together. As expected, CsTf was included within the sub-cluster of Pleuronectiformes (Japanese flounder) with a high bootstrap value ().
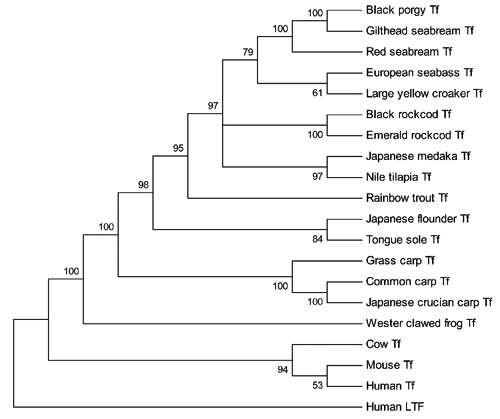
Structure of the tongue sole transferrin
The two-dimensional structure analysis showed that tongue sole Tf possessed an α-helix/β-sheet structure (a). Further, the 3-D structure of tongue sole Tf was constructed in the SWISS-MODEL server using the comparative modelling method. The results showed that CsTf was divided into a bilobal structure with an amino-terminal lobe (N-lobe, residues 23–326) and a carboxyl-terminal lobe (C-lobe, residues 337–656). The lobes were connected by a linker peptide (residues 327–336). Each lobe was further divided into two sub-domains containing a multi-α-helix/β-sheet structure (b).
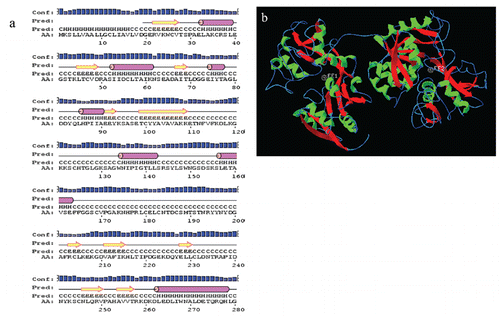
Tissue distribution of CsTf
The tissue distribution of CsTf mRNA was determined using real-time PCR. As shown in , the expression of CsTf was relatively high in the liver. Moderate levels of CsTf expression were detected in the heart, spleen and kidney, with the lowest levels being in the stomach, blood, gills and intestine.
CsTf expression profile under acute hypoxic stress
The expression change in CsTf under acute hypoxic stress (DO=0.8 mg/L) was detected using real-time PCR. Under hypoxic stress, CsTf expression exerted a tissue-specific expression pattern. For example, Tf in the heart and liver was increased from 30 min to 120 min under hypoxic stress (P<0.05), while Tf in the spleen was increased from 60 min to 120 min under hypoxic stress compared with that in the control group (P<0.05). As the hypoxic time was prolonged from 90 min to 120 min, Tf in the blood, gills and stomach returned to normal expression levels, similar to those in the control group (P>0.05; ).
Discussion
In the present study, the tongue sole Tf gene was cloned, characterised, and its expression under hypoxic stress was examined as well. Our study confirmed that tongue sole exactly possesses Tf gene homology. In addition, tongue sole Tf was up-regulated in different tissues after hypoxic stress, indicating that this gene plays a role in fisH’s response to hypoxia.
We found that the tongue sole Tf gene was 2281 bp in length, and encoded 677 aa, which shared similar aa lengths with Tfs in other vertebrates (615–695 aa). Also, the full sequence of tongue sole Tf shares a high identity with Tf in other vertebrates. Some important aa, e.g., four iron-binding sites in each lobe and two sites of anions of each lobe, remained unchanged during evolution (). The CsTf possessed the same 2-D and 3-D structure as human Tf, thus implying again that the structure of Tf remained conserved during evolution. The N-lobe and C-lobe also shared higher sequence identities with their counterparts from different species. It is speculated that Tf might have evolved from a common ancestor gene during evolution. The analysis of the sequence identity of the N-lobe and C-lobe showed that the N-lobe was more conserved than the C-lobe in different species. This suggested that the sequence coding of the primordial N-lobe might be the ancestor of Tf.
The expression of Tf has been studied in several fishes and appears to be tissue-specific in different species. The Atlantic salmon Tf expression is found at high levels in the liver, but at lower levels in the kidney and stomach. Transferrin of the Atlantic cod and roughskin sculpin is highly expressed in the liver and brain, but less expressed in other tissues (CitationDenovan-Wright et al., 1996; CitationLiu et al., 2012). Our study found that CsTf was highly expressed in the liver and moderately expressed in the heart, spleen and kidney, which is consistent with the expression pattern of the Atlantic salmon (CitationKvingedal, 1994). The high expression of Tf in the liver suggests that the fish liver is the main tissue that synthesises and secretes Tf. The reason for varying expression patterns in other tissues (e.g., the brain and spleen) needs to be further investigated by focusing on the regulation pathway of Tf in closely related species.
Aquatic hypoxia affects fish directly since it jeopardises their habitat. However, research on fisH’s response to hypoxia has only recently begun. CitationWulff et al. (2012) found that more than 40 proteins involved in maintaining iron homeostasis, energy levels and muscle structure are down- or up-regulated in rainbow trout under hypoxic stress, thus suggesting that fisH’s response to hypoxia is a complex process.
Previous studies indicated that Tf might play a role under hypoxic conditions (CitationSimpson, 1992; CitationRolfs et al., 1997). Still, little is known about hypoxia in fish. Therefore, we selected the marine fish tongue sole - which possesses a specific hypoxic adaptation strategy - as an animal model in order to detect the expression pattern of Tf under hypoxia. Our previous studies found that some genes (e.g., globins) might play a role at different stages of hypoxia (CitationWang et al., 2011a, Citation2011b). In the present study, we found that tongue sole Tf was up-regulated in some tissues (e.g., the liver and heart) under acute hypoxia (DO=0.8 mg/L). However, as hypoxia became prolonged, Tf returned to normal levels to save energy for maintaining the physiological function of important tissues (liver and heart).
Conclusions
In this paper, the Tf sequence from the marine fish tongue sole was cloned and analysed for the first time. Tongue sole Tf possesses the same structure as mammalian Tf with the N-lobe and C-lobe. Some aa sites are conserved in tongue sole Tf. Moreover, tongue sole Tf is mainly expressed in the liver and up-regulated under hypoxic stress, thus indicating that Tf also plays a role in fisH’s adaptation to hypoxia.
Acknowledgments:
this work was financed by the Project from the Natural Science Foundation of Jiangsu Province (BK2011418), the Natural Science Foundation of the Jiangsu Higher Education Institutions of China (10KJB24001), and the Foundation for Talent Recruitment of Yancheng Institute of Technology (XKR2011007).
References
- AndersonB.F. BakerH.M. NorrisG.E. RiceD.W. BakerE.N. 1989 Structure of human lactoferrin-crystallographic structure analysis and refinement at 2.8A resolution J. Mol. Biol 209 711 734
- BendtsenJ.D. NielsenH. HeijneG.V. BrunakS. 2004 Improved prediction of signal peptides: SignalP 3.0 J. Mol. Biol 340 783 795
- ClewleyJ.P. ArnoldC. 1997 MEGALIGN. The multiple alignment module of LASER-GENE Methods Mol. Biol 70 119 129
- De SmetH. BlustR. MoensL. 2001 Cadmium-binding to transferrin in the plasma of the common carp Cyprinus carpio Comp. Biochem. Physiol 128 45 53
- Denovan-WrightE.M. RamseyN.B. McCormickC.J. LazierC.B. WrightJ.M. 1996 Nucleotide sequence of transferrin cDNAs and tissue-specific expression of the transferrin gene in Atlantic cod (Gadus morhua) Comp. Biochem. Phys. B 113 269 273
- FordM.J. 2012 Molecular evolution of transferrin: evidence for positive selection in salmonids Mol. Biol. Evol 18 639 647
- JureckaP. IrnazarowI. StaffordJ.L. RuszczykA. TaverneN. BelosevicM. SavelkoulH.F. WiegertjesG.F. 2009 The induction of nitric oxide response of carp macrophages by transferrin is influenced by the allelic diversity of the molecule Fish Shellfish Immun 26 632 638
- KurowskiM.A. BujnickiJ.M. 2003 GeneSilico protein structure prediction meta-server Nucleic Acids Res 31 3305 3307
- KvingedalA.M. 1994 Characterization of the 5' region of the Atlantic salmon (Salmo salar) transferrin-encoding gene Gene 150 335 339
- LaskoswkiR.A. MacArthurM.W. MossD.S. ThorntonJ.M. 1993 PROCHECK: a program to check the stereo chemical quality of protein structures J. Appl. Crystal 26 283 291
- LiuY.Y. YuS.S. ChaiY.M. ZhuQ. 2012 Transferrin gene expression in response to LPS challenge and heavy metal exposure in roughskin sculpin (Trachidermus fasciatus) Fish Shellfish Immun 32 223 229
- NicholasK.B. NicholasH.B.Jr. DeerfieldD.W.II 1997 GeneDoc: analysis and visualization of genetic variation EMBnet.news 4 1 4 Available from: http://journal.embnet.org/index.php/emb-netnews/article/view/115/140
- RolfsA. KvietikovaI. GassmannM. WengerR.H. 1997 Oxygen-regulated transferrin is mediated by hypoxia-inducible factor-1 J. Biol. Chem 272 20055 20062
- SahooP.K. MohantyB.R. KumariJ. BaratA. SarangiN. 2009 Cloning, nucleotide sequence and phylogenetic analyses, and tissue-specific expression of the transferrin gene in Cirrhinus mrigala infected with Aeromonas hydrophila Comp. Immunol. Microb 32 527 537
- ShaoC.W. ChenS.L. ScheuringC.F. XuJ.Y. ShaZ.X. DongX.L. ZhangH.B. 2010 Construction of two BAC libraries from half-smooth tongue sole Cynoglossus semilaevis and identification of clones containing candidate sex-determination genes Mar. Biotechnol 12 558 568
- SimpsonR.J. 1992 Effect of hypoxic exposure on iron absorption in heterozygous hypo-transferrinaemic mice Ann. Hematol 65 260 264
- StaffordJ.L. NeumannN.F. BelosevicM. 2001 Products of proteolytic cleavage of transferrin induce nitric oxide response of goldfish macrophages Dev. Comp. Immunol 25 101 115
- TangeN. JongY.L. MikawaN. HironoI. AokiT. 1997 Cloning and characterization of transferrin cDNA and rapid detection of transferrin gene polymorphism in rainbow trout (Oncorhynchus mykiss) Mol. Mar. Biol. Biotech 6 351 356
- WallnerB. ElofssonA. 2005 Pcons5: combining consensus, structural evaluation and fold recognition scores Bioinformatics 21 4248 4254
- WangZ.S. QiZ.T. QiuM. ZhaoW.H. WangA.M. HuangJ.T. GuoX.J. 2011a Changes of globins expression in tongue sole, Cynoglossus semilaevis (Actinopterygii: Pleuronectiformes: Cynoglossidae) in response to short-term hypoxia Acta Ichthyol. Piscat 41 179 184
- WangZ.S. QiZ.T. TianJ.Y. QiuM. ZhaoW.H. WangA.M. HuangJ.T. GuoX.J. 2011b Cloning of hemoglobin-α1 from half smooth tongue sole, (Cynoglossus semilaevis) and its expression under short-term hypoxia Zool. Res 32 641 646
- WawrowskiA. GerlachF. HankelnT. BurmesterT. 2011 Changes of globin expression in the Japanese medaka (Oryzias latipes) in response to acute and chronic hypoxia J. Comp. Physiol. B 181 199 208
- WengerR.H. RolfsA. MartiH.H. BauerC. GassmannM. 1995 Hypoxia, a novel inducer of acute phase gene expression in a human hepatoma cell line J. Biol. Chem 270 27865 27870
- WulffT. JokumsenA. HøjrupP. JessenF. 2012 Time-dependent changes in protein expression in rainbow trout muscle following hypoxia J. Proteomics 75 2342 2351