Abstract
The effect of harvesting time and cooking on water, protein, lipid, ash and fatty acid content of farmed meagre was assessed. Significant differences in nutrient content of raw fillets were detected in relation to harvesting time. Cooking by boiling induced loss of some macronutrients, mainly lipids and some fatty acids. Retention of total lipids, C18:2n-6 and C18:3n-3 decreased significantly with harvesting time, while C20:5n-3 (EPA) and C22:6n-3 (DHA) were retained in the same quantity. DHA retention was higher than that of the other FAs considered, at all harvesting times. The changes detected did not diminish the nutritional value of the fish. Despite losses induced by cooking and the low fat content, typical of this species, 100 g of fillet ensured an intake of EPA plus DHA more than double the recommended daily intake (250 mg day–1), at all harvesting times. The ability to preserve nutrients is an essential requirement for quality maintenance and suggests the possibility of heat-processing fillets.
Introduction
Meagre (Argyrosomus regius, Asso 1801) is an emerging farm-raised species in the European seafood market, not yet well known to consumers (Monfort, Citation2010). Fast growth (Quéméner, Citation2002), good feed conversion, high adaptation capacities and resilience to stress are major aspects that make meagre an excellent candidate for large-scale fish farming in Europe (Monfort, Citation2010). The farming of this species is also important for diversifying commercial aquaculture in the Mediterranean and Eastern Atlantic areas.
Besides good farming aptitude, the increasing interest in meagre is attributed to its promising market and quality traits, such as attractive shape, good processing yield, high nutritional value, and excellent taste (Monfort, Citation2010). Even large specimens have a low lipid content, which is a valuable attribute for extending seafood shelf life (Poli et al., Citation2003). Moreover, the lipids have a good fatty acid (FA) profile, rich in long-chain omega-3 polyunsaturated FAs (n-3 LC-PUFAs), mainly EPA and DHA (Poli et al., Citation2003; Piccolo et al., Citation2008; Hernandez et al., Citation2009; Grigorakis et al., Citation2011), which have recognised beneficial effects on human health (antithrombogenic and antiatherogenic activity) (Burr et al., Citation1989; Lauritzen et al., Citation2001; Kris-Etherton et al., Citation2002; Marchioli et al., Citation2002; Buttriss and Nugent, Citation2005; Serhan, Citation2007). Farmed meagre is mainly sold fresh (Monfort, Citation2010) and prepared for domestic and catering purposes. Previous research has shown that cooking affects nutritional value of fish, causing nutrient concentration or loss in relation to cooking method. In several cases, cooking methods either induced lipid loss and oxidation (Al-Saghir et al., Citation2004; Rodriguez et al., Citation2008) or did not cause any change (Al-Saghir et al., Citation2004; Rodriguez et al., Citation2008; Erkan et al., Citation2010). In particular, boiling was associated with higher retention of lipids (Weber et al., Citation2008; Larsen et al., Citation2010). Regarding FAs, Türkkan et al. (Citation2008) and Larsen et al. (Citation2010) found considerable loss of DHA and EPA after cooking, while other studies reported that the FA profile was not significantly altered (Al-Saghir et al., Citation2004; de Castro et al., Citation2007; Rodriguez et al., Citation2008; Weber et al., Citation2008; Erkan et al., Citation2010).
In light of the present market situation, most research is conducted to expand the potential market base for meagre by investigating fillet nutritional peculiarities and aptitude for processing. There has been relatively little research into the nutritional value or macronutrient content and FA profile of farmed meagre (Poli et al., Citation2003; Hernandez et al., Citation2009; Grigorakis et al., Citation2011) compared to that addressed to other aquaculture species, and no data is available on cooked fillets. Because farmed meagre has very lean flesh, retention of lipids and n-3 FAs during cooking would enhance its quality and provide further information on its nutritional profile.
The aim of this study was to describe the effects of cooking by boiling on the proximate composition, lipid content and FA profile of fillets of farmed meagre raised in a tank and harvested at different times during the winter-spring period.
Materials and methods
Fish were obtained from Il Vigneto, a fish farm near Ansedonia (Grosseto, Italy -42°25’45.35”N and 11°16’58.85”E). Meagre were raised in a circular, outdoor, land-based tank (500 m3) at a constant density of about 50 kg/m3. Water temperature during farming ranged from 19°C to 22°C (geothermal water) independently to season, and salinity was approximately 16 ppt. The fish were reared under natural photoperiod conditions, and fed 3-4 times per day with commercial extruded feed, the proximate composition and FA profile of which are shown in .
Groups of 18 fish were harvested three times (26th January, 3rd March and 5th May; total 54 fish). Average weight was 994.07±262.41 g. Harvesting took place in the winter-spring period, when metabolic changes are more evident, one reason being the increase in food intake due to changes in environmental parameters, especially photoperiod. The fish reached the Dipartimento di Scienze delle Produzioni Agroalimentari e dell’Ambien -te in Firenze (Italy) the day after harvesting. Whole fish weight (W) and total length (TL) were recorded. After dissection and filleting, the head, axial skeletal bones, viscera, liver, gonads (when evident and separable), perivisceral fat (fat stored inside the peritoneal cavity) and right and left fillets were weighed. The condition factor (CF) was determined using the following formula CF = 100 x W (g)/TL3 (cm). Visceral fat index (VFI), viscerosomatic index (VSI), hepatosomatic index (HSI) and gonadosomatic index (GSI) were calculated as perivisceral fat, viscera, liver and gonad percentages of total body weight, respectively. Dressing yield and fillet yield were calculated as 100 x [W (g) – visceral weight (g)/W (g)] and 100 x [weight of fillets (g)/W (g)], respectively.
Left fillets were analysed raw, while right fillets were wrapped in aluminium foil, placed on a tray, immersed in water in a fish-steamer, boiled at 98-100°C for 10 min, cooled at room temperature and weighed. The cooking yield was calculated as 100 x [cooked fillet weight (g)/raw fillet weight (g)].
The determinations of fillet ash, protein, fat and total lipids content were performed on fillets without skin, homogenised, and freeze-dried prior to analysis. FA profiles were determined on lipid extract. All the analyses were carried out both on raw and cooked fillets.
Proximate analysis and total lipids
Moisture, crude protein (Nx6.25) and ash content were determined according to AOAC (Citation2000) 950.46, 976.05, and 920.153 methods, respectively. Total lipid extraction was performed according to a modification of the method of Folch et al. (Citation1956). Freeze-dried samples, reconstituted fresh by adding distilled water, were homogenised with a 2:1 chloroform-methanol (v/v) solution and filtered. The filter was washed several times, and distilled water with 0.88% KCl was added to the filtrate so that the [Choloroform: Methanol]:water ratio was 4:1. After stirring and standing overnight, a biphasic system was obtained. The lower phase containing lipids dissolved in chloroform was siphoned off and recovered. Total lipid content was determined gravimetrically after vacuum evaporation of the chloroform by lipid resuspension in a known volume of chloroform (5 mL). Lipid content was weighed in a crucible (gross weight minus tare) after complete evaporation of chloroform.
Determination of fatty acid composition
FAME (Fatty Acid Methyl Ester) analysis was performed according to a modified method of Morrison and Smith (Citation1964). Lipids were saponified with 0.5 M KOH in methanol, and FAs were hydrolysed by adding 2 N HCl. Methyl esters were prepared by transmethylation using boron fluoride-methanol at 14% concentration. Methylated FAs were dissolved in petroleum ether, dried, and finally resuspended in 1 mL hexane.
FA composition was determined by liquid gas chromatography (LGC). A GC Varian 430 gas chromatograph (Varian Inc., Palo Alto, CA, USA) equipped with flame ionization detector (FID) and a Supelco Omegawax™ 320 capillary column (30 m x 0.32 mm i.d., 0.25 μm film and polyethylene glycol bonded phase; Supelco, Bellefonte, PA, USA) was used. The oven temperature was held at 100°C for 2 min, increased to 160°C over 4 minutes at a rate of 12°C/min, increased to 220°C over 14 min at the rate of 3°C/min, and kept at 220°C for 25 min. Injector and detector temperatures were set at 220°C and 300°C, respectively. One microlitre of sample in hexane was injected into the column with the carrier gas (helium) at a constant flow of 1.5 mL/min. The split ratio was 1:20.
Chromatograms were recorded with computing integrator software (Galaxie Chromatography Data System 1.9.302.952; Varian Inc.). FAs were identified by comparing the retention time of FAME with the standard Supelco 37 component FAME mix (Supelco). FAs were quantified through calibration curves, using tricosanoic acid (C23:0) (Supelco) as internal standard.
Computation of true retention
True retention is the proportion of a nutrient remaining in the cooked food in relation to the amount originally present in a given weight of the food before cooking (Murphy et al., Citation1975). True retention (TR%) of nutrients was calculated according to Murphy et al. (Citation1975), as follows:
TR% = 100 x (nutrient content per g of cooked food × g of food after cooking)/(nutrient content per g of raw food × g of food before cooking).
Computation of fat quality indexes
The following fat quality indexes were calculated on raw and cooked fillets to assess variations induced by cooking:
- | n-6/n-3 ratio; | ||||
- | LA/ALA ratio [linoleic acid (LA; C18:2n-6)/alpha-linolenic acid (ALA; C18:3n-3)]; | ||||
- | atherogenic index (AI) according to the formula [C12:0 + (4 × C14:0) + C16:0]/(ΣPUFA n-3 + ΣPUFA n-6 + ΣMUFA) (Ulbricht and Southgate, Citation1991); | ||||
- | thrombogenic index (TI) according to the formula [C14:0 + C16:0 + C18:0]/[0.5 × ΣMUFA) + (0.5 × ΣPUFA n-6) + (3 × ΣPUFA n-3) + (ΣPUFA n-3/ΣPUFA n-6)] (Ulbricht and Southgate, Citation1991); | ||||
- | Hypocholesterolaemic/hypercholesterolaemic FA ratio (HH), according to the formula (C18:1n-9 + C18:2n-6 + C20:4n-6 + C18:3n-3 + C20:5n-3 + C22:5n-3 + C22:6n-3)/(C14:0 + C16:0) (Santos-Silva et al., Citation2002). |
Statistical analysis
Data of raw and cooked fillets was analysed separately using ANOVA with the GLM (General Linear Model) procedure of SAS® (Citation2007), using harvesting month as the fixed factor. For the fat quality indexes, the condition effect (raw or cooked) and its interaction with harvesting month were added to the model. Differences between least squares means were tested by Student’s t- test; P values <0.05 were considered significant.
Results and discussion
Morphometric and somatometric traits
The morphometric and somatometric characteristics of the fish are presented in . Differences were detected in relation to harvesting times: fish sampled in January and March had lower weight, length, VFI, VSI and head incidence (%) than fish sampled in May, and conversely showed higher dressing and fillet yields. An increasing trend in GSI (P<0.05) was evident from January to May, whereas HSI was higher in January than in March and May.
Since the rearing water was obtained from geothermal sources and its temperature was not subject to seasonal fluctuations, variability in fish somatometric indexes can mainly be attributed to fish size and seasonal changes in environmental parameters (i.e. photoperiod). Fat deposition is positively related to fish size and age(Lanari et al., Citation1999; Fontagné-Dicharry and Médale, Citation2010), and is also affected by season, which justifies the highest VFI in May, when fish were larger. Moreover, the increasing trend of GSI cannot be explained by considering that summer is the natural reproduction period of wild meagre (Quéro and Vayne, Citation1987), since reproduction rarely occurs in specimens of the size considered in this trial. Finally, the HSI was significantly higher in January and subsequently decreased. Herland et al. (Citation2010) and Piñon et al. (Citation2009) found a reduction in HSI in the reproduction phase in other species of fish, indicating allocation of energy resources to gonad production. This explanation is not compatible with our findings as concerns fish size and GSI values, which were very different from the values shown by meagre close to maturation. GSI is known to approach or exceed 1% in mature meagre (Schiavone et al., Citation2012).
In this study, VSI results were in the same range of farmed sharpsnout seabream analyzed by Piccolo et al. (Citation2007) but lower than those observed in farmed meagre of similar weight (Poli et al., Citation2003) and in other commercially valuable Mediterranean species, such as farmed sea bass (Dicentrarchus labrax) (Poli et al., Citation2001) and farmed and wild sea bream (Sparus aurata) of standard commercial size (300-400 g) (Yildiz et al., Citation2008). Conversely, the VFI was similar to those found by Poli et al. (Citation2003) and Piccolo et al. (Citation2008) in the same species, whereas it was much lower than in sea bass (Grigorakis et al., Citation2004) and sea bream (Grigorakis et al., Citation2002). By virtue of its negligible amount of offal, this farmed meagre showed particularly high dressing and fillet yields in all harvesting periods and, when compared to meagre of similar body weight analysed in previous research (Poli et al., Citation2003; Grigorakis et al., Citation2011), it showed higher fillet yields. Unfortunately, in our study, larger fish showed higher head incidence, which is a limiting market trait of meagre (Poli et al., Citation2003; Monfort, Citation2010).
Composition of raw fillets
Differences in proximate composition of meagre raw fillets in relation to harvesting time are reported in . Like somatometric traits, chemical composition varied during the study period. Lipid content increased with fish size, in line with the finding of Shearer (Citation1994). However, the fish had low muscle lipid content at all harvesting times. The limited lipid deposition in muscle observed in meagre is supported by previous findings in juveniles (Chatzifotis et al., Citation2010) and adults (Poli et al., Citation2003; Piccolo et al., Citation2008; Hernandez et al., Citation2009; Grigorakis et al., Citation2011), thus differentiating meagre from other commonly consumed Mediterranean farmed species (i.e. sea bass and sea bream) whose lipid content is generally higher (Grigorakis et al., Citation2002; Yildiz et al., Citation2008). In particular, farmed meagre of 800-1200 g showed a similar lipid content to wild sea bream of 250-450 g caught in January and May, when nutrient availability in the marine environment is less than in summer (Grigorakis et al., Citation2011).
Fatty acid profile of raw fillets
A dependence of body FA composition on dietary FAs has been reported in previous studies on meagre (Poli et al., Citation2003; Piccolo et al., Citation2008; Grigorakis et al., Citation2011) and several other farmed species, including sea bream (Grigorakis et al., Citation2002; Senso et al., Citation2007; Yildiz et al., Citation2008; Cardinal et al., Citation2011) and sea bass (Yildiz et al., Citation2008). The feed used in this trial (), which was partially of plant origin, did not have a substantial impact on the nutritional value of the fillet because the farmed meagre expressed a high quality FA profile in all samples.
Total saturated FAs (ΣSFAs) were found in similar proportions at all harvesting times, despite a moderately decreasing trend (P<0.05) from January to May for C16:0, C18:0 and ΣSFAs (). As found in previous research on the same species (Poli et al., Citation2003; Piccolo et al., Citation2008; Grigorakis et al., Citation2011), palmitic acid (C16:0) was the most abundant SFA in fillets. However, myristic acid (C14:0) and stearic acid (C18:0) were also found in sizeable amounts. ΣMUFAs were found in increasing percentages from January to May following the trend of intramuscular fat content () and C16:1n-7, C18:1n-9, C18:1n-7, C20:1n-9 and C22:1n-11 were the major MUFAs detected in total lipids as previously reported by Grigorakis et al. (Citation2011). Oleic acid (C18:1n-9), derived from both aquatic and plant components of the diet, was the most abundant.
In previous research on the same species (Poli et al., Citation2003; Piccolo et al., Citation2008; Hernandez et al., Citation2009; Grigorakis et al., Citation2011), ΣPUFAs were the most abundant FAs at all harvesting times, and n-3 FAs were three times more abundant than n-6 FAs. LA was the most abundant n-6 FA in fillets, while marine-derived EPA and DHA were the main FAs of the n-3 series. The latter FAs were derived exclusively from dietary fish oil, as marine carnivorous fish are known to endogenously biosynthesize very little LC-PUFAs (Tocher, Citation2003; Fontagné-Dicharry and Médale, Citation2010). Fish sampled in January registered the highest percentage of ΣPUFAn-3, while those sampled in May had the lowest. Conversion of EPA to DHA is limited in marine carnivorous species and presumably did not regard the large quantity of DHA found at all harvesting times. The high proportion of DHA at all harvesting times could be the result of low fat deposition, and consequently high polar lipid content, which includes mainly DHA (Grigorakis et al., Citation2011). A similar inverse relationship between low fillet fat and DHA has already been reported in farmed meagre (Poli et al., Citation2003), wild sea bream (Grigorakis et al., Citation2002) and farmed sea bream (Senso et al., Citation2007).
Composition of cooked fillets
Cooking yield (%) did not differ between harvesting times (89.3 vs 89.4 vs 90.6 in January, March and May, respectively). As shown in , differences in moisture, protein and lipid content in raw fillets, in relation to harvesting time, were nullified by cooking. Moisture loss and protein concentration after cooking were in line with results (expressed on a wet basis) obtained for boiled king salmon (Oncorhynchus tshawytscha) (Larsen et al., Citation2010) and boiled silver catfish (Rhamdia quelen) (Weber et al., Citation2008).
The FA profile of cooked fillets generally followed the same trends as those of raw fillets, with some exceptions (). Cooking nullified harvesting-time related differences in C18:0, ΣSFAs, C20:4n-6, C22:5n-6, ΣPUFAn-6, EPA and DHA.
True retention
TR% provides a good estimate of variations in nutrients after cooking. As shown in , protein TR% was 100% at all harvesting times, demonstrating that protein was completely retained. Moisture, ash and lipids were not totally retained, but harvesting-time-related differences were only found for total lipids. Major lipid losses were due more to intramuscular lipid deposits, which are mainly triglycerides and subject to melting and leaching, than to structural lipids (Pirini et al., Citation2010). Significantly higher lipid losses (P<0.05) were therefore found in lipid-rich meagre sampled in May. A similar inverse relationship between lipid retention and intramuscular lipid content was demonstrated by Pirini et al. (Citation2010) in oven-baked wild anchovy (Engraulis encrasicholus), sardine (Sardina pilchardus), horse mackerel (Sprattus sprattus) and sprat (Trachurus trachurus). FA TR% was calculated on FAs found at concentrations above 4% by total FAME, except in the case of ALA, which was analysed because of its nutritional importance.
These FAs were not completely retained at all harvesting times (), as suggested by total lipid TR%. As shown by the retention in May-sampled fish, the lipid content of fillets and FA retention indicated an inverse relationship in line with the previous findings of Pirini et al. (Citation2010) in the wild species cited above. Fish sampled in May generally had lower FA retention than other samples, but only LA (C18:2n-6) and ALA (C18:3n-3) retention differed significantly from the January and March samples. The pronounced decrease recorded in May could be the result of differential losses in polar and neutral lipids induced by cooking. As previously reported, lipids leaching out during cooking mainly belong to fat deposits largely composed of neutral lipids (Nanton et al., Citation2007). Because meagre neutral lipids are known to be rich in C18:1n-9, LA and ALA (Grigorakis et al., Citation2011), more of these three FAs may have been released after cooking in lipid-rich-May samples. The higher TR% expressed by DHA was most likely due to the preponderance of DHA in polar lipids, enhancing the retention of this FA. DHA retention is a positive characteristic of this species. DHA has well-known cardioprotective effects and lowers blood pressure, heart rate and platelet aggregation, more than does EPA, in the latter case (Cottin et al., Citation2011).
Fat indexes
The most useful indexes of fat quality and how they were influenced by harvesting times and cooking are reported in .
In all samples, the n-6/n-3 PUFA ratio was less than 1 due to the abundance of EPA and DHA. This ratio was similar to those of Grigorakis et al. (Citation2011) and Poli et al. (Citation2003) for the same species, and was also in the same range as values found for cultured sea bream (Grigorakis et al., Citation2002; Senso et al., Citation2007; Yildiz et al., Citation2008) and cultured sea bass (Alasalvar et al., Citation2002; Yildiz et al., Citation2008).
Since n-6 and n-3 PUFAs compete for the same enzymes in the endogenous elongation/desaturation process and have different biological roles (Kris-Etherton et al., Citation2002; Simopoulos, Citation2008), the balance between them has considerable importance in the human diet (FAO, Citation1994). A diet rich in n-6 FAs is prothrombotic and proaggregatory, promoting blood viscosity, vasospasm, vasoconstriction and shorter bleeding time (Simopoulos, Citation1999). The recommended balance of n-6/n-3 PUFA suggested by several authors (Budowski and Crawford, Citation1985; Nestel, Citation1987; Simopoulos, Citation1999, Citation2008; Russo, Citation2009;) for preventing cardiovascular, inflammatory, and autoimmune diseases and cancer should therefore be below 5:1. The LA/ALA ratio was in the recommended range for human intake, which should be between 5:1 and 10:1 (FAO, Citation1994) because various studies demonstrate that a ratio below 10:1 increased EPA content in plasma phospholipids (Liou et al., Citation2007), decreased total mortality of patients (De Lorgeril et al., Citation1994), and reduced the incidence of non-insulin-dependent diabetes mellitus (Raheja et al., Citation1993). Although the LA/ALA ratio was within the recommended range in all samples, the results were particularly high because of the abundance of LA and the low proportion of ALA in the fillets, which reflected the FA profile of the fish feed. The lowest LA/ALA ratio was found in May, when ALA content was significantly higher than at the other harvesting times. Atherogenic (AI) and thrombogenic (TI) indexes were very low at all harvesting times. The very low TI of meagre was due to the abundance of EPA and DHA, whose pronounced antithrombogenic activity is ascribed to their strong platelet-aggregation-inhibiting effect via prostanoid PGI3 production (Ulbricht and Southgate, Citation1991). The results of this trial were similar to those found by Poli et al. (Citation2003) but lower than those reported by Grigorakis et al. (Citation2011) and Piccolo et al. (Citation2008). Meagre showed a similar TI compared with sea bream (Senso et al., Citation2007; Hurtado-Rodriguez et al., Citation2010) but a lower TI than that of sea bass (Poli et al., Citation2001). The AI found in our research was higher than that reported by Grigorakis et al. (Citation2011) but lower than that found by Poli et al. (Citation2003) in meagre fillets. Additionally, the AI was similar to that recorded in sea bass (Poli et al., Citation2001), but higher than that found in sea bream (Senso et al., Citation2007; Hurtado-Rodriguez et al., 2011). The hypocho-lesterolaemic/hypercholesterolaemic FA ratio (HH) was always >2, which is higher than that reported for seven Brazilian freshwater fish species (Filho et al., Citation2010), but lower than that found in white hake (Sancho et al., Citation2011). No significant differences were found with respect to harvesting time. Cooking did not affect the n-6/n-3 PUFA ratio, confirming findings in boiled king salmon (Larsen et al., Citation2010) and silver catfish (Weber et al., Citation2008). Although LA and ALA were not totally retained, the higher proportion of LA and the lower proportion of ALA detected after cooking induced a significant increase in the LA/ALA ratio. TI was not significantly modified by cooking, whereas AI significantly decreased. Unlike the results found for cooked white hake (Sancho et al., Citation2011), the HH ratio of meagre was significantly improved by cooking.
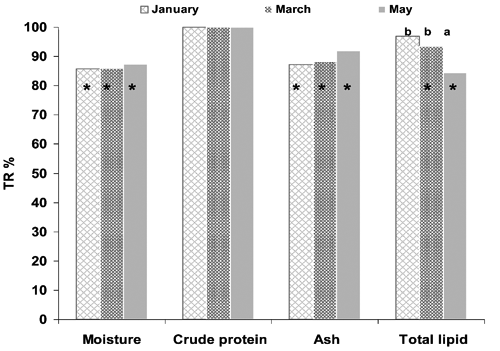
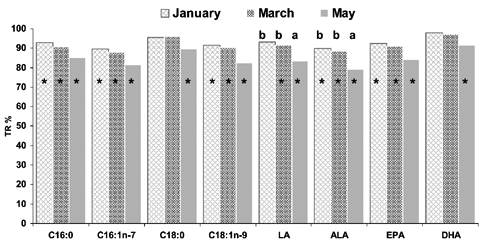
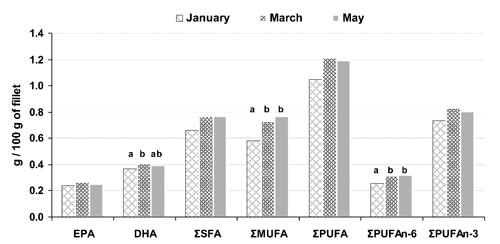
Table 1. Proximate composition and fatty acid profile of the fish diet.
Table 2. Morphometric and somatometric measurements of meagre at different harvesting times.
Table 3. Moisture (g 100 g–1), protein, ash, total lipids (g 100 g–1, wet basis), and fatty acid profile of raw meagre fillets in relation to harvesting time.
Table 4. Moisture (g 100 g–1), protein, ash, total lipids (g 100 g–1, wet basis), and fatty acid profile of cooked meagre fillets in relation to harvesting time.
Table 5. Fat quality indexes of meagre fillets at different harvesting times and before and after cooking with the interaction harvesting time x condition.
Intake of fatty acids from cooked meagre
Cooked farmed meagre was evaluated for FA content based on an average portion for adults of 100 g. As presented in , ΣSFA intake ranged from ˜650 mg in January to ˜770 mg in May. ΣMUFA intake significantly differed among harvesting times, and the highest value (˜770 mg) was found in May, despite low retention. As ΣSFA and ΣMUFA are synthesised by the body and are not essential dietary nutrients, no daily intake has been set by nutritionists.
For all harvesting periods, ΣPUFA intake exceeded 1 g, with a clear preponderance of ΣPUFAn-3. No recommended intakes have been set by EFSA (Citation2010) for ΣPUFA or for the essential FAs LA and ALA because the scientific data available is insufficient to derive an Average Requirement, a Lower Threshold Intake or a Population Reference Intake. However, the abundance of ΣPUFAs, mainly ΣPUFAn-3, are a major nutritional feature of meagre, since nutritionists generally recommend replacing ΣSFA with ΣPUFA in order to decrease the risk of coronary heart disease. A recommended intake (in grams per day) has only been suggested for EPA plus DHA, with insufficient evidence to set a specific minimum intake of either EPA or DHA alone. According to the EFSA (Citation2010), an intake of 250 mg per day of EPA plus DHA appears to be sufficient for primary prevention of cardiovascular diseases, while the FAO/WHO (Citation2008) sets intake at 300 mg/day for healthy adults with at least 200 mg of DHA for pregnant and lactating women. In the present study, a 100 g portion of meagre fillet provided much more EPA plus DHA than the recommended intake. Similar amounts of EPA were found in January, March and May-harvested fish (239, 264 and 252 mg/100 g, respectively). DHA intake was higher than that of EPA and followed a similar trend, with the lowest amount being 365 mg in January. Even if the leaner meagre sampled in January provided fillets with the lowest amount of EPA plus DHA per portion, they retained these FAs better than fatter fish sampled in March and May. This suggests that consumption of approximately two 100 g servings per week of farmed meagre (<2% lipid content) would meet EPA plus DHA dietary requirement.
Conclusions
The results of this study indicate that farmed meagre is a very lean species with a high-quality lipid profile, rich in ΣPUFA, especially ΣPUFAn-3. Although nutritional composition varied with harvesting time, the differences were less after cooking.
Cooking by boiling led to loss of some macronutrients, mainly lipids, but DHA retention was higher than retention of the other FAs considered. Despite the losses induced by cooking and low fat content, the 100 g portion of fillet taken as reference would ensure an intake of EPA and DHA more than double the recommended daily intake for EPA plus DHA (250 mg day–1) suggested by EFSA, for fish harvested at any time during the study period.
The ability to preserve nutrients is an essential requirement for quality maintenance and is a positive aspect of meagre quality. Slight nutritional losses demonstrate that this species is suitable for sale fresh and suggest that the fillets can be treated by other industrial heat processes.
Acknowledgments
This research was funded by the University of Firenze research funds Ex 60% 2010 and by the University of Padova research funds Ex 60% 2010 (60A08-0425/10).
The authors would like to thank Josè Peralta, Giulia Tasoniero and Alberto Sartori for their help. Thanks are also due to Mr. Guidi, owner of Il Vigneto farm, for providing the animals.
References
- AlasalvarC. TaylorK.D.A. ZubcovE. ShahidiF. AlexisM. 2002. Differentiation of cultured and wild sea bass (Dicentrarchus labrax): total lipid content, fatty acid and trace mineral composition. Food Chem. 79:145-150.
- Al-SaghirS. ThurnerK. WagnerK. FrischG. LufW. Razzazi-FazeliE. ElmadfaI. 2004. Effects of different cooking procedures on lipid quality and cholesterol oxidation of farmed salmon fish (Salmo salar). J. Agr. Food Chem. 52:5290-5296.
- AOAC, 2000. Official Methods of Analysis, 17th ed. Association of Official Analytical Chemists, Washington, DC, USA.
- BudowskiP. CrawfordM.A. 1985. Alphalinolenic acid as a regulator of the metabolism of arachidonic acid: dietary implications of the ratio, n-6:n-3 fatty acids. P. Nutr. Soc. 44:221-229.
- BurrM.L. FehilyA.M. GilbertJ.F. RogersS. HollidayR.M. SweetnamP.M. ElwoodP.C. DeadmanN.M. 1989. Effects of changes in fat, fish and fibre intakes on death and myocardial reinfarction: diet and reinfarction trial (DART). Lancet 2:757-761.
- ButtrissJ. NugentA. 2005. LIPGENE: an integrated approach to tackling the metabolic syndrome. P. Nutr. Soc. 64:345-347.
- CardinalM. CornetJ. Donnay-MorenoC. GouygouJ.P. BergéP. RochaE. SoaresS. EscorcioC. BorgesP. ValenteL.M.P. 2011. Seasonal variation of physical, chemical and sensory characteristics of sea bream (Sparus aurata) reared under intensive conditions in Southern Europe. Food Control 22:574-585.
- ChatzifotisS. PanagiotidouM. PapaioannouN. PavlidisM. NengasI. MylonasC.C. 2010. Effect of dietary lipid levels on growth, feed utilization, body composition and serum metabolites of meagre (Argyrosomus regius) juveniles. Aquaculture 307:65-70.
- CottinS.C. SandersT.A. HallW.L. 2011. The differential effects of EPA and DHA on cardiovascular risk factors. P. Nutr. Soc. 70:215-231.
- de CastroF.A.F. Pinheiro Sant’AnaH.M. Milagres CamposF. Brunoro CostaN.M. Coelho SilvaM.T. SalaroA.L. Do Carmo Castro FranceschiniS. 2007. Fatty acid composition of three freshwater fishes under different storage and cooking processes. Food Chem. 103:1080-1090.
- De LorgerilM. RenaudS. MamelleN. SalenP. MartinJ. MonjaudI. GuidolletJ. TouboulP. DalyeJ. 1994. Mediterranean alpha-linolenic acid-rich diet in secondary prevention of coronary heart disease. Lancet 343:1454-1459.
- EFSA, 2010. Scientific opinion on dietary reference values for fats, including saturated fatty acids, polyunsaturated fatty acids, monounsaturated fatty acids, trans fatty acids, and cholesterol. EFSA Journal 8:1-5.
- ErkanN. SelçukA. ÖzdenÖ. 2010. Amino acid and vitamin composition of raw and cooked horse mackerel. Food Anal. Method. 3:269-275.
- FAO, 1994. Fats and oils in human nutrition. Available from: http://www.fao.org/docrep/v4700e/v4700e00.HTM
- FAO/WHO, 2008. Interim summary of conclusions and dietary recommendations on total fat and fatty acids. Joint FAO/WHO Expert Consultation on fats and fatty acids in human nutrition. Available from http://www.who.int/nutrition/topics/FFA_int erim_recommendations/en/
- FilhoM.M.R. Lima RamosM.I. Aiko HianeP. Tala de SouzaE.M. 2010. Nutritional value of seven freshwater fish species from the Brazilian Pantanal. J. Am. Oil Chem. Soc. 87:1461-1467.
- FolchJ. LeesM. Sloane-StanleyG.H. 1956. A simple method for the isolation and purification of total lipids from animal tissues. J. Biol. Chem. 226:497-509.
- Fontagné-DicharryS. MédaleF. 2010. Les lipides des poissons d’aquaculture et leurs facteurs de variation. OCL-Ol. Corps Gras Li. 17:209-213.
- GrigorakisK. AlexisM.N. Anthony TaylorK.D. HoleM. 2002. Comparison of wild and cultured gilthead sea bream (Sparus aurata); composition, appearance and seasonal variations. Int. J. Food Sci. Tech. 37:477-484.
- GrigorakisK. AlexisM.N. GialamasI. NikolopoulouD. 2004. Sensory, microbiological, and chemical spoilage of cultured common sea bass (Dicentrarchus labrax) stored in ice: a seasonal differentiation. Eur. Food Res. Technol. 219:584-587.
- GrigorakisK. FountoulakiE. VasilakiA. MittakosI. NathanailidesC. 2011. Lipid quality and filleting yield of reared meagre (Argyrosomus regius). Int. J. Food Sci. Tech. 46:711-716.
- HerlandH. EsaiassenM. CooperM. OlsenR.L. 2010. Quality of farmed Atlantic cod: effects of season and storage. Aquac. Res. 41:1203-1210.
- HernandezM.D. LopezM.B. AlvarezA. FerrandiniE. Garcia GarciaB. GarridoM.D. 2009. Sensory, physical, chemical and microbiological changes in aquacultured meagre (Argyrosomus regius) fillets during ice storage. Food Chem. 114:237-245.
- Hurtado-RodriguezR. FountoulakiE. GrigorakisK. AlexisM. FlosR. 2010. Season and size effects: changes in the quality of gilthead sea bream (Sparus aurata L.). Medit. Mar. Sci. 11:117-131.
- Kris-EthertonP.M. HarrisW.S. AppelL.J. 2002. Fish consumption, fish oil, omega-3 fatty acids, and cardiovascular disease. Circulation 106:2747-2757.
- LanariD. PoliB.M. BallestrazziR. LupiP. D’AgaroE. MecattiM. 1999. The effects of dietary fat and NFE levels on growing European sea bass (Dicentrarchus labrax L.). Growth rate, body and fillet composition, carcass traits and nutrient retention efficiency. Aquaculture 179:351-364.
- LarsenD. QuekS.Y. EyresL. 2010. Effect of cooking method on the fatty acid profile of New Zealand King Salmon (Oncorhynchus tshawytscha). Food Chem. 119:785-790.
- LauritzenL. HansenH.S. JørgensenM.H. MichaelsenK.F. 2001. The essentiality of long chain n-3 fatty acids in relation to development and function of the brain and retina. Prog. Lipid Res. 40:1-94.
- LiouA.Y. KingD.J. ZibrikD. InnisS.I. 2007. Decreasing linoleic acid with constant α-linolenic acid in dietary fats increases (n-3) eicosapentaenoic acid in plasma phospholipids in healthy men. J. Nutr. 137:945-952.
- MarchioliR. BarziF. BombaE. ChieffoC. Di GregorioD. Di MascioR. FranzosiM.G. GeraciE. LevantesiG. MaggioniA.P. MantiniL. MarfisiR.M. MastrogiuseppeG. MininniN. NicolosiG.L. SantiniM. SchweigerC. TavazziL. TognoniG. TucciC. ValagussaF. 2002. Early protection against sudden death by n-3 polyunsaturated fatty acids after myocardial infarction: time-course analysis of the results of the Gruppo Italiano per lo Studio della Sopravvivenza nell’Infarto Miocardico (GISSI)-Prevenzione. Circulation 105:1897-1903.
- MonfortM.C. 2010. Present market situation and prospects of meagre (Argyrosomus regius), as an emerging species in Mediterranean aquaculture. Available from: http://www.fao.org/docrep/013/i1675e/i1675e 00.htm
- MorrisonW.R. SmithL.M. 1964. Preparation of fatty acid methyl esters and dimethylacetals from lipids with born fluoride-methanol. J. Lipid Res. 5:600-608.
- MurphyE.W. CrinerP. GrayB.C. 1975. Comparisons of methods for calculating retentions of nutrients in cooked foods. J. Agr. Food Chem. 23:1153-1157.
- NantonD.A. VegusdalA. RøråA.M.B. RuyterB. BaeverfjordG. TorstensenB.E. 2007. Muscle lipid storage pattern, composition, and adipocyte distribution in different parts of Atlantic salmon (Salmo salar) fed fish oil and vegetable oil. Aquaculture 265:230-243.
- NestelP.J. 1987. Polyunsaturated fatty acids (n-3, n-6). Am. J. Clin. Nutr. 45:1161-1167.
- PiccoloG. BoveraF. De RiuN. MaronoS. SalatiF. CappuccinelliR. MonielloG. 2008. Effect of two different protein/fat ratios of the diet on meagre (Argyrosomus regius) traits. Ital. J. Anim. Sci. 7:363-371.
- PiccoloG. De RiuN. TulliF. CappuccinelliR. MaronoS. MonielloG. 2007. Somatic indexes, chemical-nutritive characteristics and metal content in caught and reared sharpsnout seabream (Diplodus puntazzo). Ital. J. Anim. Sci. 6:351-360.
- PiñonA. AmezcuaF. DuncanN. 2009. Reproductive cycle of female yellow snapper Lutjanus argentiventris (Pisces, Actinopterygii, Lutjanidae) in the SW Gulf of California: gonadic stages, spawning sea-sonality and length at sexual maturity. J. Appl. Ichthyol. 25:18-25.
- PiriniM. TestiS. VentrellaV. PagliaraniA. BadianiA. 2010. Blue-back fish: fatty acid profile in selected seasons and retention upon baking. Food Chem. 123:306-314.
- PoliB.M. ParisiG. ZampacavalloG. IurzanF. MecattiM. LupiP. BonelliA. 2003. Preliminary results on quality and quality changes in reared meagre (Argyrosomus regius): body and fillet traits and freshness changes in refrigerated commercial-size fish. Aquacult. Int. 11:301-311.
- PoliB.M. ParisiG. ZampacavalloG. MecattiM. LupiP. GualtieriM. FranciO. 2001. Quality outline of European sea bass (Dicentrarchus labrax) reared in Italy: shelf life, edible yield, nutritional and dietetic traits. Aquaculture 202:303-315.
- QuéménerL. 2002. Le maigre commun (Argyrosomus regius): Biologie, pêche, marché et potentiel aquacole. Ifremer Ed., Plouzané, France.
- QuéroJ.C. VayneJ.J. 1987. Le maigre, Argyrosomus regius (Asso, 1801) (Poissons, Perciformes, Sciaenidae) du golfe de Gascogne et des eaux plus septentrionales. Rev. Trav. Inst. Pech. Marit. 49:35-66.
- RahejaB.S. SadikotS.M. PhatakR.B. RaoM.B. 1993. Significance of the n-6/n-3 ratio for insulin action in diabetes. Ann. NY Acad. Sci. 683:258-271.
- RodriguezA. CarrilesN. CruzJ.M. AubourgS.P. 2008. Changes in the flesh of cooked farmed salmon (Oncorhynchus kisutch) with previous storage in slurry ice (-1.5°C). LWT-Food Sci. Technol. 41:1726-1732.
- RussoG. 2009. Dietary n-6 and n-3 polyunsaturated fatty acids: from biochemistry to clinical implications in cardiovascular prevention. Biochem. Pharmacol. 77:937-946.
- SanchoR.A. de LimaF.A. Guerra CostaG. Barros MariuttiL.R. BragagnoloN. 2011. Effect of annatto seed and coriander leaves as natural antioxidants in fish meatballs during frozen storage. J. Food Sci. 76:C838-C845.
- Santos-SilvaJ. BessaR.J.B. Santos-SilvaF. 2002. Effect of genotype, feeding system and slaughter weight on the quality of light lambs. II. Fatty acid composition of meat. Livest. Prod. Sci. 77:187-194.
- SAS, 2007. SAS/STAT software, release 9.1. SAS Institute, Inc., Cary, NC, USA.
- SchiavoneR. ZilliL. StorelliC. VilellaS. 2012. Changes in hormonal profile, gonads and sperm quality of Argyrosomus regius (Pisces, Scianidae) during the first sexual differentiation and maturation. Theriogenology 77:888-898.
- SensoL. SuarezM.D. Ruiz-CaraT. Garcia-GallegoM. 2007. On the possible effects of harvesting season and chilled storage on the fatty acid profile of the fillet of farmed gilthead sea bream (Sparus aurata). Food Chem. 101:298-307.
- SerhanC.N. 2007. Resolution phases of inflammation: novel endogenous anti-inflammatory and proresolving lipid mediators and pathways. Ann. Rev. Immunol. 25:101-137.
- ShearerK.D. 1994. Factors affecting the proximate composition of cultured fishes with emphasis on salmonids. Aquaculture 119:63-88.
- SimopoulosA.P. 1999. Essential fatty acids in health and chronic disease. Am. J. Clin. Nutr. 70:560S-569S.
- SimopoulosA.P. 2008. The importance of the ratio of omega-6/omega-3 essential fatty acids. Biomed. Pharmacother. 56:365-379.
- TocherD. 2003. Metabolism and functions of lipids and fatty acids in teleost fish. Rev. Fish. Sci. 11:107-184.
- TürkkanA.U. CakliS. KilincB. 2008. Effects of cooking methods on the proximate composition and fatty acid composition of seabass (Dicentrarchus labrax, Linnaeus, 1758). Food Bioprod. Process. 86:163-166.
- UlbrichtT.L.V. SouthgateD.A.T. 1991. Coronary heart disease: seven dietary factors. Lancet 338:985-992.
- WeberJ. BochiV.C. RibeiroC.P. VictorioA.M. EmanuelliT. 2008. Effect of different cooking methods on the oxidation, proximate and fatty acid composition of silver catfish (Rhamdia quelen) fillets. Food Chem. 106:140-146.
- YildizM. ŞeneE. TimurM. 2008. Effects of differences in diet and seasonal changes on the fatty acid composition in fillets from farmed and wild sea bream (Sparus aurata L.) and sea bass (Dicentrarchus labrax L.). Int. J. Food Sci. Tech. 43:853-858.