Abstract
The aim of the study was to analyze environmental impact of cow milk production in an alpine area through a cradle-to-farm-gate Life Cycle Assessment and to identify farming strategies that can improve environmental sustainability without negatively affecting profitability. Data were collected from farmers in 28 dairy farms in an Italian alpine valley. The production of 1 kg of fat protein corrected milk (FPCM) needed 3.18 m2 of land; land use on-farm was high because a large part of farm land consisted of pastures in the highland, used extensively during summer. Also the use of energy from non-renewable sources was high, 5.14 MJ kg FPCM-1 on average. Diesel for production and transportation of feed purchased off-farm was mainly used, especially concentrates which were entirely purchased. The average emission of greenhouse and acidification causing gases was 1.14 kg CO2-eq and 0.021 kg SO2-eq kg FPCM-1. Eutrophication was on average 0.077 kg of nitrate-eq kg FPCM-1. Farms with low producing cows had higher environmental impact per kg of milk and lower gross margin per cow compared to the others. Low stocking rate farms had the best results regarding acidification and eutrophication per kg FPCM. Farms with high feed self-sufficiency had significantly lower acidification potential than the others. Increasing milk yield per cow, by selection and feeding, and enhancing feed self-sufficiency, by higher forage production and quality and more exploitation of highland pastures, seem to be the best strategies to improve ecological performances of dairy farms in the Alps while maintaining their profitability.
Introduction
During the last five decades, agriculture and livestock systems in the Alps experienced an important structural transformation: traditional and small farms have been reducing, replaced by larger, more modern and specialized farms (Streifeneder et al., Citation2007), characterized by intensive livestock and crop productions. Simultaneously, a process of abandonment of highland pastures has occurred. Such modifications were induced by a complex of reasons, including increasing competitive market pressure, high production costs of the traditional production systems, abandonment and emigration due to more attractive employment and life opportunities outside the agricultural sector and outside the mountain areas. The change in the approach and intensity of land utilization and the decline of the traditional grazing in the highlands can have negative effects on landscape quality and biodiversity, as well as on the conservative functions of managed areas such as soil protection (Streifeneder et al., Citation2007; Sturaro et al., Citation2009). Moreover, the traditional environmental sus-tainability of alpine agriculture systems can be negatively affected by opening nutrient cycles: in dairy farms the large amounts of concentrate feeds purchased to sustain high milk productions and the extensive use of fertilizers and pesticides for demanding arable crops, such as maize, in the valley floors are leading to surplus of nitrogen and phosphorus (Penati et al., Citation2011) with the risk of soil and water pollution. Especially in the Alps the environmental effect of changing agricultural systems must be closely monitored to avoid the risk of altering the fragile ecosystem and losing a unique human heritage of aesthetic, ecological and cultural resources.
Life Cycle Assessment (LCA) is a methodology for the integral assessment of the environmental impact of products and processes. In this approach the potential environmental impacts of a product are evaluated by quantifying the resources consumed and the emissions to the environment at all stages of its life cycle (Guinée, Citation2002). In recent years, LCA has emerged as an internationally accepted method, widely used in the agricultural sector for the assessment of the environmental impact and for the identification of process hot-spots. Several LCA studies have recently been conducted on milk production in Europe to compare different farming strategies (Haas et al., Citation2001; Corson and van der Werf, Citation2008; Thomassen et al., Citation2008). In particular a recent work by Alig et al. (Citation2011) focused on LCA of Swiss milk production in a mountain region.
The aim of the study was to analyze the environmental impact of cattle milk production in an Italian alpine area through LCA methodology and to identify farming strategies that can improve environmental sustainability without affecting profitability.
Materials and methods
A group of 28 conventional dairy farms located in Valtellina, an alpine valley of the region Lombardia (North Italy), was chosen among farms members of a cooperative cheese factory producing Valtellina Casera and Bitto, two typical PDO cheeses. The criteria of selection were based on farm size, location and specialization: in particular, farms smaller than 10 cows and larger than 250 cows, farms located out of the province of Sondrio and farms with multiple animal productions (swine, beef) were excluded. The 28 dairy farms involved in the study were chosen on the basis of 3 different criteria: milk production level, stocking rate and feed self-sufficiency, expressed as the percentage of feed produced on farm on the total annual feed DM consumption. For each criterion we divided the sample into 3 levels and the thresholds were chosen on the basis of data provided by the dairy cooperative. Out of the 28 farms, twelve farms transferred most of their animals, including milking cows, to highland pastures (1500-2000 m asl) for a 3-month period during the summer. Data regarding herd composition, farm land area and related crop production, housing system, manure management and energy consumption were collected through personal interviews to the farmers. All the data are referred to the year 2006.
A cradle-to-farm-gate life cycle analysis (LCA) was applied. The functional units (FU) were: 1 kg of fat protein corrected milk (FPCM, 4.0% of fat content and 3.2% of protein content) estimated using the equation: FPCM (kg) = raw milk (kg) × (0.337 + 0.116 × % fat + 0.060 × % protein) (Heuer, Citation2004) from the results of the analyses performed by the cheese factory and 1 hectare of farm valley land. Direct impacts (on-farm) originated on the farm site were distinguished from indirect impacts (off-farm) associated with the production and transport of inputs to the farm (). All the flows are referred to one year of milk production. Water consumptions, seeds, medicines, mineral salts and washing detergents were not considered in the LCA due to the lack of detailed data (Thomassen et al., Citation2008; Castanheira et al., Citation2010). Inputs (land, energy) and outputs (emissions to the air and losses to the water) were classified in different impact categories: land use (m2), non-renewable energy use (MJ), climate change (kg CO2 eq.), acidification (kg SO2 eq.), eutrophication (kg NO3 eq.). Land use change was not considered. Impact categories, contributing elements and characterization factors are reported in . The main references for Life Cycle impacts are summarized in . Allocation of impacts of co-products in off-farm purchased concentrate feeds (maize, soybean, wheat, sunflower, sugar beet, barley, cottonseed) was based on their economic value in Italian market. An economic allocation was also applied for milk and meat (bull calves and cull cows) produced within each farm. Cheese produced during summer grazing period was included in the amount of milk produced by each farm on the basis of average cheese yield efficiency. Composition of the rations of farm animals was asked to the farmers while composition of each concentrate feed was estimated on the basis of the raw materials reported on the commercial labels with the help of CPM-Dairy Ratio Analyzer software (Cornell-Penn-Miner, Citation2004). Since 20 out of 28 farms bought replacing heifers from Switzerland, a simplified LCA was performed to assess the impacts associated to heifer rearing, considering animals sold at 24 months of age, an average feed intake, an average diet composition, standard housing conditions and manure management.
The reference for land use off-farm was Faostat (Citation2006) for foreign countries and Istat (Citation2006) for Italy. Data on cropping systems outside Italy for the concentrate feed production are based on statistics and qualified estimates by agricultural experts. The life cycle inventories (LCI) of diesel, fertilizers and pesticides production and use were derived from Davis and Haglund (Citation1999), Michaelis (Citation1998), and Brand and Melman (Citation1993), respectively. Energy costs to produce meals (Guidetti, Citation2001), flakes and other processed feeds were derived from Singh (Citation1986), Cederberg (Citation1998) and personal communications from feed industry experts. With respect to LCI of electricity, a combination of conventional and renewable sources was used (Michaelis, Citation1998; GSE, Citation2008). Impact of milk powder used for calves of both Valtellina farms and Switzerland farms rearing bought heifers was derived from LCA studies for milk production in France (Corson and van der Werf, Citation2008) and Germany (Haas et al., Citation2001) adding energy consumption for milk drying (Ramirez et al., Citation2006). Ammonia emissions from dairy cow barns and manure management and spreading, methane emitted from enteric fermentation and from manure management, and nitrous oxide losses from manure in the stables and during storage and spreading were computed using the Italian software ERICA (Provolo, Citation2005) based, for emissions derived from dairy cattle, on the Corinair Inventory Default Emission Factors Handbook (Corinair/CITEPA, Citation1992), IPCC (Citation1997) and, for spreading emissions, project ALFAM (Citation2002). For methane emissions from housing algorithms included in the software ERICA are based on live weight and fixed factors depending on livestock category; methane emissions from storage are estimated on the basis of fixed coefficients depending on livestock category, storage type and treatments, volatile solids and the time of storage. For ammonia from housing the factors considered are livestock category, housing type, amount and N content of feeding ration and milk yield and milk N content. Ammonia emissions from storage are estimated considering storage type and treatment. Nitrous oxide from storage is calculated from N excretion considering amount and N content of feeding ration and milk, using a fixed emission factor related to storage type. Ammonia and nitrous oxide emissions from manure spreading are estimated considering spreading system. Fixed values of N excretion, ammonia and nitrous oxide emissions from manure produced by Swiss and Italian heifers during non-grazing period (housing, storage and spreading) were derived from EMEP/EEA (Citation2009). Methane emission fixed values were derived from ISPRA (Citation2008) and from the Switzerland’s GHG Inventory 1990-2005 (FOEN, Citation2007) for heifers in Italy and in Switzerland, respectively. All the emissions and losses from the farms in Switzerland were considered for two years (average heifer age at the sale). A methane emission of 0.03 kg/d (Estermann et al., Citation2001) and an excretion of 0.2 kg N/d (Cornell-Penn-Miner, Citation2004) were assigned to dairy cows during summer grazing. An ammonia emission factor of 0.1 (10% of the nitrogen excreted; EMEP/EEA, Citation2009) and a nitrous oxide emission factor of 0.02 (IPCC, Citation2006) were considered during grazing. These values were reduced by 50% for grazing heifers. Nitrate leaching from grassland was computed as 1.5% of all the nitrogen applied to the fields (Grignani and Zavattaro, Citation2000). Nitrate leaching from maize land was computed using the equation suggested by Audsley (Citation2000). A fixed value of manure phosphorus content of 1% on DM basis was assumed (Adani, Citation1993; Regione Lombardia, Citation1993). Phosphorus leaching and runoff for both grassland and arable land were estimated using the equations proposed by Nemececk and Kagi (Citation2007). For off-farm feed production, the Tier 1 Emission Factor (EF) for ammonia of EMEP/EEA Citation2009 (8.4%) was used in all the cases where no information was available about the chemical composition of artificial fertilizers used. Otherwise when information about fertilizer composition was available from statistics and published studies, EF from EMEP/CORINAIR (Citation2002) were used to estimate ammonia emissions from different types of artificial fertilizers. Ammonia emission from manure distribution in crop production off-farm was estimated as 20% of the nitrogen applied, as it was assumed in Thomassen et al. (Citation2008) for foreign countries. Direct and indirect nitrous oxide emissions were estimated with IPCC (Citation2006) emission factors: in particular direct emission was computed as 1% of nitrogen from artificial fertilizers, manure and crop residues. Indirect emissions (based on emissions from volatilization and atmospheric deposition and from leaching of N) were computed as 1% of ammonia emissions and 0.75% of nitrogen leaching to ground water. For nitrate leaching of each Italian crop an average percentage of total nitrogen applied and deposited to the fields was considered. For foreign crops an average value of 30% of nitrogen applied was used, as suggested by IPCC (Citation2006).
The economic performances of the farms were evaluated by quantifying gross margin, as the difference between revenues (from milk, cheese, sold animals, CAP grants) and variable costs (purchased feeds, purchased animals, bedding material, diesel, electricity, gas, drugs and veterinary costs, seeds, fertilizers, pesticides, hired labour). Information required determining revenues and costs were obtained from farm interviews and from the databases of the cooperative cheese factory.
Statistics
The 28 dairy farms included in the study were chosen on the basis of 3 different criteria: milk production level (low: <4700 kg/cow; medium: 4700-6400 kg/cow; high: >6400 kg/cow), stocking rate (low: <2.3 cows/ha; medium: 2.3-3.3 cows/ha; high: >3.3 cows/ha) and feed self-sufficiency as the percentage of feed produced on farm on the total annual feed consumption expressed on dry matter (low: <53%; medium: 53-72%; high: >72%). These thresholds were chosen on the basis of data provided by the dairy cooperative. Average annual milk yield was 5531±1349 kg FPCM per cow, stocking rate in the lowland averaged 2.7±1.0 cows per ha, feed self-sufficiency was 63.3±16.6% of the total dry matter (DM) consumed. Fixed effects (groups of milk production, stocking rate, feed self-sufficiency) were tested in a general linear model (proc GLM; SAS, Citation2000) on all dependent variables (impact estimates) without covariate effects and without random farm effects, based on the following equation (no interactions were tested):
where:
Yijkl = dependent variables;
m = general mean;
MPi = milk production level effect (i=1-3);
SDj = stocking rate effect (j=1-3);
FSk = feed self-sufficiency effect (k=1-3); eijkl = model error
All dependent variables were tested for normality and homogeneity of variances. Least Square means were performed for all levels in each fixed effect, and the significance level was computed by orthogonal contrasts.
Results and discussion
Farms studied had on average 21.5±24.4 ha of land in the valley floor, of which about 84% was grassland and 16% arable land. In 12 farms part of the herds, including milking cows and heifers, was transferred to highland pastures for a 3-months period during the summer; highland pastures averaged 40.9±26.4 ha per farm. Average number of lactating cows was 44.8±44.9 with annual milk yield of 5531±2567 kg FPCM per cow, lower than average milk yield in the province of Sondrio in 2006 (6705 kg). The most common cow breed was Italian Friesian (58.6%), followed by Brown Swiss (31.5%). All the manure was used to support on-farm production of forages. On average, 63.3±16.6% of the total dry matter (DM) of cow rations consisted of feed ingredients produced on the farm, mainly grass hay and maize silage. All the concentrate feeds were purchased from off-farm; no farmers sold forages or exported manure from their farms.
Land use
Sample farms needed 3.23±1.96 m2 of land to produce 1 kg of FPCM, on average. Land use impact components were: land use on-farm (54%) and land use off-farm for production of imported feeds (46%). Land use on-farm was very high in comparison with other European studies (Corson and van der Werf, Citation2008; Thomassen et al., Citation2008). In fact in twelve farms a large part of farm land consisted of pastures in the highland, used in a very extensive way (very low stocking rate) only during the summer season. The increase of land use per kg of FPCM is due also to the decreased milk yield during summer grazing as a consequence of the low use of concentrate feed on pastures. On a global perspective the high value of land use per unit of milk can be seen as a weak point of alpine farming sustainability. However, in the alpine context, the evaluation of land use in quantitative terms can be unsatisfactory. In fact the seasonal use of pastures in the alpine highlands is considered to have several positive effects on biodiversity, landscape quality and preservation from soil degradation (Mc Donald et al., Citation2000; Marini et al., Citation2009). LCA methodology does not seem to be the best tool to evaluate these externalities because they play their main role at a local scale while LCA deals with global impacts.
Non-renewable energy use
Farms from this study needed on average 5.14±2.05 MJ from non-renewable energy sources to produce 1 kg of FPCM. shows the main components of non renewable energy use impact: diesel use associated to production and transportation of feed purchased off-farm (33%), electricity and diesel use on-farm (25 and 22%, respectively) and production of fertilizers and pesticides used for crop production off-farm (13%). The average diesel use on-farm was 346±288 kg/ha of lowland. Electricity use on-farm was very high (826 kWh/cow); it was used mainly for milking equipment, milk cooler, manure handling, feed mixing and distribution. Non-renewable energy use is higher than in other European studies (Haas et al., Citation2001; Corson and van der Werf, Citation2008; Thomassen et al., Citation2008). In particular energy use off-farm is consistent with average values from the other studies, but energy use on-farm is much higher. The average diesel use on-farm is very high if compared to 109 and 83 kg diesel per hectare of conventional and organic grassland in the German study (Haas et al., Citation2001). Similar results were reported by Alig et al. (Citation2011) that observed higher energy demand of farms in the mountain region to produce one kilogram of milk in comparison to farms in the plain region. This can be explained partly by the topography of the mountain regions that implies higher fuel consumption and partly by the lower crop yield due to altitude that forces to cultivate a larger area for the same amount of forage. In the studied farms the high energy use on-farm per kg of milk is explained also by the great land fragmentation in the valley floors.
Climate change
The average emission of greenhouse gases (expressed as CO2 equivalents) to produce 1 kg of FPCM in the studied farms was 1.14±0.27 kg, slightly lower than the results from other studies (Haas et al., Citation2001; Corson and van der Werf, Citation2008; Thomassen et al., Citation2008). Among the three greenhouse gases considered for the estimation the most important contribution was due to methane (42%), followed by carbon dioxide (33%) and nitrous oxide (25%).
The main components of climate change impact were: livestock emissions and manure storage on-farm (39%); fertilizer and manure spreading for feed production off-farm (16%) (). Castanheira et al. (Citation2010) found that enteric fermentation and manure management were responsible together for a large part (70%) of the global warming potential. Methane is emitted on-farm by enteric fermentation of cows and by manure management (in barns and then in manure storage facilities), whereas emission of nitrous oxide on-farm occurs only from manure management.
Acidification
The average emission of acidification causing gases (expressed as SO2 equivalents) to produce 1 kg of FPCM in the sample farms was 0.021±0.006 kg, higher than the results from the other studies but the one from Germany (Haas et al., Citation2001; Corson and van der Werf, Citation2008; Thomassen et al., Citation2008). This result was mainly due to feed production off-farm (34%) and livestock and manure storage emissions on-farm (30%) (). Ammonia is released during off-farm feed production from fertilizer and manure spreading. According to Thomassen et al. (Citation2008) and Castanheira et al. (Citation2010), acidification was almost totally due to ammonia emissions (83%). The emission of acidifying gases per hectare of lowland was 326±227 kg of SO2 equivalents.
Eutrophication
The production of 1 kg of FPCM in the sample farms caused the loss of 0.077±0.019 kg of nitrate equivalents on average. The loss of nitrate equivalents was on average higher than the results from organic farms but lower than the results from conventional farms of a Dutch study (0.070 and 0.110 kg of nitrate equivalents per kg of FPCM, respectively; Thomassen et al., Citation2008). Eutrophication was mainly due to: fertilizer and manure spreading for feed produced off-farm (60%), followed by fertilizer and manure spreading on-farm (23%) and livestock and manure storage emissions on-farm (14%). According to this, the main contribution to eutrophication was given by ammonia (46%) followed by nitrates (38%) and phosphates (9%) directly lost into the water bodies. This was the case also in the conventional system of the LCA study from Cederberg (Citation1998) and in Castanheira et al. (Citation2010). In our study, eutrophication was the only impact in which the off-farm contribution was higher than the on-farm one (58%). The eutrophication potential per hectare of lowland was 1150±894 kg NO3-equivalents.
Gross margin
The average of gross margin was 2016±449 €/cow. The main source of revenues was selling cow milk, which on average accounted for 65.7±20.9% of the revenues. For farms that practiced summer grazing, the second most important source of revenues originated from production and selling of high-value cheeses (such as Bitto PDO) and other milk derivatives produced during the summer period in the highland (28.3±10.4% of total revenues). As concluded in a previous paper (Penati et al., Citation2011), highland grazing results in extra revenues for the farmers due to the sale of highland cheese and to Alpine grazing subsidies.
In terms of contribution, 52.3±11.7% of all variable costs consisted in feed costs and about 14.7±9.1% were costs for energy sources.
farm characteristics
Farms with FPCM lower than 4700 kg () tend to have higher impacts per kg of milk mainly as a consequence of low milk production. The more is the milk produced per cow, the less are the animals needed to maintain the same farm production level and so the less is the environmental impact produced by livestock manure, methane release and feed consumed per kg of FPCM. In fact these farms had significantly higher land use per kg FPCM, both total and on-farm, in comparison with farms characterized by medium and high FPCM. Most of the farms practicing highland grazing (7 out of 12) were in this group; this represents an important contribution to on-farm land use. As pointed before in farms with seasonal grazing a large part of farm land consisted of pastures in the highland, used in a very extensive way (very low stocking rate) only during the summer season. Milk yield is reduced during summer period because of low concentrate feed integration of pasture grass in the highland, thus increasing land use per kg FPCM. Farms with low FPCM had also significantlyhigher energy use on-farm potential per kg of milk compared with group of medium FPCM (P<0.05). Climate change, both total and on-farm, was higher in the low FPCM group than in the others. Farms in the low FPCM group were small sized (25.7 vs 67.7 lactating cows in the high FPCM group; 9.5 vs 31.6 lowland ha in the high FPCM group) and probably less efficient in using resources. Arsenault et al. (Citation2009) argued that confinement-based dairy systems in North America make more efficient use of resources than pasture systems, which means that they reduce the relative impact by increasing the amount of milk produced. In a study of Iribarren et al. (Citation2011) it was suggested that, while efficiency should not be exclusively linked to high productivity, inefficient practices are mainly associated with low production levels. Farms with low FPCM failed also in terms of profitability: they registered the lowest gross margin per cow among the three groups (1789 vs 1903 € and 2432 €; P<0.05). Increasing milk yield per cow in dairy farms through genetic selection and better feeding/management practices has been proposed as one strategy to reduce greenhouse gas (GHG) emissions in agriculture, as less cows are needed to produce the same amount of milk (Casey and Holden, Citation2005; Rotz et al., Citation2010). However, the whole system effects of such practices are not entirely clear. For example, higher milk yield might result in reduced fertility, requiring more replacement animals (Lovett et al., Citation2006). Moreover high milk production requirements are not adequately supported by grazing and high genetic merit dairy cows do not fit well with grazing in the highland; as a consequence farms with high producing cows tend to abandon the practice of summer highland grazing which is a traditional system used in the Alps with protective function on mountain territory.
In our study farms with more productive cows assign a higher percentage of land to maize for silage to maintain the same level of feed self-sufficiency of the other two groups of farms. In fact the high production group used more on-farm land to produce maize for silage (29.0% vs 10.6% and 12.8% in the low and medium FPCM groups, respectively; P<0.05). Considering that these dairy farms are located in the Alps, which is an area with high biodiversity and landscape value, the opportunity to increase the percentage of land sown with maize instead of permanent grassland is controversial. Moreover, farms with more milk production per cow have higher on-farm eutrophication per hectare than the other two groups (669 g NO3/ha vs 487 g NO3/ha of low milk production and 631 g NO3/ha of medium milk production cows; P<0.05) partially as a consequence of more arable land and higher use of artificial fertilizers. For environmental problems with a local aspect, environmental impact should be evaluated not only per kg of product but also per hectare of land. In particular eutrophication pertains directly to the leaching and run-off of nitrate and phosphate to the ground and surface water, and therefore, this parameter contains a local aspect (Oudshoorn et al., Citation2011).
Farms with stocking rate lower than 2.3 cow/ha () had the best results in terms of acidification and eutrophication potentials per kg FPCM. The lowest intensive farms were characterized by few animals (24.7 cows on 15.5 hectares), whereas the group with medium stocking rate was associated with higher number of cows and hectares (57.4 cows on 29.2 hectares) Gross margin was not statistically different among the three groups of farms. The more intensive farms had more animals on a few hectares (56.3 cows on 20.3 ha). Most of the farms with cows grazing during summer were in the first two groups of stocking rate (10 out of 12). The negative consequences of intensification on the environmental impact of dairy farms were also confirmed by the results presented in similar studies in Europe (Thomassen et al., Citation2008; Basset-Mens et al., Citation2009). On the other hand, Alvarez et al. (Citation2008) found a positive relationship between intensification and economic efficiency for dairy farms in NW Spain. Casey and Holden (Citation2005) evaluated GHG emissions according to the system intensity of Irish dairy farms recommending a move towards extensi-fication in terms of area but towards intensification of animal husbandry (fewer cows producing more milk at lower stocking rates). shows that farms with feed self-sufficiency higher than 72% had higher on-farm impact (energy use, acidification and eutrophication) and lower off-farm impact (land use, energy use, acidification and eutrophication). These two opposite effects counterbalance in most cases except for total acidification, which is significantly higher in farms with feed self-sufficiency lower than 53%.
Farms with low and high self-sufficiency did not differ for stocking rate (2.92 vs 2.82 cows/ha) nor for summer grazing (4 farms in the less self-sufficient group, 5 in the second and 3 in the third group). Gross margin was not statistically different among the three groups of farms. The more self-sufficient farms obtained their performance mainly growing more maize for silage in the lowland instead of grassland compared with low self-sufficient farms (29.2% vs 7.0 % of lowland). Iribarren et al. (Citation2011) found that most of the efficient farms (lower impacts associated with the same amount of milk) used maize silage and concentrate as the two main feed products, ahead of grass silage and alfalfa. But in the case of alpine farming increasing the percentage of maize land in the lowland of the Alps raises concern regarding landscape and biodiversity preservation (Pileri, Citation2008). On the other hand Müller-Lindenlauf et al. (Citation2010) found that a combination of grassland-based farms with high yielding cows resulted in the lowest GHG emission per kg of milk. In New Zealand pasture-based systems, cows ingest their feed from and apply their excreta directly on pastures (Basset-Mens et al., Citation2009). This very short cycle reduces the energy consumption and all associated impacts linked with the production and the transport of concentrated feed, and also linked with effluent management.
Dairy farms in the Alps could better exploit their highland pastures during summer, increasing in this way their self-sufficiency: to avoid the decrease of milk production of high producing cows they could conveniently transfer in the highland replacing heifers and low producing cows
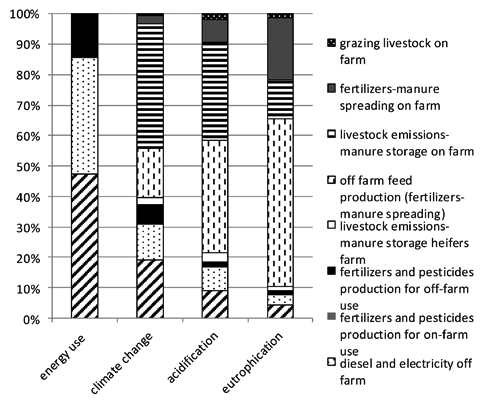
Table 1. Selected impact categories, contributing elements and characterization factors.
Table 2. Main literature references for Life Cycle impacts.
Table 3. Effect of milk production level on environmental impacts per 1 kg of Fat Protein Corrected Milk (Least Squares means).
Table 4. Effect of stocking rate on environmental impacts per 1 kg of Fat Protein Corrected Milk (Least Squares means)
Table 5. Effect of feed self-sufficiency on environmental impacts per 1 kg of Fat Protein Corrected Milk (Least Squares means).
Conclusions
Land use of sample farms was very high because a large part of farm land consisted of pastures in the highland, used in a very extensive way; it can be seen as a hot-spot for alpine production systems. However, in the alpine context the evaluation of land use in quantitative terms can be unsatisfactory. In fact the seasonal use of pastures in the highland is considered to have several positive effects on preservation of biodiversity, landscape quality and from soil degradation. Considering the other impacts the sample farms registered high non-renewable energy use mainly as a consequence of land fragmentation and soil declivity. A low stocking rate seems to be advantageous for the environment: in this mountain context it means to rear a few animals, because of the scarcity of lowland availability. From an economic point of view, farms with more cows are favourite, so any environmental solution that suggests a decrease in the animal number has to take into consideration subsidies supply. In order to diminish the local impact, feed self-sufficiency cannot be reached only by substituting grassland with maize for silage both because of the increase in energy and fertilizer use and because of aesthetic value of landscape. Feed self-sufficiency might increase by the improvement of forages production and quality and by a more efficient use of highland pastures.
In conclusion, farming strategies that can help to mitigate environmental impact of dairy production in the Alpine area could be on one hand the increase of cow productivity through selection and feeding and on another hand the increase of feed self-sufficiency through higher exploitation of highland pasture and the improvement of productivity and quality of lowland forages.
Acknowledgment
The authors would like to thank all the farmers who participated in the project and particularly the director of the cheese factory.
References
- AdaniF. 1993. Valorizzazione delle risorse in agricoltura: reflui zootecnici. Degree Diss., Università della Basilicata, Italy.
- ALFAM, 2002. Ammonia Loss from Field-applied Animal Manure. Available from: http://www.alfam.dk/
- AligM. BaumgartnerD.U. MieleitnerJ. NemecekT. 2011. Environmental impacts of Swiss milk production in the mountain region. In: PötschE.M. KrautzerB. HopkinsA. ( eds.) Grassland farming and land management systems in mountainous regions. Grassland Science in Europe, vol. 16. European Grassland Federation Publ., Zurich, Switzerland, pp 305-307.
- AlvarezA. del CorralJ. SolisD. PerezJ.A. 2008. Does intensification improve the economic efficiency of dairy farms? J. Dairy Sci. 91:3693-3698.
- ArsenaultN. TyedmersP. FreedenA. 2009. Comparing the environmental impacts of pasture-based and confinement-based dairy systems in Nova Scotia (Canada) using life cycle assessment. Int. J. Agric. Sustain. 7:19-41.
- AudsleyE. 2000. Systematic procedures for calculating agricultural performance data for comparing systems. In: WaidemaB.P. MeeusenM.J.G. ( eds.) Agricultural data for Life Cycle Assessment, vol. 1. Agricultural Economics Research Institute (LEI) Publ., The Hague, The Netherlands, pp 38-49.
- Basset-MensC. LedgardS. BoyesM. 2009. Eco-efficiency of intensification scenarios for milk production in New Zealand. Ecol. Econ. 68:1615-1625.
- BrandR.A. MelmanA.G. 1993. Energie-inhoudsnormen voor de veehouderij. TNO, Instituut voor milieu- en energietechnologie Publ., Apeldoorn, The Netherlands.
- CaseyJ.W. HoldenN.M. 2005. Analysis of greenhouse gas emissions from the average Irish milk production system. Agr. Syst. 86:97-114.
- CastanheiraE.G. DiasA.C. ArrojaL. AmaroR. 2010. The environmental performance of milk production on a typical Portuguese dairy farm. Agr. Syst. 103:498-507.
- CederbergC. 1998. Life cycle assessment (LCA) of animal production. Degree Diss., University of Göteborg, Sweden.
- Corinair/CITEPA, 1992. Corinair Inventory -Default Emission Factors Handbook. Centre Inter-professional Technique de la Pollution Atmospherique Publ., Paris, France.
- Cornell-Penn-Miner, 2004. CPM Dairy. Dairy cattle ration analyzer, version 3.0.6. Cornell University, Ithaca, NY, USA.
- CorsonM.S. van der WerfH.M.G. 2008. Effect of structural and management characteristics on variability of dairy farm environmental impacts. pp 280-285 in Proc. 6th Int. Conf. on LCA in the Agri-Food Sector, Zurich, Swizterland.
- DavisJ. HaglundC. 1999. Life cycle inventory (LCI) of fertilizer production; Fertilizer products used in Sweden and Western Europe. SIK-Report No. 654. The Swedish Institute for food and biotechnologies Ed., Göteborg, Sweden.
- EMEP/CORINAIR, 2002. Emission Inventory Guidebook - 3rd ed. October 2002 Update. Part B, Group 10. European Environment Agency Publ., København, Denmark.
- EMEP/EEA, 2009. Air pollutant emission inventory guidebook 2009. EEA Technical Report N. 9/2009. European Environment Agency Publ., København, Denmark.
- EstermannB.L. WettsteinH.R. SutterF. KreuzerM. 2001. Nutrient and energy conversion of grass-fed dairy and suckler beef cattle kept indoors and on high altitude pasture. Anim. Res. 50:477-493.
- Eurostat, 2006. External trade database. Available from: http://epp.eurostat.ec.europa.eu
- Faostat, 2006. ProdStat. Crops. Available from: http://faostat.fao.org
- FOEN, 2007. Swiss Greenhouse Gas Inventory 1990-2005. National Inventory Report 2007. Available from: http://www.bafu.admin.ch/climatereporting/00545/04333/in dex.html?lang=en
- GrignaniC. ZavattaroL. 2000. A survey on actual agricultural practices and their effects on the mineral nitrogen concentration of the soil solution. Eur. J. Agron. 12:251-268.
- GSE, 2008. Statistiche sulle fonti rinnovabili in Italia. Anno 2008. Available from: http://www.gse.it
- GuidettiR. 2001. Analisi energetica di sei aziende molitorie del nord Italia. Proc. 7th Nat. Congr. AIIA, Vieste del Gargano (FG), Italy. Available from: http://www.aiia.it/ images/Atti/VII_Vieste/c_09_39.pdf
- GuinéeJ.B. 2002. Handbook on Life Cycle Assessment. Operational Guide to the ISO Standards. Kluwer Academic Publ., Dordrecht, The Netherlands.
- HaasG. WetterichF. KopkeU. 2001. Comparing intensive, extensified and organic grassland farming in Southern Germany by process life cycle assessment. Agr. Ecosyst. Environ. 83:43-53.
- HeijungsR. GuinéeJ.B. HuppesG. LankreijerR.M. Udo de HaesH.A. Wagener SleeswijkA. AnsemsA.M.M. EggelsP.G. van DuinR. de GoedeH.P. 1992. Environmental Life Cycle Assessment of Products. Guide and Backgrounds. CML Publ., Leiden, The Netherlands.
- HeuerC. 2004. The use of test day information to predict energy intake of dairy cows in early lactation. J. Dairy Sci. 87:593-601.
- IPCC, 1997. IPCC guidelines for national greenhouse gas inventories, revised 1996. Intergovernmental Panel on Climate Change Publ., Paris, France.
- IPCC, 2006. 2006 IPCC guidelines for national greenhouse gas inventories, vol. 4, Agriculture, Forestry and Other Land Use. Intergovernmental Panel on Climate Change. Available from: http://www.ipccnggip.iges.or.jp/public/2006gl/pdf/4_Volum e4/V4_10_Ch10_Livestock.pdf
- IPCC, 2007. Climate Change 2007 Series. Cambridge University Press, New York, NY, USA.
- IribarrenD. HospidoA. MoreiraM.T. FeijooG. 2011. Benchmarking environmental and operational parameters through ecoefficiency criteria for dairy farms. Sci. Total Environ. 409:1786-1798.
- ISPRA, 2008 Agricoltura. Inventario nazionale delle emissioni e disaggregazione provinciale. Rapporti 85/2008. Available from: http://www.isprambiente.gov.it/it/pubbli-cazioni/rapporti/agricoltura-inventarionazionale-delle-emissioni-e
- Istat, 2006. Consultazione dati. Tavole per tema. Coltivazioni. Available from: http://agri.istat.it
- LovettD.K. ShallooL. DillonP. O’MaraF.P. 2006. A systems approach to quantify greenhouse gas fluxes from pastoral dairy production as affected by management regime. Agr. Syst. 88:156-179.
- MariniL. FontanaP. KlimekS. BattistiA. GastonK.J. 2009. Impact of farm size and topography on plant and insect diversity of managed grasslands in the Alps. Biol. Conserv. 142:394-403.
- Mc DonaldD. CabtreeJ.R. WiesingerG. DaxT. StamouN. FleuryP. GutierrezJ.L. GibonA. 2000. Agricultural abandonment in mountain areas of Europe: environmental consequences and policy response. J. Environ. Manage. 59:47-69.
- MichaelisP. 1998. Life Cycle Assessment of energy systems. Centre for environmental strategy. University of Surrey Publ., Berkshire, UK.
- Muller-LindenlaufM. DeittertC. KopkeU. 2010. Assessment of environmental effects, animal welfare and milk quality among organic dairy farms. Livest. Sci. 128:140-148.
- NemecekT. KägiT. 2007. Life cycle inventories of agricultural production systems. Data v2.0. Ecoinvent Report N. 15. Available from: http://www.poli.br/~cardim/PEC/Ecoinvent%20LCA/ecoinvent Reports/15_Agriculture.pdf
- OudshoornF.W. SørensenC.A.G. de BoerI.J.M. 2011. Economic and environmental evaluation of three goal-vision based scenarios for organic dairy farming in Denmark. Agr. Syst. 104:315-325.
- PenatiC. BerentsenP.B.M. TamburiniA. SandrucciA. de BoerI.J.M. 2011. Effect of abandonment highland grazing on nutrient balances and economic performance of Italian Alpine dairy farms. Livest. Sci. 139:142-149.
- PileriP. 2008. Fondovalle Alpino: un ambiente ad esaurimento. Territorio 44. Franco Angeli Ed., Milano, Italy.
- ProvoloG. 2005. Valutazione integrata ambientale degli allevamenti zootecnici. Paper N. 2006 in Proc. 8th Nat. Congr. AIIA. Catania, Italy.
- RamirezC.A. PatelM. BlokK. 2006. From fluid milk to milk powder: Energy use and Energy efficiency in the European dairy industry. Energy 31:1984-2004.
- Regione Lombardia, 1993. Norme per il trattamento, la maturazione e l’utilizzo dei reflui zootecnici. Legge Regionale 15 dicembre 1993, N. 37. Offical Journal No. 51, Suppl. 1, 20/12/1993.
- RotzC.A. MontesF. ChianeseD.S. 2010. The carbon footprint of dairy production systems through partial life cycle assessment. J. Dairy Sci. 93:1266-1282.
- SAS, 2000. SAS User’s Guide, ver. 8.1. SAS Inst. Inc., Cary, NC, USA.
- SinghR.P. 1986. Energy accounting of food processing operations. Elsevier Science Publ., New York, NY, USA.
- StreifenederT. TappeinerU. RuffiniF .V. TappeinerG. HoffmannC. 2007. Selected aspects of agro-structural change within the Alps. A comparison of harmonised agro-structural indicators on a municipal level. J. Alpine Res. 3:41-52.
- SturaroE. CoccaG. GalloL. MradM. RamanzinM. 2009. Livestock systems and farming styles in Eastern Italian Alps: an on-farm survey. Ital. J. Anim. Sci. 8:541-554.
- ThomassenM. van CalkerK.J. SmitsM.C.J. IepemaG.J. de BoerI.J.M. 2008. Life cycle assessment of conventional and organic milk production systems in the Netherlands. Agr. Syst. 96:95-107.