Abstract
The β-hexachlorocyclohexane (β-HCH) is a very stable and accumulable isomer of Lindane, a well known organochlorine pesticide. The HCHs were banned in all developed countries but to date high concern still exists for environment, animal and human health due to contaminated sites. In this study, several in vitro tests [cell viability (XTT), trypan blue exclusion (TBE), lactate dehydrogenase release (LDH) and bromodeoxyuridine (BrdU) incorporation assays] were performed to investigate the toxic effects of exposure to β- HCH (from 0.1 to 1000 μM) on bovine peripheral blood mononuclear cells (PBMCs). All the trials were performed incubating PBMCs for 2 and 7 days. At high concentrations (i.e. 1000 μM), the β-HCH approximately halved the number of living cells regardless the exposure time, significantly decreased the cell viability assessed by the XTT assay, and compromised the proliferation potential of PBMCs. At lower β-HCH exposure levels (0.1 to 100 μM), particularly after 7 days of exposure, a progressive decrease of cell viability has been observed. These adverse effects were significant at concentrations observed in the blood of cattle reared in polluted areas. The LDH results suggest that β-HCH does not clearly affect the integrity of the cell membrane in the range of exposure levels tested. All in all, these findings warn about the risk posed by the long-term exposure to β-HCH of farm animals reared in rural areas polluted by β-HCH. Further research is needed to deepen our knowledge about the mechanisms through which β-HCH affects the PBMCs functionality.
Introduction
A healthy environment is essential to support quality and safety of food products, a prerequisite for the health of consumers. However, some toxic substances can be spread into the environment and contaminate soil, water, foodstuff of plant origin, fodder and feed. As a final result, the migration of contaminants along the food chain may have adverse impacts on the health of both the wildlife and farm animals and, finally, on humans (Olivero-Verbel et al., Citation2011; Mrema et al., Citation2013). Among the wide range of chemical compounds, the organochlorine pesticides (OCPs) are able to alter the proper functioning of the endocrine system in animals and humans (Toppari et al., Citation1996; Safe, Citation2005; Mrema et al., Citation2013). Organochlorine pesticides are very stable in the environment because they are not readily metabolised, and their lipophilic character makes them highly accumulable in fat tissues as well as in breast milk, farm animal milk, meat and eggs (Olivero-Verbel et al., Citation2011). Among other OCPs, the hexachlorocycloexane isomers (mainly the α-, β-, γ-HCH), have been widely sold and used for crop protection and sanitation (Iwata et al., Citation1993) and were, but in some developing countries are still, released in the environment at rates incompatible with natural degradation processes. Many European Countries severely restricted or banned the HCHs usage between the years 1977 and 1992 (Breivik et al., Citation1999). In 1976, the United States banned technical HCH for agricultural purpose (ATSDR, Citation2003) and since 2001, all authorisations for plant protection products containing Lindane (purified γ-HCH) have been revoked. Nowadays, the indirect environmental contamination due to the accumulation of wastes in industrial areas represents the main concern regarding HCHs as well as other obsolete pesticides in Western countries. A large number of contaminated sites exist in Europe; recently, the European Environmental Agency estimated at least 250.000 sites as at risk (EEA, Citation2007). A high portion of these sites (about 2.4%) could represent a potential hazard for ecosystems (EEA, Citation2007). As a matter of fact, the presence of uncontrolled contaminated sites represent a serious threat for agro-ecosystems. Episodes of agro-environmental contamination are not rare especially in some Eastern European countries such as Romania (Covaci et al., Citation2001), Russia (Nikanorov et al., Citation2007), Serbia (Skrbic’ and Durisic-Mladenovic, Citation2007), but also in other countries such as Germany (Jürgens and Roth, Citation1989; Manz et al., Citation2001; Vijgen et al., Citation2011), Spain (Concha-Graña et al., Citation2006), Greece (Golfinopoulos et al., Citation2003), UK (Meijer et al., Citation2001), Netherlands (Langhenhoff et al., Citation2002), Turkey (Ayas et al., Citation1997), Canada and USA (Phillips et al., Citation2005). Recently, also in Italy an emergency related to agro-environmental contamination by HCHs has risen concerns for its agroecological end human health implications. For instance, Ronchi and Danieli (Citation2008) reported the occurrence of HCH isomers in soil, plants and bovine milk within a rural area devoted to dairy cow farming (the Sacco River Valley, Lazio Region). In particular, the β-hexachlorocycloexane (β-HCH) was found in the blood serum of lactating cows (Ronchi and Danieli, Citation2008). The occurrence of these contaminants was related to the chemical industry, established in that area at the beginning of 20th century, producing fine chemicals such as OCPs, phosphate esters and ketones. In that area, some unauthorised landfills within which both processing residues and unsold fine chemical stocks were disposed of in the past without any further control measures are still existing (IZSLT, Citation2005). Considering that a lot of contaminated sites in industrialised countries exist, and HCH is still used in some developing ones (e.g., India), it is interesting to understand what are the effects of this substance on farm animals. Up to now, some in vitro studies have been focused on the negative effects of HCH on the reproductive system of livestock (Tiemann et al., Citation1996; Tiemann and Küchenmeister, Citation1999; Tiemann, Citation2008). However, given the relevant role of the immune system providing the body’s first line of defence against invading pathogens, any possible adverse effect of body accumulation of HCHs on the animal immune system should be carefully evaluated. Taking in debt consideration that HCHs could predispose farm animals to the infectious diseases, resulting in unsafe animal products and economic losses for the livestock industry and that different effects and mechanisms may play a role on immune-cells functionality in vitro, and possibly in vivo, the aim of this study was to examine the effects of the in vitro exposure to β-HCH on bovine peripheral blood mononuclear cells (PBMCs) assessing the cytotoxicity potential of this highly stable and accumulable HCH isomer. For this purpose, various tests such as the trypan blue exclusion assay, XTT (2,3-bis(2-methoxy-4-nitro-5-sulfophenyl)-5- [(phenylamino)carbonyl]-2H-tetrazolium hydroxide) assay, lactate dehydrogenase release (LDH) assay and bromodeoxyuridine (BrdU) incorporation assay, have been used to evaluate the responsiveness of target cellular functions separately under different exposure conditions to the β-HCH.
Materials and methods
Cells and chemicals
Four healthy Friesian heifers (about two years old) were used as blood donors. Blood samples were collected via jugular venipuncture, using 10 mL evacuated glass tubes (BD VACUTAINER®; Becton, Dickinson and company, Franklin Lakes, NJ, USA). After collection, blood samples were stored at 4°C and promptly transferred to the laboratory for isolation of PBMCs. High purity (98.2%), crystalline β-HCH (Sigma Aldrich, St. Louis, MO, USA) was dissolved in dimethylsulfoxide (DMSO) (Sigma Aldrich) at a concentration 0.1 M; subsequently this solution was diluted in Roswell Park Memorial Institute medium (RPMI) 1640 (Lonza sales Ltd, Basel, Switzerland) to final test concentrations. The concentration range was defined on the basis of an extensive literature review regarding the toxicological and bio-medical sector; it has evidenced that, for this compound, the concentrations in the order of μM were usually tested in vitro (Steinmetz et al., Citation1996; Payne et al., Citation2001; Zou and Matsumura, Citation2003; Aubé et al., Citation2011). However, in some studies on human PBMCs, also concentrations up to the order of mM have been used (Ennaceur et al., Citation2008; Dar et al., Citation2012). The final concentration of DMSO was always 0.2% (v/v) as chosen on the basis of preliminary experiments to test the toxicity of DMSO. No adverse effects were observed cultivating PBMCs up to 7 days in medium containing 0.05, 0.1 or 0.2% DMSO as far as the proliferative potential, live cell count or cell functionality if compared with control cells (without DMSO). Consequently, the highest concentration of DMSO (0.2% v/v) that allowed us solubilising the β-HCH up to the highest concentration.
Isolation and treatment of peripheral blood mononuclear cells
The PBMCs were isolated from whole blood as previously described by Lacetera et al. (Citation2002). After isolation, living PBMCs were counted by the trypan blue exclusion (TBE) methods and properly diluted at a density of 2 106 cells/mL in RPMI 1640 with 10% of fetal bovine serum (Life Technologies, Carlsbad, CA, USA) containing 2 mM of glutamine, 100 μg/mL streptomycin and 100 U/mL of penicillin (Sigma Aldrich). After cells were seeded (50 μL per well in 96 wells microplates) in triplicate, and subsequently 50 μL aliquots of medium containing sufficient β-HCH to reach the final concentration of 0.1, 1, 10, 100 and 1000 μM was added in each well. Throughout the study, treated and control PBMCs were incubated for 2 or 7 days at 39°C in a humidified atmosphere with 5% of CO2.
Trypan blue exclusion assay
The count of viable cells was performed using the TBE method. After incubation with β-HCH, 10 μL aliquots of medium containing exposed or control cells were mixed with 10 μL of Trypan blue (0.4%) (Invitrogen, Carlsbad, CA, USA), and 10 μL of suspension were pipetted into disposable Countess® chamber slides to be read through an automated cell counter (Countess® Automated Cell Counter; Invitrogen).
Cell viability assay
The XTT assay was used to monitor the cell viability based on the capacity for active cells to metabolise 2,3-bis(2-methoxy-4-nitro-5-Sulfophenyl)-5-[(phenylamino)carbonyl]-2H-tetrazolium hydroxide) (XTT), a tetrazolium salt soluble in water, giving formazan, a soluble orange compound, which may be directly quantified by spectrophotometry. The amount of produced formazan in a given time reflects the cellular metabolic activity (Gerlier and Thomasset, Citation1986). The procedural details given below are related to the Cell Proliferation Kit II (Roche Diagnostics, Basel, Switzerland). At the end of the incubation period (2 or 7 days), an aliquot of 50 μL per well of XTT (0.3 mg/mL) was added, and plates were incubated for further 24 h. In the end, the absorbance was measured at 450 nm and 650 nm (reference wavelength) against air with a Sunrise plate reader (TECAN, Männedorf, Switzerland). For each well, the difference of absorbance at 450 and 650 nm was calculated and then the background absorbance difference (A450-A650) was subtracted as described by Scudiero et al. (Citation1988).
Lactate dehydrogenase cytotoxicity assay
The lactate dehydrogenase cytotoxicity assay is a colorimetric assay for quantitating cytotoxicity/cytolysis by measuring the activity of LDH released by damaged cells. According to the instruction of the Cytotoxicity Detection Kit PLUS (LDH) used (Roche Diagnostics), the estimate of the LDH release in the culture medium was performed after incubation for 2 or 7 days. At the end of incubation time, 5 μL/well of lysis solution were added in the high control wells (cells without treatments) and incubated for 15 min. The high control is recommended by the kit’s manufacturer and it determines the maximum rilesable LDH activity by the cells. After that, 100 μL of the reaction mixture were added to each well (control, high control and treatments), and incubated for further 15 min. Subsequently, 50 μL of stop solution were added to each well, and the absorbance was read at 492 nm and 650 nm (reference wavelength) against air by a Sunrise plate reader (TECAN). For each well, the absorbance difference of absorbance at 492 and 650 nm was calculated and then the background absorbance difference (A492-A650) was subtracted.
Bromodeoxyuridine incorporation assay
It is a quantitative colorimetric assay which measures the incorporation of BrdU (5-bromo-2’-deoxyuridine), a synthetic analog of thymine, within the cells that are in the S phase of their life cycle. Anti-BrdU antibodies are used to detect cells that have incorporated the BrdU. For this test, a Biotrack Cell Proliferation ELISA System, version 2 kit (GE Healthcare, Little Chalfont, UK) was used. At the beginning of exposure trials, the cells were seeded in the presence of Concavaline A (ConA; 1.25 μg/mL) or Pokeweed mitogen (PWM; 0.02 μg/mL) (Sigma Aldrich). After incubation for 2 or 7 days, the incorporation of BrdU was assessed according to the supplier’s instructions and the absorbance was read at 450 nm against air by using a Sunrise plate reader (TECAN).
Statistical analysis
All experimental data, expressed as relative responses compared to the control, were analysed by ANOVA using STATISTICA 7 (StatSoft Inc., Tulsa, OK, USA). The factorial model included the treatment (control, β-HCH exposure at 0.1, 1, 10, 100 and 1000 μM), the incubation time (2 or 7 days) and the animal as fixed effects, the treatment incubation time interaction plus the error term. The significance of the differences was assessed by the Fisher’s least square difference test. Significance was declared at P<0.05.
Results
With the exception of the 100 μM treatment, the results obtained by using the TBE method did not show significant differences between the two incubation times (). However, also at 10 μM a marginal difference (P=0.05) between the two incubation times was observed. Treating PBMCs with β-HCH at 1000 μM, a significant (P<0.01) reduction was seen in the number of living cells (-45.1 and -52.9% compared to the control at 2 and 7 days, respectively). The resulting data from the XTT assay () showed that, after 2 days of exposure at the concentrations of 10 and 100 μM, β-HCH reduced the cell functionality of about 10% while at the concentration of 1000 μM the metabolic activity of PBMCs was 45% lower than the control. Noteworthy, the PBMCs exposed to 1 μM of β-HCH exhibited a higher (P<0.05) response compared to the control. Overall, the exposure-dependent effect was clearer after 7 days. In fact, from 10 to 1000 μM β-HCH, a progressive reduction of the cell metabolic activity was recorded (). Within the 1-1000 μM treatment range, the differences between 2 and 7 days were always significant. Weighting the XTT data by the corresponding TBE assay values (), it was clearly confirmed the increase of metabolic activity at individual cell level after exposure for 2 days to β-HCH 0.1 and 1 μM. On the contrary, after 7 days, the exposure to β-HCH induced a reduction of the single-cell metabolic activity at all tested concentrations.
The trials conducted to study the releases of LDH by PBMCs as exposure result showed an increased (P<0.01) LDH activity in the medium of cells treated for 2 days with β-HCH from 10 up to 100 μM, if compared with control cells (). On the contrary, after 7 days of exposure, there were no significant differences between control and treated cells. The BrdU incorporation assays showed rather different results according to the different mitogens and times of exposure (). After 2 days, PBMCs stimulated with PWM exhibited a gradual reduction of proliferative activity from 0.1 μM to 1000 μM β-HCH; at the highest concentration tested (i.e. 1000 μM) the proliferative activity was 87.4% lower if compared with the control (P<0.01). A similar reduction was observed in PBMCs treated with β-HCH 1000 μM for 7 days but not at lower concentrations (). A rather different picture was seen stimulating PBMCs with the mitogen ConA. After 2 days of exposure to β-HCH at 1000 μM, a 35% reduction (P<0.01) of proliferation activity compared to the control was seen. Surprisingly, the cells treated with β-HCH at 100 μM showed a significant increase of proliferative activity while PBMCs exposed from 0.1 to 10 μM were not appreciably affected by β-HCH. After 7 days of incubation, it was seen a decrease (P<0.05) of the proliferative capacity of PBMCs exposed to 100, and 1000 μM β-HCH compared to control. However, it is interesting to notice that, regardless the mitogen used, after 7 days of exposure the PBMCs treated with β-HCH from 0.1 up to 10 μM showed a proliferative potential comparable to that of untreated cells ().
Discussion
The cell viability is routinely assessed in vitro using different colorimetric techniques. For this purpose, the XTT [as well as 3(4,5-dimethylthiazol-2-Yl)-2,5-diphenyltetrazolium bromide (MTT)] and TBE assays are often used even though they are substantially different. In fact, while the XTT monitors the mitochondrial enzymatic activity, the TBE discriminates living from non-living cells according to the permeability of the cell membranes to the staining substance used. So, any perturbation of the mitochondrial activity may interfere with the number of living cells-to-response relationship, on which the viability estimation made by XTT is relied on. Accordingly, the unexpected increase of viability measured by the XTT test on PBMCs exposed at low level (i.e. 1 μM β-HCH) for 2 days could be easily explained. Other works reported similar behaviour using an analogous assay (MTT) on CHO-K1 treated with Malathion and related molecules (Moore et al., Citation2010) and on HepG2 cells with carbamate pesticides (José Ruiz et al., Citation2006). Furthermore, the apparent stimulatory effect seen at 2 days of incubation, was in line with a recognised relatively common biological phenomenon characterised by low dose stimulation and high dose inhibition as a consequence of cell protection or adaptative response to pesticide exposure (José Ruiz et al., Citation2006).
The results showed that PBMCs treated with β-HCH at concentrations ranging from 0.1 to 100 μM had a reduced metabolic activity after 7 days of exposure as assessed by the XTT, even though this was not reflected by a commensurable decrease of countable cells through the TBE method. In particular, the data on single cell metabolic activity (XTT/TBE responses) suggest that the exposure to low β-HCH concentration (i.e. 0.1 μM) could have exerted a damaging effect altering the cell’s mitochondrial activity that was strongly reduced in PBMCs after incubation for 7 days at all concentration tested. This finding may be important in the perspective of long term exposure to β-HCH that farm animals may experience in vivo since the β-HCH concentration tested in vitro and the concentration found in vivo were comparable. In fact Ronchi and Danieli (Citation2008) found that the concentration of β-HCH in vivo was in the range 0.1 to 1 μM in the blood serum of cattle reared in the Sacco River Valley (Province of Rome, Lazio Region, Italy). It is known that a low XTT response may be explained by reduced mitochondrial activity possibly due to the depletion of nutrients in the culture medium (Figenschau et al., Citation1997) or by an increase of superoxide anion (O2–) that can interfere with the mitochondrial activity (Shimamura et al., Citation2000; Wang et al., Citation2011). In the experimental conditions of this work, a possible confusing effect imputable to the depletion of nutrients was taken into account through the way the results have been expressed (relative response to the control). In the light of these facts, it may be hypothesised that the prolonged exposure to β-HCH (i.e. 7 days) may impair the metabolic activity of PBMCs by the increase of free radicals thereby altering the oxidative status of the cell. This hypothesis is partially confirmed by others (Lowy et al., Citation1977; Bagchi and Stohs, Citation1993; Ahmed et al., Citation2011) who reported that several pesticides including Lindane (α-HCH) induce an intracellular increase of reactive oxygen species.
In addition to their established role in generating energy for the cell, mitochondria play a key role into controlling life and death by releasing cytochrome c into the cytosol, thereby activating apoptotic processes (Desagher and Martinou, Citation2000). In particular, an impaired intracellular oxidative balance may determine the selection between apoptosis and necrosis. Vairetti et al. (Citation2005) have demonstrated that the apoptotic death is characterised by low level of LDH release while necrosis is characterised by a high level of LDH release. Chan et al. (Citation2013) stated that the LDH release can be considered as a useful marker for detecting cell necrosis. On the contrary, LDH release is usually low in apoptotic tumour cells (McCourt et al., Citation2000). The results on LDH release in the culture medium indicate that under short exposure (i.e. 2 days) some damaging effects at cell membrane level occurred but not after 7 days of exposure. This finding was partially in line with other studies reporting a significant increase of LDH release in MDCK cells exposed to Lindane for 48 h (Piskac-Collier and Smith, Citation2009) or in SH-SY5Y human neuroblastoma cells exposed to tribromophenol up to an incubation time of 72 h (Ríos et al., Citation2003). However, considering also the above reported findings on TBE and XTT test, it can be alternatively hypothesised that the prolonged exposure to β-HCH could have triggered PBMCs toward mechanisms leading to cell death that not necessarily implied a damage of the plasma membrane, such as in the case of apoptosis (Desagher and Martinou, Citation2000). This hypothesis would be supported by the evidences that the OCP DDT and related metabolites may induce apoptosis in vitro on human PBMCs treated at concentrations within the 100-1000 μM range (Pérez-Maldonado et al., Citation2004).
In this study, the reduction of proliferative capacity in PBMCs treated with increasing level of β-HCH was assessed as confirmatory end-point of immune-toxic effect of β-HCH. Interestingly, the bovine PBMCs response under PWM stimulation was depressed after 2 days by β-HCH even at levels (i.e. 0.1 to 1 μm) comparable to those found in serum blood of lactating cows (Ronchi and Danieli, Citation2008). This fact could be a matter of concern about in vivo exposure to β-HCH because, especially in some circumstances (e.g., high metabolic activity in early lactating ruminants), farm animals may be highly susceptible to the immune-toxic hazard represented by blood circulating β-HCH. On the contrary, at the incubation time of 7 days, we did not observe significant effects on the PBMC proliferation activity. In this regard, after prolonged exposure (i.e. 7 days). It may be hypothesised that the mechanism of DNA repair leading to a reestablished normal proliferation potential has been taken place. In supporting this hypothesis, Yàñez et al. (Citation2004) showed that the organochlorine compound 1,1-dichloro-2,2-bis(p-chlorophenyl) ethylene (DDE, a metabolite of DDT), caused an increase of DNA fragmentation in human PBMCs after 24 to 48 h of exposure while after 72 h the DNA damage did not differ from the control. Furthermore, in our previous experiences with bovine PBMCs exposed in vitro to organic toxicants (e.g., Fumonisin B1) (Danieli et al., Citation2010), it was showed that after 2 days of exposure, the PBMCs stimulated with PWM exhibited a reduced proliferative capacity that, in turn, was substantially restored after 7 days of exposure. Data obtained by BrdU assays after 7 days of exposure seem to be in contrast with those obtained by XTT assay. However, it should be taken in consideration that in the XTT assay, the cells were not treated with mitogen. In our previous research (unpublished data) we observed that performing the MTT (an XTT analogous assay) on bovine PBMCs exposed to a toxicological relevant substance (potassium dichromate), different responses after stimulation with PWM may be expected according to the time of exposure. In fact, at 2 days of exposure, the PBMCs showed a decrease of metabolic activity compared to the control while after 7 days of exposure there were no difference between controls and treated cells. Altogether, these evidences suggest that the metabolic activity of PWM-stimulated PBMCs after prolonged exposure to β-HCH and possibly other toxicants be different in comparison with un-stimulated cells thereby explaining the contrasting results obtained by XTT and BrdU assay under stimulation by PWM.
Looking at the results obtained from BrdU assay, we can conclude that the exposure to β-HCH at concentrations between 100 and 1000 μM inhibits the PBMCs proliferation capacity, and the extent of inhibition depends upon the specific mitogen used. At lower concentrations, the response may be very different according to the specific mitogen used and exposure duration. In this respect, other works have shown that cell proliferation can be increased by treatment with pesticides at μM level (Balakrishnan et al., Citation2002; Giribaldi et al., Citation2011) while long or repeated exposure to OCPs is often associated with decreased response to mitogens (Vine et al., Citation2001; Dar et al., Citation2012). In addition, the differences in responsiveness of PBMCs under mitogen stimulation may be explained in the light of the differential impact of β-HCH on different lymphocyte sub-populations (Neri et al., Citation1973; Mellstedt, Citation1975).
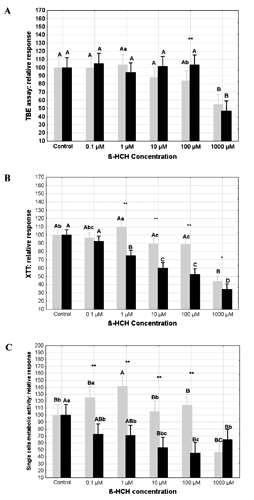
Table 1. Effect of 2 or 7 days exposure to different concentrations of β-hexachlorocyclohexane on the lactate dehydrogenase release of bovine peripheral blood mononuclear cells.
Table 2. Effect of 2 or 7 days of exposure to different concentrations of β-hexachlorocyclohexane on the peripheral blood mononuclear cells proliferation potential measured by the bromodeoxyuridine incorporation assay after stimulation with Pokeweed mitogen or Concavalin A.
Conclusions
This investigation adds new information about the effects of the organochlorine compound β-HCH on the immune system of cattle, and some interesting conclusions can be drawn and some research perspectives can be formulated. In particular, with the exception of cell membrane leakage of LDH, the exposure to β-HCH at concentrations above 100 μM may negatively affect all the investigated cell functions (cell viability, mitochondrial metabolism, cell proliferation). At lower exposure levels (i.e., 0.1-100 μM), we observed different responses according to different assays and incubation times. Overall, the state of intracellular stress, due to exposure to β-HCH followed by the activation of mechanisms in which the mitochondria are most probably involved, can be hypothesised. Based on the results of the current research and data from the literature, future studies should be aimed at monitoring the free radicals production in bovine PBMCs exposed to β-HCH to ascertain the onset of an altered oxidative status as a key mechanism inducing cell impairment and/or cell death.
Acknowledgements
This work was financially supported by the National Institute for Insurance against Accidents at Work (INAIL, Italy), Presidential Decision n. 165/2010, Project ID 7/1010, and by the University of Tuscia. The authors wish to thank Dr. Nicoletta Ascenzi, who granted the collection of blood from donor animals and Ms. Giorgina Kuzminsky for her helpful technical support.
References
- AhmedT. PathakR. MustafaM.D. KarR. TripathiA.K. AhmedR.S. BanerjeeB.D., 2011. Ameliorating effect of N-acetylcysteine and curcumin on pesticide-induced oxidative DNA damage in human peripheral blood mononuclear cells. Environ. Monit. Assess. 179:293-299.
- ATSDR, 2003. Toxicological profile for alpha-, beta-, gamma-, and delta-hexachlorocycloexane. Agency for Toxic Substances and Disease Registry, US Department of Health and Human Services, Georgia, USA.
- AubéM. LarochelleC. AyotteP., 2011. Differential effects of a complex organochlorine mixture on the proliferation of breast cancer cell lines. Environ. Res. 111:337-347.
- AyasZ. BarlasN. KolankayaD., 1997. Determination of organochlorine pesticide residues in various environments and organisms in Goksu Delta, Turkey. Aquat. Ecol. 39:171-181.
- BagchiM. StohsS.J., 1993. In vitro induction of reactive oxygen species by 2,3,7,8- tetrachlorodibenzo-p-dioxin, endrin, and lindane in rat peritoneal macrophages, and hepatic mitochondria and microsomes. Free Radical Bio. Med. 14:11-18.
- BalakrishnanS. UppalaP.T. RupaD.S. HasegawaL. EastmondD.A., 2002. Detection of micronuclei, cell proliferation and hyperdiploidy in bladder epithelial cells of rats treated with o-phenylphenol. Mutagenesis 17:89-93.
- BreivikK. PacynaJ.M. MünchJ., 1999. Use of α-, β- and γ-hexachlorocyclohexane in Europe, 1970-1996. Sci. Total Environ. 239:151-163.
- ChanF.K. MoriwakiK. De RosaM.J., 2013. Detection of necrosis by release of lactate dehydrogenase activity. Methods Mol. Biol. 979:65-70.
- Concha-GrañaE. Turnes-CarouM.I. Muniategui-LorenzoS. López-MahíaP. Prada-RodriguezD. Fernández-FernándezE., 2006. Evaluation of HCH isomers and metabolites in soils, leachates, river water and sediments of a highly contaminated area. Chemosphere 64:588-595.
- CovaciA. HuraC. SchepensP., 2001. Selected persistent organochlorine pollulants in Romania. Sci. Total Environ. 280:143-152.
- DanieliP.P. CatalaniE. LaceteraN. RonchiB., 2010. Comparative assessment of Fumonisin B1 and hydrogen peroxide in vitro exposure on bovine lymphocytes proliferative response. Page 237 in Proc. EAAP 61st Annual Meeting, Heraklion, Greece.
- DarS.A. DasS. RamachandranV.G. BhattacharyaS.N. MustafaM.D. Baneq’eeB.D. VernaP., 2012. Alterations in T-lymphocyte sub-set profiles and cytokine secretion by PBMC of systemic lupus erythematosus patients upon in vitro exposure to organochlorine pesticides. J. Immunotoxicol. 9:85-95.
- DesagherS. MartinouJ.-C., 2000. Mitochondria as the central control point of apoptosis. Trends Cell Biol. 10:369-377.
- EEA, 2007. Progress in management-of-contaminated-sites (CSI 015). The European Environmental Agency Publ., Copenaghen, Danemark. Available from: http://www.eea.europa.eu/data-and-maps/indicators/progress-in-manage-ment-of-contaminated-sites/progress-in-management-of-contaminated-1
- EnnaceurS. RidhaD. MarcosR., 2008. Genotoxicity of the organochlorine pesticides 1,1-dichloro-2,2- bis(pchlorophenyl)ethylene (DDE) and hexachlorobenzene (HCB) in cultured human lymphocytes. Chemosphere 71:1335-1339.
- FigenschauY. YousefM.I. SveinbjörnssonB. BertheussenK., 1997. A sensitive serum-free colorimetric assay for the detection of cytotoxic effects of pesticides. J. Environ. Sci. Heal. B 32:177-194.
- GerlierD. ThomassetN., 1986. Use of MTT colorimetric assay to measure cell activation. J. Immunol. Methods 94:57-63.
- GiribaldiL. ChiappiniF. PontilloC. RandiA.S. Kleiman de PisarevD.L. AlvarezL., 2011. Hexachlorobenzene induces deregulation of cellular growth in rat liver. Toxicology 289:19-27.
- GolfinopoulosS.K. NikolaouA.D. KostopoulouM.N. XilourgidisN.K. VagiM.C. LekkasD.T., 2003. Organochlorine pesticides in the surface waters of Northern Greece. Chemosphere 50:507-516.
- IwataH. TanabeS. SakaiN. TatsukawaR., 1993. Distribution of persistent organochlorines in the oceanic air and surface seawater and the role of ocean on their global transport and fate. Environ. Sci. Technol. 27:1080-1098.
- IZSLT, 2005. Contaminazione delle produzioni zootecniche da beta-esaclorocicloesano nel territorio delle produzioni zootecniche da beta-esaclorocicloesanonel territorio del bacino del fiume Sacco. Istituto Zooprofilattico Sperimentale delle Regioni Lazio e Toscana Publ., Roma, Italy.
- José RuizM. FestilaL.E. FernándezM., 2006. Comparison of basal cytotoxicity of seven carbamates in CHO-K1 cells. Toxicol. Environ. Chem. 88:345-354.
- JürgensH.-J. RothR., 1989. Case study and proposed decontamination steps of the soil and groundwater beneath a closed herbicide plant in Germany. Chemosphere 18:1163-1169.
- LaceteraN. FranciO. ScaliaD. BernabucciU. RonchiB. NardoneA., 2002. Effects on functions of ovine blood mononuclear cells for each of several fatty acids at concentrations found in plasma of healthy and ketotic ewes. Am. J. Vet. Res. 63:958-962.
- LanghenhoffA.A.M. StapsJ.J.M. PijlsC. AlphenaarA. ZweipG. RijnaartH.H.. 2002. Intrinsic and stimulated in situ biodegradation of hexachlorocycloexane (HCH). Water Air Soil Poll. 2:171-182.
- LowyR. AlbrechtR. PelissierM.A. ManchonPh., 1977. Determination of the no effect levels of two pesticides, Lindane and Zineb, on the microsomalenzyme activities of rat liver. Toxicol. Appl. Pharm. 42:329-338.
- ManzM. WenzelK.-D. DietzeU. SchuurmannG., 2001. Persistent organic pollulants in agricultural soils of central Germany. Sci. Total Environ. 277:187-198.
- McCourtM. WangJ.H. SookaiS. RedmondH.P., 2000. Taurolidine inhibits tumor cell growth in vitro and in vivo. Ann. Surg. Oncol. 7:685-691.
- MeijerS.N. HalsallC.J. HarnerT. PetersA. J. OckendenW.A. JohnsonA.E. JonesK.C., 2001. Organochlorine pesticide residues in archived UK soil. Environ. Sci. Technol. 35:1989-1995.
- MellstedtH., 1975. In vitro activation of human T and B lymphocytes by pokeweed mitogen. Clin. Exp. Immunol. 19:75-82.
- MooreP.D. YedjouC.G. TchounwouP.B., 2010. Malathion-induced oxidative stress, cytotoxicity and genotoxicity in human liver carcinoma (HepG2) cells. Environ. Toxicol. 25:221-226.
- MremaE.J. RubinoF.M. BrambillaG. MorettoA. TsatsakisA.M. ColosioC., 2013. Persistent organochlorinated pesticides and mechanisms of their toxicity. Toxicology 307:74-88.
- NeriA. ZoriniC.O. BrugiatelliM. PodagrosiD., 1973. Attivazione linfocitaria in vitro da concanavalina a. Res. Clin. Lab. 3:566-580.
- NikanorovA.M. KorotovaL.G. KlimenkoO.A., 2007. Estimation of long-term trends in the discharge of organochlorine pesticides into seas by rivers of Russia. Water Resour. 34:415-422.
- Olivero-VerbelJ. Guerrero-CastillaA. RamosN.R., 2011. Biochemical effects induced by the hexachlorocyclohexanes. Rev. Environ. Contam. T. 212:1-28.
- PayneJ. ScholzeM. KortenkampA., 2001. Mixtures of four organochlorines enhance human breast cancer cell proliferation. Environ. Health Persp. 109:391-397.
- Pérez-MaldonadoI.N. Dìaz-BarrigaF. de la FuenteH. Gonzàles-AmaroR. CalderònJ. YànezL., 2004. DDT induces apoptosis in human mononuclear cells in vitro and is associated with increased apoptosis in exposed children. Environ. Res. 94:38-46.
- PhillipsT.M. SeechA.G. LeeH. TrevorsJ.T., 2005. Biodegradation of hexachlorocyclohexane (HCH) by microorganisms. Biodegradation 16:363-392.
- Piskac-CollierA.L. SmithM.A., 2009. Lindane-induced generation of reactive oxygen species and depletion of glutathione do not result in necrosis in renal distal tubule cells. J. Toxicol. Env. Heal. A 72:1160-1167.
- RíosJ.C. RepettoG. JosA. del PesoA. SalgueroM. CameánA. RepettoM., 2003. Tribromophenol induces the differentiation of SH-SY5Y human neuroblastoma cells in vitro. Toxicol. In Vitro 17:635-641.
- RonchiB. DanieliP.P., 2008. Contamination by persistent chemical pesticides in livestock production systems. In: FayeB. SinyavskiyY. (eds.) Impact of pollution on animal products. Springer, Amsterdam, The Netherlands, pp 147-162.
- SafeS., 2005. Clinical correlates of environmental endocrine disruptors. Trends Endocrin. Met. 16:139-144.
- ScudieroD.A. ShoemakerR.H. PaullK.D. MonksA. TierneyS. NofzigerT.H. CurrensM.J. SeniffD. BoydM.R., 1988. Evaluation of a soluble tetrazolium/formazan assay for cell growth and drug sensitivity in culture using human and other tumor cell lines. Cancer Res. 48:4827-4833.
- ShimamuraT. TakamoriA. UkedaH. NagataS. SawamuraM., 2000. Relationship between the reduction of tetrazolium salt XTT and DNA strand breakage with aminosugars. J. Agr. Food Chem. 48:1204-1209.
- Skrbic’B. Durisic-MladenovicN., 2007. Principal component analysis for soil contamination with organochlorine compounds. Chemosphere 68:2144-2152.
- SteinmetzR. YoungP.C.M. Caperell-GrantA. GizeE.A. MadhukarB.V. Ben-JonathanN. BigsbyR.M., 1996. Novel estrogenic action of the pesticide residue β-hexachlorocyclohexane in human breast cancer cells. Cancer Res. 56:5403-5409.
- TiemannU., 2008. In vivo and in vitro effects of the organochlorine pesticides DDT, TCPM, methoxychlor, and lindane on the female reproductive tract of mammals: a review. Reprod. Toxicol. 25:316-326.
- TiemannU. KüchenmeisterU., 1999. Influence of organochlorine pesticides on ATPase activities of microsomal fractions of bovine oviductal and endometrial cells. Toxicol. Lett. 104:75-81.
- TiemannU. PöhlandR. SchneiderF., 1996. Influence of organochlorine pesticides on physiological potency of cultured granulosa cells from bovine preovulatory follicles. Theriogenology 46:253-265.
- ToppariJ. LarsenJ.C. ChristiansenP. GiwercmanA. GrandjeanP. GuilletteL.J. JegouB. JensenT.K. JouannetP. KeidingN. LeffersH. McLachlanJ.A. MeyerO. MullerJ. Rajpert-De MeytsE. ScheikeT. SharpeR. SumpterJ. SkakkebaekN.E., 1996. Male reproductive health and environmental xenoestrogens. Environ. Health Persp. 104:741-803.
- VairettiM. FerrignoA. BertoneR. RichelmiP. BertèF. FreitasI., 2005. Apoptosis vs. necrosis: glutathione-mediated cell death during rewarming of rat hepatocytes. Biochim. Biophys. Acta 1740:367-374.
- VijgenJ. AbhilashP.C. LiY.F. LalR. ForterM. TorresJ. SinghN. YunusM. TianC. SchäfferA. WeberR., 2011. Hexachlorocyclohexane (HCH) as new Stockholm Convention POPs: a global perspective on the management of Lindane and its waste isomers. Environ. Sci. Pollut. R. 18:152-162.
- VineM.F. SteinL. WeigleK. SchroederJ. DegnanD. TseC.J. BackerL., 2001. Plasma 1,1-Dichloro-2,2-bis(p-chlorophenyl)ethylene (DDE) levels an immune response. Am. J. Epidemiol. 153:53-63.
- WangS. YuH. WickliffeJ.K., 2011. Limitation of the MTT and XTT assays for measuring cell viability due to superoxide formation induced by nano-scale TiO2. Toxicol. In Vitro 25:2147-2151.
- YàñezL. Borja-AburtoV.H. RojasE. de la FuenteH. Gonzàlez-AmaroR. GòmezH. JongitudA.A. Dìaz-BarrigaF., 2004. DDT induces DNA damage in blood cells. Studies in vitro and in women chronically exposed to this insecticide. Environ. Res. 94:18-24.
- ZouE. MatsumuraF., 2003. Long-term exposure to β-hexachlorocyclohexane (β-HCH) promotes transformation and invasiveness of MCF-7 human breast cancer cells. Biochem. Pharmacol. 66:831-840.