Abstract
Background: Pretreatment of biomass at regional depots could simplify supply logistics for the emerging biofuels industry. Ammonia Fiber Expansion (AFEX™) could be an appropriate pretreatment method for use at depots, if the process can be simplified. Here we investigate pretreatment of corn stover and wheat straw using a simple packed bed AFEX system that exploits the high porosity of biomass to facilitate ammonia transport. Results & discussion: Steam stripping vertical packed beds allowed recovery of more than 90% residual ammonia as substantially dry vapor. AFEX-treated biomass gave enzyme hydrolysis yields equal to biomass treated in stirred batch reactors, and formed durable pellets. Conclusion: Packed bed AFEX shows significant promise as a biomass pretreatment for use at distributed depots.
COMPR: Compressor; COND: Condenser; EVAP: Ammonia evaporator; P: Pressure gauges; PSV: Pressure safety valve; RD: Rupture disks.
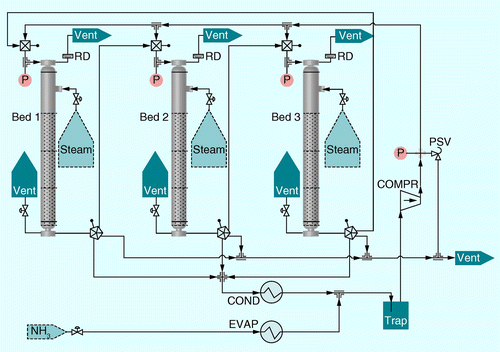
H: Reactor oriented horizontally; V: Reactor oriented vertically.
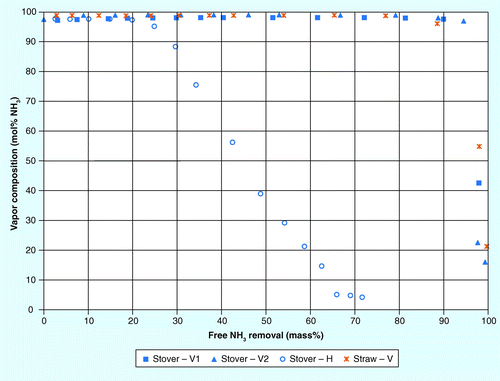
Error bars indicate minimum and maximum values of triplicates.
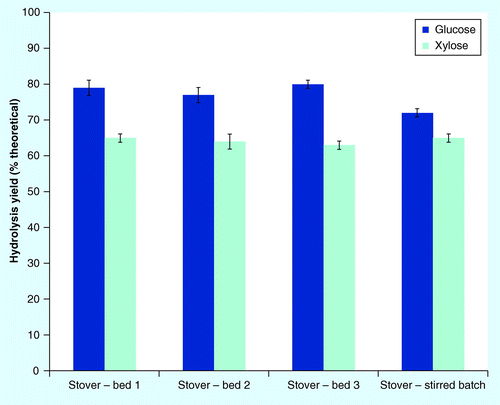
Error bars indicate minimum and maximum values of duplicates.
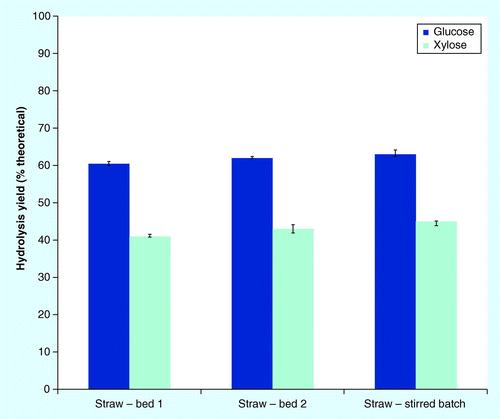
(A) corn stover, loose; (B) corn stover, pellets; (C) wheat straw, loose; (D) wheat straw, pellets. Pellets are 0.25 inches in diameter.
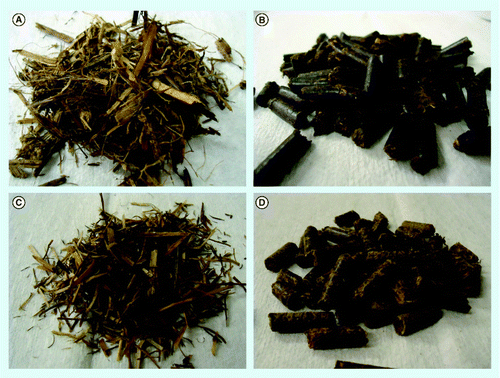
Agricultural residues such as corn stover and wheat straw offer enormous potential as renewable, domestic feedstocks for the production of liquid transportation fuels Citation[1]. In a conventional model for cellulosic biofuels production, raw biomass feedstock is transported directly from agricultural lands to large centralized biorefineries for conversion to fuels, in much the same way that corn grain is transported to dry-mill plants for conversion to ethanol Citation[2]. This centralized model of cellulosic biofuels production faces a number of significant challenges due to the differences between raw biomass and commodity feedstocks such as field corn. Low bulk density, variable composition, poor flowability and storage instability are some of the properties that will complicate handling logistics, and increase costs of transport and storage, for raw biomass feedstocks.
A biomass processing depot concept has been proposed as one way to reduce costs and overcome many of the logistic challenges of the lignocellulosic biomass feedstock supply chain Citation[3,4]. In this concept, raw biomass harvested from farmlands is transported relatively short distances to small-scale depots for processing into denser and more uniform stable commodities. Those commodity feedstocks can then be stored economically and transported readily over longer distances to large-scale biorefineries for conversion into biofuels and bio-based chemicals. Methods for pretreatment of biomass at depots may include mechanical treatments such as size reduction or pelletization, as well as chemical treatments. Chemical pretreatment of raw lignocellulosic biomass at regional depots can increase digestibility, enhance stability and facilitate densification. While the regional depot concept offers significant opportunities for improving the logistics and economics of the cellulosic feedstock supply chain, biomass pretreatment methods will not be practical at depots unless specific challenges are addressed. Pretreatment methods at distributed depots will necessarily be practiced at small scales, at which capital and labor costs per ton of biomass processed may be uneconomical. Chemical pretreatment at depot scales will require low-cost, mechanically simple equipment in order to keep capital and labor costs at viable levels.
Ammonia Fiber Expansion (AFEX™) is one method that could be used for chemical pretreatment of biomass at regional depots. AFEX treatment involves contacting biomass at moisture levels from 20 to 80 wt% with ammonia liquid or vapor in a pressure vessel to achieve ammonia:biomass weight ratio of 0.2–4 at temperatures from 40 to 120°C and pressures from 2 to 40 atm for treatment times ranging from 10 to 60 min, followed by release of ammonia vapor from the vessel, residual ammonia removal from the biomass by drying or steam stripping and recovery of the treated biomass. AFEX does not significantly hydrolyze hemicellulose to soluble sugars or oligomers, does not generate any liquid side streams and does not significantly alter the composition of the biomass. AFEX has been shown to be a highly effective pretreatment for corn stover Citation[5], and a wide range of other agricultural residues and energy crops, including Miscanthus, switchgrass and wheat straw Citation[6–8]. AFEX treatment has also been shown to mobilize lignin to act as a natural binder, facilitating densification of biomass Citation[101]. However, AFEX process designs that have been proposed for commercial-scale biomass treatment have used complex, costly operations such as plug screw feed and discharge devices Citation[102], continuous stripping columns for ammonia removal from treated biomass Citation[9] or arrangements of flash and quench tanks for ammonia recovery Citation[10]. As with similar process designs for dilute acid, steam explosion or other biomass pretreatments, these AFEX process designs may be suitable for large biorefinery scales, but are likely to be too costly and complex for biomass pretreatment at depot scale.
While the low bulk density of raw biomass presents a number of problems in transport and storage of lignocellulosic feedstocks, this low bulk density may also be exploited by forming highly porous packed beds that facilitate transport of vapor streams such as steam and ammonia. Packed bed bulk density ρBed and porosity εBed are inversely related as εBed (volume%) = 100 × (1 – ρBed / ρPart), where ρPart is biomass particle density Citation[11]. Agricultural residues such as corn stover and wheat straw, when ground to particle sizes of less than 1 inch, typically have particle densities greater than 1000 kg/m3 and loose-packed bulk densities less than 150 kg/m3, and so form beds with porosities greater than 85 vol% Citation[12]. The high porosities of biomass beds are routinely exploited in presteaming bins used to preheat and displace air from beds of wood chips prior to thermomechanical pulping Citation[103,104] and beds of agricultural residues such as wheat straw prior to steam explosion pretreatment Citation[13]. The high porosities of biomass packed beds, combined with the high moisture absorption capacities of many biomass types, means that packed beds of moist biomass can have very high capacity for absorption of water-soluble gases such as ammonia. One example of an application that exploits the high ammonia absorption capacity of moist biomass is the use of packed beds of agricultural residues such as sugarcane bagasse as ammonia biofilters Citation[14].
In this paper, we introduce a method for AFEX pretreatment of raw biomass that exploits the high porosities and ammonia absorption capacities of biomass packed beds in a mechanically simple process that may be suitable for depot operation. To establish the technical feasibility of the packed bed AFEX method, the effects of a cyclic batch process using presteaming followed by absorption of compressed ammonia vapor, then release of bed pressure and direct steam stripping to remove residual ammonia, on packed beds of corn stover and wheat straw, were investigated at laboratory scale. The effectiveness of this packed bed AFEX treatment for enhancing enzyme hydrolysis sugar yields and facilitating pellet formation were measured. The commercial potential for commoditization of raw biomass using the packed bed AFEX pretreatment at depot scale is discussed.
Experimental
▪ Corn stover, wheat straw sources & size reduction
Conventional multipass, low-cob corn stover was harvested and baled by Iowa State University (IA, USA) on 23 October 2011. The stover was sourced from a field located at the GPS coordinates (42.213953, -93.742377). Following grain harvest, the stover was windrowed using a Hiniker 5600 Series side discharge windrowing stalk chopper (MN, USA), and baled using a Massey Ferguson MF2170XD large square baler (Kenilworth, UK). The average bale weight was 420 kg. The bales were stored under tarps until milled for use. This material was then milled to pass through a 1 inch screen using a Vermeer BG 480 grinder prior to drying to less than 5% moisture. Mini bales of wheat straw labeled as Rhino EZ-Straw® (MI, USA) (weighing approximately 18 kg, with 5% moisture) were purchased from a local farm (Webberville, MI, USA). The wheat straw was ground to pass through a 1.2-inch screen using a Wiley® mill (Thomas Scientific, NJ, USA).
The composition of corn stover and wheat straw was analyzed using the laboratory analytical procedures developed by the National Renewable Energy Laboratory (CO, USA) and the data are presented in Citation[15].
Ground biomass at moisture content of approximately 5 wt% (total weight basis) was moistened to 25 wt% by spraying with deionized water, then packed into stainless steel baskets at bed density of 100 kg (dry) per m3. A photo of a basket packed with corn stover is shown in the Supplementary Figure 1 inset; three 12-inch long baskets were inserted into each reactor tube, as indicated in Supplementary Figure 2. Packing loose biomass into baskets for insertion into the bed tubes offered two advantages over simply dumping loose biomass in and out of the bed tubes. First, the use of baskets simplified transport of the treated biomass out of the reactor tubes. AFEX treatment mobilizes the lignin in raw biomass and the mobilized lignin acts as a natural binder, causing the fibers to stick together. The flowability of the treated biomass was inadequate to allow it to be dumped easily out of the bed tubes. The baskets, however, could be easily removed from the bed tubes, and then the treated biomass was readily removed from the baskets by flexing the mesh shell. The second advantage was the ability to control the compression of the biomass as it was packed into baskets. Packed bed AFEX requires bed porosity greater than 85 vol% to facilitate steam and ammonia vapor transport through the bed. Loose corn stover at an average 1-inch particle size typically has a bulk density of only 50 kg/m3, while wheat straw loose bulk density is only 51 kg/m3Citation[12]. Both corn stover and wheat straw at 1-inch particle size can be compressed to 100 kg/m3 bed density, allowing more biomass to be treated per bed cycle, without reducing the bed porosity below 85 vol%. The basket shells were fabricated by spot welding stainless mesh with 40 openings per inch (McMaster-Carr, Atlanta, GA, USA) into a cylindrical sleeve approximately 3.75 inches in diameter and 12 inches long, then welding the sleeve to a disc of 22 gauge perforated stainless steel sheet with 0.0625-inch diameter round holes, 41% open area (McMaster-Carr). A 0.25-inch diameter stainless steel threaded rod attached to the perforated sheet disc ran through the axis of the basket and was used to attach a second perforated sheet disk to the opposite end of the basket, forming a lid to hold the biomass in place. The basket shells fit closely to the inner diameters of the bed tubes, but were able to slide easily in and out of the tubes.
▪ Packed bed AFEX system
The packed bed AFEX reactor system was assembled on a portable skid, which supported three bed tubes (Supplementary Figure 1). Dimensions and ports of the three bed tubes are shown Supplementary Figure 2. In normal operation, the bed tubes were mounted vertically, with the inlet at the top and exit at the bottom; however, to investigate buoyancy effects during steam stripping, a bed of corn stover was treated with the bed tube mounted horizontally. Each individual bed tube was fabricated from 4-inch outer diameter stainless steel tube, wall thickness 0.085 inch, with sanitary ferrules welded to the tube at either end; overall length was 48 inches. Each bed tube had a 2-inch diameter side port located 5 inches from the top. At the top and bottom of each bed tube, a reducing ‘bell’ tee was connected by an ethylene propylene diene M-class rubber gasket, using a high-pressure bolted sanitary clamp. Each bell tee had two 1.5-inch diameter ports for connection to inlet and exit multiport valves, as well as vent valves. The three baskets containing the packed biomass were inserted into the bed tubes by removing the top bell tee. The top bell tee of each bed tube was fitted with a 1.5-inch diameter 300-psig rupture disk (Fike Corp., Blue Springs, MO, USA). Each bed tube and bell tee was insulated with a 1/16-inch thick coating of Temp-Coat 101 ceramic insulating coating (Temp-Coat® Brand Products LLC, LA, USA). Despite the coating of insulation, heat loss from the bed tubes to the surrounding air was substantial during the high-temperature steps of the AFEX treatment cycle. Bed tube temperatures were monitored using two surface-mounted resistance temperature detector elements (Omega Engineering, Inc., Stamford, CT, USA) adhered to the bare steel of the outer tube wall, one located 6 inches from the top and one 2 inches from the bottom of the tube. Bed tube inlet and exit ports were connected to multiport valves by 0.5-inch inner diameter flexible braided hose with compression fittings. Bed pressure was measured using a 0–250 psi gauge connected by means of a gauge tee to each bed inlet hose.
A schematic diagram showing the interconnection of the three bed tubes with the other system components is shown in . Anhydrous liquid ammonia (Tanner Industries Ltd, Philadelphia, PA, USA) for initial and makeup charging was delivered from a storage cylinder by means of a diaphragm pump (Lewa, Inc., Holliston, MA, USA) at a rate of 80–100 g per min. The liquid ammonia was vaporized at approximately atmospheric pressure in the inner tube of a 0.11 m2 tube-in-tube heat exchanger (Exergy LLC, Garden City, NY, USA) with saturated steam at less than 15 psig condensing in the outer tube Fresh ammonia vapor from the heat exchanger or vapor released from the treated biomass beds was compressed in a two-cylinder single-stage ammonia compressor with 10.5 m3 per h displacement (GEA Bock GmbH, Frickenhausen, Germany) . Vapor recovered from the biomass beds was dried on the suction side of the compressor by passing through the 5-inch diameter shell of a 1.5 m2 stainless steel shell-and-tube heat exchanger (ITT Standard, Buffalo, NY, USA) with industrial cold water passing through the 3/8-inch tubes Condensate exiting the heat exchanger was collected in a 1-l trap vessel. The compressor discharge manifold was connected to a 0.5-inch 250 psig relief valve (Leser LLC, Charlotte, NC, USA), with the relief port connected to vent
▪ Biomass treatment sequence
Baskets containing ground biomass were assembled into the reactor tubes and AFEX-treated using a cycle of five steps: presteam, ammonia charge, soak, depressurize and steam strip. For treatment of several beds in series, the ammonia charge, depressurize and steam strip steps involved transfer of ammonia vapor from a lead bed to a lag bed, and the lag bed presteaming step was timed to end just as the soak period of the lead bed was ending, so that depressurization and steam stripping of the lead bed coincided with ammonia charging to the lag bed. To minimize operator exposure to ammonia vapor, the packed bed AFEX skid was operated within a curtained ventilation area with adequate air volume turnover to rapidly dilute any fugitive vapors to below exposure limits. Detailed descriptions of the operating sequence steps are:
▪ Presteam – presteaming served to both preheat the biomass beds and displace entrained air. The biomass beds were presteamed by admitting steam at a rate of approximately 60 g per min to the top of the bed tube using a steam nozzle attached to the 2-inch diameter side port. During presteaming steam pressure at the top of the bed was less than 3 psig, and the bottom vent port valve of the tube was left open to the atmosphere to allow displaced air to escape from the bed. Less than 30 s after introducing steam to the bed, the bed tube top temperature reading increased to more than 80°C. Presteaming continued until the bottom temperature reached within 5°C of the top temperature, at which point steam flow was stopped and the bottom vent port valve was closed; | |||||
▪ Ammonia charge – following immediately after presteaming, the bed was charged with compressed ammonia to a load of 1 kg ammonia per kg dry biomass. Compressed vapor was charged to the biomass bed until a pressure of 200 psig was reached. The bed was then allowed to soak until the pressure had dropped below 160 psig, and then charged with compressed vapor back to 200 psig. This procedure was repeated until the target ammonia mass loading of 1 kg per kg of dry biomass was achieved. For initial or makeup charging, the vapor sent to the compressor suction was fresh ammonia from the evaporator, while for treatment of several beds in series, the vapor source was from the previous bed pressure release and steam stripping; | |||||
▪ Soak – following ammonia charging, beds were allowed to soak for 30 min. During the soak period, the bed pressure typically dropped below 110 psig, due both to ammonia vapor permeation into the moist biomass and heat loss from the bed to the surrounding air; | |||||
▪ Depressurize – at the end of the soak period, the bed exit valve was slowly opened to release vapor from the bed. The vapor released from bed depressurization was sent to the presteamed lag bed, until the two bed pressures were equal, at which point the lead bed was pulled down to atmospheric pressure using the compressor suction, with the compressed vapor sent to the lag bed. Alternatively, if the bed being treated was the last in the series, the vapor from depressurization was simply vented until the bed reached atmospheric pressure; | |||||
▪ Steam strip – approximately one half of the ammonia charge remained on the bed after depressurization. This residual ammonia was removed from the bed by direct steam stripping. As in presteaming, steam was admitted to the top of the bed at a rate of approximately 60 g per min using a steam nozzle attached to the 2-inch diameter side port. The vapor driven off of the bottom of the bed was sent through the condenser and trap to the compressor suction, and the compressed vapor sent to the next bed in series. Steam stripping was stopped intermittently to allow the lag bed pressure to drop from 200 psig to below 160 psig. Steam stripping was stopped when the bed tube bottom temperature had climbed to within 10°C of the top temperature, which was typically greater than 80°C. For the last bed in a series, the vapor from steam stripping was vented to atmosphere. |
Following bed-to-bed ammonia transfer, makeup ammonia was added to the lag bed as part of its ammonia charging step, to compensate for residual ammonia not removed from the lead bed, and for ammonia lost as condensate collected in the compressor suction trap. Based on the measured ammonia mass balance and measurements of the condensate volume (data not shown), it was calculated that 2% of the ammonia charge was not removed by steam stripping, and that 8% of the ammonia charge was recovered as condensate; therefore, 10% makeup ammonia was added to each bed in series after the initial bed. Note that while the 2% ammonia not removed by steam stripping represents ammonia bound to the biomass, the 8% in the condensate is recoverable by purging, although that was not done in these experiments. The 2% of the ammonia charge bound to the biomass is available as a nitrogen source for subsequent downstream fermentation of sugars liberated from the biomass by enzyme hydrolysis.
▪ Ammonia recovery procedure
A set of experiments were conducted to measure the quantity and composition of ammonia vapor removed from beds of corn stover and wheat straw during steam stripping. In these experiments, beds of biomass were treated as described in steps 1–4 above. The beds were presteamed, charged with fresh ammonia (not ammonia recovered from another bed) then allowed to soak for 30 min, and then the pressure released to vent. A resistance temperature detector probe (Wahl Instruments, Inc., Culver City, CA, USA) was then inserted into the bottom bell tee of the tube, with the sensing element located less than 4 inches from the bottom of the lowest basket in the bed. A flexible hose was connected to the bottom bell tee, with the other end of the hose immersed in a 1-l portion of 0.5 molar citric acid. As steam stripping proceeded as described above, the vapor exiting the bed was trapped in the citric acid for 1 min, then the hose was switched to a second portion of 0.5 molar citric acid and the temperature of the vapor was recorded. In this way, 1-min fractions of stripped vapor were trapped in citric acid. When the steam stripping was complete, the pH of the fractions were measured using a standardized probe, and the ammonia mass content of each fraction determined by comparing the pH to an ammonia/citric acid titration curve. The composition of the ammonia–water vapor mixture was determined from the temperature recorded for each fraction by interpolating from a table of saturated ammonia–water vapor properties Citation[16].
▪ AFEX treatment in batch stirred reactor
To provide a performance benchmark, corn stover and wheat straw were treated in a 1-gallon pressure reactor as described previously Citation[17]. The process conditions were 60% moisture, reaction time of 30 min, temperature at 90°C and ammonia loading at 1 g of ammonia per g dry biomass. Temperature and concentration gradients were minimized in this small agitated vessel so that the biomass charge was uniformly treated under the AFEX conditions, providing a useful benchmark for comparison to the packed bed AFEX reactors.
▪ Enzyme hydrolysis procedure
The performance of the packed bed AFEX process in treatment of corn stover and wheat straw was evaluated via enzyme hydrolysis. The enzymatic digestibility of AFEX-treated biomass was determined via enzymatic hydrolysis at low solid loading Citation[6]. The hydrolysis test was carried out in Erlenmeyer flasks and the conditions were 50°C, pH 4.8 (0.05 M sodium citrate buffer), 3% solid loading (equivalent to 1% glucan loading) and agitated at 150 rpm in a constant temperature incubator shaker for 72 h. Combination of cellulase (Cellic Ctec2, provided by Novozymes), hemicellulase (Cellic Htec2, provided by Novozymes) and Multifect Pectinase (provided by Genencor®) was used in the enzymatic digestibility tests. Samples of all enzymes were sent to ServiTech laboratory (Hastings, NE, USA) for crude protein analysis (based on total nitrogen).
One of the unique features of the AFEX process is that the cellulose, hemicellulose and lignin present in the biomass are preserved and the composition of the biomass before and after the treatment is essentially the same. Therefore, composition of the untreated samples was used in enzymatic digestibility calculations. The required amount of enzyme was calculated based on 30 mg of enzyme protein (Ctec2 plus Htec2 plus pectinase) per gram of glucan. The enzyme cocktail containing 30 mg protein enzyme per gram of glucan consisted of 70% Ctec2, 15% Htec2 and 15% pectinase. In each set of experiments, appropriate controls were run to account for the amount of sugars that came with the enzyme cocktails. Hydrolysis yields of glucose and xylose were determined via high-performance liquid chromatography using a Bio-Rad Aminex® 87P carbohydrate column (Hercules, CA, USA), and calculated as percent of theoretical yield based on the composition of the biomass .
▪ Pelletization procedure
After packed bed AFEX treatment, biomass was pelletized using a Buskirk Engineering (Ossian, IN, USA) flat die pellet mill. Both PM605 and PM810 model pellet mills were used with 0.25-inch pore size. Untreated switchgrass was processed first in order to warm the die and roller up to a temperature of at least 75°C. After the temperature was reached, packed bed AFEX-treated biomass at the desired moisture content and particle size was added manually to the pellet mill. Pellet temperature was measured as the pellets were exiting the mill using an infrared sensor. Pellets were collected in a 20-l plastic bucket and allowed to cool on a perforated metal tray. If necessary, pellets were dried overnight in a 50°C convection oven (Blue M Electric Company Class A Batch Oven, Blue Island, IL, USA) to prevent spoilage. The power consumption of the pellet mill was not measured. Accurate measurement of the electrical load of the Buskirk mill requires steady-state operation over extended periods of time, which consumes large quantities of treated biomass. We intend to make these load measurements using biomass treated in the pilot-scale packed bed AFEX system, which will be reported in a future paper.
Pellet properties were measured after the pellets were dried and cooled to room temperature. Moisture content was measured by placing a preweighed sample of pellets in a 105°C oven overnight and measuring the weight loss. Bulk density was measured by filling a 2-l beaker with pellets and measuring the total weight of pellets added and compensating for the moisture content. Pellet durability was measured via the American Society of Agricultural Engineers 269.4 standard using a Seedburo pellet durability tester (Seedburo Equipment Company, Des Plaines, IL, USA) Citation[18]. Briefly, 500 g of pellets were tumbled in a metal box at 50 rpm for 10 min and then sieved through a #10 mesh to remove any generated fines. The percentage of total biomass that remained in the sieve was determined and recorded as the pellet durability index.
Results & discussion
▪ General process results
Process conditions during the packed bed AFEX cycle varied only slightly from bed to bed. Starting with biomass at 20–25% moisture at 22–25°C, the presteaming step increased bed moisture to 35–40% and temperature to 80–85°C. At the end of ammonia charging, pressure was 200 psig, top temperature was 35–40°C and bottom temperature was 70–80°C. During the soak period, significant heat was lost from the beds to the surrounding air. By the end of the soak period, top and bottom bed temperatures typically had dropped to 35–40°C. Depressurization dropped the bed pressure to 0 psig, top temperature to 30–35°C and bottom temperature to 10–20°C. By the end of steam stripping, top and bottom temperatures were 80–90°C and moisture was 55–60%.
▪ Ammonia recovery results
During bed depressurization only approximately half of the ammonia charge was released as vapor. During depressurization, the ammonia concentration of the absorbed liquid dropped, while the endotherm of vaporization cooled the bed, until the saturation pressure of the absorbed liquid reached atmospheric. At that point, approximately half of the ammonia charge still remained as residual absorbed ammonia, and steam was added during the steam stripping step to drive further vaporization so that the residual ammonia could be recovered. As shows, more than 90% of the residual free ammonia was recovered as substantially dry vapor having greater than 90% ammonia content, from vertical beds of both corn stover and wheat straw (Stover – V1 and – V2 and Straw – V in ). Only during removal of the last 10% of free ammonia did the composition of the vapor drop below 90%. In contrast, during steam stripping of a horizontally oriented corn stover bed (Stover-H), less than half of the free ammonia was removed as substantially dry vapor.
The significant difference in steam stripping efficiency between horizontal and vertical beds is evidence of a buoyant effect in the stripping process. Supplementary Figure 3 shows compositions and densities of ammonia–water vapor mixtures at atmospheric pressure over the range of temperatures encountered during ammonia steam stripping from biomass packed beds. As the vertical beds were stripped, steam entered the top of the bed and condensed on the cold biomass, liberating heat to generate vapor. The density of saturated steam at atmospheric pressure is indicated for reference by the dashed line in Supplementary Figure 3. As cold, ammonia-rich vapor is released from the bed, that vapor is denser than the incoming steam. The buoyancy effect due to the density difference between the steam entering the top of the bed and the vapor exiting the bottom of the bed increases the efficiency of the stripping process by segregating the steam and ammonia vapor, so that only substantially dry vapor leaves the bed. When the bed is oriented horizontally, this beneficial buoyancy effect is lost. The incoming steam penetrates rapidly across the top of the horizontal bed and quickly breaks through to the bed exit, so that more than half of the residual ammonia is recovered as wet vapor. Recovery of ammonia as wet vapor is less efficient than recovery as dry vapor, both because more steam is required to completely strip the bed and because more ammonia must be condensed to dry the vapor before recompression. The condensed wet ammonia can be recovered by steam purging the condensate, but purging requires additional energy. Recovery of ammonia as substantially dry vapor suitable for recompression from vertical packed beds, as shown in , significantly simplifies the AFEX process compared with conventional AFEX designs. Direct recompression of the dry vapor stripped from a lead bed, coupled with the absorption of that compressed vapor on a lag bed, eliminates the need for an ammonia recovery dryer or column, or arrangements of ammonia flash and quench tanks, as required in conventional designs described in the literature Citation[9,10,102]. This reduction in equipment requirements yields significant reduction in the capital cost of the system, particularly at the smaller scales anticipated for biomass processing depots. Our preliminary analysis of a depot processing 100 tons of corn stover per day shows that greater than 50% reduction in capital cost can be realized using a packed bed system in place of a conventional AFEX design. Note that during the packed bed AFEX cycle, the compressor operates only intermittently, during bed-to-bed ammonia transfer and, consequently, the cost associated with the compressor electrical load is not a significant component of the overall treatment cost.
▪ Enzyme hydrolysis results
Enzyme hydrolysis yields of glucose and xylose from corn stover and wheat straw treated in sequential packed bed AFEX reactors with bed-to-bed transfer of ammonia are shown in . For reference, sugar yields from stover and straw treated in the benchmark stirred batch reactor are also shown. Both stover and straw were as effectively pretreated in the packed bed process as in the stirred batch. As described above, temperature gradients from top to bottom of the beds were observed during ammonia charge and soak steps of the packed bed AFEX cycle, while temperature and concentration gradients were minimized in the stirred batch. Because the vertical beds were charged from the top down, the ammonia concentration was probably highest at the top, where the temperature was lowest, while ammonia concentration was lower at the bottom of each bed, where the temperature was the highest. These two gradients may have compensated for each other, so that when the biomass beds were unpacked and mixed, the composite was uniformly treated, and the hydrolysis results were as good as the stirred batches. It is unclear at this time how this behavior will change as the packed bed AFEX process is scaled up to greater bed lengths. Comparing the stover – bed 1, bed 2 and bed 3 results in , the glucose and xylose yields are indistinguishable from bed-to-bed, indicating that sequential beds are treated approximately equally as the ammonia charge is moved from bed-to-bed. More work will be needed to demonstrate that this trend can be continued over longer bed sequences.
In preliminary experiments, enzyme hydrolysis of packed bed AFEX-treated and pelletized stover and straw also gave good yields of glucose and xylose, similar to the yields shown in . Enzyme hydrolysis of the AFEX-treated corn stover pellets at 18 wt% solids loading have shown that glucose and xylose concentrations of 56 and 28 g/l, respectively, can be obtained without increasing enzyme load or hydrolysis time.
Comparing , it is clear that both glucose and xylose yields were significantly lower from wheat straw than from corn stover. Note that in these hydrolyses, Multifect pectinase was not added and the enzyme cocktail (containing 30 mg of protein per gram of glucan) consisted of 70% Ctec2 and 30% Htec2. With the use of an optimized enzyme cocktail, including addition of pectinase, it may be possible to improve the sugar yields from AFEX-treated straw. Even without the use of optimized enzyme cocktails, the hydrolysis yields from both stover and straw were high enough to demonstrate that packed bed AFEX is an effective pretreatment method for production of biofuels and bio-based chemicals from these agricultural residues. We did not compare enzyme hydrolysis results of pelletized versus nonpelletized AFEX-treated material in this paper. While the performance of the pelletized material in enzyme hydrolysis will be a key factor in conversion to biofuels and chemicals, this topic deserves a full paper by itself, and is currently under investigation.
▪ Pelletization results
One major advantage of using AFEX pretreatment in a depot setting is that pelletization can be performed more efficiently due to the tackiness of the treated biomass, which results from the redistribution of lignin. This is partially seen by the range of moistures and particle sizes at which AFEX-treated material can be pelletized. AFEX-treated wheat straw and corn stover were pelletized at moisture contents ranging between 11 and 50%. In contrast, untreated material would only pelletize at a narrow range of moisture, approximately 15–20%. This expanded range provides greater flexibility at the depot, enabling the depot to produce pellets with different properties or allowing drying to be performed either before or after pelletization. Different properties may be desired for biofuel purposes versus animal feed, for example. AFEX-treated biomass pelletized at low moisture formed very hard pellets with a dark, shiny, smooth outer surface, as seen in . AFEX-treated biomass pelletized at higher than 25% moisture content did not have this hard, shiny outer layer, nor did untreated material. Fungal growth was observed on material pelletized at higher than 35% moisture and not dried. In contrast, no fungal growth was observed over a period of 3 months on pellets that were stored at less than 20% moisture. As with corn grain, AFEX-treated biomass may be stored at low moisture in silos for extended periods without significant degradation, microbial growth or mass loss. We expect that material AFEX-treated and pelletized at 25% moisture or less can be successfully stored without further drying.
AFEX treatment also tended to improve the properties of the pellets, as seen in . Pellet durability was above 90% for all pellets produced except wheat straw at low (12%) moisture content. Furthermore, AFEX tended to improve pellet durability over untreated samples. Both wheat straw and corn stover pellets were more than 99% durable when AFEX-treated, compared with 94–96% durable for untreated pellets. The high durability of AFEX-treated pellets means they are suitable to be stored, handled and shipped without producing many fines. Bulk density of AFEX-treated pellets were also high, reaching 575 kg/m3 for corn stover pellets that were produced from dry material. This approaches the bulk density of corn grain, which is approximately 700 kg/m3. When pelletizing wet material, the excess moisture may prevent pore collapse, thus maintaining a relatively low bulk density when dried. AFEX-treated corn stover pellets had higher density than untreated pellets at similar moisture contents, 444–575 kg/m3 for coarsely milled corn stover at 18–20% moisture content, but no difference in density was observed for wheat straw pellets.
Conclusion
Chemical pretreatment of corn stover and wheat straw using a laboratory-scale packed bed AFEX reactor system was investigated. The results show that the cycle of presteam, ammonia charge, soak, depressurize and steam strip steps could be repeated with ammonia transfer from bed-to-bed achieved by mechanical recompression of the recovered vapor. More than 90% of the residual ammonia removed during the steam stripping step could be recovered as substantially dry vapor when the bed was oriented vertically. This efficient steam stripping performance may be due to a buoyancy effect driven by the density difference between the steam and the colder stripped vapor. Temperature gradients were observed over the length of the bed during the ammonia charge and soak steps. Despite these gradients, the glucose and xylose enzyme hydrolysis yields from the composited biomass recovered from the beds were equivalent to those obtained from stover and straw that were AFEX-treated in a stirred batch reactor. The corn stover and wheat straw treated in the packed bed AFEX system formed durable pellets when densified in a conventional pellet mill without added binders. Pellets with good durability were formed from AFEX-treated corn stover at 40% moisture, while untreated stover at this moisture did not pelletize. Based on the efficient ammonia recovery with minimal equipment requirements, good enzyme hydrolysis yields and durable pellet formation observed, the packed bed AFEX approach shows significant promise for chemical pretreatment of corn stover and wheat straw at regional depots.
Future perspective
The scalability of the packed bed AFEX biomass treatment will be critical to commercial deployment of the technology. To prove scalability, MBI (Lansing, MI, USA) is presently working to design and build a pilot-scale packed bed AFEX reactor system that will treat 40–50-times as much biomass per bed as the laboratory-scale system described in this paper. The pilot-scale beds will be approximately 17 inches in diameter and 108 inches in length. Bed length is a key parameter to be investigated at pilot scale, as bed compression with loss of porosity may impact performance at larger scales. This pilot-scale system is scheduled to be operational by early 2013. Data obtained from operation of the pilot-scale packed bed reactor system will be used to design a commercial-scale facility suitable for biomass pretreatment at regional depots. It is anticipated that the commercial depot-scale reactors will treat approximately 40-times as much biomass per bed as at pilot scale, and a pair of depot-scale reactor vessels will treat approximately 50 tonnes of biomass per day. Environmental compliance issues associated with AFEX treatment of biomass at regional depots will also be investigated at pilot scale. The amount of ammonia released to the atmosphere during operation of the laboratory-scale skid is very small; this will be validated by operation of the pilot-scale system. In rural areas, where depots are most likely to be located, release of small quantities of ammonia during storage and handling is not normally regulated. A technoeconomic analysis based on the depot-scale design will be used to estimate the viability of packed bed AFEX pretreatment of agricultural residues at regional depots to produce commodity feedstocks. The most significant factors in determining viability will be capital, labor and ammonia costs, as well as the expected market price for the AFEX-preconverted biomass commodity. Capital and labor costs will be minimized by maximizing the system throughput, while ammonia cost will be minimized by maximizing ammonia recovery. The market price of the preconverted commodity product will depend in part on the number of markets the commodity can be sold into. A product that meets defined specifications for properties such as composition, storage stability, digestibility, pellet durability and flowability, will likely support a higher market price than a similar product for which these properties are unknown.
AFEX-treated biomass may have several end uses. In addition to increasing enzyme hydrolysis yields, AFEX treatment has also been shown to increase rumen digestibility of forage materials, including corn stover Citation[8]. However, enzyme hydrolysis and fermentation of AFEX-treated biomass has not yet been demonstrated at pilot-scale, and large-scale animal feed trials with AFEX-treated forage materials have yet to be conducted. In 2013, MBI plans to use the pilot-scale packed bed AFEX reactor system to produce the multi-ton quantities of AFEX-treated, pelletized corn stover needed to complete both pilot-scale enzyme hydrolysis and fermentation use tests, and beef cattle feed trials. Further investigation into high-solids enzyme hydrolysis and fermentation of packed bed AFEX-treated and pelleted biomass is presently ongoing. Deployment of the packed bed AFEX technology at regional depots to produce animal feed materials may offer a shorter path to commercialization than production of biofuels feedstocks because large animal feed markets are already available, while a cellulosic biorefinery industry may take time to develop. Widespread deployment of regional depots producing AFEX-treated biomass for animal feed may mean that future biorefinery projects will be less risky and easier to finance, as preconverted commodity feedstocks will already be available. In this way, adoption of the packed bed AFEX technology for biomass pretreatment at regional depots could help to resolve the ‘chicken-and-egg’ conundrum that is currently a barrier to the development of a US bioeconomy. The opportunity for regional depots to sell commodity products into more than one market may increase the financial stability of the depots, offering farm communities a way to add significant value to crop residues, thereby increasing economic stability and facilitating rural development.
Table 1. Ash and carbohydrate composition of corn stover and wheat straw (based on dry weight)†.
Table 2. Bulk density and durability of pellets produced at a variety of conditions.
Use of chemicals to reduce recalcitrance, aid densification, enhance storage stability or otherwise preprocess biomass before conversion to biofuels or bio-based chemicals.
Facility where biomass from nearby farmlands or forests may be densified, stabilized, preprocessed and stored, before shipment to distant biorefineries or other end users.
Biomass processing to increase density, including operations such as size reduction, pelletization or briquetting.
Alkaline biomass pretreatment developed by Bruce Dale of Michigan State University (MI, USA). AFEX™ is a trademark of MBI.
Results & discussion
▪ Packed bed Ammonia Fiber Expansion (AFEX™) is an effective pretreatment for agricultural residues such as corn stover and wheat straw. | |||||
▪ Ammonia recovery by steam stripping vertical packed beds of AFEX-treated biomass is a simple and efficient process, with only 2% of the ammonia charge lost to the biomass, and more than 90% of the ammonia recovered as substantially dry vapor that is suitable for mechanical recompression and re-use. | |||||
▪ Enzyme hydrolysis yields of glucose and xylose from packed bed AFEX-treated corn stover and wheat straw were equivalent to those treated in a benchmark stirred batch reactor. | |||||
▪ Corn stover and wheat straw treated in the packed bed AFEX process are readily densified by pelletization, without added binders. |
Conclusion
▪ Packed bed AFEX is a mechanically simple cyclic batch process that shows significant promise for economical pretreatment of agricultural residues at regional depots. |
tbfu_a_10816028_sm0001.doc
Download MS Word (4.8 MB)Acknowledgements
MBI holds the exclusive license to distribute the Ammonia Fiber Expansion (AFEX™) technology. AFEX is a trademark of MBI. The authors thank D Thompson and co-workers at Idaho National Laboratory (ID, USA) for providing the milled corn stover used in this study.
Disclaimer
This report was prepared as an account of work sponsored by an agency of the US Government. Neither the US Government nor any agency thereof, nor any of their employees, makes any warranty, express or implied, or assumes any legal liability or responsibility for the accuracy, completeness, or usefulness of any information, apparatus, product, or process disclosed, or represents that its use would not infringe privately owned rights. Reference herein to any specific commercial product, process, or service by trade name, trademark manufacturer or otherwise does not necessarily constitute or imply its endorsement, recommendation, or favoring by the US Government or any agency thereof. The views and opinions of authors expressed herein do not necessarily state or reflect those of the US Government or any agency thereof.
Financial & competing interests disclosure
This material is based upon work supported by the US Department of Energy, Golden Field Office, under Award No. DE-EE0005071 and Subaward No. FAR-0018581 under North Dakota State University Prime Award No. R-010-022 from the North Dakota Industrial Commission. The authors have no other relevant affiliations or financial involvement with any organization or entity with a financial interest in or financial conflict with the subject matter or materials discussed in the manuscript apart from those disclosed.
No writing assistance was utilized in the production of this manuscript.
References
- Perlack R, Wright LL, Turhollow AF, Graham RL, Stokes BJ, Erbach DC. Biomass as Feedstock for a Bioenergy and Bioproducts Industry: The Technical Feasibility of a Billion Ton Annual Supply. Oak Ridge National Laboratory, Oak Ridge, TN, USA (2005).
- Humbird D, Davis R, Tao L et al.Process Design and Economics for Biochemical Conversion of Lignocellulosic Biomass to Ethanol: Dilute-Acid Pretreatment and Enzymatic Hydrolysis of Corn Stover. National Renewable Energy Laboratory, Golden, CO, USA (2011).
- Hess JR, Kenney KL, Park L, Searcy EM, Wright CT. Uniform-Format Solid Feedstock Supply System: A Commodity-Scale Design to Produce an Infrastructure-Compatible Bulk Solid from Lignocellulosic Biomass. Idaho National Laboratory, Idaho Falls, ID, USA (2009).
- Eranki PL, Bals BD, Dale BE. Advanced regional biomass processing depots: a key to the logistical challenges of the cellulosic biofuel industry. Biofuels Bioprod. Bioref.5(6),621–630 (2011).
- Teymouri F, Laureano-Perez L, Alizadeh H, Dale BE. Optimization of the ammonia fiber explosion (AFEX) treatment parameters for enzymatic hydrolysis of corn stover. Bioresour. Technol.96(18),2014–2018 (2005).
- Murnen KH, Balan B, Chundawat SH, Bals BD, Costa Sousa L, Dale BE. Optimization of ammonia fiber expansion (AFEX) pretreatment and enzymatic hydrolysis of Miscanthus x giganteus to fermentable sugars. Biotechnol. Prog.23(4),846–850 (2007).
- Alizadeh H, Teymouri F, Gilbert TI, Dale BE. Pretreatment of switchgrass by ammonia fiber explosion (AFEX). Appl. Biochem. Biotechnol.121–124,1133–1141 (2005).
- Bals BD, Murnen H, Allen M, Dale BE. Ammonia fiber expansion (AFEX) treatment of eleven different forages: improvements to fiber digestibility in vitro. Anim. Feed Sci. Technol.155(2–4),147–155 (2010).
- Sendich E, Laser M, Kim S et al. Recent process improvements for the ammonia fiber expansion (AFEX) process and resulting reductions in minimum ethanol selling price. Bioresour. Technol.99(17),8429–8435 (2008).
- Laser M, Jin H, Jayawardhana K, Lynd LR. Coproduction of ethanol and power from switchgrass. Biofuels Bioprod. Bioref.3(2),195–218 (2009).
- Zhou B, Ileleji KE, Ejeta G. Physical property relationships of bulk corn stover particles. ASABE Trans.51(2),581–590 (2008).
- Mani S, Tabil LG, Sokhansanj S. Grinding performance and physical properties of wheat and barley straws, corn stover and switchgrass. Biomass Bioenergy27(4),339–352 (2004).
- Fang H, Deng J, Zhang X. Continuous steam explosion of wheat straw by high pressure mechanical refining system to produce sugars for bioconversion. BioResources6(4),4468–4480 (2011).
- Gangagni Rao A, Gandu B, Swamy YV. Mass transfer of ammonia in high rate biomethanation of poultry litter leachate. Bioresour. Technol.109,234–238 (2012).
- Sluiter JB, Ruiz RO, Scarlata CJ, Sluiter AD, Templeton DW. Compositional analysis of lignocellulosic feedstocks: 1. Review and description of methods. J. Agric. Food Chem.58(16),9043–9053 (2010).
- Tillner-Roth R, Friend DG. A Helmholtz free energy formulation of the thermodynamic properties of the mixture {water + ammonia}. J. Phys. Chem. Ref. Data27(1),63–96 (1998).
- Hanchar RJ, Teymouri F, Nielson CH, McCalla D, Stowers MD. Separation of glucose and pentose sugars by selective enzyme hydrolysis of AFEX-treated corn fiber. Appl. Biochem. Biotech.136–140(1–12),313–325 (2007).
- Temmerman M, Rabier F, Jensen PD, Hartmann H, Bohm T. Comparative study of durability test methods for pellets and briquettes. Biomass Bioenergy30(11),964–972 (2006).
▪ Patents
- Dale BE, Ritchie B, Marshall D: US046525 (2011).
- Holtzapple M, Davison R, Stuart D: US5171592 (1992).
- Kirov VH, Sethy A, Sorensen BL: US7771565 (2010).
- Sabourin M: US0085505 (2012).