Abstract
A significant proportion of anthropogenic GHG-generating activities are concentrated in cities. As centers of high consumption, wealth and creativity, cities must play a significant role in tackling climate change. Action to reduce emissions at a local level requires that municipal and local governments have a good understanding of emissions sources and reduction potentials. To achieve this, municipal governments require adequate tools and resources to enable effective policy decision making. The carbon footprint is becoming an increasingly recognized tool for the management of climate change. The term carbon footprint originated in the ‘gray literature’, and it is widely recognized in the public arena. It offers the opportunity to municipal governments to develop models to inform climate change strategy decision making, and enables municipal authorities to localize the issue of climate change and promote the benefits of climate change mitigation at the local level. Existing framework guidance often fails to include all relevant emissions or follow widely varying methodologies, limiting comparability. This article examines the concept of climate change localization and management. The carbon footprint is explored in the context of a tool for municipal government management of GHG emissions. We conclude by suggesting that the carbon footprint become a cost-effective, practical and repeatable metric that can be adopted by municipal governments across the globe as a ‘baseline’ indicator.
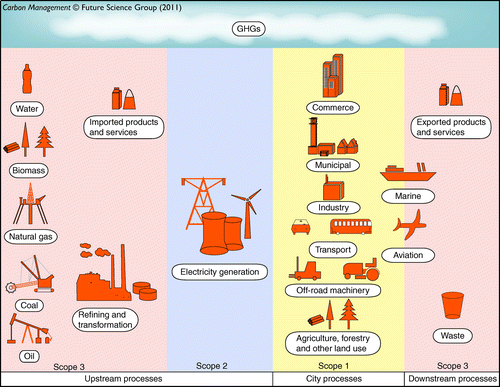
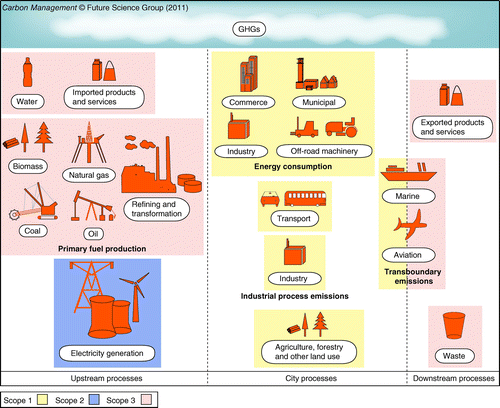
The threat posed by global climate change requires international action that includes commonly agreed approaches for the measurement and management of GHG emissions, target setting and the development of climate change mitigation measures. Cities are areas of high consumption and population density, often responsible for a plethora of environmental problems Citation[1]; more than two-thirds of the world’s energy was consumed in cities in 2006 Citation[2] and at least half of the world’s population now lives in urban areas, with the numbers of mega cities (populations greater than 10 million) increasing rapidly Citation[3]. As a consequence, the activities within cities and management of cities are disproportionately important in terms of global climate change policy-making.
Local and regional governments are responsible for many decisions that affect climate change management in cities, including transport and land-use planning, zoning and setting building standards as well as the management of their own activities Citation[4,5]. These governments must play a central role in reducing GHG emissions. In order for this to happen, a better understanding of local responsibilities in relation to national targets must be developed Citation[6]. This requires the localization of national climate change legislation and targets. Political emphasis has traditionally focused on global solutions negotiated through agreements such as the Kyoto Protocol Citation[7] and national legislation such as the UK’s Climate Change Act 2008 Citation[8]. A shift to subnational, regional and municipal climate change governance has emerged in recent years, giving rise to programs such as Cities for Climate Protection (CCP) Citation[9].
In pursuit of climate change localization, the concept of the ‘carbon footprint’ is increasingly being recognized as a valuable tool in GHG emissions management. Despite its proliferating use, there is still little consensus in the academic community as to what it actually means Citation[10]. Following a previous review, we understand the carbon footprint as a life cycle climate change metric: “A measure of the total amount of CO2 and CH4 emissions of a defined population, system or activity, considering all relevant sources, sinks and storage within the spatial and temporal boundary of the population, system or activity of interest. Calculated as CO2 equivalents using the relevant 100-year global warming potential” Citation[10]. The development of the carbon footprint was driven largely by organizations, and as a result, the majority of research has focused on methods for the calculation of product carbon footprints, organizational carbon footprints, and individual carbon footprints. Interest in the carbon footprints of cities is increasing Citation[4,6,11–13]. Carbon footprinting provides policy makers with a mechanism for the localization of climate change. Carbon footprints can provide an uncomplicated, low-cost solution, which is ideal for widespread uptake in the public and governmental sectors. A more comprehensive metric for the assessment of all GHGs may be required in some circumstances; where complete data is obtainable it can be used to provide a ‘climate footprint’ Citation[10]. This name reflects the addition of noncarbon-based gases and encompasses the full range of gases used in the global political community’s response to managing climate change. As with other forms of carbon footprinting, city carbon footprinting must be as accurate as reasonably possible to track progress and to form the basis of future carbon taxation and pricing schemes Citation[5], whilst still considering the time and financial constraints often placed on municipal governments.
In many ways, a city can be considered to be similar to a large incumbent organization, utilizing the same principles developed for the carbon footprinting of organizations Citation[13]. City-based footprints often include direct emissions and emissions from electricity. Less common is the inclusion of further production chain emissions owing to data collection complications and difficulties in boundary assignment. The capacity of urban areas for carbon sinks and storage may be significant, in which case there is a strong argument to additionally report sinks and storage within the system boundary.
Boundary definition presents a significant issue, cities do not just serve residents, but have strong involvement at both national and international scales.
There is increasing interest in the carbon footprint of regions, local areas and cities (e.g., New York Citation[14] and London Citation[15]), although the cataloging process is yet to mature Citation[11]. The models vary considerably in complexity, boundary conditions and emissions sources included in an estimation, making comparison of the overall carbon footprint between cities difficult or, at worst, invalid. Successful achievement of emission targets depends on the availability of high-quality data, which implies transparency, consistency, completeness and accuracy Citation[16]. However, whilst voluntary frameworks have been developed, no internationally accepted standard methodology currently exists providing detailed guidance for the collection of a city-based carbon footprint Citation[1]. The development of a standardized methodology requires consistent boundary selection, driven by clear, defined responsibility and emissions allocation, and methods to be utilized for the calculation of the urban carbon footprint between cities.
This article aims to critically evaluate the carbon footprint as a potential tool for management of emissions at a municipal level, and discuss key methodological issues arising for urban carbon footprinting, including boundary selection, allocating responsibility for emissions and treatment of carbon sinks.
Cities & GHG management
The 1987 Bruntland Report included a specific chapter on the environmental issues facing cities, arguing that as an increasing proportion of the population live in urban areas cities are key to attaining sustainability objectives Citation[17]. Not only are cities a major source of GHG emissions, their populations also possess the knowledge, creativity and resources to reduce them. These principles were taken up at the 1992 Rio Earth Summit in Agenda 21 chapter 28, which called for municipal authorities to establish a local Agenda 21 (LA21) Citation[18]. LA21 promotes the localization of global issues, including climate change, the so-called ‘act local, think global’. Through cooperation with their local communities, municipal governments establish mechanisms for local action on GHG reduction and climate change management. In addition, LA21 includes mechanisms to facilitate and encourage communication with other local authorities. In theory, via this process, cities may learn from each other, developing and adapting suitable strategies for the reduction of GHG emissions Citation[19].
As demonstrated by the development of LA21, climate change requires policy response at the international level, although the impacts and solutions are local Citation[20]. In any local action, the initial motivator will have an element of global awareness; however, the action involves local actors working with local projects to reduce emissions, contributing to national and international reduction targets. Political rhetoric must frame climate change in a way that makes it solvable at the local level. Concepts, such as the carbon footprint, provide a mechanism for the measurement and management of emissions on a local scale. Policy makers and constituents can relate and understand the concept more easily than they can the global nature of climate change. Elements of global climate change policy can mutually reinforce local sustainability agendas, where action can be reconciled with secondary benefits. Some benefits are environmental, emphasizing the linkages between global and local environmental problems. Such links include improved local air quality, improvements in public health, reduced traffic congestion and better urban livability. Additional benefits focus on the social and economic areas, such as reduced costs of municipal operation and local job creation Citation[21]. Localization of the global issue of climate change is most likely to occur where appropriate policy responses (to control CO2 emissions) are already present (e.g., in the UK, local air quality monitoring areas Citation[22]), rather than providing a justification for policy action in itself Citation[23]. By publishing the co-benefits of climate change policy, local authorities can localize the issue of climate change for their constituents, and justify expenditure on climate protection initiatives Citation[24].
Municipal governments have significant influence over areas that are crucial to GHG reduction and management; they are pivotal in managing both transport demand and supply, with responsibility for supply and maintenance of infrastructure and traffic control mechanisms Citation[20]. They have considerable buying power, and through the provision of healthcare, education and municipal services, are poised to develop new markets for energy-efficient products and services Citation[25]. Furthermore, they have significant influence over land-use planning, waste management and energy consumption, all of which have implications for CO2 emissions Citation[26].
To establish an effective, workable climate change emissions reduction policy and action, municipal governments must first identify the appropriate means of monitoring and assessment. Many cities have established inventories of CO2 or wider GHG emissions, using simple pragmatic approaches, such as the approach of the International Council for Local Environmental Initiatives Citation[9]. These inventories provide the basis for action on climate change, providing a measurement and method of identification of cities with lower per capita emissions. For strategies to work, it is necessary for cities to have reliable, consistent and transparent inventories and to understand why emissions totals differ between cities Citation[11]. This requires the development and widespread uptake of a common indicator, such as the carbon footprint. This also neccessitates cooperation at the national and local levels, and even where the initiative exists, the translation of political rhetoric to policy is often hampered. Research on the CCP program identified two obstacles for the development of policy at the local level: the global framing of climate change; and institutional barriers Citation[27].
The global framing of climate change leaves individuals and policy makers feeling distanced, in both space and time, and they are therefore unlikely to see the problem as a legitimate local or individual concern. GHG emissions do not respect international boundaries or treaties, effecting climatic systems at a complex global level, over prolonged periods of time; local regions are only affected through impacts on the global-scale climate system. Officials and individuals have a limited understanding of the future implications, and how they might mitigate the problem. At the individual level, the scientific rationale and moral concerns of climate change are often outside the temporal and spatial perspective. People do not define climate change in terms of personal risk because the linkages between cause and effect are perceived to be weak. Reasoned choice may suggest that it is not good business sense for a municipal authority to expend effort reducing its GHG emissions since it cannot be certain that action in the local environment will make a measurable difference to the global threat of climate change Citation[27].
The CCP campaign successfully localized the policy of controlling GHG emissions rather than localizing the problem of climate change Citation[9]. The CCP campaign does not discuss climate change with reference to climate change or the harm it causes nature, but with reference to local benefits and the need to appeal to many diverse interests Citation[21]. The CCP campaign did also try to frame climate change in a picture of vulnerability for city dwellers (i.e., city dwellers are at risk of climate change and therefore should cut emissions). However, using risk as a basis of local climate action is not easy; no clear links have been identified between local action and the effect on global climate change Citation[21]. Effective action requires the localization of climate change emissions, impacts, and actions. The carbon footprint provides a mechanism to achieve this localization.
Boundaries & scope
Cities looking at developing carbon footprints must first examine the issue of boundary selection. In very simple terms, municipal leaders are presented with three choices for boundary setting: municipal operations; all emissions directly emitted from the city; or all territorial emissions, including process-linked emissions (e.g., production and generation). The options offer progressively more completeness at the cost of increased complexity.
Cities generate GHG emissions as the result of a range of processes and activities, within and outside their geographic boundaries. Emissions sources can be categorized in two forms: ‘direct’ and ‘indirect’. Direct emissions include those emissions occurring within the geographic boundary of the city, not associated with energy production. This includes all emissions associated with in-boundary fuel consumption, industrial processes and transport. Emissions from the production of energy are traditionally excluded from ‘direct’ sources owing to the commonly national nature of energy distribution. Energy produced in a given locale will probably be utilized at a national level through ‘grid’ type transmission networks. Indirect emissions include those associated with sources that are outside of the geopolitical spatial boundary, including emissions from primary energy production, electricity generation, waste and imports/exports. The World Resource Institute differentiates emissions sources in terms of ‘scopes’ (1–3) that relate to the spatial boundary of the activity or process. Scope 1 is defined as direct emissions within the geographic or administrative boundary of the city; for example, emissions from the domestic combustion of heating fuels, or industrial process emissions Citation[28]. Scope 2 considers emissions from electricity generation at a grid level, apportioned to end-users at the city level. Scope?3 considers further nonelectricity, out-of-boundary indirect emissions sources; for example, emissions associated with energy production chains, or imports and exports. Emissions sources can be further classified by life cycle perspective Citation[12], either production chain or single-process emissions. Upstream emissions occur as the result of the services, energy and products supplied to a city. Downstream emissions result from the outputs of in-boundary processes, including waste, and exported products and services. Some transboundary processes, for example, shipping and aviation, are difficult to categorize. Emissions from these sources can be considered both direct (Scope 1) and indirect (scope?3). Some studies completely exclude emissions from aviation, other studies Citation[6] exclude international flights and include emissions from the take-off and landing cycles of domestic flights at the local level. The UN Framework Convention on Climate Change suggests emissions from cruising aircraft be excluded from emissions inventories. Other studies, for example, from London Citation[15] and New York City Citation[14], consider emissions from aircraft in terms of fuel loaded at airports within city boundaries. Whilst technically considering movement away from the host city, this approach begins to consider the economic importance of aviation to the host city. This method does not consider the movement of passengers between flights and the surface movement of passengers from outside of city limits, although this could be considered to be a function of the city Citation[12]. The majority of emissions from these processes will be outside the geographic boundary of the city. However, cities must take responsibility for emissions as a direct process owing to the social and economic benefits gained (e.g., local economic growth and employment), and a certain level of control through mechanisms such as tourism and business development. demonstrates the concepts of emissions sources in cities in relation to scope and life cycle perspective, from the perspective of the processes occurring within a city. demonstrates the same concept, although in terms of the wider process boundaries.
The inclusion of all Scope 1–3 emissions sources does raise an issue with regards to equity and fairness. Cites with net exporting energy production industries will be penalized over cities who are net energy importers. Thus, a boundary that considers process-linked emissions becomes favorable, since process energy emissions can be assigned on an end-user basis to ensure fairness and equity. More difficult to categorize are emissions as a result of transboundary transport processes, for example, shipping and aviation. These processes may well cross international boundaries, let alone city boundaries. The further inclusion of all imported and exported products and services with scope?3 is a possibility. However, it is arguable as to how much influence municipal government can exert over consumer purchasing decisions. Thus, inclusion of emissions from imported and exported products and services may not represent an effective use of resources in the context of local policy decisions.
Assigning emissions responsibility
Most cities in North America and Europe were formed and grew as a result of industrial activities, and still require these activities for employment and revenue generation. The global pattern of industrial activity has shifted; developed countries are increasingly reliant on developing countries for resources, in part owing to large transnational organizations seeking lower costs and increased profitability. Legislative changes (although these rarely consider GHG emissions specifically) have also changed the geographic distribution of industry Citation[1]. In turn, this has caused a global shift in GHG emissions distributions. For emissions reduction measures to be effective, allocation of responsibility must be consistent to allow change over time to be monitored and avoid double counting Citation[29]. Broadly, there are two methodologically different accounting principles: production (at source) versus consumption (end-user) Citation[30]. The distinction is made on the difference between ‘emission’ and ‘responsibility’. Production accounting assigns responsibility to the producer of emissions, in this way emissions are located to the actual site of the emitting process (e.g., to the site of a factory or industrial process). Conversely, consumption accounting locates emissions to the final consumption of goods and services. Emissions associated with the manufacture and transport of all goods and services are appropriated to the point of consumption.
Production accounting is the most commonly used procedure and it is the procedure advised by the Intergovernmental Panel on Climate Change (IPCC) for national emissions accounting Citation[31]. Spatial boundaries can be easily defined in accordance with geographic and political boundaries. Given this perspective, national accounts consider emissions from exported products and services, but exclude those from the life cycle of imports Citation[32]. Upstream emissions are attributed to the producer, not the consumer.
Production accounting may lead to large underestimates of carbon emissions from products and services since a large proportion of carbon emissions are from supply chain processes Citation[33]. It often conceals issues of ‘burden shifting’; energy intensive process are exported to less-developed countries, and imports of products then increase in consumer countries Citation[34]. The consumer state is thus ‘rewarded’ with lower absolute emissions, yet a higher consumer-driven lifestyle. This issue can also be realized at the city scale, whereby high production regions are penalized with higher absolute emissions over those with high consumerism. This has led to concerns over ‘burden shifting’ and ‘carbon leakage’, the association of trade relations in national policy shifting carbon emissions Citation[35].
The allocation of carbon footprints in this way is also at odds with methods of national economic assessment, such as GDP. GDP is based on the value that is added for all institutional units within an economy regardless of the geographical location of activities Citation[36]. This means that national carbon footprints cannot be directly compared with economic measures. Consumption is the main driver of environmental degradation Citation[29], so allocating full responsibility for emissions to producers, and giving no responsibility to consumers seems inequitable and unrealistic. This is especially true when both producer and consumer countries have legally binding emissions reduction targets Citation[36].
Consumption-based accounting defines spatial scope on the target economy; emissions from the manufacture, transport and consumption of imports are counted. Owing to the macroeconomic spatial scale, uncertainties and complexity are generally higher than production accounting, especially at national and international scales. In this model, the end-user of a product is responsible for the emissions associated with the life cycle of the product. End-user accounting does not penalize areas with high emissions for exported services, making it more equitable and reducing the risk of ‘carbon leakages’. Consumption-based allocation of responsibility also gives a more realistic indication of a nation’s total environmental impact than production-based. It also removes some of the barriers that have previously prevented developing nations from becoming involved in international agreement for the reduction of GHG emissions, since they would not be held responsible for GHG emissions embodied in their exports to developed nations Citation[37]. The main disadvantage of this approach is that nations are made responsible for GHG emissions generated by processes they do not have any direct control over Citation[30]. Conversely, institutions that have direct control over processes that cause GHG emissions are not given a direct incentive to take measures to reduce emissions Citation[29]. Peters discusses these issues in detail, and provides a useful summary, see Citation[32].
Taking a consumption-based approach may more accurately represent the GHG emissions caused by the activities of the city. However, there may be equity issues since highly industrialized cities would benefit from business revenues and taxes, whilst not being held responsible for the carbon emissions of their industries. Largely residential cities would be held responsible for large carbon footprints owing to their higher density of consumers. In addition, they have a limited ability to influence consumer decisions. They have a greater ability to influence areas such as industrial process and infrastructure, through local legislative controls and policy. Given these considerations, a form of shared responsibility accounting whereby emissions from some process sources, such as energy and waste, are considered on a consumption approach, and other direct emission sources, such as industrial processes and transport, are considered using a production approach, would seem appropriate. This has the methodological benefits and equity advantages offered by both perspectives Citation[32].
Emissions estimation
Emissions factors, an emissions rate per unit of fuel or energy consumption, per activity, are the most easily applied and, indeed, widely used method of conversion. The IPCC guidelines emphasize the requirement to follow standardized, scientifically accepted principles, procedures and processes, collectively referred to as ‘good practice’ Citation[31]. In addition, the guidelines consider the concept of ‘tiers’ of data complexity, Tier 1 being the most basic, utilizing average and/or default emissions factors, tier 3 the most complex, utilizing country, fuel and process-specific emissions factors. The tier system allows for extrapolation across spatial levels, from national level inventories to the local level.
Tier 1 methods are simple and quick to apply, but are the least accurate and specific, relying on national and international statistics and default emissions factors. An emissions inventory can be relatively rapidly compiled through the application of national data and assumptions based on regulatory requirements and default values. GHG emissions are calculated by multiplying standard emissions factors by activity data. The method can be applied in the case of emissions removal, storage and capture, whereas one refers to an emissions factor, this could be termed a ‘removal coefficient’ Citation[31].
Tier 2 methods are similar to Tier 1 methods but are characterized by the use of more specific data such as country-specific emissions factors; engineering estimates of technology efficiency; per capita rates of fuel and electricity use augmented to take into account annual temperature variations; estimates of distances traveled within a community based on vehicle counts and road segment lengths; and quantity of fuel used based on price paid and annual average fuel cost. These methods are likely to require more work, but yield more accurate results that are more specific to local conditions. Similar to Tier 1 methods, emissions are calculated from a combination of emissions factors and activity data Citation[31].
Tier 3 methods are the most complex, time consuming and costly methods, but also the most accurate. Utilizing material mass balance calculations for fuel combustion; direct emissions monitoring and further mathematical modeling, estimates can be extremely accurate. In a city context, data from local energy supply can be collated and used for the estimation of emissions from energy consumption; for example, annual collection of vehicle odometer readings can be used to calculate emissions from vehicles within a city Citation[5]. In reality, these methods are often not practical at the city scale or indeed necessary to achieve reasonable levels of data accuracy. The IPCC and International Council for Local Environmental Initiatives guidelines suggest that the highest practicable tier of methods should be used Citation[9,31]. In practice, methods of calculation should be determined by the intended use of the footprint and the time and resources available for the calculations Citation[33].
In the context of a city footprint, this would indicate that all emissions associated with the upstream supply, direct process and downstream outputs of a city should be accounted for. The majority of existing and previous emissions inventories factor in emissions from direct fuel use (Scope 1) and indirect emissions associated with the provision of electricity, heat and steam (Scope 2) Citation[30]. However, calculation of indirect emissions embodied in goods and services consumed are often neglected Citation[38]. Estimates suggest that as much as 52% of UK household emissions are embodied in goods and services consumed Citation[39]. Whilst this may appear significant, inclusion of these emissions is highly dependent on the desired final use and purpose of the footprint. In terms of municipal government management of GHG emissions, their ability to influence consumer purchase decisions is limited, and effort would perhaps be better expended in managing infrastructure.
There are three principal approaches to emissions inventories: environmental input–output analysis (EIOA); process analysis (PA), hybrid environmental input–output life cycle assessment (hybrid-EIO-LCA). EIOA is a top-down approach that uses national economic and environmental data such as fuel mix and technologies used to estimate emissions caused by the activities of industry sectors within an economy Citation[25]. Top-down EIOA utilizes aggregate data at the meso level to attribute emissions based on economic flow. Combined with environmental accounting data, they can be used to calculate the carbon footprint, setting the economic system as the boundary Citation[10]. PA is a bottom-up LCA approach to analyze the emissions associated with specific processes, leading to a greater level of accuracy than EIOA Citation[40], but requires more time and resources Citation[25]. To overcome the shortfalls of EIOA and PA, hybrid-EIO-LCA is becoming widely accepted within the academic community as the best approach to carbon footprint calculation Citation[10,25,33,40]. Hybrid-EIO-LCA is a combination of PA and EIOA approaches, where PA is carried out for specific processes and embedded within an EIOA Citation[33]. The merits and limitations of each method are assessed below in the context of municipal government carbon footprints.
Applying the EIOA method for city carbon footprinting is problematic given that input–output data are only available at a national scale, and therefore to apply them to a city scale requires pro-rata scaling of national data Citation[13]. The accuracy of the local footprint then becomes dependent on how closely local conditions match national averages. EIOA uses national input–output tables as the basis for assessment. Industries with very different environmental impacts are often aggregated into a single sector for reporting (e.g., paper production and publishing) and represented by average emissions intensity for the sector Citation[40,37]. This is relatively unproblematic in national carbon footprinting, although at a city scale, this approach to carbon footprinting would not be sensitive to many of the strategies that might be employed to reduce emissions, such as site-specific energy reduction measures. In the UK, where EIOA has been applied on a subnational scale, it has been augmented with data regarding household expenditure (Family Expenditure Survey annually produced by the Office of National Statistics), and national census data. Census data include classification of small groups of households, termed output areas, by their economic status. This can be matched to the spending patterns of families in that economic category to generate a carbon footprint that varies depending on socioeconomic factors Citation[41]. These expenditure survey data are based on an annual survey of 7000 households. Accuracy will depend on how closely households within a given socioeconomic category within a city match the national average. The survey results could form the basis of a more accurate and specific application of EIOA for use in city carbon footprinting, although this would increase the time and resources needed to carry out the EIOA.
Process analysis is a bottom-up methodology originally developed for the environmental assessment of products Citation[10]. The bottom-up approach ensures that accuracy and transparency are high, and uncertainties minimized. PA is process-specific leading to a greater level of accuracy than EIOA Citation[40], but requires more time and resources Citation[25], which are not always available to municipal governments. The methodology excels when examining microsystems, enabling the study of impacts from cradle-to-grave. However, the bottom-up nature of PA can lead to truncation and cut-off errors when scaling up to subjects such as cities.
Hybrid-EIO-LCA is rapidly becoming widely accepted as the best approach to the carbon footprint calculation Citation[10,25,33,40]. Hybrid-EIO-LCA uses PA to calculate emissions for the main industrial processes undertaken within a sector as well as the main industrial processes in the immediate upstream supply chain, and downstream processes to the product end-of-life phase. EIOA is used for all upstream supply chain processes for which PA is not or cannot be performed Citation[40]. This has the benefit of being accurate and specific to the industry sector and would monitor carbon footprint variation due to changes in controlled processes while also including complete upstream emissions. In the context of city footprinting, hybrid-EIO-LCA energy-intensive processes, emissions associated with waste processing and disposal, and the carbon footprints of major suppliers of goods and services could be assessed by PA. Less significant and higher order upstream processes could be included in the EIOA.
▪ Treatment of ‘carbon sinks’
Biogenic carbon stocks are recognized as having an important role in GHG management Citation[42]. Land use and land-use change are also recognized as having a complex and significant effect on albedo, nutrient cycling, biodiversity, heat fluxes and carbon sequestrationCitation[43]. With over 50% of the world’s population now living in urban areas, there is a potential for changes in soil carbon stocks due to urban land-use change Citation[44]. However, understanding of urban biogeochemical cycles is still limited, making urban carbon sinks hard to quantify Citation[45].
The estimation of GHG removals by sinks is required in national GHG inventories. At a national level, agriculture, forestry and land-use change is the only category to include a form of carbon sink Citation[31]. The majority of existing methodological approaches to inventorying cities neglect to include GHG removals or biogenic processes. Green space management practices have been identified as having considerable impact on the rate of carbon sequestration within cities Citation[46]. Lawns and gardens in residential areas can also sequester considerable amounts of carbon Citation[46,47].
To motivate municipal governments to maximize the favorable management of green space vegetation and soil carbon stocks must be included in city carbon footprints. To prevent emissions ‘offsetting’ and burden shifting, only green space GHG removals within the administrative boundary of the city should be counted. Municipal governments have little or no influence over green space that is external to their administrative boundaries. As discussed previously, to ensure equitability and fairness, accounting must consider all sources within the city boundary. In the same sense municipal governments cannot claim the benefit of offsets outside of this defined boundary of emissions sources. The approach outlined by the IPCC for national inventories, to estimate the stock change over time, could feasibly be adapted for use at the local level Citation[31].
Conclusions
As centers of population and of high concentrations of anthropogenic GHGs, cities are key to the global response to climate change. Action to reduce emissions at a local level requires the localization of climate change and that municipal and local governments have a good understanding of emissions sources and reduction potentials. In pursuit of climate change localization, the concept of the ‘carbon footprint’ is increasingly being recognized as a valuable tool in emissions management. This article has examined the methodological challenges that still abound the development of a model for city carbon footprinting; issues including boundary selection, emissions responsibility and methodological choice.
Municipal governments are presented with several options for boundary selection. It is important to realize the need for complete inclusion of all sources and sinks of emissions including those indirect process emissions occurring outside the geographic boundaries whilst also considering the limits of municipal government influence and control. Inclusion of transboundary processes, for example, energy provision, is vital to ensure equitable emissions apportioning. Whilst inclusion of emissions from products and services is outside the scope of municipal government control, and less can be gleaned from inclusion.
Selection of boundary conditions is informed by issues surrounding emissions responsibility. Action for reduction of these emissions is difficult until responsibility and roles of leadership are established. Equitable apportioning of emissions is also vital to ensure responsibility is taken for emissions management, mitigation and reduction, and to prevent burden shifting. This includes those emissions from transboundary processes, such as shipping and aviation, where equitable apportioning is a subject of much debate. This also raises the issue of fairness and equitability. Emissions must be allocated fairly. To achieve this, work is required to establish appropriate methods to apportion both the social and economic benefits and the environmental impacts of emissions.
The accuracy of a model depends on the quality of data. The prerequisite of this is the desired end-use of the model. Model developers must remain aware of the financial and time constraints faced by municipal governments, as well as the requirement to produce a decision-assisting tool. Tier 1 methods are simple and quick to apply, but are the least accurate and specific. tier 3 methods are the most complex and accurate, but also the most resource intensive. We suggest that Tier 2 methods offer the most cost-effective balance; indeed, the added accuracy offered by tier 3 methods is unlikely to be required in the context of city-level policy decisions. However, this is not to rebuke the use of tier 3 methods where data and resources are available to produce improved accuracy.
Models must be developed that are transferable to enable comparisons and foster a sense of competitive emissions reduction. Without a consistent methodology whereby different cities can be footprinted using the same underlying structure, comparison of models is invalid. The majority of current research agrees that the most successful and inclusive method of carbon footprinting at the sub-national level is achieved through the application of hybrid-LCA, where EIOA is utilized to assess high-level processes, supplemented with PA where possible. This results in increased accuracy, completeness and the ability to capture city and sector level emissions reduction measures.
Adequate tools are required for municipal governments and policy decision makers to make informed decisions to improve city level climate change management. A number of tools have already emerged, but for the reasons presented above these tools do not constitute a definitive approach. Further development to develop a comparable, consistent, complete, transferable and accurate carbon footprinting tool is greatly needed.
Future perspective
The urban population is predicted to increase; as cities grow and expand, their role in the mitigation of climate change will rise proportionally in prominence. Municipal leaders and local policy makers will need access to the appropriate tools if they are to make effective and workable decisions. Such tools must be developed in such a way as to make them useable with the limited time and resources available to local governments.
Not all cities are identical; they have different industries, locations, topographies, levels of commercial activity and demographic profiles, among other factors. Carbon footprint models for local government GHG management in cities must be developed in such a way so as to fairly, and accurately assign emissions data. In addition, it is not worth such models assigning emissions to cities for which municipal government cannot take responsibility or cannot influence. Emissions need to be allocated in such a way as to localize the issue of climate change and promote action at the local level. Emergence of models for the carbon footprinting of cities has begun; refinement and the development of new models within the foreseeable future is a high probability.
Table 1. Comparison of production, consumption and shared responsibility GHG accounting.
A measure of the total amount of CO2 and CH4 emissions of a defined population, system or activity, considering all relevant sources, sinks and storage within the spatial and temporal boundary of the population, system or activity of interest. Calculated as CO2 equivalents using the relevant 100-year global warming potential.
A natural or artificial reservoir that accumulates and stores CO2 or other GHGs for an indefinite period of time.
An average emissions rate of given pollutant from a given source per unit of activity; for example, kilograms of carbon emitted per kWh of electricity generated for a given method of generation.
An average rate of a given pollutant removal due to sequestration, storage or capture per unit of activity; for example, kg CO2 sequestered per ha of land for a given land use type.
The process by which CO2 is removed from the atmosphere, deposited and stored in a reservoir or ‘carbon sink’. Referred to in geoengineering as a technique for the long-term storage or CO2 and other forms of carbon as a method of climate change mitigation.
Introduction
▪ The concept of the ‘carbon footprint’ is increasingly being recognized as a valuable tool in GHG emissions management. | |||||
▪ The carbon footprint represents a powerful climate change policy decision aiding tool for municipal governments. | |||||
▪ A number of attempts have been made to catalog the carbon footprint of regions, local areas and, most significantly, cities. However, no international standard methodology currently exists requiring the reporting of urban emissions. |
Cities & GHG management
▪ The 1987 Bruntland Report argued that cities are key to attaining sustainability objectives. | |||||
▪ Cities are a major source of GHG emissions; they also possess the knowledge, creativity and resources to reduce them. | |||||
▪ Municipal governments have significant influence over areas that are crucial to GHG reduction and management; they are pivotal in managing both transport demand and supply, with responsibility for supply and maintenance of infrastructure and traffic control mechanisms. | |||||
▪ To establish an effective, workable emissions reduction policy, municipal governments must first identify the appropriate means of monitoring and assessment. |
Assigning emissions responsibility
▪ One of the major factors affecting carbon footprints, from the national to the individual level is the treatment of responsibility. Broadly there are two accounting principles: production (at source) versus consumption (end user). | |||||
▪ Production accounting assigns responsibility to the producer of emissions. Consumption accounting locates emissions to the final consumption of goods and services. | |||||
▪ End-user accounting does not penalize areas with high emissions for exported services, providing a more realistic indication of a nation’s total environmental impact than production-based accounting. | |||||
▪ Municipal governments have a limited influence over consumer decisions. They have a greater influence regarding industrial processes and infrastructure. A form of hybrid accounting whereby emissions from some process sources are considered on a consumption approach, and other ‘direct’ emission sources are considered using a production approach, may be most appropriate. |
Emissions estimation
▪ The Intergovernmental Panel on Climate Change describes ‘tiers’ of methods used for estimating GHG emissions. Tier 1, the least specific, to tier 3, involving the most specific methods. Tier 1 methods may not provide the required level of detail, while tier 3 methods are often too resource intense. | |||||
▪ City carbon footprints should include all emissions associated with the upstream supply, direct process and downstream outputs of a city. | |||||
▪ Emissions factors, an emissions rate per unit of fuel or energy consumption, per activity, are the most easily applied and, indeed, widely used method of activity to emissions conversion. | |||||
▪ There are three principal life cycle approaches to emissions inventories: environmental input–output analysis; process analysis; and hybrid environmental input–output life cycle assessment. |
Conclusions
▪ Cities are key to the global response to climate change. Reduction of emissions requires the localization of climate change and an understanding of emissions sources and reduction potentials. | |||||
▪ The complete inclusion of all sources and sinks of emissions is required. Whilst also considering the limits of municipal government influence and control. | |||||
▪ Equitable apportioning of emissions is also vital to ensure responsibility is taken for emissions management. | |||||
▪ Tier 2 methods offer the most cost-effective methodological option; Tier 1 methods are less accurate and specific; tier 3 methods are too resource intensive. | |||||
▪ Research suggests the use of hybrid-life cycle assessment, where environmental input–output analysis is utilized to assess high-level processes, supplemented with process analysis where possible. |
Financial & competing interests disclosure
This study is funded by Southampton City Council. The authors have no other relevant affiliations or financial involvement with any organization or entity with a financial interest in or financial conflict with the subject matter or materials discussed in the manuscript apart from those disclosed.
No writing assistance was utilized in the production of this manuscript.
Bibliography
- Dodman D. Blaming cities for climate change? An analysis of urban greenhouse gas inventories. Environment Urbanization21(1),185–201 (2009).
- Bader N, Bleischwitz R. Measuring urban greenhouse gas emissions: the challenge of comparability. S.A.P.I.EN.S2(3),1–15 (2009).
- UN Population Fund. State of World Population 2007 Unleashing the Potential of Urban Growth. UNFPA, NY, USA (2007).
- Fleming PD, Webber PH. Local and regional greenhouse gas management. Energy Policy32(6),761–771 (2004).
- Salon D, Sperling D, Meier A, Murphy S, Gorham R, Barrett J. City carbon budgets: a proposal to align incentives for climate-friendly communities. Energy Policy38,2032–2041 (2010).
- Carney S, Shackley S. The Greenhouse Gas Regional Inventory Project (GRIP): Designing and employing a regional greenhouse gas measurement tool for stakeholder use. Energy Policy37(11),4293–4302 (2009).
- United Nations. Kyoto protocol to the United Nations framework convention on climate change. (1998).
- Great Britain. The Climate Change Act 2008 (c. 27). HMSO, London, UK (2008).
- ICLEI – Local Governments for Sustainability. Local Government Operation Protocol: For the Quantification and Reporting of Greenhouse Gas Emissions Inventories. (2008).
- Wright LA, Kemp S, Williams ID. ‘Carbon footprinting’: towards a universally accepted definition. Carbon Management2(1),61–72 (2011).
- Kennedy C, Steinberger J, Gasson B et al. Greenhouse gas emissions from global cities. Environ. Sci. Technol.43(19),7297–7302 (2009).
- Kennedy C, Steinberger J, Gasson B et al. Methodology for inventorying greenhouse gas emissions from global cities. Energy Policy38(9),4828–4837, (2010).
- Peters GP. Carbon footprints and embodied carbon at multiple scales. Curr. Opin. Environ. Sustainability2,245–250 (2010).
- Mayor’s Office of Long-Term Planning and Sustainability, New York City. Inventory of New York greenhouse gas emissions. NY, USA (2007).
- Greater London Authority. Action Today to Protect Tomorrow: The Mayor’s Climate Change Action Plan. GLA, London, UK (2007).
- Rypdal K, Winiwarter W. Uncertainties in greenhouse gas emission inventories – evaluation, comparability and implications. Environ. Sci. Policy4(2–3),107–116 (2001).
- World Commission on Environment and Development. Our Common Future, Report of the World Commission on Environment and Development. Published as Annex to General Assembly document A/42/427. Geneva, Switzerland (1987).
- United Nations. Results of the World Conference on Environment and Development: Agenda 21. Presented at: The UNCED United Nations Conference on Environment and Development. United Nations, NY, USA (1992).
- Betsill M, Bulkeley H. Cities and the multilevel governance of global climate change. Global Governance12(2),141–159 (2006).
- Collier U, Löfstedt RE. Think globally, act locally? Local climate change and energy policies in Sweden and the UK. Global Environ. Change7(1),25–40 (1997).
- Lindseth G. The cities for climate protection campaign CCPC and the framing of local climate policy. Local Environment9,325–336 (2004).
- Great Britain. The Environment Act 1995 (c. 25). London, HMSO (1995).
- Bulkeley H, Betsill M. Cities and Climate Change: Urban Sustainability and Global Environ. Governance. Routledge, NY, USA (2003).
- Kousky C, Schneider SH. Global climate policy: will cities lead the way? Climate Policy3(4),359–372 (2003).
- Larsen HN, Hertwich EG. The case for consumption-based accounting of greenhouse gas emissions to promote local climate action. Environ. Sci. Policy12(7),791–798 (2009).
- Bulkeley H, Kern K. Local government and the governing of climate change in Germany and the UK. Urban Stud.43(12),2237–2259 (2006).
- Betsill M. Mitigating climate change in us cities: opportunities and obstacles. Local Environment6(4),393–406 (2001).
- Greenhalgh S, Broekhoff D, Daviet F et al.The GHG Protocol for Project Accounting. WRI/WBCSD, Washington DC, USA (2003)
- Lenzen M, Murray J, Sack F, Wiedmann T. Shared producer and consumer responsibility – theory and practice. Ecological Economics61(1),27–42 (2007).
- Munksgaard J, Pedersen KA. CO2 accounts for open economies: Producer or consumer responsibility? Energy Policy29(4),327–334 (2001).
- Intergovernmental Panel on Climate Change. 2006 IPCC Guidelines for National Greenhouse Gas Inventories 1. General guidance and reporting. Egglestone S, Buendia L, Miwa K, Ngara T, Tanabe K (Eds). Institute for Global Environmental Strategies, Hayama, Japan (2006).
- Peters GP. From production-based to consumption-based national emission inventories. Ecological Economics65(1),13–23 (2008).
- Matthews HS, Hendrickson CT, Weber CL. The importance of carbon footprint estimation boundaries. Environ. Sci. Technol.42(16),5839–5842 (2008).
- Druckman A, Bradley P, Papathanasopoulou E, Jackson T. Measuring progress towards carbon reduction in the UK. Ecological Economics66(4),594–604 (2008).
- Munksgaard J, Minx J, Christofferson LB, Pade LL, Suh S. Models for national co2 accounting. In: Handbook of Input–Output Economics in Industrial Ecology. Suh S (Ed.). Springer 533–558 (2009).
- Hertwich EG, Peters GP. Carbon footprint of nations: a global, trade-linked analysis. Environ. Sci. Technol.43(16),6414–6420 (2009).
- Wiedmann T. A review of recent multi-region input–output models used for consumption-based emission and resource accounting. Ecological Economics69(2),211–222 (2009).
- Bin S, Dowlatabadi H. Consumer lifestyle approach to us energy use and the related CO2 emissions. Energy Policy33(2),197–208 (2005).
- Druckman A, Jackson T. The carbon footprint of UK households 1990–2004: a socio–economically disaggregated, quasi-multi-regional input-output model. Ecological Economics68(7),2066–2077 (2009).
- Suh S, Lenzen M, Treloar GJ et al. System boundary selection in life cycle inventories using hybrid approaches. Environ. Sci. Technol.38(3),657–664 (2004).
- Druckman A, Sinclair P, Jackson T. A geographically and socio–economically disaggregated local household consumption model for the UK. J. Cleaner Production16,870–880 (2008).
- Ostle NJ, Levy PE, Evans CD, Smith P. UK land use and soil carbon sequestration. Land Use Policy256,5274–5283 (2009).
- Robinson DT, Brown DG, Currie WS. Modeling carbon storage in highly fragmented and human dominated landscapes: linking land cover patterns and ecosystem models. Ecological Modeling220,1325–1338 (2009).
- Pouyat R, Groffman P, Yesilonis I, Hernandez L. Soil carbon pools and fluxes in urban ecosystems. Environ. Pollution116,S107–S118 (2002).
- Lorenz K, Lal R. Biogeochemical c and n cycles in urban soils. Environment Int.35,1–8 (2009).
- Tratalos J, Fuller RA, Warren PH, Davies RG, Gaston KJ. Urban form, biodiversity potential and ecosystem service. Landscape Urban Planning83,308–317 (2007).
- Qian YL, bandaranayake W, Parton WJ, Mecham B, Harivandi MA, Mosier AR. Long-term effect of clipping on nitrogen management in turf grass on soil organic carbon and nitrogen dynamics: the century model simulation. J. Environ. Quality32,1694–1700 (2003).