Abstract
Limiting the effects of global climate change requires reductions in anthropogenic GHG emissions. However, without regulations mandating decarbonization of industrial society, emission reduction strategies must provide opportunities for generating profit. Product carbon footprinting (PCF) has emerged as a means to identify emission reduction opportunities for all economic activity by analyzing the full life cycle of goods and services. The detailed emissions inventories obtained through PCF can facilitate identification of areas in a supply chain where GHG emissions can be reduced with a positive return on investment. Despite recent efforts to standardize PCF, a lack of data and data uncertainty remain barriers to restructuring supply chains and manufacturing processes to achieve cost-effective emissions reductions. We provide a basic framework to conceptually demonstrate how data gaps can be filled and uncertainty in PCF can be more accurately assessed, while simultaneously reducing the costs of conducting PCF and yielding positive return on investment.
Targeted data collection for a full life cycle PCF begins in a cradle-to-gate manner, generating a partial PCF, starting with natural resource extraction (flow 1) by primary producers, who operate at the interface of the natural environment and the industrial supply chain. All GHG emissions produced by processes under the control of each producer in the supply chain (primary producers and secondary producers) are quantified by that producer (gate-to-gate accounting). As products are transported between actors in the life cycle of the product (producers, consumers and disposal facilities), emissions of GHGs generated by transportation are applied to the partial PCF of the receiving organization, illustrated by the dashed boundaries.
PCF: Product carbon footprint.
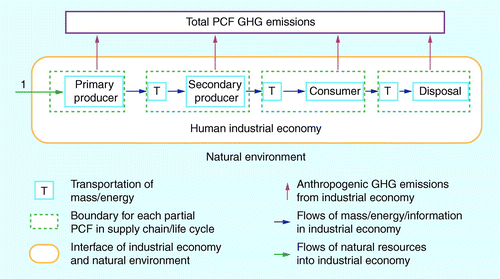
Targeted data collection begins in a cradle-to-gate manner starting with natural resource extraction (flow 1) by primary producers. Transportation emissions (GHGs-T) from one producer to the next are applied to the partial PCF of the downstream producer. Movement of materials, intermediate and finished products, and energy between any actors in the life cycle can be sequentially described using circuits of the numbered flows. The total life cycle PCF is the aggregate of the partial PCFs of all actors in the life cycle.
GHGs-C: GHG emissions resulting from all unit processes controlled by the consumer; GHGs-D: GHG emissions resulting from all unit processes controlled by the disposal facility; GHGs-P: GHG emissions resulting from all unit processes controlled by the primary producer; GHGs-S: GHG emissions resulting from all unit processes controlled by the secondary producer; PCF: Product cabon footprint.
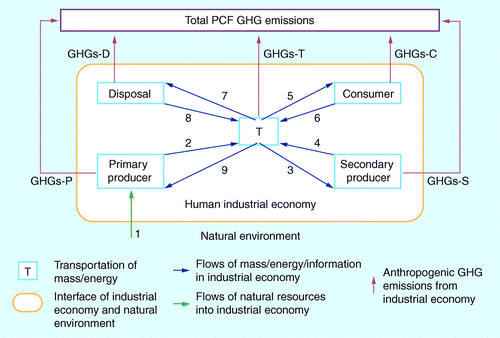
The current concentration of CO2 in the atmosphere is approximately 394 ppm Citation[101]. In order to limit the effects of climate change on society, the IPCC recommends substantial reductions in carbon emissions over the coming decades Citation[1]. To attain the emission reductions necessary, a suite of technologies will be required to decarbonize the global economy Citation[2]. While wholesale implementation of noncarbon energy sources and energy efficiency measures may be technologically feasible, industries are unlikely to undertake emission reduction actions without a positive return-on-investment in the absence of GHG emission regulation. Emission reduction strategies with a positive return-on-investment include eliminating wasteful practices, by increasing efficiency of material and energy use in both production systems and business operations. Actions such as reducing energy consumption by using more efficient lighting or videoconferencing tend to have clear-cut economic benefits and can be easily implemented. However, other emission reduction strategies require the wholesale restructuring of supply chains and manufacturing processes, and involve substantial investments of time and capital. The risks associated with these investments necessitate the availability of accurate emission inventories to properly conduct a cost–benefit analysis for different emission reduction options. At the same time, the interests of cost control in climate change mitigation demand that accurate emissions inventories be made available with minimum expenditure. Conceptually, the requirements for emissions reductions, the positive return on investment for efficiency gains, the need for accurate emission inventories and the demand for cost–effectiveness in climate change mitigation are not mutually exclusive. The synergy between these goals can be realized through product carbon footprinting (PCF) and represents a logical next step toward emission reductions in the absence of regulatory requirements.
Often, it is more effective for organizations to gain efficiency by focusing on specific sectors of their operations, rather than viewing the entire organization in aggregate. This same approach can be used to determine the return-on-investment of specific emission reduction actions, while facilitating cost-effective emission inventory accounting with high accuracy. Much of the discussion surrounding emission reduction has focused on the emissions inventory for an organization in aggregate. Termed the carbon footprint, this concept has become ubiquitous in the climate change lexicon, yet it continues to lack a universally accepted definition Citation[3]. PCF, the full life cycle accounting of GHG emissions associated with an individual product (see Box 1 for definition of product) from raw material acquisition through all stages of production, use and final disposal Citation[4], has emerged as a means of calculating a carbon footprint, which provides refined, disaggregated inventory data. PCF has two main advantages over entity-level carbon accounting. First, through application of life cycle assessment (LCA) methodology, it can provide an organization with process-specific information on the materials consumption, energy use and carbon intensity of each product it produces throughout the entire product life cycle Citation[5,6]. Second, it provides a means by which to report the per unit carbon intensity of its products to consumers and other producers Citation[7].
By using process-specific data, PCF can help producers identify high GHG intensity areas (hot spots) in their supply chains and production lines. In addition, the same process-specific data can allow producers to identify the most wasteful areas, in terms of materials and energy, in their supply chains and production lines. Armed with this knowledge, producers can focus their efforts related to decarbonization and efficiency gains in areas with the highest potential Citation[7]. Where a reduction in energy consumption from fossil fuel sources is achieved for efficiency purposes, a concomitant decarbonization is achieved; and, with rising energy prices, such a decarbonization can be achieved with a positive return on investment.
For consumers, PCF enables product carbon footprint labeling (PCFL), which offers a free market approach to carbon management, provided a consistent methodology is used in the accounting and the claim is third-party verified. With PCFL, consumers can use product labels to voluntarily choose to lower their GHG emissions through consumption of less carbon intensive finished products Citation[7]. Likewise, PCFL can allow producers to choose less carbon intensive input materials and products for their production system when applied to intermediary products in a supply chain. However, finding both hot spots and potential efficiency gains at the manufacturing process level and accurately reporting the emission intensity of a product on a per unit basis requires accurate data on the entire manufacturing process and supply chain. In a complicated global economy with rapidly shifting supply chains, the availability and accuracy of these data can often be limited Citation[8].
Over the past few years, multiple PCF standards and product category rules (PCRs) have been published in an effort to harmonize PCF methodology Citation[4,9,10]. Despite efforts to standardize PCF, uncertainty in PCF calculations remains a problem Citation[11,12]. In addition, an integrative framework that outlines the starting point for data acquisition and a coherent flow of data dissemination through the macroeconomy has not yet been developed. In this article, we present a basic framework for focused data collection and data sharing to enhance the user friendliness and accuracy of PCF information. This framework is a means of compartmentalizing supply chains, thereby providing discrete process-level boundaries to reduce the cost of data collection for all members of the supply chain and create boundaries within which uncertainty is estimated. Coupled with making the resulting data publicly available, the proposed framework will increase methodological transparency, providing final product producers with the data necessary to quantify the carbon footprint of their products on a per-unit basis at minimum cost and help to quantify error in these estimates.
Background
PCF is a simplified form of an LCA Citation[5,9], which aims to quantify the total environmental impacts of a product along the entire supply chain and all life cycle stages, from natural resource extraction through manufacturing, use and maintenance, and eventual disposal or recycling Citation[13]. This is in contrast to a typical GHG emissions inventory, which aim to quantify GHG emissions solely from the operations of a single business or organization within a supply chain or product life cycle. Unlike standard LCAs, the focus of PCF is solely on GHG emissions and their effects on the global climate and does not consider other environmental impact categories (e.g., photochemical oxidant creation) Citation[14,15]. Thus, the goal of PCF is to quantify the impacts from the total GHG emissions associated with a particular product or service throughout the entire life cycle. PCF simplifies LCA methodology by employing the IPCC global warming potentials of individual GHG in order to compute the climate impacts of products and/or services Citation[9]. In order to compute these impacts, a full life cycle GHG emissions inventory analysis is needed.
The strength of PCF comes in the LCA methodology that is employed during the inventory analysis phase for data collection. This methodology has been formalized in the requirements of the International Organization for Standardization’s (ISO) 14040/14044 standards Citation[13,16]. Through application of this methodology, GHG emissions are accounted for all the way to the unit process level, which is not the case with other GHG inventory accounting techniques. For instance, accounting for facility-wide emissions can be accomplished by using data gathered from utility providers during routine billing (Scope 2 emissions calculation using the Greenhouse Gas Protocol methodology) Citation[17]. While this methodology can be employed to inform a company about aggregate energy consumption (an entire factory), it does not provide information about the emissions associated with a unit of the organization’s product and cannot provide information about which processes within a production facility (a single processing step) are consuming the most energy. Data collection at the unit process level requires the measurement of all inputs and outputs of materials and energy for each unit process. As an example, the New Belgium Brewing Company conducted a PCF for a six-pack of its beer. They determined that approximately 5.4% of the emissions associated with their product are produced within the boundary of their facility Citation[102]. Of these emissions, natural gas use accounted for 71% and is used in processes such as water treatment. This accounting elucidates the GHG intensity of each unit process, and in so doing reveals the resource consumption intensity of each unit process. Refinement of the inventory makes the data useful for efficiency auditing and, in turn, makes efficiency-auditing data useful for PCF.
For a complete PCF, every unit process must be analyzed for the entire supply chain for a given product and all associated GHG emissions summed. However, tracking unit process information throughout the supply chain presents a major challenge for PCF Citation[8]. Collection of inventory data requires communication between multiple businesses in the supply chain. A single firm that produces a final product does not have direct access to information concerning the unit processes employed by upstream firms that provide materials and intermediary products. Downstream firms must gather information about upstream unit processes from the upstream firms who control those unit processes. With complex products, this can require multiple firms communicating across large distances and requires that consistent accounting methodology be employed in order to generate reliable unit process information.
In recent years, multiple PCF standards have been developed with the aim of facilitating communication of information between firms and establishing common, consistent PCF methodology Citation[4]. These standards are a positive step forward for PCF, but remain lacking in the treatment of uncertainty in the GHG emissions inventory analysis, including scope and boundary selection. Guidance for assessing and quantifying uncertainty is given, but quantified uncertainty is not required to be reported Citation[5,6,18]. Uncertainty is inevitable when final product producers at the downstream end of the supply chain attempt to back-track GHG emissions through the supply chain, as is prescribed by the current standards Citation[5,6,18]. Uncertainty results from downstream producers not knowing which unit processes are employed by upstream producers, as well as not knowing the variability of those unit processes. In cases where primary data (direct measurement of the unit processes) are not available, secondary data (such as published studies for similar processes) are employed to estimate GHG emissions from upstream processes Citation[5,6]. Such a strategy introduces a source of uncertainty that is impossible to quantify. Even in cases where primary data are available for a given unit process (such as the volume of fuel consumed for a single leg of a transportation step) uncertainty is present in emission factors (kg CO2 per l of fuel consumed) that are used to estimate GHGs resulting from that unit process Citation[11].
The magnitude and sensitivity of uncertainty in PCF has been demonstrated by many researchers for products ranging from potatoes to petroleum, and services such as tourism Citation[11–12,19–26]. For example, uncertainty in PCF within the context of developing nations has been analyzed for sugar production in Africa. Plassmann et al. showed that, even while using a standardized PCF methodology, uncertainty in land-use change emissions can translate into a 1900% difference in the size of the carbon footprint of a unit of sugar Citation[11]. In addition, the researchers demonstrated that emission factors for transportation obtained from commonly used sources vary by more than a factor of 2. Such large errors and variation caused by uncertainty reduce the overall value of PCF. When attempting to find supply-chain efficiencies that have a positive return-on-investment, organizations are not likely to accept such a high level of uncertainty.
In addition to uncertainties introduced due to a lack of accurate data, boundary selection in PCF also introduces uncertainty. Boundary selection includes choosing which GHGs to include in the analysis, as well as which unit processes to consider in the accounting Citation[3,27]. For example, when comparing biofuels to fossil fuels, emissions could be included only up to delivery of the fuel to a fueling station. Alternatively, the analysis could consider emissions generated from fuel combustion, as well as the differences in fuel economy in vehicles based on fuel type Citation[28]. The choices made in selecting the system boundaries impact the results of the PCF, and thus introduce a source of model uncertainty leading to variability across studies Citation[29]. Standardizing PCF methodology can help to reduce this variability by defining common approaches to PCF Citation[4,9] and establishing common rules for boundary selection. A true life cycle PCF, however, must include all GHGs and all unit processes. The current PCF standards exclude embodied emissions associated with capital goods in their methodology Citation[6] and provide de minimis thresholds, under which emissions need not be included in the PCF Citation[5,6]. For some products, emissions embodied in capital goods are significant Citation[9,30], and in complex supply chains the large number of unit processes can make even the smallest emitters significant once aggregated Citation[30]. A standardized methodology that includes all embodied emissions will improve the accuracy of PCF results and improve the use of PCF for comparing goods and services.
In addition, global supply chains with interchangeable component suppliers present one of the greatest challenges in PCF Citation[8]. A component supplier can be substituted rapidly to deal with disruptions, such as those caused by natural disasters. Therefore, there is the potential for change in the emissions associated with a specific component over a short period of time, increasing the difficulty in quantifying the PCF for the final product. This variability, coupled with the difficulty associated with collecting and analyzing inventory data for each process in the supply chain, can make the inventory analysis phase of PCF challenging. As an example, when a company has quantified the emissions associated with every step in its supply chain, including the associated error, and one step in the supply chain must be substituted with a supplier that has not completed an emission inventory, uncertainty increases because a mean per unit emission value must be estimated for that step.
Overall, the factors outlined above create multiple barriers for many organizations by reducing the confidence in PCF results, as well as the utility of PCF with respect to influencing decision making Citation[19,31]. In order for PCF to affect business decision making in the absence of regulation, the results must be as accurate as possible and the error quantified. Only then can PCF provide organizations with enough information to assess if a particular investment will result in a large enough efficiency gain to justify the risk of expenditure. PCF results must be readily available and capable of changing in real time with shifting supply chains. It is also paramount, with a lack of required GHG reporting via regulations, that PCF results be made available in a cost-effective manner. The current approach to PCF as presented in the freely available standards does not facilitate these needs. An alternative is needed.
Alternative framework for PCF
In order to accurately account for individual production methods and supplier substitution within individual supply chains, the specific inputs and outputs of all unit processes for all individual producers must be accounted for and uncertainty accurately estimated. Such detailed data collection and analysis can be resource intensive and prohibitively expensive for individual smaller businesses and organizations, especially when a producer utilizes many intermediary products from an array of global suppliers Citation[8,31]. In addition, a lack of incentive to conduct PCF in the absence of regulation may present a barrier for many organizations. Data gaps, high uncertainty and resource barriers, however, can be overcome (while incorporating profit incentive) by compartmentalizing the supply chain and using an open PCF process for data sharing. This forms the foundation of the alternative framework: each supplier focuses on gathering primary data and accurately assessing uncertainty within their scope of direct control, contributes to overall PCF cost reduction through low or no cost data sharing, enjoys the financial return on investment from efficiency gains within their scope of direct control and produces real environmental benefit through reduced GHG emissions resulting from lowered resource consumption.
▪ Boundary selection for the alternative framework
In this alternative framework, all GHGs are included in the emissions inventory along with all other inputs and outputs for unit processes. This boundary choice eliminates a source of uncertainty that is introduced when only a select few gases are accounted for in the carbon footprint (see Box 2 for issues related to defining ‘carbon footprint’). The total life cycle PCF has the boundary of all unit processes involved in the entire life cycle of the product analyzed. These boundary selections can reduce boundary selection uncertainty by including all GHGs released throughout the product life cycle.
Using a boundary that includes the entire supply chain and all GHGs does not imply uncertainty will be reduced in practice when secondary data are used in place of primary data, or no data are available at all. For this reason, the total life cycle PCF (and supply chain) has been compartmentalized in the alternative framework, with boundaries for each firm in the supply chain selected based on ‘manageability’ of emissions (shown as dashed boundary lines in ). A firm is responsible for accounting of emissions from all unit processes that are owned or under direct control of that firm. Ownership and control imply a firm has access to primary data concerning those unit processes. By setting individual firm boundaries in this manner, primary data are generated for all unit processes in the PCF by those with direct control and who are most familiar with the unit processes and their variability. Uncertainty introduced by a lack of data can potentially be reduced through gathering of primary data and more accurate uncertainty estimates may be generated.
▪ Mechanics of the alternative framework (propagation of information & targeted data collection)
Each producer contributes to increased accuracy in overall uncertainty estimates by accounting for all direct and indirect emissions, including quantification of error, for each unit process owned and operated by the firm. Using an open PCF process for data sharing, life cycle PCF can be aggregated from individual firms in the supply chain. It is also possible to derive bounded error estimates for the life cycle PCF by propagating the individual producer estimates of error through the supply chain Citation[20,29]. This approach requires that the PCF process begins with the most upstream producers operating at the interface of the natural environment and industrial supply chain. We refer to these upstream producers as ‘primary producers’, with all downstream producers and transporters involved in the supply chain up to the interface with the consumer referred to as ‘secondary producers’. Flows of mass, energy and information through the proposed model of the industrial economy are outlined in . A key element of the proposed framework is that information for all life cycle stages of a given product and its constituent parts flows with mass and energy in real time, making upstream PCF information available to the receiving party at the time of purchase/delivery. This is in contrast to current backward looking PCFs, where a firm manufacturing and distributing a final or intermediary product attempts to back-track the GHG emissions through its own supply chain, producing a so-called cradle-to-gate PCF.
A true cradle-to-gate approach can only be undertaken if primary producers operating at the interface of the natural environment and the industrial supply chain (i.e., oil field extraction of crude oil, mineral and metal mines, timber harvested forests and agricultural production) have quantified their emissions. Producers at this interface have the ability to directly measure the initial cradle-to-gate GHG inventory for their primary product, and their inventory will represent the emissions associated with raw material extraction and processing. It is at the primary production level where initial data gathering and error quantification should begin, preventing the need for emissions estimation and the associated uncertainty for this step in the supply chain. In the language of ISO 14040 Citation[13], materials extracted by primary producers are so called ‘elementary flows’ of original inputs from the natural environment (represented by flow 1 in ).
After the initial extraction of resources, the majority of firms in the supply chain (secondary producers), including transporters, act as intermediaries investing only labor and energy into the movement, transformation and assembly of the upstream raw materials or products, depending on the location of the intermediary firm in the supply chain. These investments of labor, energy and input products should be well known as a matter of routine business. If upstream producers have accounted for their inputs, starting with the first cradle-to-gate inventory, the total supply chain embodied GHG emissions can be easily aggregated by any downstream firm, by accounting for the inputs of energy and labor they contribute and adding them to the GHG emissions embodied in the upstream materials and products. In this fashion, secondary producers complete a gate-to-gate PCF (including quantification of error) and add to the growing cradle-to-gate PCF by incorporating the information for their individual inputs and transportation needs. In this framework, the secondary producers receiving intermediary products take ‘ownership’ of transportation emissions and incorporate these into their gate-to-gate partial PCF. This choice is made to reflect the ability of receiving firms to reduce transportation-related emissions by choosing suppliers in closer proximity.
Although primary producers may not have data for emissions embodied in capital goods and input products initially, these emissions can be accounted for and incorporated into the primary producer inventory once the supply chain of those input products has accounted for the cradle-to-gate emissions of the products. This alternative framework allows for feedback of embodied emissions information to primary producers, with information being delivered along with the input products. In this manner, a lack of initial information is overcome through normal economic activity after primary producers conduct the first cradle-to-gate PCF. By quantifying the mean emissions and associated error per unit of product for the gate-to-gate measurements, the overall PCF uncertainty can be accurately estimated. All producers need only focus on their own measurements and processes directly under their control, which substantially lessens the burden of data collection and resource investment for all members of the supply chain. In addition, measurements of energy use and input products/materials may already be available with known error estimates from quality control programs. In this case, calculating the gate-to-gate PCF would consist of simply collecting and organizing data already available for other purposes, and would represent a minimal investment in resources.
▪ Example of proposed framework
The connections between the constituent partial PCFs of the total life cycle PCF can be illustrated by various circuits of mass, energy and associated information in our model. This is most clearly shown by rearranging the basic elements of the model as shown in , where transportation is central for the movement of mass, energy and information through the economy. For instance, let us consider the supply chain for an aluminum beverage can into the economy (in a simplified fashion). Ore is provided by the environment and extracted in an open pit mine using heavy equipment, diesel fuel and electricity. In this example, the information flow would be as follows. Metal starts as ore in the environment, is extracted at the mine and converted to aluminum stock (; circuit 1). GHG emissions from these primary production processes are shown as GHGs-P . Finished aluminum is then shipped to a can and beverage manufacturer via circuits 2 and 3. Because the transportation stage was used (as required for delivery) en-route to the can and beverage manufacturer, this manufacturer takes ownership of these transport-related GHG emissions (shown as GHGs-T in ) and adds them to its own process emissions (GHG emissions from these processes are shown as GHGs-S in ). This same accounting approach would apply to all receiving firms/actors in this model. A finished can is filled and then shipped to the retailer (a secondary producer in our model) via circuits 4 and 3. The retailer takes ownership of transport emissions from this circuit and adds them to their process-related emissions (i.e., electricity consumption for lighting and refrigeration). The can is then delivered to the consumer following circuits 4 and 5, either by shipping or personal transport by the consumer. Emissions associated with this transport are owned by the consumer and added to the consumer-use stage emissions (GHGs-C in ), such as electricity consumption for refrigeration of the can at home. Following consumption, the aluminum can travels to a disposal site following circuits 6 and 7 and the emissions associated with disposal are captured in GHGs-D . Thus, the simplified circuit in this example, neglecting inputs for the production of the actual beverage (which would each require a similar circuit), is 1–2–3–4–3–4–5–6–7, where iterations of a specific circuit represent multiple secondary producers.
Alternatively, if the can is recycled into aluminum stock, GHG emissions at the primary producer level would be reduced because of the absence of the ore extraction phase and the circuit would be 8–9–2–3–4–3–4–5–6–7 for direct delivery of recyclables to the primary producer. Direct delivery (8–9) could also be replaced by routing through a separate recycling plant (a secondary producer) before delivery to the primary producer (8–3–4–9). This framework clearly defines the boundaries for each firm in the supply chain and actor in the life cycle, as well as assigns transport related emissions to each firm and actor. As an example, the primary producer would be responsible for quantifying the emissions associated with circuit 1 and their processing, but would not incorporate transportation emissions from shipping to the first secondary producer. Likewise, a landfill would quantify emissions from its operations and take ownership of transportation emissions for collection of waste from consumers.
Embodied within each of these circuits are the emissions associated with energy use, which would require that each entity have a quantification of these emissions. In the case of fuel for transportation, this would require an emissions inventory from well-head to refinery to distribution center. In this case the same framework can be utilized, where the transportation company is effectively the consumer, with the refinery being the secondary producer and the oil field owner being the primary producer. The flow of materials and energy also applies to electricity production; for instance, from natural gas, which would require an emissions inventory from well-head to generating plant and through the grid to any actor in the system. Here, the primary producer is the owner of the well field, while the secondary producers include: the gas pipeline owner (transport of gas from the well field), the generating plant owner (electricity production) and the electric grid owner (transmission of electricity). The energy consumer is the end-user in the life cycle of electricity production.
Although we have oversimplified the global supply chain and the complexities involved with mining, petroleum production and electrical generation, the above example outlines an alternative to working through the system backwards, as is the current approach to PCF. The proposed approach, instead, has each producer (primary and secondary) focus on their own contribution to the total life cycle product carbon footprint. Thus, for each producer, the PCF becomes a more manageable activity. After each circuit transferring mass and energy between secondary producers (4–3 circuits), the downstream producer merely adds their own GHG emissions to the growing life cycle PCF and passes the aggregated PCF value on along with the physical (or intangible) item that is being shipped to the downstream receiver. Each producer must also quantify the error in their gate-to-gate partial PCF. This error will then propagate downstream from primary producer to secondary producer, and from secondary producer to secondary producer. As long as errors are accurately quantified at each processing step and accurately propagated through the supply chain, tracking error in this manner allows for more accurate uncertainty bounds in estimates of PCF. As multiple iterations of this framework occur through the real economy, uncertainty will be more accurately assessed because emissions from each input (e.g., embodied emissions in capital goods, among others) will be quantified, reducing the need for downstream producers to estimate upstream emissions. This work is not outside the scope of normal business, and has already begun within the minerals and metals sector, with uncertainty being a primary concern for wider adoption Citation[32].
▪ Data sharing
Frameworks for public data sharing and aggregation have been discussed by previous authors for Life Cycle Inventories (LCI) Citation[103] and are commonly termed ‘open source LCI’. A similar model can be used for PCF. Several open access data sources are available and work to expand open access data has been underway for some time Citation[26]. Examples include the National Renewable Energy Laboratory’s LCI database Citation[104], Carnegie Mellon’s EIO LCA tool Citation[105] and the European Commission Joint Research Centre’s European Reference Life Cycle Database Citation[106], among others. These data sources are important first steps in the open source LCI/open PCF process, and incorporation of uncertainty information has already begun Citation[29,21]. However, the open source LCI/open PCF process and all data resources could benefit from increased uncertainty analysis and precise, brand-specific information on the individual producer level. To facilitate data sharing and ease of accessibility, individual producers could post their cradle-to-gate and gate-to-gate PCF to individual websites for use by downstream producers. The data could also be deposited in a central repository for open access on the web. However, these data would require third-party verification to ensure their validity prior to posting.
▪ Barriers & incentives
Individual firm financial barriers & incentives
The alternative framework addresses two financial barriers facing firms with regard to carbon accounting. First, firms may not have funds available or set aside for carbon accounting activities. Second, when funds are available, firms may see little financial incentive to conduct carbon accounting. Through the alternative framework, PCF can be initiated with minimal upfront investment compared with backward-looking PCF because a firm is only analyzing operations under their control. Therefore, individual firms can focus their resources on in-house processes that they may already have detailed information on (i.e., through quality control programs) utilizing in-house expertise. This substantially reduces the resource investment necessary to contact suppliers and gather data. Focusing efforts in-house can also provide financial incentives by allowing firms to identify potential efficiency gains within the processes analyzed.
The data requirements for conducting a PCF inventory analysis are the same data required for efficiency auditing. A focus on an individual firm’s gate-to-gate PCF not only allows for potential incorporation into total life cycle PCF, but also informs the firm about unit processes that can be targeted for both decarbonzation and efficiency improvement. Efficiency improvements that lead to lower resource consumption (be it energy or input materials) provide a means to lower emissions while offering a positive return on investment. In this manner, the alternative framework redirects resources that may otherwise be dedicated to backtracking emissions in a supply chain toward both financial gain and emissions reductions. The alternative framework moves a firm beyond GHG accounting and into profitable GHG reduction.
Supply chain financial incentives
The potential for increased efficiency at the scale of the individual firm gate-to-gate should reinforce the viability of PCF for both reducing emissions and increasing profits. Even if upstream data are unavailable, efficiency gains can be made within the supply chain by individual firms and production costs of intermediary products potentially decreased. Thus, the alternative framework for PCF of a product can help reduce the resource intensity and overall costs of finished products through similar reductions for inputs. Lowering the cost of the final product offers the potential for increased market share if more resource-intensive products are displaced by a lower-cost alternative.
The open PCF framework also leads to efficiency gains in conducting PCF for the entire supply chain. Each gate-to-gate PCF completed lowers the costs for downstream producers to consider their own life cycle PCF by reducing the amount of resources required to conduct an inventory analysis. Not only is the effect to reduce costs (and these costs reductions will most likely be proportional to the number of firms who pursue a gate-to-gate PCF), but will also result in better industry averages for comparison of an individual firm’s production system and PCF. Cost-effective PCF also translates into cost-effective labeling of goods, considering that conducting the PCF is the most resource-intensive component of PCFL. The open-PCF framework, therefore, provides a means to cheaply conduct accurate PCFs and provide reliable information for PCFL.
These financial incentives are enjoyed by the whole supply chain, not a single firm. Such distributed incentives may not motivate individual firms with small supply chains; however, they may represent a significant motivation for supply chains where substantial vertical integration is present. In vertically integrated supply chains, individual firms may depend heavily on one another, and cooperation for mutual benefit through increased sales can be an attractive proposition.
Confidentiality & disclosure barriers & incentives
In order for the open PCF process to be successful and life cycle PCF calculated, individual firms must disclose their gate-to-gate PCF information to downstream producers. However, individual firms currently enjoy the right to confidentiality with regard to PCF information. Therefore, firms must be provided with an incentive to disclose their PCF data. As discussed above, PCF can lead to lower costs in the supply chain with the subsequent financial benefits of increased market share. In addition to these benefits, PCFL can offer market incentives for firms to disclose PCF data through branding and subsequent increased market share. PCFL market share increase, however, occurs only if the market demands lower PCF products. As has been noted by some, high demand for low carbon footprint products has not been widely demonstrated Citation[8]. Possible future incentive scenarios and outlooks for PCFL are outlined in the ‘Open PCF & future PCFL work’ section below.
Disclosure disincentives also exist for PCF. If a producer determines that his gate-to-gate PCF is substantially higher than a competitor, it is not in the interests of that producer to disclose his PCF information. If the market does demand lower PCF products, the high carbon intensity producer may lose market share. However, application of the alternative framework can still provide individual firm incentives without disclosure of PCF data through the open PCF process. Efficiency gains realized through the alternative framework can result in financial gain and decarbonization of the supply chain without any two firms ever communicating or disclosing their PCF data; the only information that need be exchanged is price adjustments. In this manner, the alternative framework provides a means by which decarbonization can be achieved at a profit in complete confidentiality. This benefit is not offered by backward-looking PCF approaches. Of course, without disclosure of individual firm PCF data the life cycle PCF cannot be calculated and PCFL cannot be supported. Whereas we do not advocate for PCF without disclosure, proceeding with the alternative framework and conducting gate-to-gate PCF without disclosure can provide incentives to begin PCF work without the disincentives presented by disclosure. However, regulatory intervention may be necessary to adequately address the disclosure disincentives. Future regulatory options are discussed in the ‘Future regulatory support of open PCF & PCFL’ section below.
▪ Product use & disposal
In PCF, the use/maintenance and end-of-life stages of the product life cycle quantify GHG emissions that will occur in the future from the supply chain’s perspective, and therefore are inherently uncertain. Incorporation of these emissions into a PCF is difficult. The producer closest to retail distribution or direct consumer purchase (e.g., online retailers) is likely to have the most knowledge on expected consumer behavior and how their final product is intended to be used. However, because a product’s use and ultimate disposal are not under the direct control of the producer, direct data gathering and aggregation of emissions from this stage may not be possible through the proposed framework. Expressing the GHG footprint per unit of consumption (based on a representative disposal scenario) could help to inform consumers about the possible impacts of their consumption. Post-purchase consumer research might also allow for the estimation of average product usage, as well as the quantification of uncertainty in these life cycle stages.
Future perspective
▪ PCRs, PCF standards & environmental product declarations
PCRs have been proposed and developed to guide producers in specific industries to enhance comparability and promote standardization of PCF and associated environmental product declarations (EPDs) Citation[4,107]. These rules suggest standard boundaries for PCF, common emissions factors to be used within a given industry and other unifying methodological considerations. PCRs and PCF standards could benefit from the results of open PCF, as outlined here, by enabling individual producers to more precisely account for their unique supply chains and individual processing methods. Standardized estimation of GHG emissions in the use/maintenance and end-of-life disposal scenarios within a given product category or industry may be the greatest contribution these rules and standards have to make in PCF. Development of both PCRs and standards should focus on collecting and disseminating actual primary data with quantified error for all unit processes used in applicable supply chains, and periodically updating data as supply chains develop. These efforts may help to increase confidence in comparative decisions made between products based on EPDs.
▪ Information technology & logistics
PCF can benefit from recent developments in information technology. Ubiquitous access to the internet allows for virtually free posting of PCF information. The spread of technologies, such as radio frequency identification technology for logistics purposes, can enable automated tracking of PCF information and real-time transportation data for calculating GHG emissions at the individual shipment level. This technology is already being investigated in Japan, where electronic monitoring of truck fuel economy is coupled with radio frequency identification tags in individual packages Citation[8]. This electronic monitoring allows for direct measurement of carbon footprints that can change daily for an individual product Citation[8]. Application of such automated information technologies to other unit processes in the supply chain has the capacity to more accurately track uncertainty by replacing estimates of unit process data with direct measurements, while lowering the cost of collecting and sharing PCF information by reducing the associated labor costs. Whereas there are real barriers to adoption of such technologies, including cost Citation[8] and compatibility, application of information technologies for PCF may provide fertile grounds for future research.
▪ Open PCF implementation issues & integration with current standards
Implementation of the alternative framework will bring about similar difficulties encountered by previous voluntary GHG reporting programs. These will include methods of ensuring transparency and accuracy, and establishing public trust for PCF data reported to consumers. In addition, open PCF must achieve methodological consistency, validity and reliability for data to be correctly and accurately aggregated throughout the supply chain. The methodological complexities encountered through application of LCA techniques in PCF addressed by the current standards must also be addressed in open PCF (e.g., allocation of emissions among co-products) Citation[5,6]. This alternative framework does not offer inherent advantages over current PCF standards with regard to these implementation issues. In addition, other carbon accounting initiatives are addressing issues of including more life cycle GHGs in their inventories (such as the inclusion of emissions embodied in capital goods by the Greenhouse Gas Protocol’s Corporate Value Chain [Scope 3] Accounting and Reporting Standard) Citation[33]. However, this alternative framework does offer unique financial incentives not clearly apparent in the current PCF standards, as well as an economically efficient and self-improving system of data dissemination and increasing accuracy of uncertainty estimates. For these reasons, we do not propose that the alternative framework for open PCF replace current standards, nor stand apart in isolation from them. Instead, the principles proposed here would most effectively be implemented via incorporation into current standardization efforts (PAS 2050, for instance, does support gate-to-gate PCF) Citation[6]. One potential advantage over current PCF methodology is that open PCF can provide a funding source for PCF and implementation strategies. Cost savings and increased profits realized through application of the alternative framework could generate a source of funding to support third-party verification programs, while the narrowed focus of gate-to-gate partial PCFs could potentially reduce the costs associated with verification.
▪ Open PCF & future PCFL work
Application of open PCF for PCFL of consumer products can provide a cost-effective means of supplying PCF data for EPD programs, as previously discussed, providing a potential incentive over backward looking PCF methodologies. Future work to incentivize open PCF in PCFL programs could include establishment of EPDs that preserve confidentiality of unit process data, but still disclose aggregated, finished product PCF data gathered through open PCF, thereby removing confidentiality disincentives. Such a program could be supported by third-party verification to ensure methodological transparency without compromising security of trade secrets. Some preliminary research has shown an increased acceptance of such simplified PCF EPDs (such as a stoplight presentation with three tiers of PCF intensity) over detailed numerical disclosures Citation[34]. Additionally, the incentives created by branding and potential market share increase may become greater as the economic and political environment surrounding climate change evolves throughout North America. In the grey literature, Terrachoice (a member of the Underwriters Laboratories network) noted a 73% increase in the number of products carrying ‘green claims’ in retail stores in North America from 2007 to 2009 Citation[108]. From 2009 to 2010, an additional 79% increase was noted with 5296 products carrying 12,061 green claims Citation[109]. These claims were not all related to climate impact, but do provide an interesting glimpse into the expectations of marketing strategies and the hope for branding through EPDs. Future work in this area could include case studies evaluating actual market share increase through PCFL based branding and comparison to costs accrued through application of the alternative framework.
▪ Future regulatory support of open PCF & PCFL
Terrachoice also noted that in 2010, 95% of the green claims were displaying some amount of ‘greenwashing’ (or deceptive marketing practices) by their own rubric Citation[109]. Their work suggests that validity of data in PCFL will continue to be a concern in future years. Whereas financial incentives may induce firms to engage in PCFL, regulatory intervention may be necessary to ensure transparency and accountability. The work of Terrachoice, among other influences, contributed to the revised ‘green guides’ drafted by the US Federal Trade Commission (FTC) Citation[110], the federal enforcement agency that regulates marketing claims in the USA. The green guides provide guidance to marketers on what is considered deceptive marketing practice with regard to product environmental claims. Of interest is that the green guides specifically exclude guidance on product claims based on LCA (e.g., PCF), due to variability of methodology and disagreement within the practice Citation[110]. If regulatory support is to be sought in the future with regard to PCF, it will inevitably involve PFCLs and the FTC.
Incorporation of regulatory incentives (including disincentives through enforcement) will be an interesting area of work in the years to come. Achieving standardization in PCF that is acceptable to the FTC for inclusion in the green guides is one possible goal. Mandatory PCF labeling required through the FTC is another. Such a labeling program will be in effect for light bulbs in the USA in 2012 Citation[111], although the focus is transparency in energy consumption and bulb performance. Similarly, mandatory PCF labeling through regulation can address the disclosure disincentives presented in open PCF by requiring PCF data disclosure, while establishing consistent and transparent accounting methodology. In time, product performance standards for carbon intensity could be phased in, similar to performance standards for light bulbs in the USA taking effect in 2012 Citation[112]. Regardless of the regulatory approach taken, PCF data will need improvement in accuracy and transparency in order to support arguments for PCF based rules, which can be achieved in a cost-effective manner through open PCF.
Conclusion
The open PCF framework outlined here is analogous to proposed carbon tax systems. The idea behind some carbon tax proposals is to levy the tax at the most upstream point in the fossil fuel supply chain (i.e., at the well head for oil), with resulting increased costs being passed on to downstream consumers. In the PCF framework, however, the flow through the free-market supply chain is information rather than money, and the effect is not to increase costs for downstream producers and consumers, but to potentially lower them. Furthermore, the experience and data structures gained from open PCF will be tractable to any environmental impact category of interest and other footprinting efforts (e.g., water footprinting).
The standard for life cycle assessment International Organization for Standardization (ISO) 14040 defines a ‘product’ as any product or service. Fitting with the ISO 14040 definition, the term ‘product’ is used in this article to refer to all goods and services produced and provided in the economy. ISO 14040 offers the following categories of products (reproduced from the ISO 14040 standard):
▪ Services (e.g., transport); | |||||
▪ Software (e.g., computer program and dictionary); | |||||
▪ Hardware (e.g., engine mechanical part); | |||||
▪ Processed materials (e.g., lubricant); | |||||
▪ An activity performed on a customer-supplied tangible product (e.g., automobile to be repaired); | |||||
▪ An activity performed on a customer-supplied intangible product (e.g., the income statement needed to prepare a tax return); | |||||
▪ The delivery of an intangible product (e.g., the delivery of information in the context of knowledge transmission); | |||||
▪ The creation of ambience for the customer (e.g., in hotels and restaurants). |
Product carbon footprinting encompasses all economic activity using this definition of product. Unlike carbon accounting techniques that focus on an individual firm, product carbon footprinting focuses on individual products. Through full life cycle accounting of all products, theoretically, all anthropogenic GHGs can be accounted for.
As discussed by Wright et al., the term ‘carbon footprint’ can be ambiguous with respect to which GHGs are included in the emissions inventory Citation[3]. For instance, does the term imply only carbon containing GHGs be accounted for or all GHGs? Similarly, should the carbon footprint include only Kyoto Protocol GHGs and exclude GHGs covered by the Montreal Protocol? As an alternative, Wright et al. and others have proposed use of the term ‘climate footprint’ to refer to an inventory that includes all GHG species (including noncarbon-containing species), as is typical of a thorough life cycle assessment. We assert that the life cycle nature of product carbon footprinting (PCF) justifies inclusion of all GHGs for the purposes of PCF. Inclusion of all GHGs in PCF has potential benefits when a firm is attempting to identify the most cost-effective means of reducing GHG emissions and identifying potential efficiency gains. Exclusion of any GHG can cause a firm to lose out on potential efficiency gains for processes involving excluded gases, although the relative contribution of each GHG to a firm’s inventory is dependent on inputs to individual production systems. For instance, the US EPA estimates the average US supermarket store emits 1383 metric tonnes CO2eq/year from electricity consumption, while the same store emits 1556 metric tonnes CO2eq/year through refrigerant leakage Citation[113]. Exclusion of refrigerants from the GHG inventory would result in omitting 53% of the total GHGs and prevent the supermarket from exploring GHG reduction options through refrigerant retrofitting. Without elucidation of the total GHG emissions profile of a firm’s production systems, relative cost–effectiveness of emission reduction measures cannot be carried out. The term PCF is used in this article for consistency with previous publications. However, fitting with the convention of a climate footprint, our conception of PCF could also be labeled product climate footprinting. Regardless of the term chosen, PCF in this article refers to a process in which the GHG inventory includes all GHG species involved in a product’s life cycle.
A life cycle assessment-based technique for quantifying GHG emissions resulting from all life cycle stages of a product.
Method of quantifying the environmental impacts resulting from all life stages of a product or process.
Freely available and accessible to all parties, including the public.
Introduction
• In the absence of GHG regulations, emission reduction actions must provide a positive return on investment. | |||||
• Product carbon footprinting (PCF) provides process-specific data, which can be used to identify potential efficiency gains in supply chains and production systems, allowing for emission reductions and cost savings. | |||||
• Despite efforts to develop PCF standards, prohibitive costs and data uncertainty are problematic. |
Background
• Using standard PCF methodology, carbon footprint values have been demonstrated to change as much as 1900% when process-specific primary data are lacking. | |||||
• An alternative approach to PCF is needed to meet the demands of decision making. |
Alternative framework
• Data gaps can be filled by individual producers focusing only on processes and associated uncertainty under their direct control in a gate-to-gate manner. | |||||
• A life cycle PCF starts with primary producers at the point of natural resource extraction, conducting an initial cradle-to-gate PCF, followed by downstream secondary producers adding their own gate-to-gate emissions to the growing life cycle PCF provided by upstream producers, resulting in a compartmentalized and aggregated PCF. | |||||
• Through compartmentalizing tasks and using an open data sharing framework, generation of life cycle PCF can be made manageable and cost effective for all firms. |
Barriers & incentives
• The alternative framework shows capacity for future reductions in overall production costs and increased market share for individual firms. | |||||
• The open PCF process makes conducting PCF’s more efficient, and can further reduce the costs of high quality GHG emissions inventories and carbon labeling programs. |
Future perspectives
• The unique financial incentives, self-improving system of data acquisition and potential funding opportunities offered by the open PCF process would best be implemented through integration with current standardization, Product Category Rule (PCR), and Product Carbon Footprint Labeling (PCFL) development efforts. | |||||
• The open PCF process can provide cost effective and accurate data to support regulatory rule making, such as PCF guidance in the US Federal Trade Commission Green Guides and product carbon intensity performance standards. |
Conclusions
• Open PCF information propagates through the economy much as a well-head carbon tax would; however, the effect of open PCF will be to reduce costs for all downstream actors in the economy instead of increasing them. |
Financial & competing interests disclosure
The authors have no relevant affiliations or financial involvement with any organization or entity with a financial interest in or financial conflict with the subject matter or materials discussed in the manuscript. This includes employment, consultancies, honoraria, stock ownership or options, expert testimony, grants or patents received or pending, or royalties.
No writing assistance was utilized in the production of this manuscript.
References
- IPCC. Climate Change 2007: Synthesis Report. Contribution of Working Groups I, II and III to the Fourth Assessment Report of the Intergovernmental Panel on Climate Change. Pachauri RK, Reisinger A (Eds). IPCC, Geneva, Switzerland, 104 (2007).
- Pacala S, Socolow R. Stabilization wedges: solving the climate problem for the next 50 years with current technologies. Science305(5286),968–972 (2004).
- Wright LA, Kemp S, Williams I. ‘Carbon footprinting’: towards a universally accepted definition. Carbon Management2(1),61–72 (2011).
- Micallef-Borg C. Product carbon footprinting: calculation and communication standards in the making. Climate Carbon Law Rev.2,178–189 (2010).
- World Resources Institute, World Business Council for Sustainable Development. Product Accounting and Reporting Standard. Draft. World Resources Institute, Washington, DC, USA (2010).
- British Standards Institution. Specification for the Assessment of the Life Cycle Greenhouse Gas Emissions of Goods and Services. PAS 2050. British Standards Institution, London, UK (2008).
- Vandenbergh MP, Dietz T, Stern P. Time to try carbon labeling. Nature Climate Change1,4–6 (2011).
- McKinnon AC. Product-level carbon auditing of supply chains environmental imperative or wasteful distraction? Int. J. Phys. Distribution Logistics Manag.40(1/2),42–60 (2009).
- Sinden G. The contribution of PAS 2050 to the evolution of international greenhouse gas emission standards. Int. J. Life Cycle Assess.14,195–203 (2009).
- SETAC Europe LCA Steering Committee. Standardisation efforts to measure greenhouse gases and ‘carbon footprinting’ for products. Int. J. Life Cycle Assess.13(2),87–88 (2008).
- Plassmann K, Norton A, Attarzadeh N, Jensen MP, Brenton P, Edward-Jones G. Methodological complexities of product carbon footprinting: a sensitivity analysis of key variables in a developing country context. Environ. Sci. Policy13,393–404 (2010).
- Vankatesh A, Jaramillion P, Griffin WM, Matthews HS. Uncertainty analysis of life cycle greenhouse gas emissions from petroleum-based fuels and impacts on low carbon fuel policies. Environ. Sci. Technol.45,125–131 (2011).
- International Organization for Standardization. Environmental Management. Life Cycle Assessment. Principles and Framework. International Organization for Standardization, Geneva, Switzerland (2006).
- Finkbeiner M. Carbon footprinting – opportunities and threats. Int. J. Life Cycle Assess.14,91–94 (2009).
- Weidema BP, Thrane M, Christensen P, Schmidt J, Lokke S. Carbon footprint a catalyst for life cycle assessment? J. Ind. Ecol.12(1),3–6 (2008).
- International Organization for Standardization. Environmental Management. Life Cycle Assessment. Requirements and Guidelines. International Organization for Standardization, Geneva, Switzerland (2006).
- World Resources Institute, World Business Council for Sustainable Development. A Corporate Accounting and Reporting Standard (Revised Edition). World Resources Institute, Washington, DC, USA (2004).
- British Standards Institution. How to Assess the Carbon Footprint of Goods and Services. Guide to PAS 2050. British Standards Institution, London, UK (2008).
- Basset-Mens C, Kelliher FM, Ledgard S, Cox N. Uncertainty of global warming potential for milk production on a New Zealand farm and implications for decision making. Int. J. Life Cycle Assess.14,630–638 (2009).
- Hong J, Shaked S, Rosenbaum RK, Jolliet O. Analytical uncertainty propagation in life cycle inventory and impact assessment: application to an automobile front panel. Int. J. Life Cycle Assess.15,499–510 (2010).
- De Konigg A, Schowanek D, Dewaele J, Weisbrod A, Guinee J. Uncertainty in a carbon footprint model for detergents; quantifying the confidence in a comparative result. Int. J. Life Cycle Assess.15,79–89 (2010).
- Roos E, Sundberg C, Hansson PA. Uncertainties in the carbon footprint of food products: a case study on table potatoes. Int. J. Life Cycle Assess.15,478–488 (2010).
- Whitaker J, Ludley KE, Rowe R, Taylor G, Howard DC. Sources of variability in greenhouse gas and energy balances for biofuel production: a systematic review. Glob. Change Biol. Bioenergy2,99–112 (2010).
- Basset-Mens C, van der Werf HMG, Durand P, Leterme P. Implications of uncertainty and variability in the life cycle assessment of pig production systems. Int. J. Life Cycle Assess.11(5),298–304 (2006).
- Malca J, Freire F. Uncertainty analysis in biofuel systems: an application to the life cycle of rapeseed oil. J. Ind. Ecol.14(2),322–334 (2010).
- Finnveden G, Hauschild MZ, Ekvall T et al. Recent developments in life cycle assessment. J. Environ. Manag.91,1–21 (2009).
- Matthews HS, Hendrickson CT, Weber CL. The importance of carbon footprint estimation boundaries. Environ. Sci. Technol.42(16),5839–5842 (2008).
- Gnansounou E, Dauriat A, Villegas J, Panichelli L. Life cycle assessment of biofuels: energy and greenhouse gas balances. Bioresour. Technol.100,4919–4930 (2009).
- Llyod M, Ries R. Characterizing, propagating, and analyzing uncertainty in life-cycle assessment a survey of quantitative approaches. J. Ind. Ecol.11(1),161–179 (2007).
- Reap J, Roman F, Duncan S, Bras B. A survey of unresolved problems in life cycle assessment part 1: goal and scope and inventory analysis. Int. J. Life Cycle Assess.13,290–300 (2008).
- Berners-Lee M, Howard DC, Moss J, Kaivanto K, Scott WA. Greenhouse gas footprinting for small businesses – the use of input-output data. Sci. Total Environ.409,883–891 (2011).
- Yellishetty M, Ranjith PG, Tharumarajah A, Bhosale S. Life cycle assessment in the minerals and metals sector: a critical review of selected issues and challenges. Int. J. Life Cycle Assess.14,257–267 (2009).
- World Resources Institute, World Business Council for Sustainable Development. Corporate Value Chain (Scope 3) Accounting and Reporting Standard. World Resources Institute, Washington, DC, USA (2011).
- Upham P, Dendler L, Bleda M. Carbon labelling of grocery products: public perceptions and potential emissions reductions. J. Cleaner Prod.19,348–355 (2011).
▪ Websites
- National Oceanic and Atmospheric Administration. Earth System Research Laboratory, trends in atmospheric CO2. www.esrl.noaa.gov/gmd/ccgg/trends
- New Belgium Brewery and the Climate Conservancy. The carbon footprint of Fat Tire® amber ale. www.newbelgium.com/Files/the-carbon-footprint-of-fat-tire-amber-ale-2008-public-dist-rfs.pdf
- Sam Walton School of Business. White paper: open source lifecyle inventory. A collaborative approach to creating business, environmental and social values. http://asc.uark.edu/348.asp
- National Renewable Energy Laboratory. US life cycle inventory database. www.nrel.gov/lci
- Carnegie Mellon EIO LCA Tool. www.eiolca.net
- ELCD core database version II. http://lca.jrc.ec.europa.eu/lcainfohub/index.vm
- The International EPD System. www.enviondec.com
- Terrachoice. The seven sins of greenwashing 2009. http://sinsofgreenwashing.org/findings/greenwashing-report-2009
- Terrachoice. The sins of greenwashing 2010. http://sinsofgreenwashing.org/findings/greenwashing-report-2010
- Federal Register Notice for proposed revised green guides, 2010. www.ftc.gov/os/fedreg/2010/october/101006greenguidesfrn.pdf
- US Federal Trade Commission. FTC extends deadline for new lighting facts labels to January 1, 2012. www.ftc.gov/opa/2011/04/bulblabeling.shtm
- US DOE. New lighting standards begin in 2012. www.energysavers.gov/your_home/lighting_daylighting/index.cfm/mytopic=11977
- US EPA. Profile of an average US supermarket’s greenhouse gas impacts from refrigeration leaks compared to electricity consumption. www.epa.gov/greenchill/downloads/GC_AverageStoreProfile_FINAL_JUNE_2011_REVISED.pdf