Abstract
Background: Reactive oxygen species are associated with inflammation implicated in cancer, atherosclerosis and autoimmune diseases. The complex nature of inflammation and of oxidative stress suggests that dual-target agents may be effective in combating diseases involving reactive oxygen species. Results: A novel series of N-substituted 2,4-diaminopteridines has been synthesized and evaluated as antioxidants in several assays. Many exhibited potent lipid antioxidant properties, and some are inhibitors of soybean lipoxygenase, IC50 values extending down to 100 nM for both targets. Several pteridine derivatives showed efficacy at 0.01 mmol/kg with little tissue damage in a rat model of colitis. 2-(4-methylpiperazin-1-yl)-N-(thiophen-2-ylmethyl)pteridin-4-amine (18f) at 0.01 mmol/kg exhibited potent anti-inflammatory activity (reduction by 41%). Conclusion: The 2,4-diaminopteridine core represents a new scaffold for lipoxygenase inhibition as well as sustaining anti-inflammatory properties.
Oxidative stress is closely associated with chronic inflammation and plays a crucial role in cancer [Citation1], dyslipidemia [Citation2], atherosclerosis [Citation3] and autoimmune diseases such as systemic lupus erythematosus and rheumatoid arthritis [Citation4]. In many diseases, the rate of production of reactive oxygen species (ROS) is increased compared with normal levels of ROS [Citation5,Citation6]. ROS are produced during the inflammatory process by phagocytic leukocytes that invade the tissue. Under conditions of oxidative stress, ROS including superoxide anion, hydroxyl radical and hydrogen peroxide and their reactive products can attack various biological macromolecules (e.g., proteins, enzymes, DNA and lipids) resulting in DNA mutations, lipid peroxidation and protein oxidation, [Citation7] or they may indirectly interfere with mechanisms of DNA repair [Citation8]. Thus, ROS activity consists of a mixture of deleterious and beneficial roles, depending on the type, concentration and location of the species involved. The breadth of those factors suggests that focused targeting of ROS, probably in a dual-target or multiple-target approach, could be of therapeutic value.
Reagents and conditions: (A) Na, ethanol, reflux. 2 h; (B) methyl iodide, ethanol, reflux 1.5 h; (C) NaNO2 aqueous acetic acid, 0°C, 2 h then 4°C, 16 h; (D) 1-methylpiperazine ethanol, reflux 0.75 h then add water and reflux 0.75 h; (E) sodium dithionite then aqueous 40% glyoxal (5a) or butane-2,3-dione (5b, or benzil [5c]), reflux 8 h, 18 h and 24 h, respectively.
![Figure 2. Synthesis of pteridines (5).Reagents and conditions: (A) Na, ethanol, reflux. 2 h; (B) methyl iodide, ethanol, reflux 1.5 h; (C) NaNO2 aqueous acetic acid, 0°C, 2 h then 4°C, 16 h; (D) 1-methylpiperazine ethanol, reflux 0.75 h then add water and reflux 0.75 h; (E) sodium dithionite then aqueous 40% glyoxal (5a) or butane-2,3-dione (5b, or benzil [5c]), reflux 8 h, 18 h and 24 h, respectively.](/cms/asset/3ecd2469-f47d-49c7-96af-e3e08c0ccbfe/ifmc_a_12363343_f0002.jpg)
Reagents and conditions: (A) 1-methylpiperazine, reflux, 18 h; (B) NaNO2 aqueous acetic acid, 0°C, 3 h; (C) sodium dithionite then aqueous 40% glyoxal, reflux 7 h; (D) NaHB(OAc)3 (9 eq for 10a and 1 eq for 10b), acetic acid 48 h, 20°C.
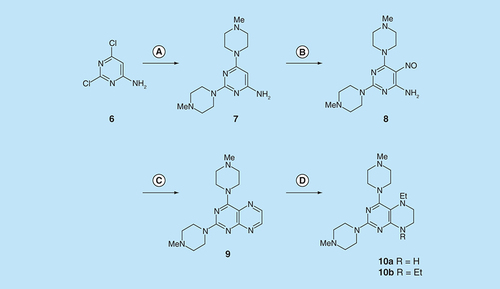
Reagents and conditions: (A) 3-hydroxypiperazine, reflux 5 h; (B) NaNO2 aqueous acetic acid 0°C, 3 h; (C) sodium dithionite then aqueous 40% glyoxal, reflux 6 h.
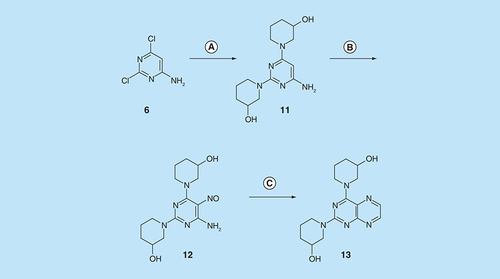
Reagents and conditions: (A) R1CH2NH2 diglyme, reflux, 5 h; (B) NaNO2 aqueous acetic acid, 0°C, 2 h then 4°C, 16 h; (C) 1-methylpiperazine or 4-methyl-1,4-diazepane, ethanol, reflux, 2 h then add water and reflux 1 h; (D) sodium dithionite then aqueous 40% glyoxal or butane-2.3-dione, reflux.
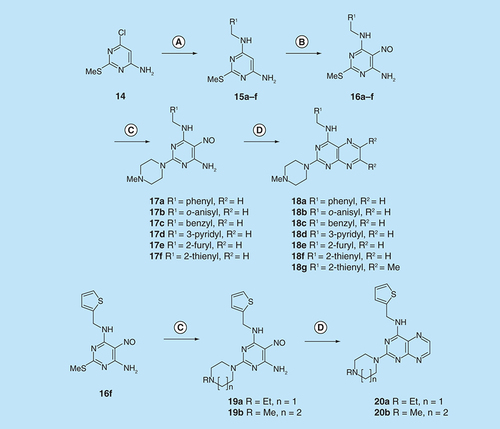
Reagents and conditions: (A) H2, 10% Pd on C, ethanol; then NaNO2, glacial acetic acid, 90°C, 2 h; (B) sodium thiosulfate pentahydrate, aq. 20% acetic acid 90°C, 1.5 h; (C) lead tetraacetate, acetic acid, 20°C, 4 h.
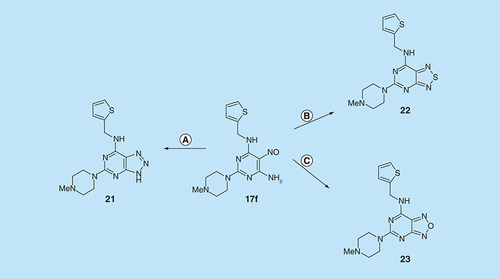
Energy minimizations were carried out using the AMBER99SB-ILDN force field [Citation41] with GROMACS as the molecular simulation toolkit [Citation42]. AutoDock Vina (1.1.2) [Citation41] was used for docking. Iron is rendered as a brown sphere. Prepared using PyMOL, this figure represents the preferred pose according to scoring function.
![Figure 7. Docking pose of pteridine 18d (depicted in turquoise) bound to soybean lipoxygenase (LOX-1) derived by modification of PDB code: 3PZW.Energy minimizations were carried out using the AMBER99SB-ILDN force field [Citation41] with GROMACS as the molecular simulation toolkit [Citation42]. AutoDock Vina (1.1.2) [Citation41] was used for docking. Iron is rendered as a brown sphere. Prepared using PyMOL, this figure represents the preferred pose according to scoring function.](/cms/asset/c6fa3bc8-3f7b-41da-be5d-2afa6a09e389/ifmc_a_12363343_f0007.jpg)
Reactive oxygen species are centrally involved in the cyclooxygenase (COX)- and lipoxygenase (LOX)-mediated conversion of arachidonic acid (AA) into proinflammatory intermediates [Citation9,Citation10]. LOX exerts its biological role via a carbon-centered radical mechanism. LOX metabolism results in many bioregulatory molecules such as leukotrienes, lipoxins and hepoxylins, mediators in the pathophysiology of variety of diseases such as rheumatoid arthritis, bronchial asthma, psoriasis, cancer and other inflammatory diseases [Citation11].
In the search for new antioxidants and anti-inflammatory agents, the pteridine ring () was selected for study for several reasons: many naturally-occurring derivatives possess important biological activity, including the coenzymes 5,6,7,8-tetrahydrobiopterin and the pterins; the ring system has low toxicity; synthetic pteridine derivatives show a wide range of clinically useful properties, for example, as antioxidants, immunosuppressants, and anti-inflammatory and anticancer agents. Consequently, multitarget properties were deemed likely, as were the discovery of new agents for a range of diseases, and also the use of pteridine derivatives to probe the putative targets of diseases.
Substituted pteridines are known to act upon a wide range of targets of therapeutic potential. Thus, a 6,7-disubstituted-2,4-diaminopteridine is a PI3 kinase inhibitor with potential for treatment of myocardial infarction involving ischemia reperfusion injury [Citation12]. 2-Amino-4-piperazin-1-yl-6-(3,4-dimethoxyphenyl)-pteridines have been shown to possess immunosuppressive and anti-inflammatory properties [Citation13], and analogues of the potent phosphodiesterase-4 inhibitor 7-benzylamino-6-chloro-2-piperazin-1-yl-4-pyrrolidin-1-ylpteridine which inhibits growth in tumor cell lines required a 2-piperazin-1-yl substituent for optimal potency [Citation14]. Some 2-amino-4-(N,N-diarylmethyl)-6-arylpteridines inhibit neuronal nitric oxide synthase [Citation15] and some 2,4-diamino-4,6-diarylpteridines inhibit inducible or inflammatory nitric oxide synthase [Citation16]. Several 2,4-diamino-6-arylaminomethylpteridines are potent inhibitors of parasitic pteridine reductases, and have promising antiparasitic potential [Citation17,Citation18]. Adequate dietary content of folic acid (pteroyl l-glutamic acid; ) is a requirement for human health and on which DNA synthesis, DNA repair and DNA methylation depend [Citation19].
In addition to the treatment of a variety of cancers, the antifolate methotrexate reduces inflammation in the bowel and is used for treating Crohn's disease, ulcerative colitis, rheumatoid arthritis, psoriasis and other autoimmune diseases. Therefore, novel compounds bearing the pteridine ring could exhibit anti-inflammatory and antioxidant activities and have potential as therapy for a wide range of diseases involving inflammation. The 2,4-diaminopteridine core of methotrexate (), was used as the scaffold in this study. Alkylamino and dialkylamino substituted 2,4-diaminopteridines were investigated since many have a range of drug-like clogP values (1.0–4.0; ) and because synthesis should afford the required structural diversity. We describe here the synthesis and preliminary evaluation of some novel N-alkylated 2,4-diaminopteridine derivatives as dual-target agents through their radical scavenging properties, inhibition of lipoxygenase and in vivo anti-inflammatory activity.
Results & discussion
Chemistry
Routes to 2,4-diaminopteridine derivatives with no substituents at the 6- or 7-postions are sparse. In particular, very few such compounds contain a tertiary amine at the 2-position (). Two relevant 6,7-disubstituted pteridines had been prepared by reaction of 4-amino-2-methylthio-6,7-diphenylpteridine with morpholine and with piperidine to give the corresponding 2-substituted 4-amino-6,7-diphenylpteridines, but a large excess of the amine was used as the solvent, and it was not known whether this route would permit the introduction of amines other than amino at the 4-position, since only 4,5-diamino-2-methylthiopyrimidine has been used as the early precursor in this route [Citation20]. Accordingly, it was decided to introduce the desired 2,4-diamino substitution prior to the formation of the pteridine ring. Since dimethylamine, pyrrolidine and piperidine had been shown to displace the 2-methylthio group of 4,6-diamino-2-methylthio-5-nitrosopyrimidine (3) [Citation21], the route envisaged here involved 5-nitrosation, 2-amination, then reduction and cyclization to give the pteridine ring system ().
4,6-diamino-2-thiopyrimidine (1), prepared by condensing thiourea and malononitrile in the presence of sodium ethoxide [Citation22], was S-methylated to give 4,6-diamino-2-methylthiopyrimidine (2) by heating with ethanolic methyl iodide at reflux [Citation20]. In a modification of the literature procedure [Citation23], treatment of 2 in aqueous sodium nitrite containing acetic acid afforded the nitrosopyrimidine 3 (95%) which with 1-methylpiperazine in ethanol at reflux was converted into the 2-(4-methylpiperazin-1-yl)-5-nitrosopyrimidine 4 (57%). Reduction of 4 with aqueous sodium dithionite afforded the corresponding tetraamine which without isolation was treated with aqueous 40% glyoxal and then heated at reflux to give 2-(4-methylpiperazin-1-yl)pteridin-4-amine (5a). In the same way, butane-2,3-dione and benzil afforded the corresponding pteridines 5b (68%) and 5c (59%).
For 2,4-diaminopteridine derivatives bearing identical 2- and 4-substitution, 6-amino-2,4-dichloropyrimidine was heated at reflux with the appropriate secondary amine to give the triamino-substituted pyrimidines 7 (71%) and 11 (89%) ( & ). Using the previous procedure, nitrosation afforded 8 (98%) and 12 (71%) which with aqueous sodium dithionite afforded the tetraamines, and again without isolation those were treated with aqueous 40% glyoxal to afford the corresponding pteridine derivatives 9 (48%) and 13 (41%). The diol 13 was obtained as a mixture of two diastereoisomers that were not separated. Reduction using sodium triacetoxyborohydride (9 mole equivalents) in glacial acetic acid gave the 5-ethyl-5,6,7,8-tetrahydropteridine 10a (29%), after column chromatography. However, when one mole equivalent of triacetoxyborohydride was used, the 5,8-diethyl-5,6,7,8-tetrahydropteridine 10b (46%) was obtained, after column chromatography.
For dissimilar substitution at the 2- and 4-positions of pteridine-2,4-diamine derivatives, a stepwise introduction of those substituents was required. 2,4-dichloropteridine [Citation24] and its derivatives [Citation25] react regioselectively at the 4-position with one equivalent of amine, which makes this approach unsuitable for preparing a range of pteridines with the same 2-amino substituent. Additionally, the use of 2,4,6,7-tetrachloropteridine for related successive displacements with different amines, while succinct, afforded extensive mixtures and with isolation of desired single regioisomers usually in low yields, after chromatography [Citation14]. Since the formation of pteridines from 5-nitrosopyrimidines had proved robust and had delivered single regioisomers, this approach was adopted, in which the 4-amino group would be introduced by displacement of 4-amino-6-chloro-2-(methylthio)pyrimidine 14 (). However, displacement of the chloro group had been reported only for pyrimidine 14, using dimethylamine to install a 4-dimethylamino group [Citation26]. 4,6-Diamino-2-methylthiopyrimdines such as 15 would be required, and would be obtained by displacement of 14 with benzylamine derivatives or with heteroarylmethylamines (). Displacement of the 2-methylthio group in 16 by a secondary amine would give the unsymmetrically substituted 2,4,6-triamino-5-nitrosopyrimidines, and hence the corresponding pteridines 18.
4-Amino-6-chloro-2-(methylthio)pyrimidine 14 reacted with a variety of amines (2.1 equivalent) in diglyme at reflux to give the corresponding pyrimidines 15a–f [Citation27] which underwent 5-nitrosation with acidic aqueous sodium nitrite () [Citation23]. The 2-methylthio group of the resulting 5-nitrosopyrimdines 16a–f underwent displacement with 1-methylpiperazine or 4-methyl-1,4-diazepane in ethanol at reflux to give the corresponding 2-amino derivatives 17a–f. Those were subjected to the above reduction with sodium dithionite followed by condensation with aqueous 40% glyoxal to give the pteridine derivatives 18a–g. Compounds 20a and 20b were prepared analogously, the latter by using 4-methyl-1,4-diazepane in ethanol at reflux to give 19b. 1H NMR spectra of the nitroso compounds 16, 17 and 19 in chloroform are consistent with the presence of two rotamers arising from hydrogen bonding between the C-5 nitroso oxygen atom and the adjacent NH hydrogen atoms at C-4, and also with an NH hydrogen atom at C-6, as established for related pyrimidines [Citation28–30].
A representative 5-nitrosopyrimidine, 17f, was transformed into three 6,5-fused systems () in a brief survey of the relevance of the fused pyrazine ring in the pteridine series. The triazolo[4,5-d]pyrimidine 21 was obtained by hydrogenation of 17f to the corresponding amine, nitrosation and subsequent ring closure at 90°C, following standard methods [Citation31]. The 5-nitrosopyrimdine 17f was also converted into 22 by sodium thiosulfate [Citation32], and into 23 by lead tetraacetate [Citation33]. Synthetic procedures for the new pteridine derivatives are described in detail in the Supplementary Information. All new compounds showed spectroscopic data consistent with the structures proposed. The purity of tested compounds was assessed as at least 95% by HPLC–MS, unless otherwise indicated.
In vitro lipoxygenase inhibition
The substituted pteridines prepared were assayed for inhibition of soybean lipoxygenase () [Citation34,Citation35] (Supplementary Information). Many studies have used readily obtainable soybean lipoxygenase, which is a homologue of mammalian lipoxygenase and well examined [Citation36,Citation37]. The availability of soybean LOX and its well-characterized crystal structure [Citation38] led to its use in this study.
In the soybean LOX inhibition assay () the three 4-amino-2-(4-methylpiperazin-1-yl)pteridines (entries 1–3) showed only weak inhibition, with increasing bulk of 6,7-substituents not enhancing potency; similarly, the 6,7-dimethyl substitution in compound 18g lowered LOX inhibitory activity, the 6,7-unsubstituted pteridine 18f exhibiting the greater potency. Substitution at the 4-amino group generally increased potency, and within the (hetero)arylmethyl series, polarity in a substituent (18b) or in the appended ring (18d) further increased potency, in the latter case vary greatly. However, the 4-(hetero)arylmethyl series and the 4-(phenethylamine)pteridine 18c showed only moderate potency. Comparison of 18f with 20a and 20b shows that both 2-(4-ethylpiperazin-1-yl) and 1-ethyl-1,4-diazepanyl groups conferred lower potency compared with a 2-(4-methylpiperazin-1-yl) moiety. Of the compounds containing a 4-(4-methylpiperazin-1-yl) substituent (entries 4–6), 9 was the most potent pteridine, and 10a the most potent 5,6,7,8-tetrahydropteridine, both having IC50 = 5 μM for inhibition of LOX. However, 5,8-diethyl substitution, as in 10b, was less well tolerated than the 8-unsubstituted 10a. A limitation on ring tolerance was also identified; the 3-hydroxypiperidin-1-yl 2,4-disubstitution in 13 conferred some tenfold less potency than the preferred 2,4-di-(4-methylpiperazin-1-yl) substitution present in 9. The pyrimido[4,5-d]azoles 21–23, containing the standard 2- and 4-substituents, were only moderately potent LOX inhibitors. Lastly, the lipophilicities of the pteridines were considered since a correlation of lipophilicity with LOX inhibition has been reported [Citation39] in other studies but was not detected here [Citation34]. Lipophilicity, as assessed using calculated clogP values of the substituted pteridines (), does not appear to inhibit significantly in vitro LOX, for example, pteridine 9 and the 5,6,7,8-tetrahydropteridine 10a showing equipotent inhibition of LOX (IC50 = 5 μM) although their clogP values differ by 2.5; however, the most potent LOX inhibitor identified, 18d (IC50 = 0.10 μM), does have a relatively low clogP (0.92).
The LOX inhibition data () show that the 4-amino substituent plays a crucial role in determining the potency of the substituted pteridine. Thus, although a 4-benzylamino group (entry 8) is almost equipotent to an unsubstituted amino group (entry 5a), a 4-(4-methylpiperazin-1-yl) group (entry 4) shows some tenfold increase in potency. However, a 3-hydroxypiperidin-1-yl moiety (entry 7) affords only moderate LOX inhibition. A nitrogen atom in the 4-substituent can confer excellent potency (entry 11). Soybean LOX is able to accommodate the very rigid 4-methylpiperazin-1-yl group present in 9 (IC50 = 5.0 μM) although the flexible (3-pyridylmethyl)amino group present in 18d (IC50 = 0.10 μM) confers much greater potency. That both substituents are proton acceptors is consistent, in each case, with the distal nitrogen atom engaging in hydrogen bonding. Entries 5 and 8 () suggest that in regard to LOX inhibition some steric bulk is tolerated at the 5-position, but is much less well tolerated at the 8-position of the pteridine ring. Although no definite conclusions can currently be drawn, tolerance of some substituents at the 6- and/or 7-positions seems likely.
Molecular modeling of LOX
Being the most potent inhibitor of soybean LOX of the compounds studied and also possessing efficacy as an antioxidant, pteridine derivative 18d was selected for in silico docking. The molecular modeling study performed (see Supplementary Information for details) provided useful interpretation of the experimental results. The preferred docking orientation for compound 18d is shown in . The binding of 18d to soybean LOX (PDB code: 3PZW) has a higher AutoDock Vina score (-8.5 kcal/mol) than any of the other pteridines docked. Pteridine 18d is able to accommodate the extensively hydrophobic cavity close to the active site, incorporating Ile552, Ile553, Ile538 and Leu546 among other residues. Ile553 and especially Leu496 are proximate to the hydrophobic 6,7-flank of the pteridine ring, Ile553 also extending to the hydrophobic C4-C6 region of the pyridine ring in 18d. The increased potency of 18d over its phenyl analog 18a is considered to be due to hydrogen binding, perhaps to Ser747. The simplest explanation is that the extension scaffold of 18d into the hydrophobic domain blocks approach of substrates to the active site, and hence prevents oxidation by soybean LOX. The docking simulations of NDGA and 18d show a common pattern of interaction with LOX (Supplementary Figure 1), the terminal rings and central core of each compound showing appreciable overlap. Additionally, Ser747 is engaged in hydrogen bonding with the 3-hydroxyl group of the catechol unit of NDGA, and also with the pyridine nitrogen atom of 18d. The relatively weak antioxidant properties of 18d () are also consistent with the main mode of action of LOX inhibition being other than by diminishing general ROS concentrations and hence antioxidant activity [Citation40]. Preliminary screening tests of the pteridines against COX did not present any significant inhibition.
In vitro antioxidant activity
Previous work has shown that pterins, their dihydro- and their tetrahydro-derivatives can each be antioxidants or pro-oxidants, depending on the particular conditions [Citation43]. In the present study, several assays were used to assess in vitro antioxidant activity in order to obtain representative information; each method involves the generation of a different radical. The three assays chosen measured in vitro antioxidant activity in terms of: reduction of the stable free radical 1,1-diphenyl-2-picrylhydrazyl (DPPH), whose oxidized form possesses an absorption maximum at 517 nm; hydroxyl radical scavenging activity; extent of reduction of the water-soluble 2,2′-azo-bis(2-amidinopropane) dihydrochloride (AAPH) and inhibition of soybean lipoxygenase (Supplementary Information).
The pteridine derivatives were evaluated for their antioxidant activity () and compared with that of nordihydroguaiaretic acid (NDGA), the reference compound [Citation39]. Although most compounds at 100 μM did not show significant reducing ability, key exceptions were the potent 5-ethyl-5,6,7,8-tetrahydropteridine 10a (81%) and very potent 5,8-diethyl-5,6,7,8-tetrahydropteridine 10b (97%) (), the latter being more effective than the reference compound.
Competition of the novel pteridine derivatives with dimethyl sulfoxide for hydroxyl radicals was measured. Hydroxyl radicals were generated using the Fe3+/ascorbic acid system and expressed as a percentage inhibition of formaldehyde production in the presence of each pteridine derivative at 100 μM () [Citation34]. Pteridine derivatives 5a, 10b and 18g strongly inhibited the oxidation of dimethyl sulfoxide (33 mM). The majority of the derivatives were excellent scavengers of hydroxyl radicals with activity higher than the reference compound 6-hydroxy-2,5,7,8-tetramethylchroman-2-carboxylic acid (Trolox).
Azo compounds that generate free radicals through spontaneous thermal decomposition are useful for in vitro studies of free radical production. The water-soluble AAPH has been extensively used as a clean and controllable source of thermally produced alkylperoxyl free radicals [Citation44]. In this assay, compound 18g (IC50 = 0.1 μM) was the most potent in protecting against lipid peroxidation; next, and almost equipotent, were the pteridines 13 and 18e, and the triazolo[4,5-d]pyrimidine 22 and the oxadiazole[3,4-d]pyrimidin-4-amine 24 (each of approximate IC50 = 0.3 μM). The 4-(thiophen-2-yl)methylamino-substituted pteridine derivatives showed a range of three orders of magnitude, compound 18g showing by far the greatest protection against lipid peroxidation, the 2-(4-ethylpiperazin-1-yl) derivative 20a being moderate, the 1,4-diazepane derivative 20b being poor and the 2-(4-methylpiperazin-1-yl) derivative 18f being very weak. Evidently, the presence of 6,7-dimethyl groups on the pteridine ring greatly enhances protection against lipid peroxidation; the nature of the alkyl group and ring size on the 2-substituent has some, but much less, effect. Of the 4-aminopteridines, the 6,7-diphenyl derivative 5c was by far the most potent inhibitor, whereas of the 2,4-bis(4-methylpiperazin-1-yl) derivatives, 13 is more than twice as potent as the 2,4-(3-hydroxydipiperidin-1-yl derivative 9. Reduction of the pteridine ring in compound 9 to the corresponding 5,6,7,8-tetrahydropteridines 10a and 10b decreased protection of lipid peroxidation, with potency decreased by 13-fold and 20-fold, respectively. Conversely, reduction of the pteridine ring in the 4-(4-methylpiperazin-1-yl) derivative 18f to give 18i increased potency by a factor of about 2.5. In the 6,5-fused heteroaromatic compounds studied, 22 and 24 showed similar and potent inhibition of lipid peroxidation whereas compound 23 was half as potent. The role of lipophilicity (as assessed from calculated clogP values; ) is not clear, but substituent bulk plays a significant role.
In vivo anti-inflammatory activity
LOX has also been associated with inflammation and ulcerative colitis [Citation45]. In the present study, a model for colitis involving intracolonic administration of aqueous 4% acetic acid in the rat was used, leading to acute inflammatory reaction [Citation46]. Treated rats presented partial to diffuse petechial bleeding, single erosion and limited ulceration indicating an overall healing effect of the compounds. Substituted pteridines presenting a satisfactory combination of activities 5a, 18a, 18d and 18f were tested using this in vivo model characterized by diffuse exfoliated mucosa as well as multiple and extended erosion and ulcers of the colon (). No mortality was encountered. Pteridine 5a (score 1–2) was the most potent in this series, followed by its 4-(N-benzyl) analog, 18a. Rats treated with 5a or 18a showed less loss in body weight compared with the control group.
6-chloro-2-(4-methylpiperazin-1-yl)-N-(thiophen-2-ylmethyl)quinazolin-4-amine possesses in vivo anti-inflammatory properties in the rat [Citation47], so on the basis of its close structural analogy with pteridine 18f, that latter was tested for anti-inflammatory effects using the carrageenin paw edema model (). The incipient pattern of this edema is characterized by the effects of histamine and 5-hydroxytryptamine. After 1 h, reduction of edema in the rat paw achieved by the pteridine 18f was appreciably greater than the reduction induced by the reference compound indomethacin, a nonselective COX-inhibitor and commonly used nonsteroidal anti-inflammatory drug. Thus, 18f and some related pteridines offer significant protection against reactive oxygen species produced in a model of colitis, probably on account of their properties as antioxidants and radical scavengers.
Conclusion
A general synthetic approach to N, N,N′-trialkylated and N,N,N′N′-tetraalkylated 2,4-diaminopteridines has been described. 2,4-diaminopteridine derivatives have been identified as a new and promising class of radical scavengers, anti-inflammatory agents and inhibitors of LOX. Potent inhibitors of soybean lipoxygenase include 9, 10a and especially 18d (IC50 = 0.1 μM). To our knowledge, 2,4-aminopteridine is a novel scaffold for LOX inhibitors, although the extent of any LOX isoform selectivity remains to be established. Many of the pteridine derivatives studied displayed potent radical-scavenging activity, especially 5c, 9, 13, 18e, 18g, 22 and 24, of which 18g is the most potent (IC50 = 0.1 μM) in the linoleic acid peroxidation assay. Several pteridine derivatives showed efficacy at 0.01 mmol/kg with little tissue damage in a rat model of colitis. 2-(4-methylpiperazin-1-yl)-N-(thiophen-2-ylmethyl)pteridin-4-amine (18f) at 0.01 mmol/kg showed 60% greater reduction of edema in rat paw than that achieved by the anti-inflammatory agent indomethacin, a nonselective COX-inhibitor. Accordingly, this study demonstrates that some pteridine derivatives have at least a dual-target action. These results prompt a more detailed structural, mechanistic and medicinal investigation of substituted 2,4-diaminopteridines, whose therapeutic potential might lead to new agents for the treatment of inflammatory bowel disease, among other inflammatory diseases.
Future perspective
Inflammation is a multifactorial phenomenon that is implicated in a wide range of diseases. Enhanced formation of ROS by phagocytic leukocytes during the process of inflammation leads to tissue dysfunction and damage in a number of pathological conditions. ROS oxidize lipids generating peroxides and aldehydes that have pronounced biological effects including damage to DNA and protein, selective alterations in cell signaling and cytotoxicity [Citation42]. Oxidative stress evidently plays a crucial role in those processes.
Given the importance of radical species in inflammation there is an unmet and timely need for new radical-scavenging agents. In addition, multiple-target anti-inflammatory agents have potential for the control of a range of diseases including arthritis, cancer and atherosclerosis. The wide-ranging biological activities of pteridine derivatives, including the reduction in reperfusion injury by the 4-amino analog of tetrahydrobiopterin [Citation48], suggest that pteridines may find therapeutic applications in unexplored or little-charted areas. New pteridine derivatives could also be of value as probes of specific biological oxidation.
Table 1. Inhibition of soybean lipoxygenase by substituted pteridines.
Table 2. Reducing ability in 2,2-diphenyl-1-picrylhydrazl assay, scavenging activity of hydroxyl radicals, and in vitro antilipid peroxidation activity of substituted pteridines.
Table 3. In vivo colitis studies†.
Table 4. Inhibition of carrageenin-induced rat paw edema.
Oxidative stress: Cellular damage resulting from the action of reactive oxygen species on biological molecules in which detoxification of the reactive intermediates and/or molecular repair is insufficient.
Inflammation: Pattern of biological responses arising in attempts to overcome challenges by harmful stimuli, including damaged cells, irritants or pathogens.
Reactive oxygen species: Chemically reactive oxygen species such as anions, radical anions, radicals and peroxides formed as by-products of metabolism.
Lipoxygenases: Iron-containing redox enzymes that catalyze the oxygenation of fatty acids, lipoproteins and other molecules.
Pteridines: Bicyclic heteroaromatic compounds comprising pyrazino[2,3-d]pyrimidine ring fusion.
Reactive oxygen species are associated with inflammation implicated in cancer, atherosclerosis and autoimmune diseases. The pteridine ring, suitably substituted, offers a diverse array of biological activity with potential for the treatment of those diseases.
New N-alkylated 2,4-diaminopteridines were prepared in good chemical yields and possess drug-like properties.
N-alkylated 2,4-diaminopteridines were shown to possess a range of antioxidant properties and to be inhibitors of lipoxygenase, depending on their N-substitution.
N-alkylated 2,4-diaminopteridines are dual-target agents, and can be radical scavengers and inhibitors of lipoxygenase. Some also exhibited potent anti-inflammatory properties, either in an in vivo model of colitis or in a model of odema.
Ethical conduct of research
The authors state that they have obtained appropriate institutional review board approval or have followed the principles outlined in the Declaration of Helsinki for all human or animal experimental investigations. In addition, for investigations involving human subjects, informed consent has been obtained from the participants involved.
Pteridine-2,4-diamine Derivatives as Radical Scavengers and Inhibitors of Lipoxygenase that can possess Anti-Inflammatory Properties
Download MS Word (1.3 MB)Acknowledgements
Use of the C-QSAR program (A Leo, Biobyte Corp., 201 West 4th Str., Suite 204, Claremont, CA 91711, USA) and is acknowledged and D Davies is thanked for helpful discussions.
Financial & competing interests disclosure
Financial support from the Onassis Public Benefit Foundation for a postdoctoral fellowship to E Pontiki is gratefully acknowledged. Financial support from Wellcome for a vacation bursary scholarship to T Tran is also acknowledged. The authors have no other relevant affiliations or financial involvement with any organization or entity with a financial interest in or financial conflict with the subject matter or materials discussed in the manuscript apart from those disclosed.
No writing assistance was utilized in the production of this manuscript.
Additional information
Funding
References
- Glasauer A , ChandelNS. Targeting antioxidants for cancer therapy. Biochem. Pharmacol.92 (1), 90–101 (2014).
- Ku IA , ImbodenJB, HsuePY, GanzP. Rheumatoid arthritis: model of systemic inflammation driving atherosclerosis. Circ. J.73 (6), 977–985 (2009).
- Rho YH , ChungCP, OeserAet al. Interaction between oxidative stress and high-density lipoprotein cholesterol is associated with severity of coronary artery calcification in rheumatoid arthritis. Arthritis Care Res. (Hoboken)62 (10), 1473–1480 (2010).
- Khan F , SiddiquiAA. Prevalence of anti-3-nitrotyrosine antibodies in the joint synovial fluid of patients with rheumatoid arthritis, osteoarthritis and systemic lupus erythematosus. Clin. Chim. Acta370 (1–2), 100–107 (2006).
- Uttara B , SinghAV, ZamboniP, MahajanRT. Oxidative stress and neurodegenerative diseases: a review of upstream and downstream antioxidant therapeutic options. Curr. Neuropharmacol.7 (1), 65–74 (2009).
- Waris G , AhsanH. Reactive oxygen species: role in the development of cancer and various chronic conditions. J. Carcinog.5, 14 (2006).
- Lambeth JD , KrauseKH, ClarkRA. NOX enzymes as novel targets for drug development. Semin. Immunopathol.30 (3), 339–363 (2008).
- Vera-Ramirez L , Sanchez-RoviraP, Ramirez-TortosaMCet al. Free radicals in breast carcinogenesis, breast cancer progression and cancer stem cells. Biological bases to develop oxidative-based therapies. Crit. Rev. Oncol. Hematol.80 (3), 347–368 (2011).
- Garrido G , GonzálezD, DelporteCet al. Analgesic and anti-inflammatory effects of Mangifera indica L. extract (Vimang). Phytother. Res.15 (1), 18–21 (2001).
- Weber V , RubatC, DurouxE, LartigueC, MadesclaireM, CoudertP. New 3- and 4-hydroxyfuranones as anti-oxidants and anti-inflammatory agents. Bioorg. Med. Chem.13 (14), 4552–4564 (2005).
- Pontiki E , Hadjipavlou-LitinaD. Targeting lipoxygenases (LOs): drug design and discovery. Curr. Enzyme Inhibition9 (2), 89–105 (2013).
- Palanki MS , DneprovskaiaE, DoukasJet al. Discovery of 3,3′-(2,4-diaminopteridine-6,7-diyl)diphenol as an isozyme-selective inhibitor of PI3K for the treatment of ischemia reperfusion injury associated with myocardial infarction. J. Med. Chem.50 (18), 4279–4294 (2007).
- De Jonghe S , MarchandA, GaoLJet al. Synthesis and in vitro evaluation of 2-amino-4-N-piperazinyl-6-(3,4-dimethoxyphenyl)-pteridines as dual immunosuppressive and anti-inflammatory agents. Bioorg. Med. Chem. Lett.21 (1), 145–149 (2011).
- Merz KH , MarkoD, RegiertT, ReissG, FrankW, EisenbrandG. Synthesis of 7-benzylamino-6-chloro-2-piperazino-4-pyrrolidinopteridine and novel derivatives free of positional isomers. Potent inhibitors of cAMP-specific phosphodiesterase and of malignant tumor cell growth. J. Med. Chem.41 (24), 4733–4743 (1998).
- Matter H , KotsonisP, KlinglerOet al. Structural requirements for inhibition of the neuronal nitric oxide synthase (NOS-I): 3D-QSAR analysis of 4-oxo- and 4-amino-pteridine-based inhibitors. J. Med. Chem.45 (14), 2923–2941 (2002).
- Ma F , LüG, ZhouWF, WangQJ, ZhangYH, YaoQZ. Synthesis and biological evaluation of 2,4-diaminopteridine derivatives as nitric oxide synthase inhibitor. Chinese Chem. Lett.20 (4), 420–422 (2009).
- Cavazzuti A , PagliettiG, HunterWNet al. Discovery of potent pteridine reductase inhibitors to guide antiparasite drug development. Proc. Natl Acad. Sci. USA105 (5), 1448–1453 (2008).
- Corona P , GibelliniF, CavalliAet al. Structure-based selectivity optimization of piperidine-pteridine derivatives as potent Leishmania pteridine reductase inhibitors. J. Med. Chem.55 (19), 8318–8329 (2012).
- Langie SAS , AchterfeldtS, GorniakJPet al. Maternal folate depletion and high-fat feeding from weaning affects DNA methylation and DNA repair in brain of adult offspring. FASEB27, 3323–3334 (2013).
- Taylor EC , CainCK. Pteridines. VII. The synthesis of 2-alkylaminopteridines. J. Am. Chem. Soc.74 (7), 1644–1647 (1952).
- Cresswell RM , StraussT. Displacement of the 2-methylmercapto group in pyrimidines bearing a 5-nitroso substituent. J. Org. Chem.28 (10), 2563–2564 (1963).
- Bendich A , TinkerJF, BrownGB. A synthesis of isoguanine labeled with isotopic nitrogen. J. Am. Chem. Soc.70 (9), 3109–3113 (1948).
- Baddiley J , LythgoeB, McneilD, ToddAR. Experiments on the synthesis of purine nucleosides. Part I. Model experiments on the synthesis of 9-alkylpurines. J. Chem. Soc.74, 383–386 (1943).
- Su Q , IoannidisS, ChuaquiCet al. Discovery of 1-methyl-1H-imidazole derivatives as potent Jak2 inhibitors. J. Med. Chem.57 (1), 144–158 (2014).
- Daly JW , ChristensenBE. The preparation and properties of certain 2- and 4-chloro substituted pteridines. J. Am. Chem. Soc.78 (1), 225–228 (1956).
- Baker BR , JosephJP, SchaubRE. Puromycin. Synthetic studies. Synthesis of 6-dimethylaminopurine, an hydrolytic fragment. J. Org. Chem.19 (4), 631–637 (1954).
- Ward AD , BakerBR. Irreversible enzyme inhibitors. 200. Active-site-directed inhibitors of deoxycytidine kinase. J. Med. Chem.20 (1), 88–92 (1977).
- Procházková E , CechováL, JanebaZ, DracínskyM. A switchable intramolecular hydrogen bond in polysubstituted 5-nitrosopyrimidines. J. Org. Chem.78 (20), 10121–10133 (2013).
- Zhang F-L , XuM, VasellaA. A new synthesis of pteridines substituted with branched and linear alkenyl groups at C(6). The nitroso-ene reaction of 4-(alkenoylamino)-5-nitrosopyrimidines. Helv. Chim. Acta90 (3), 521–534 (2007).
- Marchal A , NoguerasM, SánchezAet al. Alkoxy-5-nitrosopyrimidines: useful building block for the generation of biologically active compounds. Eur. J. Org. Chem.2010 (20), 3823–3830 (2010).
- Fu J , YangY, ZhangXW, MaoWJ, ZhangZM, ZhuHL. Discovery of 1H-benzo[d][1,2,3]triazol-1-yl-3,4,5-trimethoxybenzoate as a potential antiproliferative agent by inhibiting histone deacetylase. Bioorg. Med. Chem.18 (24), 8457–8462 (2010).
- Nagamatsu T , IslamR, AshidaN. Facile synthesis and evaluation of antitumor and antiviral activities of [1,2,5]thiadiazolo[3,4-d]pyrimidines (8-thiapurines) and 4-β-D-ribofuranosyl[1,2,5]thiadiazolo[3,4-d]pyrimidines. Heterocycles72, 573–588 (2007).
- Boyle PH , LockhartRJ. Preparation of 5-amino-7(6H)-furazano[3,4-d]pyrimidinone an analog of pterin. Tetrahedron40 (5), 879–886 (1984).
- Pontiki E , Hadjipavlou-LitinaD, LitinasK, NicolottiO, CarottiA. Design, synthesis and pharmacobiological evaluation of novel acrylic acid derivatives acting as lipoxygenase and cyclooxygenase-1 inhibitors with antioxidant and anti-inflammatory activities. Eur. J. Med. Chem.46 (1), 191–200 (2011).
- Pontiki E , Hadjipavlou-LitinaD. Synthesis and pharmacochemical evaluation of novel arylacetic acid inhibitors of lipoxygenase, antioxidants, and anti-inflammatory agents. Bioorg. Med. Chem.15 (17), 5819–5827 (2007).
- Minor W , SteczkoJ, BolinJT, OtwinowskiZ, AxelrodB. Crystallographic determination of the active site iron and its ligands in soybean lipoxygenase L-1. Biochemistry32 (25), 6320–6323 (1993).
- Skrzypczak-Jankun E , AmzelLM, KroaBA, FunkMO. Structure of soybean lipoxygenase L3 and a comparison with its L1 isoenzyme. Proteins29 (1), 15–31 (1997).
- Chruszcz M , WlodawerA, MinorW. Determination of protein structures – a series of fortunate events. Biophys. J.95 (1), 1–9 (2008).
- Pontiki E , Hadjipavlou-LitinaD. Antioxidant and anti-inflammatory activity of aryl-acetic and hydroxamic acids as novel lipoxygenase inhibitors. Med. Chem.2 (3), 251–264 (2006).
- Denisov ET , Afanas'evIB. Oxidation and Antioxidants in Organic Chemistry and Biology. CRC Press, FL, USA (2005).
- Pettersen EF , GoddardTD, HuangCCet al. UCSF Chimera – a visualization system for exploratory research and analysis. J. Comput. Chem.25, 1605–1612 (2004).
- Fiser A , SaliA. Modeller: generation and refinement of homology-based protein structure models. Meth. Enzymol.374, 461–491 (2003).
- Oettl K , ReibneggerG. Pteridine derivatives as modulators of oxidative stress. Curr. Drug Metabol.3 (2), 203–209 (2002).
- Betigeri S , ThakurA, RaghavanK. Use of 2,2′-azobis(2-amidinopropane) dihydrochloride as a reagent tool for evaluation of oxidative stability of drugs. Pharm. Res.22 (2), 310–317 (2005).
- Hassan GS , SolimanGA. Design, synthesis and anti-ulcerogenic effect of some of furo-salicylic acid derivatives on acetic acid-induced ulcerative colitis. Eur. J. Med. Chem.45 (9), 4104–4112 (2010).
- Fabia R , WillénR, Ar'rajabA, AnderssonR, AhrénB, BengmarkS. Acetic acid-induced colitis in the rat: a reproducible experimental model for acute ulcerative colitis. Eur. Surg. Res.24 (4), 211–225 (1992).
- Smits RA , de EschIJ, ZuiderveldOPet al. Discovery of quinazolines as histamine H4 receptor inverse agonists using a scaffold hopping approach. J. Med. Chem.51 (24), 7855–7865 (2008).
- Brandacher G , ZouY, ObristP, SteurerW, Werner-FelmeyerG, MargreiterR. The 4-amino analogue of tetrahydrobiopterin efficiently prolongs murine cardiac-allograft survival. J. Heart Lung Transplant.20, 747–749 (2001).