Abstract
The desirable physical and physiochemical properties of clay minerals have led them to play a substantial role in pharmaceutical formulations. Clay minerals like kaolin, smectite and palygorskite-sepiolite are among the world's most valuable industrial minerals and of considerable importance. The elemental features of clay minerals which caused them to be used in pharmaceutical formulations are high specific area, sorption capacity, favorable rheological properties, chemical inertness, swelling capacity, reactivity to acids and inconsiderable toxicity. Of course, these are highly cost effectual. This special report on clay minerals provides a bird's eye view of the chemical composition and structure of these minerals and their influence on the release properties of active medicinal agents. Endeavor has been made to rope in myriad applications depicting the wide acceptability of these clay minerals.
With a variegated range of physical, chemical and physicochemical properties, clay minerals have an undisputable role in pharmaceutical industries. The minerals employed primarily are oxides, carbonates, sulphates, chlorides, hydroxides, sulphides, phosphates, nitrates, borates and phyllosilicates. These are widely put into use owing to their unique features. The significant contributions of these minerals are in therapeutic, cosmetics and as bulk forming agents. These are biologically compatible and hence ensure the genesis of safe and effective drug delivery systems.
Figure 1. Basic composition of clay minerals.
Diagrammatic representation of the (A) octahedral sheet and (B) tetrahedral sheet.
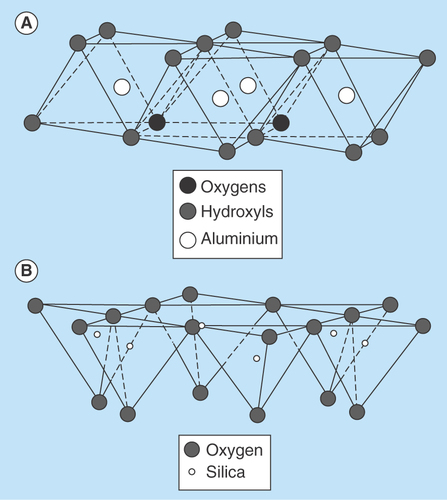
Clay, a group of natural minerals with plastic properties are primarily composed of hydrous-layer silicates of aluminium, occasionally containing magnesium and iron particles of smaller size, in other words, less than 2 μm (7.9 × 10−5 inch). Hence, in broader terms, clay minerals practically involve minerals of the above-cited particles size. These are essentially composed of silica, alumina, magnesia, iron and water with varying degree of potassium, sodium and calcium [Citation1].
Besides clay minerals no other minerals quite as immensely attract pharma people. Since the 19th century clay minerals have been explored on the geological, geotechnical and mineralogical fronts; their myriad therapeutic aspects were highlighted primarily in the pharmaceutical research. Clay minerals are a class of phyllosilicates which usually form as a result of chemical weathering of silicate minerals at the surface of the Earth [Citation2]. Clay minerals are widely used in the pharmaceutical industry as lubricants, desiccants, disintegrants, diluents, binders, pigments and opacifiers. The other vital uses are as emulsifying, thickening, isotonic and anticaking agents. These also serve as flavor correctors and carriers of active ingredients. Other unique features are dispersivity, hygroscopicity, unctuosity, thixotropy and their rendering a slightly alkaline reaction as pH is slightly more than 7, plasticity, opacity and various high-quality colors [Citation3].
Composition & structural features of clays
Clay minerals are primarily of a fine-grained natural material with particle size <2 μm [Citation4]. The physical and chemical properties of a particular clay mineral are dependent on its structure and composition. The structure and composition of the major industrial clays, in other words, kaolins, smectites and palygorskite–sepiolite, are very different even though each is comprised of octahedral and tetrahedral sheets as their basic building blocks. However, the arrangement and composition of the octahedral and tetrahedral sheets, as depicted in , account for most differences in their physical and chemical properties [Citation5]. Clay minerals are stacked, polymeric sandwiches of tetrahedral and octahedral sheet structures. They are classified first into ‘layer types,’ differentiated by the number of tetrahedral and octahedral sheets that have combined, and then into ‘groups,’ differentiated by the kinds of isomorphic cation substitution that have occurred [Citation6,Citation7]. Clay minerals can be classified into seven groups as illustrated in . Thus, mineral products for pharmaceutical use vary according to composition, crystallinity, habit and texture, greatly affecting their properties [Citation8]. The individual layers of clay minerals are composed of two, three or four sheets [Citation9]. The sheets are formed either by tetrahedrons [SiO4]4-, abbreviated as ‘T’ or by octahedrons, for example, [AlO3(OH)3]6-, abbreviated as ‘O.’ The interior of tetrahedrons and octahedrons contain smaller metal cations, their apices being occupied by oxygen, which are with protons (as OH). All these fundamental structural elements are arranged to form a hexagonal network in each sheet. Based on the number and the ratio of sheets in a fundamental structural layer, the existing cation substitutions in the octahedrons and tetrahedrons and based on the resulting charge of the layers, the crystalline clay minerals are classified [Citation10]. A detailed description of the chemical composition of the various clay minerals is given in .
Some predominantly employed clay minerals are kaolinite, 2SiO2·Al2O3·2H2O; pyrophyllite, 4SiO2·Al2O3·H2O; talc, 4SiO2·3MgO·H2O and chamosite, 3SiO2·Al2O3·5FeO·4H2O. The SiO2 ratio in a formula is the key factor determining clay mineral types [Citation1].
A sneak peek into the open-ended research on clay minerals
Clay minerals are used as excipients in pharmaceutical preparations to enhance their organoleptic characteristics, such as flavor (flavor correctors) and color (pigments), improve their physicochemical properties, such as viscosity of the active ingredients (emulsifying, thickening and anticaking agents), facilitate their elaboration (lubricants, diluents, binders, isotonic agents) or conservation (desiccants, opacifiers) and facilitate liberation of the active ingredient within the organism (disintegrants, carrier releasers) [Citation3].
Clay minerals with very fine, thin particles and high adsorbent properties are quite useful for the antibiotics sorption. Kim et al. studied the sorption of oxytetracycline on clay minerals especially in acidic soils with high organic matter content [Citation10]. The adsorption of four widely used drugs, carbamazepine, diclofenac, ibuprofen and ketoprofen, was investigated onto a porous silica under varied ionic strengths, and with different anions, divalent cations (Ca2+ and Mg2+), trivalent cations (Al3+ and Fe3+) and natural organic matter. The studies demonstrated that at a given pH the adsorption was most affected by ionic strength, trivalent cations and properties of pharmaceuticals. The increase of ionic strength resulted in an increase in the adsorption of ketoprofen, but a decrease in the adsorption of carbamazepine [Citation11]. Cation exchange was the major mechanism of ciprofloxacin desorption from clay mineral surface. Ciprofloxacin desorption from kaolinite and montmorillonite was investigated under different pHs, different concentrations of metal cations of various valencies (Na+, Ca2+ and Al3+) and a cationic surfactant hexadecyl trimethylammonium (HDTMA), with different desorption cycles [Citation12]. Enhanced desorption hysteresis of carbamazepine was observed for the smectites with negatively charged sites compensated with inorganic cations such as K+, Ca2+ and NH4+ than the desorption from organic cation-modified smectites (e.g., HDTMA clay), suggesting that the intercalated carbamazepine molecules are more resistant to release than carbamazepine partitioning in alkyl organic phase [Citation2]. In addition, the large cation exchange capacity and surface area make the clay a good candidate to remove cationic pharmaceuticals from the effluent of waste water treatment facilities [Citation13]. The protein adsorption capacity and selectivity of kaolinite and metakaolinite show a clear dependence on the chemical nature of the adsorbents surface and on the textural properties. Kaolinite and metakaolinite exhibit a very high affinity and good retention capacity for proteins like bovine serum albumin specially A-LA and B-LG [Citation14]. The clay/poly(N-isopropylacrylamide) (PNIPAm) nanocomposite hydrogels, using lithium magnesium silicate hydrate as a clay mineral physical cross-linker were prepared to remove crystal violet from aqueous solution [Citation15]. Similarly, Ballav and associates studied the absorption behavior of polypyrrole-coated halloysite nanotube nanocomposite [Citation16]. Recently, clays have been modified through several approaches like conventional ion exchange reactions, sol-gel linking, atom transfer radical polymerization and polymer intercalation. The organic interaction incorporates different noncovalent bonding forces, such as amido acid five-membered ring chelation, carboxylic acid chelation, intermolecular hydrogen bonding and double-layer hydrophobic alignment in a layered clay confinement. Furthermore, the layered structure could be totally exfoliated and structurally randomized into individual silicate platelets using different mechanisms, such as the phase inversion of amphiphilic copolymer emulsifiers and phase transitions that involve zigzag Mannich polyamines. Different intercalation and exfoliation strategies help in developing detailed understanding of clay chemistry, thus exploring wider horizons of clay applications [Citation17].
Clay minerals sorption activity is the most suitable application in veterinary science. Kaolins and smectites are most commonly used in animal nutrition as growth promoters and supplements for the treatment of gastrointestinal disturbances, particularly diarrhea [Citation18].
The antibacterial activity of silver and cationic surfactant modified smectites from North Patagonia, Argentina, were tested in growth inhibition of Escherichia coli bacteria by the test of susceptibility on solid medium [Citation19]. Minerals also enjoy diagnostic, odontological and traumatological applications, and are used in spas and aesthetic centers for therapeutic proposes [Citation20]. Research focused on the role of clay minerals in kerogen formation, kerogen conversion to petroleum, oil migration and entrapment in reservoirs identify significant interactions arising from the adsorptive and catalytic properties of clay minerals and structural changes during diagenetic transformations [Citation21]. Recently, Das et al., 2014 explored the significant antibacterial activity of copper nanoparticle-decorated organically with modified montmorillonite/epoxy nanocomposites against ubiquitous Gram- negative bacteria Klebsiella pneumonia and Gram-positive bacteria Staphylococcus aureus [Citation22].
Kaolinite, talc, palygorskite and smectites are used for therapeutic purposes in pharmaceutical formulations as active principles or excipients. The possible use of sepiolite as active principle or excipient in pharmaceutical formulations was also investigated. Kaolinite, talc, palygorskite and smectites are used as excipients in cosmetics and pharmaceutical preparations [Citation23]. A summary of the pharmacological activities of the clay minerals is described in . They also have an admirable role in the targeted and modified drug delivery system as in .
Conclusion & future perspective
Research trends on clay minerals are heading toward the synthesis of minerals based on atomic and molecular scale design by affecting their physicochemical properties and thus they have a wide scope of applications in pharmaceutics. Chemical and physical interactions of clays with water and many other chemical species, and their dynamics, offer further scope. Biological implications related to clays are likely to be investigated more extensively. Novel materials based on nanotechnology, biochemical and medical applications, and environmental aspects are envisaged [Citation55]. Chemically modified clay mineral electrodes are also being explored for many chemical sensor applications. Tuning the process and coupling it with a separation technique can achieve effective DNA quantification. Because of the stability of clays, combining them with enzymes and suitable redox mediators – for example, clay in conjunction with hydroquinone mediator – could be a new way to quantify microbiological systems such as fungi and bacteria such as Escherichia coli [Citation56]. Novel-layered nanohybrid materials with controlled functions and microstructures are also being extensively explored. Nanocomposites based on clays and organic compounds are expanding. Studies of domain structures in layer silicates will continue, and further refinements in mixed-layer structure analysis can be expected. The study of clay mineral synthesis and alteration in nature shows signs of important reactivation. Furthermore, the self-assembled film of clay minerals has a highly regular multilayered nanostructure over a large area and could appreciably entrap in between the volume of air [Citation57]. The combination of regular structure and substantial air volume contributes to the low thermal conductivity and flame blocking property of the film.
Clay-organic studies are developing in many directions. An understanding of the surface chemistry, particle shape and relative size distribution are crucial in developing such materials for an increasingly demanding and diverse world [Citation58]. Organo-clays receive great interest for applications based on their capacity for selective adsorption of molecules. Thus, they have been used for application in chromatography separations, to remove organic pollutants from air and water, and to develop improved formulation for pesticides, as chemical sensor and molecular sieves, and so on. Among other properties applications based on special structural, gas barrier, antiflammability or others can be mentioned. Interesting photochemical behavior may also arise from the specific structure of those nanocomposites. Depending on the layer structure and specific properties, such as high-specific surface area, ion exchange capacity or hydration property, clay minerals are widely used in pharmaceuticals, and as adsorbents, catalysts or catalyst supports, ion exchangers and decolorizing agents.
Table 1. Chemical composition of clay minerals.
Table 2. Pharmaceutical activity of clay minerals.
Table 3. Applications of clay minerals in drug delivery.
Unique features of clay minerals in pharmaceutical industry
The enormous surface area, surface chemistry and surface charge impart significant and unique physical properties to the clay minerals, owing to which these possess tremendous scope to be utilized as therapeutic, cosmetics, functional, inert and bulk agents.
Most commonly employed clay minerals in pharmaceuticals and cosmetics are kaolinite, talc, montmorillonite, saponite, hectorite, palygorskite and sepiolite.
Precisely clay minerals serve as lubricants, desiccants, disintegrants, diluents, binders, pigments and opacifiers. The other imperative one are emulsifying, thickening, isotonic and anticaking agents.
The predominant curative properties include antacids, gastrointestinal protectors, antidiarrheaics, laxatives, homeostatics, emetics, antianemics and so on.
Financial & competing interests disclosure
The authors have no relevant affiliations or financial involvement with any organization or entity with a financial interest in or financial conflict with the subject matter or materials discussed in the manuscript. This includes employment, consultancies, honoraria, stock ownership or options, expert testimony, grants or patents received or pending, or royalties.
No writing assistance was utilized in the production of this manuscript.
References
- Grim RE . Clay minerals. Encylopedia Britannica. http://www.britannica.com/EBchecked/topic/120723/clay-mineral.
- Zhang W , DingY, BoydSA, TeppenBJ, LiH. Sorption and desorption of carbamazepine from water by smectite clays. Chemosphere81, 954–960 (2010).
- Carretero MI , PozoM. Clay and non-clay minerals in the pharmaceutical industry: Part I. Excipients and medical applications. Appl. Clay Sci.46, 73–80 (2009).
- Guggenheim S , AdamsJM, BainDCet al. Summary of recommendations of nomenclature committees relevant to clay mineralogy: report of the Association Internationale pour l'Etude des Argiles (AIPEA) Nomenclature Committee for 2006. Clay Minerals41, 863–877 (2006).
- Murray HH . Applied Clay Mineralogy – Occurrences, Processing and Application of Kaolins, Bentonites, Palygorskite-Sepiolite, and Common Clays. 141–145 (2006). In: Developments In Clay Science. (Volume 2). BergayaF, ThengBKG, LagalyG ( Eds), Elsevier, The Netherlands.
- Sposito G , SkipperNT, SuttonR, ParkSH, SoperAK, GreathouseJA. Surface geochemistry of the clay minerals. Proc. Natl Acad. Sci. USA96, 3358–3364 (1999).
- Liu H , ShenT, LiT, YuanP, ShiG, BaoX. Green synthesis of zeolites from a natural aluminosilicate mineral rectorite: effects of thermal treatment temperature. Appl. Clay Sci.90, 53–60 (2014).
- Viseras C , Lopez-GalindoA. Pharmaceutical applications of some spanish clays _sepiolite, palygorskite, bentonite/: some preformulation studies. Appl. Clay Sci.14, 69–82 (1999).
- Konta J . Clay and man: clay raw materials in the service of man. Appl. Clay Sci.10, 275–335 (1995).
- Kim YK , LimSJ, HanMH, ChoJY. Sorption characteristics of oxytetracycline, amoxicillin, and sulfathiazole in two different soil types. Geoderma185, 97–101 (2012).
- Bui TX , ChoiH. Influence of ionic strength, anions, cations, and natural organic matter on the adsorption of pharmaceuticals to silica. Chemosphere80, 681–686 (2010).
- Wu LM , ZhouCH, KeelingJ, TongDS, YuWH. Towards an understanding of the role of clay minerals in crude oil formation, migration and accumulation. Earth Sci. Rev.115, 373–386 (2012).
- Zhaohui L , ChangPH, JiangWT, JeanJS, HongH, LiaoL. Removal of diphenhydramine from water by swelling clay minerals. J. Colloid Interface Sci.360, 227–232 (2011).
- Duarte-Silva R , Villa-GarcíaMA, RenduelesM, DíazM. Structural, textural and protein adsorption properties of kaolinite and surface modified kaolinite adsorbents. Appl. Clay Sci.90, 73–80 (2014).
- Zhang Q , ZhangT, HeT, ChenL. Removal of crystal voilet by clay/PNIP Am nanocomposite hydrogels with various clay contents. Appl. Clay Sci.90, 1–5 (2014).
- Ballav N , ChoiHJ, MishraSB, MaityA. Polypyrrole-coated halloysite nanotube clay nanocomposite: synthesis, characterization and Cr (VI) adsorption behaviour. Appl. Clay Sci.102, 60–70 (2014).
- Wang YC , HuangTK, TungSH, WuTM, LinJJ. Self-assembled clay films with a platelet–void multilayered nanostructure and flame-blocking properties. Sci. Rep.3, 2621 (2013).
- Slamova R , TrckovaM, VondruskovaH, ZralyZ, PavlikI. Clay minerals in animal nutrition. Appl. Clay Sci.51, 395–398 (2011).
- Parolo ME , FernándezLG, ZajonkovskyI, SánchezMP, BaschiniM. Science against microbial pathogens: communicating current research and technological advances. In: Antibacterial Activity of Materials Synthesized from Clay Minerals. VilasAM ( Ed.). FORMATEX, 144–151 (2011). http://www.formatex.info/microbiology3/book/144–151.pdf.
- Viseras C , AguzziC, CerezoP, Lopez-GalindoA. Uses fo clay minerals in semisolid health care and therapeutic products. Appl. Clay Sci.36, 37–50 (2007).
- Wu Q , LiZ, HongH, WTR. Desorption of ciprofloxacin from clay mineral surfaces. Water Res.47, 259–268 (2013).
- Das G , KalitaRD, GogoiP, BuragohainAK, KarakN. Antibacterial activities of copper nanoparticle-decorated organically modified montmorillonite/epoxy nanocomposites. Appl. Clay Sci.90, 18–26 (2014).
- Bergaya F , LagalyG ( Eds). General Introduction: Clays, Clay Minerals, and Clay Science. In: Handbook of Clay Science (Volume 1). ThengBKG. Elsevier, Amsterdam, The Netherlands, 1–19 (2006).
- Robert M F , ElsassF, HardyM. Crystallochemistry, Properties and Organization of Soil Clays Derived from Major Sedimentary Rocks in France. Clay Minerals26, 409–420 (1991).
- Carretero MI , GomesCSF, TateoF. Clays and Human Health. In: Handbook of Clay Science (Volume 1).BergayaF, ThengBKG, LagalyG ( Eds). Elsevier, 717 (2006).
- Murray HH . Traditional and new applications for kaolin, smectite, and palygorskite: a general overview. Appl. Clay Sci.17, 207–221 (2000).
- Arab S , AlshikhA. The use of Clay Minerals of the Dead Sea as Drugs. In: Clay Minerals (Volume 5). MurrayHH ( Ed), NY, USA5, 112–115 (2012).
- Silva PSC , OliveiraSMB, FariasL, FávaroDIT, MazzilliBP. Chemical and radiological characterization of clay minerals used in pharmaceutics and cosmetics. Appl. Clay Sci.52, 145–149 (2011).
- Carretero MI , PozoM. Clay and non-clay minerals in the pharmaceutical and cosmetic industries Part II. Active ingredients. Appl. Clay Sci.47, 171–181 (2010).
- Williams LB , HaydelSE. Evaluation of the medicinal use of clay minerals as antibacterial agents. Int. Geol. Rev.52, 745–770 (2010).
- Maheshwari RK , SharmaSN, JainNK. Adsorption studies of phenformin hydrochloride on some clays and its application in formulating sustained release dosage forms. Ind. J. Pharm. Sci.50, 101–105 (1988).
- Forni F , IannuccelliV, CameroniR, CoppiG, BernabeiMT. Swellable heterogeneous matrixes: physical properties and release mechanisms. Farmaco Prat.41, 155–164 (1986).
- Forni F , IannuccelliV, CoppiG, BernabeiMT. Effect of montmorillonite on drug release from polymeric matrices. Arch. Pharm.322, 789–793 (1989).
- Aguzzi C , ViserasC, CerezoP, RossiS, FerrariF, CaramellaC. Control of tetracyclines delivery by their interaction with veegum. In: Proceedingsa of the 30th Annual Meeting & Exposition of the Controlled Release Society. 19–23 July (Glasgow, Scotland, UK (2003). Abstract 730).
- Aguzzi C , ViserasC, CruzJ, ZafraM, CerezoP, RossiS. Development of new controlled release systems based on mineral supports: III. Release studies of tetracyclines–silicate interaction products. Proceedings Of The 1st Eufeps Conference On Optimising Drug Delivery And Formulation: New Challenges In Drug Delivery. Acta del Congreso, Versailles, 136–137 (2003).
- Cerezo P . Mecanismos Y Cineticas De Adsorcion-Liberacion De Farmacos En Soportearcilloso. Contribución Al Estudio De Complejostimololmaleato Con Esmectitas, Paligorskitas Y Sepiolitas. University of Granada, Granada (E), [PhD thesis] (2003).
- Ambrogi V , FardellaG, GrandoliniG, PerioliL. Intercalation compounds of hydrotalcite-like anionic clays with antinflammatory agents. I. Intercalation and in release of ibuprofen. Int. J. Pharm.220, 23–32 (2001).
- Ambrogi V , FardellaG, GrandoliniG, PerioliL, TiraltiMC. Intercalation compounds of hydrotalcite-like anionic clays with antinflammatory agents. II. Uptake of diclofenac for a controlled release formulation. AAPS PharmSci Tech.3, E26 (2002).
- Rodrigues LA A , VeigaF, de FreitasRMet al. The systems containing clays and clay minerals from modified drug release: a review. Colloids Surf. B Biointerfaces103, 642–651 (2013).
- Shrivastava R , JainSR, FrankSG. Dissolution dialysis studies of metronidazole–montmorilloniteadsorbates. J. Pharm. Sci.74, 214–216 (1985).
- Lin FH , LeeYH, JianCH. A study of purified montmorillonite intercalated with 5-fluorouracile as drug carrier. Biomaterials23, 1981–1987 (2002).
- Aguzzi C , FerrariF, GattiA, RossiS, BonferoniMC, CerezoP. Novel controlled release formulations based on tetracycline–clay interaction products for periodontal administration. Proceedings of the 45th Simposio AFI. Rimini Italy (2005). 177.
- Aguzzi C , FerrariF, CerezoP, RossiS, BonferoniMC, DacarroC. Suitability assessment of tetracycline-loaded clays in the treatment of periodontitis. Proceedings of the 1stPharmSciFair, Pharmaceutical Sciences Fair and Exhibition. NICE, France PO-258 (2005).
- Wai KN , BankerGS. Some physicochemical properties of the montmorillonites. J. Pharm. Sci.55, 1215–1220 (1966).
- Fielden KE . US5556639 (1996).
- Viseras MT , AguzziC, CerezoP, ViserasC, Lopez-GalindoA, ValenzuelaC. Study of adsorption kinetics of 5-aminosalicilyc acid in phyllosilicates for controlled drug delivery in the treatment of inflammatory bowel disease. Pharmaceutical Sciences Fair and Exhibition.Nice, France, PO-253 (2005).
- Zhu Y , AdjeiAL. US2002110527 (2002).
- Price RR , GaberBP, LvovY. In vitro release characteristics of tetracycline HCl, khellin and nicotinamide adenine dinucleotide from halloysite; a cylindrical mineral. J. Microencapsul.18, 713–722 (2001).
- Byrne R S , DeasyPB. Use of porous aluminosilicate pellets for drug delivery. J. Microencapsulation.22, 423–437 (2005).
- Levis SR , DeasyPB. Use of coated microtubular halloysite for the sustained release of hydrochloride and propranolol hydrochloride. Int. J. Pharm.253, 145–157 (2003).
- Lopez-Galindo A , ViserasC, CerezoP. Compositional, technical and safety specifications of clays to be used as pharmaceutical and cosmetic products. Appl. Clay Sci.36, 51–63 (2007).
- Arco MD , FernándezA, MartínC, RivesV. Release studies of different NSAIDs encapsulated in Mg,Al,Fe-hydrotalcites. App. Clay Sci.42, 538–544 (2009).
- Choy JH , ChoiSJ, OhJM, ParkT. Clay minerals and layered double hydroxides for novel biological applications. Appl. Clay Sci.36, 122–132 (2007).
- Sironmani TA . Comparison of nanocarriers for gene delivery and nanosensing using montmorillonite, silver nanoparticles and multiwalled carbon nanotubes. Appl. Clay Sci.103, 55–61 (2015).
- Liu P . Polymer modified clay minerals: A review. Appl. Clay Sci.38, 64–76 (2007).
- Guggenheim S , AdamsJM, BainDCet al. Summary of recommendations of nomenclature committees relevant to clay mineralogy: report of the Association Internationale pour l'Etude des Argiles (AIPEA) Nomenclature Committee for 2006. Clay Minerals41, 863–877 (2006).
- Chiu C , HuangT, WangY, AlamaniB, LinJJ. Intercalation strategies in clay/polymer hybrids. Progr. Polymer Sci.39(3), 443–485 (2014).
- Sposito G , SkipperNT, SuttonR, ParkSH, SoperAK, GreathouseJA. Surface geochemistry of the clay minerals. Proc. Natl Acad. Sci. USA96, 3358–3364 (1999).