Abstract
The intricate pathophysiology of brain disorders, difficult access to the brain, and the complexity and high risks and costs of drug development represent major hurdles for improving therapies. Nose-to-brain drug transport offers an attractive alternative or addition to formulation-only strategies attempting to enhance drug penetration into the CNS. Although still a matter of controversy, many studies in animals claim direct nose-to-brain transport along the olfactory and trigeminal nerves, circumventing the traditional barriers to CNS entry. Some clinical trials in man also suggest nose-to-brain drug delivery, although definitive proof in man is lacking. This review focuses on new nasal delivery technologies designed to overcome inherent anatomical and physiological challenges and facilitate more efficient and targeted drug delivery for CNS disorders.
The unmet need
Disorders of the central nervous system (CNS) represent a great emotional, financial and social burden to patients, their families and society. Despite intense research efforts, great unmet needs for better treatment modalities remain for the many 'diseases of the mind' and CNS delivery of therapeutics may offer benefits for other disorders as well [Citation1]. The intricate pathophysiology of brain disorders, the difficulty in accessing the brain with both small and large molecule medications, and the risk, complexity and enormous costs of the clinical trials required for regulatory approval are significant hurdles in the research and development of new treatments for brain disorders [Citation2]. These difficulties have, in recent years, apparently resulted in a declining effort in the pharmaceutical industry, with reduced investment being reported for the development of drugs for many CNS disorders. Although some high-risk/high-reward programs, such as Alzheimer's disease-modifying therapies, may have appeared to be an exception to the trend, recent results continue to disappoint [Citation1,Citation2], and legitimate concerns remain about a declining effort in this area of unsurpassed need.
In normal circumstances, the blood–brain barrier (BBB) plays a vital role in protecting the delicate milieu of the brain; however, when the introduction of exogenous treatment into the CNS is desired, the BBB prevents 98% of small-molecules and an even greater percentage of large molecules from reaching their intended targets. This lack of access to the brain is a major bottleneck for CNS drug development [Citation3]. Researchers with promising concepts have been forced to discard innumerable drugs with high therapeutic potential due to their inability to deliver the medication across the BBB in therapeutic concentrations and, thus, their inability to reach the target sites.
New strategies for crossing the BBB
Some new strategies for crossing the BBB involve synthesis of small molecules to exploit existing carrier-mediated transport (CMT) or re-engineering large molecules with molecular 'Trojan horse' delivery systems. These approaches may allow transport via receptor-mediated transfer (RMT) systems in the BBB [Citation3,Citation4]. An alternative or supplementary approach is targeted intranasal delivery, an approach potentially applicable to a broader array of molecules, where the goal is to circumvent, rather than cross, the BBB.
The nose is the only place where the brain 'meets the outside world'. Nerve filaments, or axons, of the 12th cranial nerve (the olfactory nerve) extend directly from the olfactory bulb in the limbic region of the brain to the upper posterior segments of the nose, penetrate the mucosal lining and allow direct contact with the environment without a peripheral sensory receptor relay. Millions of these neurons are present, acting as a chemical sensor, detecting scents from food and playing a role in social behaviors. In the past, these unusual neurons were also believed to be the only group of neurons capable of regeneration. This unique and important anatomic arrangement also offers a potential route for direct medication access into the CNS [Citation5–7]. Cranial nerves are important to this discussion because animal studies, and a few human studies, published in the last decade suggest the potential existence of a functional pathway for medications to pass into the CNS from structures deep in the nose innervated by cranial nerves, a pathway sometimes called 'nose to brain' (N2B) transport () [Citation5,Citation6,Citation8].
Ethical and practical limitations related to the design and methodologies of human clinical trials to study N2B transport have slowed development in the field, and unequivocal evidence of direct N2B transport in man may still be considered lacking. Furthermore, the fact that the science of N2B lies at the crossroad between several disciplines of medicine, pharmacology and engineering has contributed to several important long-lived misconceptions that may also have hampered progress in this field.
Barriers to accessing the brain
The endothelium of intracerebral blood vessels is optimized to maintain the delicate brain homeostasis by allowing entrance of essential nutrients while restricting exposure to harmful substances [Citation9]. A combination of low permeability and a specialized system of cellular transport mechanisms together constitute this essential barrier between the blood and the brain. However, this vital barrier also prevents efficient delivery of therapeutic drugs into the CNS. Transport from the blood to the CNS is only effective for 2% of small molecules [Citation3]. While some small lipid-soluble molecules like ethanol and caffeine penetrate into the brain easily, most water-soluble molecules are unable to traverse the barrier without specialized carrier-mediated transport systems. Moreover, many new therapeutic molecules in development are macromolecules, which in general show minimal entry into the CNS.
A fact that is often ignored or underappreciated is that this protective barrier between the blood and the CNS actually consists of two conceptual barriers with different properties: the blood–cerebrospinal fluid barrier (BCSFB) and the blood-–brain barrier (BBB). Both of these conceptual barriers interpose passive and active transport barriers to the exchange of molecules [Citation3]. The BBB separates the blood flowing through the circulatory system from the brain extracellular fluid and neurons (). The BBB is structural, comprised of capillary endothelium lined with tight junctions (unlike most of the systemic circulation), and functional, with a variety of active transport mechanisms. The BCSFB is different in that it can be thought of as two components in series. The first component of the BCSFB separates the blood from the CSF and is formed by the choroid plexus, a complex neuroendocrine structure where the CSF is produced and which provides passive transport, active transport and perhaps even hormonal activity for the CSF (). The capillaries of the choroid plexus are lined with fenestrated endothelium rather than the tight junctions lining the capillaries forming the BBB, and it is the epithelium of the choroid plexus that appears to provide a functional barrier. The second component of the BCSFB separates the CSF from the neurons and brain extracellular fluid and is formed by the arachnoid membrane, the cells of which are lined by tight junctions. The BCSFB is functionally distinct from the BBB is several ways, and has been considered more 'leaky' than the BBB [Citation3,Citation10].
The BBB
The surface area of the BBB is very large due to the large endothelial surface area of the >100 billion brain capillaries forming the BBB. This is very different from the human choroid plexus (∼20 cm2), which comprises the first component of the BCSFB, and which is very small in comparison () [Citation3,Citation11]. Due to the dense microvasculature in the brain, every neuron is perfused by and in direct contact with a capillary. Thus, for the limited number of substances that cross the endothelial barrier of the brain capillaries, equilibration throughout the entire brain extravascular volume occurs almost immediately [Citation11,Citation12]. The main challenge for efficient drug delivery is to cross, or to bypass, the pervasive tight barrier that is otherwise vital to the homeostasis and normal functioning of the brain. This challenge is further complicated because a number of known or candidate CNS drugs are substrates for BBB efflux transporters, which further reduces the ability to reach therapeutic concentrations at the site of action without reaching systemic concentrations associated with unacceptable adverse events [Citation9].
The blood–cerebrospinal fluid barrier & the limitations of CSF drug levels
Due to the relative 'leakiness' of the first component of the blood–cerebrospinal fluid barrier (BCSFB), virtually all molecules – small and large – in the blood distribute into CSF to some extent at a rate inversely related to the molecular weight [Citation11]. In man, a high volume of CSF, approximately 500 ml, is produced daily in the four ventricles. From the ventricles, CSF moves by bulk flow into the cranial and spinal sub-arachnoid spaces (total volume ∼140 ml), after which it is returned by the arachnoid villi into the venous sinuses, and then undergoes relatively rapid turnover. Along with the second component of the BCSFB, the bulk flow through the CSF macrocirculation and the relatively rapid CSF turnover may contribute to what has been described as possibly 'quantitatively unimportant' penetration of drug from the CSF into the brain [Citation11,Citation13].
It is believed that substances in the CSF of the subarachnoid spaces may enter the brain via the perivascular spaces (Virchow–Robin spaces) surrounding the penetrating arteries at the pial surface and along the basement membrane capillaries; importantly, however, the second component of the BCSFB would still come into play [Citation11,Citation14]. Specifically, although both small and large molecules are transported along these perivascular channels, the extent and rate of distribution across the ensheathing astrocytic end-feet (second component of the BCSFB) into the surrounding interstitial fluid bathing neurons appears highly dependent on the molecular weight of the substance ( [Citation14]. This route is conceivably particularly relevant for nasally delivered drugs that may enter directly into the subarachnoid CSF surrounding the olfactory bulb (). Similar channels were identified surrounding veins acting as drainage pathways for substances from the brain interstitium [Citation14].
In light of these various considerations, the value of simple CSF concentrations (as might be obtained by lumbar puncture) for predicting the effect, or even presence, of centrally acting drugs at a central site of action appears to be quite limited [Citation11,Citation13]. Irrespective of the reasons, the critical observation that penetration from CSF to brain is limited suggests the need for great caution in the interpretation of data on N2B delivery, and on other efforts to bypass or penetrate the BBB, based on the presence (or absence or concentration) of drug in the CSF: measurement of drug directly by intracerebral microdialysis, or perhaps drug activity (e.g., using non-invasive methods based on PET or MRI) in the brain itself may be necessary [Citation13].
Assessing brain penetration by measurements in CSF versus brain tissue
The issues of BBB and BCSFB discussed above influence interpretation of research into the nature and magnitude of N2B transport. For example, the fraction of the delivered dose reaching the brain through N2B transport has been reported to be <1%, and even as low as 0.1% or less, suggesting that very potent drugs would be required to achieve the desired effects [Citation12]. However, quantification of the fraction of drug entering into the brain is difficult, especially in humans. CSF sampling is an available method, but may be inappropriate and subject to controversy over the value of results, and even if considered appropriate is likely to be impractical. There are several problems in relying solely on CSF to define CNS exposure. According to Pardridge, it is a widespread misconception that drug levels in the CSF are a reflection of, and a valid marker of, transport across the BBB [Citation11]. Among other implications, this information challenges the validity of the conclusions of a comprehensive review from 2007 of 104 papers evaluating N2B transport in animals and humans, which is discussed further below [Citation8]. Although controversy continues to exist, some more recent studies and reviews conclude that both small and large molecules can pass rapidly from the nose into the brain along olfactory nerves, and into the CNS along branches of the first and second trigeminal nerve structures, without primarily passing via the CSF, further challenging the measurement of CSF levels as an appropriate research approach to assessing N2B transport of therapeutic molecules [Citation5,Citation6].
Strategies to cross or bypass the BBB
A number of complex strategies involving re-engineering of drug molecules to exploit endogenous mechanisms for transport across the BBB are being explored [Citation15,Citation16]. Small-molecule drugs can be chemically modified to attempt to take advantage of the carrier-mediated transport (CMT) system in the capillary endothelium. These are lipid-mediated carrier mechanisms that allow free diffusion across the BBB of certain molecules <400 Da and with <8 hydrogen bonds [Citation17,Citation18]. Large-molecule drugs including peptides and proteins can potentially be delivered across the BBB with 'Trojan horse' molecules (antibodies, recombinant proteins, non-viral gene medicines or RNA-interference drugs) targeting receptor-mediated transport systems in capillaries [Citation3]. Carrier-mediated transport mechanisms, molecular Trojan horse delivery to access receptor-mediated transfer systems, and nanotechnology-based approaches have shown promising early results in animal studies and some are in clinical testing in man [Citation19,Citation20]. These approaches generally involve modifications of the carrier molecule that entail a significant additive layer of complexity and risk, both clinical and otherwise, in the development of CNS therapeutics [Citation17,Citation18,Citation21,Citation22].
The nose: an alternative route of brain delivery
Even if efforts to exploit active transport mechanisms from the blood into the CNS enable BBB penetration of some drugs, additional challenges remain. Many drugs, and in particular macromolecules, are degraded in the gastrointestinal (GI) tract and/or metabolized in the liver, severely limiting the fraction of drug reaching the blood in the first place. Increasing the oral dose to compensate may cause unacceptable GI or systemic adverse events. Injection is an obvious alternative route to get drugs into the blood, but remains an impractical and unattractive delivery route from many perspectives, particularly for treatment requiring frequent dosing or home administration. This limitation is even more pronounced for intrathecal drug administration. Nasal delivery has been proven to offer a route into the systemic circulation for a number of systemically acting drugs with low oral bioavailability (BA) and/or where fast absorption and onset of action is desirable [Citation23]. In this respect, nasal delivery could serve as an attractive non-invasive delivery method for CNS drugs that do pass the BBB and for drugs formulated to exploit active transport mechanisms to cross from the blood into the brain, such as the CMT and RMT transport systems as mentioned above [Citation3].
The nose does more: circumventing the BBB through direct nose to brain (N2B) transport
Although nasal delivery to enhance access to the systemic circulation is potentially important for some applications, it is the potential for circumventing the systemic circulation and delivering drugs directly into the brain that represents a particularly novel, attractive and little understood application of nasal delivery [Citation21,Citation24]. Direct transport of drugs along the olfactory and trigeminal nerves is increasingly considered an important and promising route whereby small and large molecules, perhaps even stem cells, delivered to the nose can access the brain in therapeutic concentrations [Citation5,Citation6,Citation20,Citation21,Citation25–27].
Data addressing N2B transport and/or N2B pharmacodynamic activity in animals & man
More than 100 animal studies published over the last decade claim proof of transport from the nose directly to the brain tissue and/or to the CSF of both small molecular weight drugs and large macromolecules/peptides [Citation6,Citation8,Citation28]. Some of these animal studies include an appropriate intravenous (IV) comparator, enabling documentation of preferential direct N2B transport (rather than transport to the CNS via the blood) [Citation29–31]. Other studies with subcutaneous (SC) or intraperitoneal comparators suggest preferential N2B transport, some with accompanying enhancement of pharmacodynamic effects [Citation32–37]. Nasal delivery of neuroprotective agents can also reduce brain injury after acute conditions such as stroke and subarachnoid hemorrhage in animal models [Citation5,Citation21,Citation38,Citation39]. Some studies convincingly suggest the dynamics of rapid transport of intact and biologically active peptides along the olfactory and trigeminal nerves to central regions of the brain and the trigeminal nuclei in the brainstem by using radiolabeled peptides that characteristically have poor BBB penetration [Citation40,Citation41]. Accompanying activation of signaling pathways in several brain areas expressing high levels of radioactivity also suggest delivery of intact and biologically active peptides to target sites in the CNS [Citation32,Citation38]. Transport of intact and active peptides is further supported by pharmacodynamic functional parameters such as reduction in stroke volume following delivery of IGF-I and improved neurological function and behavioral scores after IGF-I and Orexin-A in rats and monkeys [Citation36,Citation37,Citation42,Citation43]. Interestingly, dynamic gamma images from research directly comparing brain access from nasal versus IV delivery suggest a pattern of efficient transport into certain deep brain regions and the interstitial fluid of the brain following nasal delivery, in contrast with images following IV delivery where virtually no signal is observed in the brain tissue [Citation41]. Unlike the observations following intranasal delivery, when images are obtained after IV delivery it is only when the resolution of gamma imaging is increased by a factor of 100 that some radioactivity becomes evident in CNS images; however, the pattern of these images suggests that the minimal observed activity is located in the CSF [Citation41]. Taken together, these studies strongly suggest transport from the nose to deeper structures and into interstitial fluid of the brain without passing via the CSF. They also suggest that the transport efficiency and distribution pattern is distinctly different from that observed after IV delivery [Citation41].
Preferential N2B transport is also supported by an important crossover placebo-controlled study in Rhesus monkeys given Orexin-A intranasally (IN) and IV [Citation43]. The naturally occurring peptide Orexin-A counteracts the effects of sleep deprivation. The monkeys were taught a number of tasks they could perform after having slept normally, but not when sleep-deprived. Investigators evaluated a low IN Orexin-A dose, only 10% of the comparison IV dose. After receiving the Orexin-A IN the sleep-deprived monkeys were able to perform the task as if they had slept normally, whereas the monkeys on placebo performed poorly. Monkeys receiving the tenfold higher IV dose showed some improvement compared with placebo, but still far worse than the level reached after IN delivery. Furthermore, fMRI performed on the monkeys during and after drug delivery showed brain activation corresponding well with the differences in task performances [Citation43].
In man, several clinical trials with peptides such as insulin and oxytocin, as well as small molecules, suggest functional activity consistent with N2B transport based on clinical response alone and/or functional MR imaging, but convincing unequivocal proof of N2B transport via demonstration of mass transport is still missing in man, largely due to methodological and ethical limitations [Citation28,Citation44–46]. Furthermore, the few human studies providing measurements of drug concentration both in blood and CSF are conflicting and the therapeutic potential of N2B in man has been subject to controversy [Citation8,Citation47].
For example, the validity of the methodology applied in the many animal studies as well as the few human studies prior to 2007 was called into question in a review by Merkus and van den Berg [Citation8]. The authors reviewed 104 papers published prior to 2007 (98 animal studies and six human studies) on N2CSF or N2B transport. A set of criteria were applied by the authors, including what they assert to be 'realistic' dosing volumes (rats: 10–25µl per nostril; man: ≤100µl per nostril), documented safety of excipients/enhancers, use of both IN and IV drug delivery targeting comparable plasma concentrations, and PK measurements in plasma and CNS (CSF and/or brain) [Citation8]. Only 12 of the 104 studies were found by these authors to pass their personal criteria related to experimental design and methodology. Nine of these 12 were studies reported N2CSF transport whereas the remaining three studies found evidence of direct N2B transport. Of these 12 studies, only two studies in rats were categorized by Merkus and van den Berg as ‘indicative for direct nose to CNS transport’ and none of the articles were considered to have shown convincing proof. The only study that met all the criteria and was categorized by these authors as 'indicative' of N2B transport reported enhanced brain uptake of morphine after IN delivery compared with IV delivery [Citation29].
Among the studies in this 2007 review, one by Born and coworkers from 2002 titled 'sniffing neuropeptides' is frequently cited as 'proof' of nose-to-brain in man [Citation47]. This study showed significantly higher levels of insulin, vasopressin and melanocortin in the CSF after nasal delivery when compared with placebo and without significant increase in the serum levels. This study was critiqued by Merkus et al. due to the delivery of a larger volume then they defined as 'realistic' (2 × 100µl) to each nostril and due to the lack of IV comparator [Citation8,Citation47]. While lack of IV comparator in this study is a valid limitation, the delivery volume of 2 × 100 µl to each nostril is by no means universally viewed as an unacceptable dosing volume in man, especially after the introduction of Breath Powered™ devices that broadly deposit the dose in the upper posterior nasal cavity beyond the nasal valve [Citation24,Citation48,Citation49]. The only human study among the 12 that passed the screening criteria of Merkus and van den Berg was a study where the authors themselves were involved [Citation50,Citation51]. This study did not show any sign of N2CSF transport following nasal delivery of melatonin and hydroxocobalamin (vitamin B12) when compared with an IV delivery mimicking the serum profiles of IN delivery in postoperative neurosurgical patients [Citation51]. Unfortunately, no information on the patency of the patients’ nasal cavities or the actual deposition pattern in the nose was provided for this study [Citation8,Citation51]. Based on available information about the deposition pattern produced by conventional nasal spray pumps in the literature, much of the dose in this study was likely deposited in the anterior non-ciliated region of the nose (lined largely with squamous epithelium) with limited drug delivery to the olfactory region. Furthermore, as pointed out by Pardridge, lack of drug in the CSF does not exclude the presence of drugs in the brain [Citation11]. Thus, while the 2007 review offered an interesting perspective, the controversy over N2B in man remained unresolved even then. Animal studies including apparently adequate IV PK comparator profiles supported direct N2B transport, and, in our opinion, other parenteral delivery routes (SC, intramuscular or intraperitoneal), and even oral delivery, may also serve as comparators as long as the PK profiles are adequately measured and matched [Citation29,Citation30,Citation33,Citation35].
Nasal delivery of chemotherapeutic agents to combat brain tumors
Studies in animals and humans suggest a future for N2B delivery in the drug treatment of brain tumors like glioblastoma [Citation27]. Nasal delivery of substances such as peptoids (peptide-like substances with enhanced properties), telomerase inhibitors, methotrexate, raltitrexed and 5-fluorouracil have been shown to reduce brain tumors and increase survival in animal models [Citation27]. Interestingly, the oligonucleotide telomerase inhibitor GRN163 appeared to kill tumor cells selectively without toxic effects in normal brain tissue [Citation52]. Tumor-tropism has also been observed after nasal delivery of oncolytic viruses selectively infecting and killing olfactory bulb gliomas [Citation53]. Another recent study employing neural stem cell-based virotherapy to treat induced glioblastoma in mice showed synergistic therapeutic efficacy when applied with radiation [Citation54]. Furthermore, it was reported that nasal delivery of methotrexate, an antitumor agent that passes through the BBB very poorly, significantly reduced tumor weight compared with intraperitonal delivery, despite significantly lower serum levels [Citation32]. Brain tumors may cause disruption of the BBB and potentially facilitate drug transport across the BBB, but the substantially lower systemic exposure associated with intranasal delivery suggests that this route of delivery may provide a different and more efficient route of accessing the tumor. A report of IN administration of monoterpene perillyl alcohol in humans with recurrent glioblastoma, a Ras-protein inhibitor, found noticeable tumor regression and a significant increase in survival compared with historically matched controls, suggesting antitumor CNS activity of IN perillyl alcohol [Citation55,Citation56].
N2B delivery of stem cells
A recent study suggests that stem cells delivered to the nose in rats migrated to the olfactory bulb and other parts of the brain and also entered into the CSF and subsequently into the brain parenchyma [Citation42]. More recently, the same group reported nasal delivery as a highly promising noninvasive alternative to the traumatic surgical procedure of stem cell transplantation in Parkinson's disease. In one recent study, stem cells delivered nasally survived for a period of at least 6 months, improved previously depleted dopaminergic activity, showed a strong anti-inflammatory effect in the lesioned hemisphere and prominently improved the motor deficit [Citation25]. IN delivery of neural stem/progenitor cells (NSPCs) has recently been suggested as a noninvasive route to target intracerebral gliomas [Citation57]. Following IN delivery of a suspension of human NSPCs in mice with frontal gliomas induced by inoculation of human glioma cells, a rapid and targeted migration of the cells towards and into the gliomas, mainly along the olfactory pathways, was observed [Citation57,Citation58]. NSPCs may serve as a vehicle for biologically active gene products targeting tumors, as well as reversal of other pathological and inflammatory processes or deficiencies in the brain with reduced systemic exposure and minimal accumulation in peripheral organs [Citation27,Citation57,Citation59]. Currently, intracerebral delivery of genetically modified NSPCs during surgery to carry an enzyme converting the non-toxic prodrug 5-fluorocytosine to the active 5-fluorouracil is being tested in a Phase I clinical trial in humans [Citation27,Citation60]. A recent study showed improvement in survival of mice after nasal delivery of modified mesenchymal stem cells in combination with radiotherapy [Citation54]. The nose offers an alternative route for NSPCs into the gliomas and other CNS disorders allowing for repeated non-invasive dosing [Citation54,Citation57,Citation59].
Possible mechanisms for direct N2B transport
At first look, one may conceive of the N2B transport occurring along nerve fibers as a slow intra-axonal process; however, this is not the mechanism supported by emerging research. Recent studies supporting N2B transport along olfactory and trigeminal nerves () [Citation5,Citation26,Citation30,Citation40,Citation41] suggest rapid transport of substances is occurring along ensheathed channels accompanying the nerve filaments to their respective projections in the brain and/or brain stem. Importantly, the distribution of radiolabeled-drug imaged in the brain was distinctly different following IN delivery compared with IV delivery, with the highest concentrations following IN delivery occurring in the brain tissues and structures connected to the olfactory and trigeminal nerves and with minimal drug in regions corresponding to the location of CSF [Citation41]. In contrast, following IV delivery, the small amount of drug found 'inside the brain' was found in a distribution consistent with a CSF location, suggesting transport to the CSF and not directly to the brain [Citation41]. The relevant transport routes and mechanisms are well described in recent reviews and are outlined in () [Citation5,Citation44].
Based on the rapid speed of transport from the nose to CNS structures (occurring within minutes), bulk transport along the ensheathed channels surrounding the olfactory and trigeminal nerves is the most likely mechanism of N2B transport. Once inside the brain, further transport to other regions can be expected to occur by perivascular pump mechanisms driven by arterial pulsations [Citation6,Citation61]. In contrast, axonal transport is slow and cannot account for the detection of the radiolabeled substances within different regions of the brain within minutes after delivery in animals [Citation62]. The authors conclude that the observed concentrations suggest that a substance entering the brain along the olfactory and trigeminal nerves can reach the brain tissue in therapeutic levels [Citation6,Citation61,Citation62]. Furthermore, the pharmacodynamic effects consistent with central activity that were observed in animal studies with adequate IV, SC or intraperiotoneal comparators support the notion that therapeutic levels can be reached following IN delivery [Citation30,Citation33,Citation35,Citation43].
Critiques of the anatomic relevance of animal models to man
There are valid reasons to be skeptical of the potential for N2B transport in man based on evidence from animal studies. For example, several authors point out the relatively smaller surface area of the olfactory epithelium as a limitation for the potential of N2B in humans [Citation8,Citation12]. While clearly an important point to consider, simple comparisons of ratios between nasal mucosal surfaces (and olfactory epithelium surfaces) to bodyweight represent crude oversimplifications that may prove inadequate when assessing the potential for N2B delivery [Citation12]. Specifically, it has been pointed out that the ratio between the 10 cm2 olfactory region and 70 kg average body weight in man provides a ratio of 0.14, which is 100–200-times lower than the ratio for animals such as rats and dogs where the olfactory epithelium covers roughly 50% of the nasal mucosa [Citation12]. However, data from human biopsy studies suggest that the olfactory region actually extends 1–2 cm forward and downward beyond the upper 8–10 mm of the olfactory cleft. The size of the region considered to contain nerve filaments suitable for N2B transport in man may be substantially greater than the often cited 3–8% from cadaver studies [Citation63]. In addition this does not take into account the innervation of the nasal cavity by the first and second branches of the trigeminal nerve, which is even more extensive than the olfactory nerve. The majority of the human nasal mucosal surface (150 cm2) is lined by respiratory mucosa densely innervated by sensory and parasympathetic trigeminal nerves with a potential for N2B transport. The number of substances/formulations that can readily access this dense network of trigeminal nerve endings is limited by their ability to cross a single mucosal layer. Of course, the actual penetration potential of a substance will be determined not only by its molecular size and other physiochemical properties, but also by adjuvant penetration enhancers potentially added to a formulation.
The mucociliary clearance rate in humans is three- to four-times slower than in rats, potentially leaving more time for absorption to occur [Citation64]. However, many animal studies are conducted under general anesthesia and with a head down position, which may influence mucociliary function, making the translation to man less clear. However, although pulmonary mucocliliary activity may be reduced during anesthesia, studies in rabbits and man with volatile anesthetic agents administered via intubation did not show modified mucociliary clearance of nasal and sinus epithelium [Citation65–67]. There are also studies in non-anesthetized animals that do suggest N2B transport [Citation43]. Clearance in man can also be slowed by muco-adhesives and ciliostatic excipients used to increase contact time. It is also conceivable that other differences between animal and human physiology offset the potential disadvantages of human anatomy; for example, the CSF turnover rate in rats is five- to six-times higher compared with man, which may be important for drugs that enter the brain via the CSF [Citation64]. Finally, a particularly important problem with the 'nose surface to body weight' critique is that the target sites for N2B delivery are not distributed throughout the entire body, but instead are in the brain (total brain mass 1200 g), and more specifically are in selected regions of the brain. This may render comparisons to total body weights and even brain weights irrelevant. For example, transport along the olfactory nerves may offer direct and targeted delivery to structures of the limbic system, essential for, among other things, integrating emotional states and memories of physical sensations. The olfactory bulb is part of the limbic system in man, and directly contacts structures involved in the pathophysiology of many psychiatric and neurodegenerative disorders () [Citation68].
N2B pathways
There are several anatomic considerations that increase hope of adequate pathways for N2B transport in man. As noted above, human biopsy studies suggest that olfactory filaments are more broadly distributed than reported in older cadaver studies [Citation63], and although the density may be decreased relative to the historically recognized olfactory epithelium, islets of olfactory epithelium can be found throughout the middle turbinate (). Furthermore, sensory fibers of the ophthalmic branch of the trigeminal nerve innervating the anterior and upper parts of the nasal cavity, although still posterior to the nasal valve, penetrate the cribriform plate and contribute to olfaction and offer a supplementary access route to the CNS [Citation69]. In addition, the maxillary branch of the trigeminal nerve innervating the remainder of the respiratory nasal mucosa offers a potential route for drug transport to the regions of the brainstem where these nerves fiber project [Citation26,Citation27]. Transport may also occur along the parasympathetic fibers of the trigeminal nerve accompanying the sensory nerves to the sphenopalatine ganglion () [Citation70,Citation71]. The clinical observations suggesting CNS effects of macromolecules such as insulin and oxytocin delivered with conventional spray pumps may in fact be attributable primarily to this trigeminal pathway [Citation45,Citation46,Citation72]. Thus, assuming that drug transport along the ensheathed layers surrounding the nerves is not restricted to the olfactory pathway, the potential for N2B drug delivery in man may be greater than would otherwise be predicted provided the drug to be delivered can be reliably deposited in the innervated areas. However, in the absence of unequivocal proof of N2B transport in man it cannot be excluded that the observed effects after IN delivery of insulin and oxytocin may be due to small amounts passing through the human BBB and/or effects mediated via drugs absorbed into the blood and action on receptors for these peptides present in peripheral tissues. Central effects have also been observed after IV delivery of oxytocin, but a lack of PK data impedes definitive conclusions as to the relative impact of peripheral and central action [Citation73–75]. Studies directly comparing PK levels and cognitive, physiological and behavioral effects following delivery of oxytocin via different routes are needed.
Anatomical & physiological challenges in man
Despite the extensive emerging evidence for N2B activity, limited attention has been given to the crucial role of the mechanism by which drug is delivered into the nasal cavity in man. This is extremely important particularly because of the limitations of conventional nasal delivery devices in reaching beyond the nasal valve to the upper and posterior regions of the nasal cavity with the richest cranial nerve innervation, the target region that is likely to be the optimal target for N2B delivery [Citation75]. Insufficient appreciation of the anatomical and physiological challenges of the nasal airway generally, and of the inadequacies of conventional delivery technologies in particular, suggest that the potential for N2B delivery has been underestimated in human studies that achieve inadequate IN delivery, for example by use of conventional spray pumps. Understanding and addressing these issues may be the key to unlocking the ultimate potential of IN delivery for treatment of brain disorders in man.
The impact of the complex nasal anatomy and physiology on device performance and clinical results is often neglected when discussing the potential of IN drug delivery in man. A recent review describes the inherent anatomical and physiological challenges for efficient nasal drug delivery in man from a clinical perspective for a variety of therapeutic applications [Citation23]. In the following paragraphs we summarize some key factors that seem most relevant for targeted nose-to-brain delivery.
Introduction to the physiology of the nasal airway
Nasal breathing is vital for most animals and also for human neonates in the first weeks of life. Newborn babies are obligate nasal breathers and the nose remains the normal and preferred airway during sleep, rest and even during mild-to-moderate exercise throughout life. In a healthy adult more than 12,000 liters of air pass through the nose every day [Citation76]. The complex structure, physiology and aerodynamic profile of the nasal passages reflect the structures’ multifaceted role, including acting as airway, filter, air temperature regulator, component of the immune system and important sensory organ [Citation77].
The nasal valve & aerodynamics
Although most of the nasal cavity is lined with richly vascularized respiratory epithelium, the nasal vestibule, which is the portion of the nasal cavity anterior to the nasal valve, is covered by non-ciliated squamous epithelium and is, therefore, not a target for nasal drug delivery, although small lipophilic molecules may be absorbed even from this surface (). At the nostril, the entrance to the nose, the shape of the nasal cavity varies between circular and oval. As the nasal passage bends and constricts, the cross-sectional shape of the cavity becomes more elongated and triangular with the narrowest dimensions of the triangle lying superiorly (). This narrow constriction is termed the nasal valve region and is located approximately 2–3 cm from the nostril, with a mean cross-sectional area of only 0.5–0.6 cm2 on each side [Citation78]. The nasal valve is the narrowest segment of the entire respiratory tract accounting for as much as 50–75% of the total airway resistance and represents an often underestimated hurdle for nasal drug delivery [Citation79]. The soft-walled valve region does not have a static conformation, expanding and contracting in response to changing pressures and dynamically regulating nasal airflow by disrupting the laminar flow pattern and redirecting the inspired air to create local turbulence that traps particles. The cross-sectional area of the nasal cavity increases beyond the nasal valve as the epithelium transitions to ciliated respiratory type, and airflow is slowed down and distributed primarily across the narrow passages and convoluted mucosal surfaces of the turbinates of the lower and middle segments. The nasal valve plays a key and dynamic role in fulfilling a prime function of the nose as an air temperature regulator, a particle filter and an efficient scrubber to remove hazardous particles and gaseous/vapor phase pollutants, and protect the sensitive lower airways from hazardous exposures [Citation80].
In addition, vigorous nasal inhalation/sniffing – often attempted in an effort to draw air or medication deep into the nasal cavity – creates a negative pressure that actually causes dynamic narrowing and even collapse of the nasal valve, particularly in the upper narrow regions of the valve. Consequently, only a very small fraction of the inspired air actually reaches the olfactory region during nasal respiration () [Citation81]. However, to increase olfactory airflow and optimize olfaction the valve collapse associated with sniffing can be at least partially counteracted by the voluntary activation of dilating muscles of the nostrils known as 'flaring' [Citation82]. The nasal valve also plays an important role during exhalation by acting as an expiratory 'brake' increasing the time for gas exchange in the alveoli [Citation83,Citation84]. In the context of nasal drug delivery, and in particular for drug delivery to the upper parts of the nose housing the olfactory nerves, the small dimensions and dynamic inspiratory obstruction represent essential and often ignored obstacles for efficient nasal drug delivery of all types, and particularly for N2B drug delivery where access to the more superior and posterior segments of the nasal cavity is particularly desired.
The nasal cycle
The turbinates and the medial (septal) and lateral walls of the nasal cavity contain richly vascular 'erectile' tissues that are responsible for a normally observed physiological cycle, alternating between sides, of congestion and decongestion observed in 80% of healthy individuals [Citation78]. Due to this reciprocal 1–4 h autonomic cycling of mucosal swelling, at any given time one of the nostrils is generally considerably more congested with most of the airflow passing through the other nasal passage while a stable total combined intranasal resistance is maintained [Citation85]. Normal physiologic cycling is modified by a variety of stimuli including physical and sexual activity, emotional states and by infection and inflammation. Consequently, on the cyclically congested side the access to intranasal structures beyond the nasal valve, and in particular to the region of the olfactory cleft, becomes restricted.
Filtration & clearance
The dense vascularization of the respiratory epithelium allows efficient exchange of heat and moisture of inhaled air. During exhalation, the process is reversed; breathing out through the nose reduces the body's loss of heat and moisture as compared with oral exhalation. The respiratory epithelium is covered by a normal mucus layer which helps remove noxious gases and to trap particles smaller than 3–10 μm. Trapped particles and infective agents behind the nasal valve are moved posteriorly by the beating cilia at a mean pace of 6–10 mm/min where they are presented to the abundant specialized lymphatic tissues of the nose and nasopharynx and ultimately swallowed (mucociliary clearance) [Citation77,Citation86].
It takes about 10–20 min for a particle deposited at the head of the inferior turbinate to reach the base of the tongue [Citation86]. The density and beat frequency of cilia varies [Citation87]. However, contrary to common perception, recent gamma deposition studies suggest that the clearance from the upper nasal segment including the olfactory region is as fast as from the other ciliated regions of the nose [Citation48]. Powder seems to be cleared somewhat slower, possibly due to the time it takes for the powder to dissolve, but in general particles have only minutes available for absorption or receptor binding [Citation48]. Excipients that slow or temporarily halt ciliary action may increase the residence time for a drug and may be beneficial for delivery of some drugs and in treatment for some indications, but may also introduce adverse effects on tolerability, reduce the surface covered by the drug, add complexity of formulation work, and possibly add to regulatory hurdles.
Nasal & sinus vasculature & lymphatic system
The specific site of IN drug deposition may influence not only N2B transport, but also the extent, mechanism and pathway of systemic absorption. While branches of the facial artery supply the region of the vestibule, the ophthalmic and maxillary arteries supply the mucous membranes covering the rest of the nasal passage and adjacent sinuses [Citation86,Citation88].
Similarly the venous drainage of the anterior region of the nose is primarily via the external jugular veins, whereas drugs absorbed across the mucosa beyond the nasal valve are more likely to drain to the cavernous venous sinuses, which lie adjacent to the pituitary gland and are traversed by cranial nerves III, IV, V and VI, and also by the internal carotid arteries. For some small molecules that easily pass the BBB (e.g., midazolam), this mechanism of local 'counter-current transfer' has in rats been shown to offer a preferential first-pass distribution to the brain [Citation89,Citation90]. The significance of this mechanism in man is unknown, but the cavernous sinus–carotid artery complex has a similar structure in rat and man. The lymphatic vessels from the vestibule and external nose drain to submandibular lymph nodes, whereas the deeper nasal structures and paranasal sinuses drain to lymph nodes in the nasopharynx and adjacent internal structures [Citation77]. The brain does not have lymphatic vessels but, as described above, the perivascular spaces along the olfactory and trigeminal nerves and as recently demonstrated also alongside cerebral arteries and veins, may act as lymphatic pathways between the CNS and the nose and have been implicated in the transport of molecules from the nasal cavity to the CNS [Citation14,Citation91].
Innervation of the nasal mucosa
The olfactory and trigeminal nerves interact in a complex manner to minimize the exposure to, and effects of, potentially noxious substances by alteration of the nasal patency and mucous blanket properties [Citation69]. Odorous sensations may be amplified by trigeminal input through perception of nasal airflow and at the chemosensory level. Interestingly, an area of increased trigeminal chemosensitivity is found in the anterior part of the nose, mediating touch, pressure, temperature and pain [Citation69]. Trigeminal pain receptors in the nose are not covered by squamous epithelium, which gives chemical stimuli almost direct access to the free nerve endings (). In fact, loss of trigeminal sensitivity and function, and not just olfactory nerve function, may severely reduce the sense of smell [Citation92,Citation93].
Olfactory nerve axons originating in the olfactory bulb penetrate the cribriform plate and extend downwards on both sides of the olfactory cleft. Filaments of the olfactory nerves are not restricted to the olfactory cleft, but that islets of olfactory epithelium are present in both the anterior and posterior parts at the middle turbinate ( & ) [Citation63,Citation94]. Furthermore, trigeminal nerve sensory fibers contribute to olfaction by mediating a 'common chemical sense' [Citation69]. The ophthalmic branch of the trigeminal nerve innervates the anterior part of the nose including the vestibule, whereas sensory maxillary branches innervate the deeper nasal segments including the olfactory cleft. This suggests that, in contrast to the prevailing opinion, a combination of targeted delivery to the olfactory region and a broad distribution to the mucosa innervated by the trigeminal nerve may be optimal for N2B delivery. It is of course necessary to consider both the benefits and potential toxicity of delivering specific therapeutics broadly and deeply in the nasal cavity, and safety considerations are addressed further in more detail below.
Sensitivity of the nasal mucosa & limitations of in vitro predictive models
The high sensitivity of the mucosa in the vestibule and in the valve area as a natural nasal defense is also often neglected when the potential of nasal drug delivery is discussed. High-speed impaction and locally concentrated anterior drug deposition on the septum, as well as direct physical contact with a nasal spray device tip during actuation, may cause mucosal irritation and injury, reducing patient acceptance and compliance [Citation69,Citation95]. During anesthesia and/or sedation often applied to laboratory animals, normal reflexes driven by sensory input are suppressed, potentially limiting the clinical relevance of findings. Furthermore, despite the attraction of relatively inexpensive, quick and easy in vitro model approaches to study of the nasal physiology associated with drug delivery, there are limitations on the predictive value of in vitro testing of airflow and deposition patterns such as that performed in nasal casts and with computational fluid dynamics (CFD) models. In fact, the in vitro approaches available today may be severely limited by multiple factors, including the lack of sensory feedback responses, unrealistic surface properties and/or dimensions (especially dynamic dimensions and the nasal valve, variability in human nasal anatomic structure and function and an inability to properly represent the effects of device-user interaction [Citation96,Citation97]. Of note, objective assessment of the dimensions at the critically important nasal valve region of a particular cast described as 'anatomically correct' and used in some recent deposition studies has found dimensions that appear to be much larger and outside the normal range (Abstract ARS annual meeting 2012) [Citation98–100]. Consequently, research data gathered from use of this cast, which is made for educational purposes, can be highly misleading (data on file). Unfortunately, many authors continue to advocate cast studies and CFD as valid surrogates for human testing and clinical trials, rather than simply as interesting preliminary indicators to help inform the range of required human testing [Citation98–101].
The mismatch between spray plume & nasal geometry
The obvious mismatch between the shape of the expanding plumes generated by mechanical nasal spray pumps, pressurized metered dose inhaler (pMDI)'s and nebulizers, and the gradually constricting dimension of the nasal vestibule, the narrow barrier of the nasal valve region, and the complex slit-like labyrinthine geometry beyond the nasal valve, is given surprisingly little attention in many reviews on the potential of nasal drug delivery.
The plume generated by standard mechanical spray is hollow with particles mainly at the periphery [Citation101,Citation102], a phenomenon potentially accentuated with 'vortex' style plumes. Furthermore, a standard conical spray plume of about 60 degrees has a diameter of 2 cm at a distance of only 1 cm from the spray nozzle, and at 3 cm from the tip the diameter is >3cm [Citation101,Citation102]. Thus, even if the spray tip is inserted as much as 10–15 mm into the vestibule of the nose there is an obvious mismatch between the dimensions of the narrow triangular shaped nasal valve region and the expanding circular spray plume. The drug particles located primarily in the periphery of the plume will impinge in the non-ciliated mucosal walls of the nasal vestibule, anterior to the valve. Particles actually penetrating the nasal valve will do so primarily in the lower (wider) part of the triangular shaped valve and, thus, will tend to pass along primarily to the lower part of the nasal passages [Citation101]. Sniffing, either intentionally or by reflex to avoid liquid drip-out, will dynamically further narrow the nasal valve and passages, accentuating this pattern. Drug deposited inferiorly or passing through the nasal valve inferiorly will tend to pass, particularly in the presence of sniffing, along the nasal floor to the base of the tongue where it is swallowed. This pattern of deposition, accentuated by sniffing, further reduces access to the upper target sites in the nose, which are of particular interest for N2B delivery. A similar mismatch exists between the constricting geometry of the nasal vestibule and the expanding plumes produced by other devices such as pMDIs and nebulizers/atomizers delivering liquid or powders.
Reaching target sites in the human
A more comprehensive general description of all existing and emerging nasal delivery technologies can be found in a recent review paper [Citation23]. The following review, in the context of issues surrounding achievement of N2B drug delivery, will focus on technologies where quantification of deposition in the olfactory regions and/or in the upper posterior segment of the nasal cavity has been conducted and/or technologies that specifically claim such capabilities.
Spay pumps, drops & syringes with tubes
The distance from the nostril to the olfactory epithelium is only a few centimeters, but its location in the slit-like olfactory cleft behind the narrow nasal valve and at the end of a complex labyrinth of passages severely complicates access. Otorhinolaryngologists routinely inspect this area and the other remote regions of the nose as part of a rhinological examination using either thin flexible or rigid rod-like endoscopes. An experienced rhinologist can therefore often, in the absence of major anterior nasal pathology, insert a thin semi-flexible tube attached to a syringe and squirt a drug directly into the olfactory region. Applying local anesthesia and/or decongestion prior to the delivery procedure may further improve access to the olfactory cleft.
In a study evaluating the olfactory deposition of a liquid dye delivered with this type of syringe-and-tube performed by a trained physician, after decongestion and in recumbent subjects, delivery to the olfactory cleft was achieved in 11 out of 15 subjects [Citation103]. In the same study, delivery of drops and of a traditional spray by the subjects themselves, also after decongestion, showed olfactory deposition in only one subject after spray and in none after drops, clearly illustrating the inadequacy of these traditional methods in reaching the olfactory cleft [Citation103]. This demonstrates that although olfactory delivery is achievable with an impractical medical procedure it is unsuited for routine drug delivery at home (and even in most clinics). These data, and other data, also show that commonly used nasal delivery devices are relatively useless for targeting drug delivery to the olfactory region as would be desired for N2B delivery. In fact, the 'human factor' is often ignored in studies assessing nasal in vivo deposition and in Phase 1 trials. Even results obtained after spray delivery by an assistant may not reflect the real-life situation of suffering patients [Citation23,Citation104,Citation105]. In fact, in a combined PK and gamma deposition study where insulin was self-administered with a traditional spray pump, deposition was strictly on the anterior non-ciliated mucosa in five out of six subjects and with no sign of systemic insulin absorption [Citation105]. The authors commented that this effect of individual administration technique raises a separate question on the usefulness of nasal spray doses for delivery of insulin intended for systemic absorption [Citation105].
The inability to reach the olfactory region with traditional devices has been confirmed in a study assessing deposition using a endoscopic fluorescein technique [Citation106]. Administration to one nostril of 120 ml with a squeeze bottle (Sinus Rinse™ NeilMed) and 0.15 ml 1% sodium hyaluronate and 0.2 ml isotonic saline delivered with a spray showed that regardless of the method used, the vast majority of fluorescein was deposited on the septum and inferior turbinate with minimal deposition even at the level of the middle turbinate [Citation106]. This conclusion was further confirmed by a recent radiographic CT imaging study using radiopaque contrast showing that neither spray nor drops were detected in superior nasal spaces [Citation107].
As expected, CFD studies in an MRI-based nasal airway model also confirmed that olfactory deposition is possible with deep intubation with particle release close to the olfactory region [Citation97]. CFD studies of delivery from a spray device at the nasal entrance, however, show very limited deposition in the olfactory region [Citation101]. These findings are in agreement with a comprehensive review from 2004 concluding that existing delivery methods are suboptimal in reaching target sites beyond the nasal valve [Citation108]. Another very recent review highlighted the increasing concerns related to the variability of the response to intranasal oxytocin, as delivered with a nasal spray, between studies and individuals [Citation75]. The authors state: “most researchers using oxytocin nasal spray, however, do not have a history of expertise in using this technology and our knowledge about how nasal sprays impact on the CNS and peripheral sites in humans is limited”. They go on to state that; “if the critical pathway is mediated by deposition onto the olfactory cleft, it is almost certainly the case that standard spray bottles are not the ideal delivery device for such treatment. In this case, alternative delivery devices need to be identified” [Citation44,Citation75].
Nebulizers & atomizers
Nebulizers utilize compressed air/gas, mechanical or ultrasonic power to break up liquid solutions or suspensions into small aerosol droplets. The aerosol can be administered passively or assisted by active nasal inhalation or even assisted by suction from the contralateral nostril [Citation109–111]. Nebulizers are primarily used to deliver topically acting drugs such as antibiotics or steroids in patients with chronic rhinosinusitis. The openings to the sinuses are located primary under the middle turbinate in the upper posterior parts of the nose. Thus, optimizing delivery to this region is compatible with a delivery approach suitable for drugs intended for N2B delivery.
In the late 1990s, Suman et al. described that nasal inhalation of smaller particles (6 micron) produced by a nebulizer could enhance deposition to the upper parts of the nasal cavity when compared with a traditional spray pump [Citation109]. More recently, vibrating mesh and sonic jet nebulizers have been introduced for nasal drug delivery of small particles employing use of special breathing techniques by the patient (e.g., breath hold during delivery) and/or suction from the contralateral nostril in order to attempt to mitigate the undesirable side-effects produced by unintended deposition of small drug particles in the lungs during delivery [Citation110,Citation111].
Vortical flow atomizer
A handheld battery-driven nasal atomizer has been introduced (ViaNase™ by Kurve Technology Inc., Lynnwood, WA, USA). A vortical flow of liquid droplets is produced and the flow characteristics can be altered in circular velocity and direction to achieve different droplet trajectories [Citation112,Citation113]. The ability to achieve 'customized' delivery and administer a wide variety of solutions and suspensions with varying surface tension and viscosity are claimed to be advantages of this technology. Gamma images following delivery with this device have suggested that this technology is capable of targeting the upper parts of the nose and sinuses. However, no numerical quantification of nasal or sinus deposition verifying the claimed improved deposition has been published [Citation112,Citation113]. Although it is unknown how effectively this device delivers drug to the upper posterior region of the nasal cavity, insulin delivered with this device has shown effects in patients with early Alzheimer's disease (AD) [Citation114,Citation115]. In these studies, the nasal inhalation of insulin from the device was performed over a 2-min period, obviously exposing study subjects to drug deposition (in this case insulin) in the lungs [Citation116]. The ViaNase device is also to be used in a project involving delivery of an investigational possible fast-acting antidepressant (thyrotropin-releasing hormone). The key focus of the latter study, however, is not direct N2B transport, but enhanced systemic absorption of a TRH formulation with nanoparticles, a formulation hypothesized to move the drug past the BBB. Apart from this press release, there has been no news about the use of this technology since 2007 [Citation117].
Pressurized metered dose inhalers
The particles from a pMDI are released at a high speed via the expansion of a compressed gas, which is also responsible for discomfort and dryness described as the 'cold Freon' effect. Following the ban in 2003 of the ozone-depleting chlorofluorocarbons (CFC) propellant, the propellant hydrofluoroalkanes (HFA) has been introduced for both pulmonary and nasal products [Citation118]. The HFA pMDIs produce a slower particle velocity than the old CFC-driven pMDIs (15 vs 52 m/s at a distance 1–2 cm from the actuator tip). The new 'slow mist' HFA-based pMDIs are reported to give less discomfort compared with the CFC pMDIs, and without the tendency to cause as much 'drip out' as is typical of traditional liquid spray pumps [Citation118]. Notably, the speed of the emitted particles from the HFA driven pMDIs is still similar to or higher than the speed of particles emitted from a conventional spray pump (5–20 m/s) [Citation101,Citation119]. Recently two nasal products based on the HFA pMDI technology have been introduced, but for topical steroids indicated for allergic rhinitis and not for N2B-type applications [Citation120,Citation121].
Impel Pharma is developing a nitrogen-driven pressurized delivery device producing a narrow plume that is claimed to be suitable for N2B delivery [Citation122]. The human experience with this device is very limited, but in general, pressurized gas driven devices are easy to use and can be operated by assistants. A study in rats found that >50% more of the dose was deposited in the region of the rat olfactory epithelium with this device when compared with drops deposited at the nares to be inhaled, and with evidence of enhanced transport of morphine and fentanyl from the nose to the brain [Citation123]. While this supports previous findings that deposition in the olfactory regions enhances direct N2B transport in rats, deposition of drops at the nares in anesthetized rats to be inhaled may not be an appropriate representation of most human drug delivery alternatives. Reaching the olfactory epithelium, which covers 50% of the nasal mucosa in rats, in anesthetized animals is also not very challenging. However, with a narrow plume directed towards the nasal valve, it may be possible to enhance disposition in the upper parts of the nose beyond the nasal valve compared with the wider plume of standard nasal sprays. Interestingly, Impel recently announced a study in seven human volunteers with a labeled peptide that allegedly demonstrates 'first proof in man' of N2B transport. The data available publicly are only in the form of a press release, and no details concerning the peptide or the labeling process are available. In an interview after the press release, it was stated that the increased activity (relative to a standard spray) was observed in the brain stem, which suggests that the primary site of deposition produced by the device may be in the regions of the nose innervated by the trigeminal nerve rather than regions innervated by the olfactory nerve [Citation124]. As previously noted, however, pMDIs are associated with a number of potential limitations. Although the reported findings are promising for the field of N2B in man it remains to be seen if a pressurized 'open-palate' nebulizer will be capable of delivering on the promises. Details on the recent trial are expected in a peer-reviewed publication.
Breath Powered Bi-Directional™ nasal delivery
The Breath Powered delivery concept was developed to overcome the anatomical and physiological characteristics of the nasal airways that limit the delivery efficiency of traditional delivery technologies [Citation23]. Devices using this technology can be adapted to a variety of dispersion technologies for powder and liquid, and have been described in detail in previous publications. The devices have a flexible mouthpiece and a shaped sealing nosepiece designed for a suitable fit while mechanically expanding particularly the superior portion of the entrance of the nasal valve region without obstructively compressing soft tissues ( & ). The nosepiece is first inserted to form a seal with the nostril and then the user exhales forcefully into the mouthpiece (Breath Powered). The sealing nosepiece introduces the naturally warmed and humidified exhaled air into the nasal cavity. This process automatically elevates and seals the soft palate to separate the oral cavity from the nasal cavity, as occurs when inflating a balloon. The positive pressure transferred into the nose due to the sealing nosepiece also further expands the nasal valve and the narrow slit-like passage to propel the particles to the target sites beyond the valve region. Importantly, the variable transferred air pressure in the nasal cavity correctly balances the variable air pressure applied to the soft palate from the oropharynx, thus preventing excessive elevation of the soft palate which may obstruct the passage of air posteriorly around the nasal septum. The entry air passes posteriorly around the nasal septum and then anteriorly to exit through the opposite nostril (Bi-Directional) ().
Implementations of this technology for delivery of powder and liquid treatments have been developed and tested in multiple human clinical trials [Citation125–129]. The most tested dry powder device uses disposable unit-dose nosepieces to deliver 5–25 mg of medication per administration. The most tested multidose liquid device uses a different design. With the liquid device, a static positive pressure is created when first exhaling into the mouthpiece. The user then actuates a spray pump in the device to simultaneously release the drug and the exhaled air pressure, thus creating a burst of warm humid air that carries the liquid droplet mist into the upper deeper parts of the complex nasal passages. In the Breath Powered powder device the particles are carried with the airflow. In the Breath Powered liquid device the velocity of the airflow burst through the nosepiece at the applicator tip of the device is similar to the velocity of the emitted particles for the spray nozzle, thus reducing the high speed impaction and associated discomfort of particle impingement and minimizing the unwanted anterior deposition of traditional spray pumps [Citation23]. With all devices using Breath Powered Bi-Directional delivery, a positive oropharyngeal pressure is present at all times during delivery and the soft palate is also sealed, completely preventing the risk of lung inhalation during the drug-delivery process [Citation130]. The flow rate and particle size profile can also be optimized to enable targeted delivery. It is inherent to the nature of closed-palate Breath Powered delivery that a certain level of user participation is generally required. For the devices currently available, the subject must be awake, conscious and able to insert the device properly and perform the exhalation procedure as instructed. Consequently, in young children and individuals unable or unwilling to cooperate (e.g., ictal subjects with epilepsy, certain subjects with stroke, ICU patients) the advantages cannot be fully exploited. In uncooperative patients and others where there is an absence of exhalation, the liquid device performs similarly to a traditional spray pump. In principle, a variant device (powder or liquid) can be developed to be operated by an assistant blowing through the device in a fashion that transfers balanced pressure to both the oral cavity and one nostril, but this application is at a prototype stage and has not been developed in the fashion of the other devices.
As noted, the positive driving pressure and airflow during Breath Powered delivery tend to open the narrow valve and nasal passage. This effect is likely to be an advantage in case of infectious or allergic rhinitis with accompanying mucosal swelling. Finally, the nature of the Breath Powered Bi-Directional delivery concept significantly reduces the issues and variability typical to conventional spray pumps as discussed in a recent review [Citation23]. The range of issues include the anatomical and physiological state of the nasal passage, the device delivery techniques and the inhalation maneuvers, plume angle and spray tip direction and insertion depth, and particle size distribution [Citation119].
A study comparing pharmacokinetics and sedation after of the same dose of midazolam (liquid) delivered with a Breath Powered device, a traditional spray pump and IV injection is suggestive of direct N2B effects, potentially due to enhanced deposition with Breath Powered delivery [Citation131]. Other studies comparing pharmacokinetics and migraine pain relief after delivery of sumatriptan with a Breath Powered powder device in comparison to liquid nasal spray and SC injection also suggest the possibility that the targeted deposition may enhance the effects in a manner that cannot be attributed to improved serum absorption of sumatriptan alone [Citation125,Citation127,Citation129,Citation132].
Regional quantification of human nasal deposition with different delivery technologies
A recent MedLine search identified only five relevant studies reporting human in vivo regional quantification of nasal deposition by gamma scintigraphy to assess delivery to deep target regions in the nose by various drug-delivery technologies [Citation48,Citation49,Citation96,Citation109–111,Citation133]. One study compared a traditional spray pump to nasal inhalation from a standard nebulizer delivering small particles intended to increase deposition beyond the nasal valve [Citation109]. Another study, using the same method for regional quantification, compared two spray pumps with different plume characteristics [Citation96]. Radioactive helium was used to outline the contours of the nasal cavities, and in both studies the nasal cavity was divided into nine segments and ratios between inner (I) and outer (O) segments and upper (U) and lower (L) segments were reported. Another study compared two novel nasal nebulizers, a sonic jet nebulizer and a vibrating mesh nebulizer with suction from the exit nostril [Citation111]. Finally, two studies compared the deposition patterns of traditional spray pumps to liquid and powder versions, respectively, of Breath Powered Bi-Directional delivery devices [Citation48,Citation49].
The mean U:L and I:O ratios were higher with the nebulizer versus spray pump, suggesting more deposition in the upper and posterior regions, but up to 56% of the dose was inhaled to the lungs, raising questions about the safety of the nebulizer form of nasal administration, particularly with smaller particles [Citation109]. Deposition from the two spray pumps compared was not statistically different [Citation96]. The comparison of two novel nebulizers (sonic jet and vibrating mesh with exit suction) showed maximum deposition for both to be 2 cm from the nostril in the horizontal plane, corresponding to the location of the narrow nasal valve. In the vertical plane the maximum distribution with the mesh nebulizer was closer to the nasal floor than the sonic jet nebulizer (0.75 vs 1.2 cm) [Citation111]. Finally both Breath Powered devices were found to deposit significantly higher fractions in the upper posterior parts of the nose compared with traditional spray pumps [Citation48,Citation49,Citation134]. Traditional spray pumps deliver primarily to the lower anterior and posterior segments, leaving important parts of the nose largely unexplored ( & ). Importantly, comparison of clearance curves demonstrate that the overall nasal clearance curve reported in many studies is of limited value [Citation48,Citation49].
Breath Powered device optimized for N2B delivery
For the purpose of further accentuating targeted delivery to the olfactory region and the upper posterior third of the nasal passage, a dedicated nosepiece variant has been developed for Breath Powered devices, with an elongated tip capable of flexing laterally and made from a soft material to enable better insertion into the narrow upper part of the nasal valve ().
Data collected [Unpublished Data] on this 'N2B device' powder variant suggest higher deposition in the upper third of the nose than reported for a standard Breath Powered devices, albeit in different groups of healthy subjects (), which in turn have been shown to be superior to conventional spray delivery [Citation48,Citation49]. With the N2B nosepiece, more that 30% of the delivered dose was deposited in the upper third of the nose, and approximately 80% in the upper two thirds where olfactory filaments may be found (, & ). Less than 20% is deposited in the lower third with the both Breath Powered powder variants compared with the 60% that has been previously reported for a liquid spray (). The regional clearance patterns over time show that the particles in the upper ciliated parts of the nose move backwards and downwards into the segment below, explaining an increase in exposure in the lower posterior segment from about 8–10 min onwards [Citation48].
Nasal deposition systemic absorption & biological responses
As noted previously, it is a true challenge when attempting to assess and predict the efficacy of delivery from the nose into the CNS that systemic absorption/exposure does not, by definition, predict the biological and clinical responses that might be expected from activity produced by direct entry into the CNS. Even for systemic absorption of drugs from the nose there is a delicate and complex balance between a large number of factors, including the surface area covered, type of mucosa reached, the molecular size and other physiochemical properties of the molecule, the dissolution rate and the rate of drug removal by mucociliary clearance [Citation119].
For predicting CNS entry, the complexity may be even greater. However, with inadequate delivery to the target regions in the nose where cranial innervation (and perhaps preferential venous drainage) is present, even optimal formulations will be less likely to succeed. Deposition in the properly innervated regions of the nasal cavity can therefore be expected to be a critical component of the overall approach towards realizing the full potential of N2B delivery. An illustration of this is a study in rats showing that drug deposition of a small molecule in the olfactory regions results in doubling of the CNS exposure and half of the systemic exposure compared with deposition in the regions covered simply by respiratory mucosa [Citation97,Citation135,Citation136].
Safety considerations
Many CNS disorders where N2B delivery is attractive are chronic disorders that may require lifelong treatment. The nasal mucosa is designed to trap and handle foreign particles, but it is also an important sensory organ. Long-term or frequent exposure to nasal drugs and excipients may have potential to cause irritation and local toxicity.
The crusting and epistaxis occasionally observed after long-term use of steroid nasal sprays are primarily device-related side-effects caused by a combination of mechanical irritation of the spray tip and/or high drug exposure in the region lined with non-ciliated mucosa anterior to the nasal valve. This illustrates the potential importance of evaluating devices and drugs for intranasal delivery to assess potential for local irritation or intolerance. It is fortunate that the respiratory nasal mucosa beyond the nasal valve regenerates rapidly and does not display tachyphylaxis typical to long-term skin exposure. Furthermore, several macromolecules such as oxytocin and vasopressin have been used for decades without the emergence of significant concerns over local toxicity. On the other hand, nasal products containing zinc have been associated with reduction/loss in olfaction [Citation137]. Topical decongestants are approved only for short-term use due to the risk of irritation and rebound nasal congestion (rhinitis medicamentosa) [Citation138]. Physiochemical factors such as concentration, pH and solubility play important roles in the safety and tolerability of any substance being delivered to the nose, and tolerability of both the primary active molecule and of any adjuvants and other formulation components in combination must be considered. Delivery of some therapeutics, most notably macromolecules and stem cells, to the nasal mucosa may also pose the risk of undesirable immunological reactions.
There are multiple safety and tolerability questions that must be addressed in the development of new intranasal therapeutics, much as is true for other routes of delivery. Extensive testing of local toxicity and long-term safety is required for new substances intended for nasal delivery in humans. Naturally, this also applies to new excipients such as absorption enhancers. It is a long way from animal studies to approval for human use, explaining why only a few enhancers have reached or are close to reaching the market [Citation139]. However, similar and potentially greater challenges exist for new substances intended for CNS action delivered through other routes and for formulation strategies intended to open the BBB. Despite the described challenges, nasal delivery has the unique potential advantage that drugs may be transported directly to the brain with reduced systemic exposure. Irrespective of approach, safety and tolerability considerations will need to be balanced against potential benefits in the development of new treatments.
When discussing the CNS potential of direct N2B delivery of drugs which poorly penetrate the BBB, it is important to remember that systemic absorption is not the goal, although it may be desirable in other intranasal applications. Many drugs intended for CNS actions may have undesirable systemic effects and the goal is to limit systemic absorption while promoting direct transport to the relevant brain regions. Thus, enhancers intended specifically to improve systemic absorption seem to offer no advantages for N2B transport. With regard to the use of topical vasoconstrictors, the data are conflicting. In a study applying topical phenylephrine in combination with the macromolecule hypocretin-1, increased N2B transport and reduced systemic absorption was observed [Citation140], whereas co-administration of ephedrine and a small molecule did not show increased N2B transport [Citation141]. Interestingly, the same group reported significantly improved N2B transport and reduced systemic absorption by delivering a small molecule directly on the olfactory epithelium in rats (15 mm) when compared with delivery on the respiratory epithelium 7 mm from the nostril [Citation136]. The crucial role of the deposition site is often forgotten when discussing the possible advantages of absorption enhancers. Nevertheless, as long as the correct segment in the nose is reached, it seems reasonable that excipients that can reversibly and safely slow clearance may offer advantages. Moreover, for drugs with some degree of BBB penetration, a combination of systemic absorption and direct transport may be ideal and absorption enhancers may have an important place. Ideally, an excipient that can promote direct transport from the nose to the brain could be developed.
Conclusion
N2B transport is a promising emerging field that may be one of the solutions for the significant difficulties in getting emerging brain therapies into the CNS where they can achieve the desired activity. The barriers to CNS entry of drugs include both the BBB and the BCSFB, and these challenge research into N2B transport. The evidence for N2B transport that has already been developed in animals and the mechanisms for such transport and the issues that surround translating animal research into human implications have been discussed.
We have reviewed core concepts in human nasal anatomy and physiology, how they influence nasal drug deposition patterns and the potential for achieving N2B drug transport in man. We have also discussed the limitations of in vitro models and CFD simulations and the important need for human studies of drug deposition. Lastly, we have provided a scan of available technologies for delivering drugs in a manner that may produce N2B transport and a review of the available data to suggest successful N2B type deposition and transport.
Future perspective
Much research is still needed in the field of N2B delivery of CNS therapeutics. The lack of relationship, and/or variable relationship, between the serum PK profile (and perhaps even CSF levels) of a drug and the desired clinical effects in the brain complicates the development and regulatory approval of such therapies. Insufficient appreciation of the inherent challenges imposed by the complex nasal anatomy and physiology may also have slowed progress in this field. With increased recognition of these hurdles, new nasal delivery devices have been designed enabling more targeted delivery to the sites innervated by the cranial nerves that offer direct pathways from the nose to the brain. In our opinion, progress in nasal device performance, alone or in combinations with novel formulation strategies, represents a potentially important contributor to future success in CNS delivery. Reliable methods for assessment of regional deposition and clearance in man are essential for comparison of new devices and formulation technologies and to document the relationship between device performance and clinical outcomes. Innovations in imaging technologies will hopefully allow direct labeling of drugs or ligands that can be safely used to detect N2B transport in man. Progress in fMRI and PET technologies allows for ever more detailed mapping of brain activity that, in combination with PK measurements and functional assessment such as cognitive testing, may provide improved evidence of human N2B transport. These and other approaches may help to better characterize N2B transport in ways that allow prediction of transport activity, and thereby facilitate the development of future CNS therapies. Ultimately, the success of N2B in man will be realized when new therapeutic benefits are demonstrated for brain diseases in man that were not possible before the development of technologies capable of exploiting this fascinating new approach to drug delivery. The nose may know the way.
Nose-to-brain transport: Transfer of substances into the brain via the olfactory and trigeminal nerves occurring by slow intra-axonal transport or by faster transfer along the perineural space surrounding the nerve cells into the cerebrospinal fluid and/or into the interstitial fluid of the brain.
Blood–cerebrospinal fluid barrier: The capillaries in the choroid plexus differ from those of the brain in that molecules can move more freely across the endothelial cell through fenestrations and intercellular gaps by diffusion, facilitated diffusion and active transport into cerebrospinal fluid.
Nasal valve: The narrow anterior triangular-shaped flow-limiting segment of the nasal airway that dynamically modifies the direction and distribution of the nasal airflow and drug particles delivered to the nose.
Nasal mucociliary clearance: Transport system that protects the respiratory system clearing particles and drugs trapped in the mucus layer covering the ciliated respiratory mucosa found beyond the nasal valve.
Breath Powered™ Bi-Directional™ delivery: Nasal delivery concept designed to overcome the anatomical challenges of the nasal airways that limit the delivery efficiency of traditional delivery technologies.
The unmet need
In the context of the intricate pathophysiology of brain disorders and increasingly complex, high-risk and costly drug development, difficulty in delivering therapeutics into the CNS is a major hurdle for new CNS therapies.
Barriers to accessing the brain
The blood and the CNS are separated by two protective conceptual barriers with different properties, the blood–brain barrier (BBB) and the blood–cerebrospinal fluid (CSF) barrier (BCSFB).
The BBB is comprised of capillaries lined with endothelium connected by tight junctions, which severely limit penetration of substances that cannot exploit dedicated active transport mechanisms.
The BCSFB has two components in series (blood to CSF and CSF to brain) and is functionally distinct and more 'leaky' than the BBB.
Drug penetration from the CSF into the brain may be quantitatively unimportant and the value of CSF levels in predicting central effects appears to be limited.
Complex strategies involving re-engineering of drug molecules to exploit endogenous mechanisms for transport across the BBB are being explored.
The nose – an alternative route of brain delivery
Animal studies show that rapid direct drug transport along the olfactory and trigeminal nerves allows brain access for small and large molecules and even stem cells in therapeutic concentrations ('nose to brain' or N2B).
Based on the rapid speed of transport from the nose to CNS structures, bulk transport along the ensheathing channels surrounding olfactory and trigeminal nerves is the most likely mechanism.
Once inside the brain, further transport to other regions can be expected to occur by 'perivascular pump' mechanisms driven by arterial pulsations.
Anatomical & physiological challenges in man
Intranasal delivery to achieve N2B has been pursued, but the impact of the complex nasal anatomy and physiology on device performance and clinical results is often neglected when discussing the potential of nasal drug delivery in man.
The mismatch between the expanding plumes generated by mechanical nasal spray pumps, pressurized metered dose inhalers (pMDIs) and nebulizers and the anterior narrow nasal valve region is given surprisingly little attention.
Regional quantification of human nasal deposition with different delivery technologies
Traditional devices like spray pumps, drops, nebulizers and pMDI's are suboptimal and deliver primarily to the lower anterior and posterior segments, leaving important parts of the nose largely unexposed.
Breath Powered™ nasal devices have been best demonstrated to deposit significantly higher fractions in the upper posterior parts of the nose compared with traditional spray pumps.
Nasal deposition systemic absorption & biological responses
Systemic absorption/exposure is not the goal and blood levels do not predict the biological and clinical responses that might be expected from activity produced by direct entry into the CNS.
The crucial role of intranasal deposition site is often forgotten when discussing the possible advantages of nasal absorption enhancers.
Future perspective
N2B transport is a promising emerging field which may be one of the solutions for the significant difficulties in getting innovative brain therapies into the CNS where they can achieve the desired activity.
In our opinion progress in nasal delivery device performance, alone or in combination with novel formulation strategies, represent key opportunities for future success in developing treatments for brain disorders.
(A) Lateral section of the human nose and brain showing key anatomical structures and nerves relevant to drug transport from the blood to brain and from the nose to the brain. (B) The potential transport routes for substances into the brain from the blood across the blood–brain barrier and along the olfactory and the trigeminal pathways. In order to reach the neurons in the brain, substances in the blood must cross the tight endothelial barrier of the brain capillaries (the blood–brain barrier), or first cross the more 'leaky' barrier of the choroid plexus into the CSF and subsequently cross the barrier between the CSF and the brain interstitium (blood–CSF barrier). The olfactory filaments penetrate the nasal mucosa of the upper part of the nose and substances may be transported inside the nerve axon (intracellular). Transport across the mucosa also occurs between the cells (paracellular) or through the cells (transcellular). After crossing the mucosa substances may follow channels surrounding the nerve bundles (perineuronal), be absorbed into submucosal blood and lymphatic vessels or move into the subarachnoid CSF where they may enter the brain interstitum via perivascular channels. The trigeminal nerve endings do not penetrate the mucosal surface. Substances must cross the mucosa and continue along the same transport routes as described above. A part of the ophthalmic branch (V1) innervates the upper anterior nasal segment with similar projections as the olfactory nerve. The maxillary branch (V2) provides sensory and parasympathetic innervation to the majority of the respiratory mucosa and projects to the brain stem.
CSF: Cerebrospinal fluid.
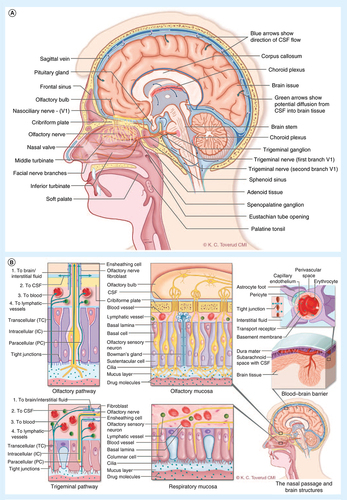
Cross-sections of the complex nasal passages and endoscopic images highlighting the small dimensions and narrow geometry of the nasal valve, as well as its dynamics during nasal inhalation and when a positive pressure is applied. The target sites for N2B delivery are located in the upperconvoluted slit-like nasal passages beyond this narrow anterior nasal valve.
Reproduced with permission from [Citation132].
![Figure 2. The complex nasal anatomy and the nasal valve.Cross-sections of the complex nasal passages and endoscopic images highlighting the small dimensions and narrow geometry of the nasal valve, as well as its dynamics during nasal inhalation and when a positive pressure is applied. The target sites for N2B delivery are located in the upperconvoluted slit-like nasal passages beyond this narrow anterior nasal valve.Reproduced with permission from [Citation132].](/cms/asset/44ffeaa7-dd20-4cc8-a4aa-57e1082a4f98/itde_a_12366113_f0002.jpg)
Lateral and superior view of the nasal passages illustrating the Breath Powered Bi-Directional nasal delivery concept (see text for more detailed description).
Reproduced with permission from [Citation23,Citation132].
![Figure 3. Breath Powered™ Bi-Directional™ nasal delivery concept.Lateral and superior view of the nasal passages illustrating the Breath Powered Bi-Directional nasal delivery concept (see text for more detailed description).Reproduced with permission from [Citation23,Citation132].](/cms/asset/99a5ce25-ff04-448e-b120-86640f19b161/itde_a_12366113_f0003.jpg)
Multiuse Breath Powered powder device incorporating a standard size 3 capsule in disposable nosepiece unit and the Breath Powered multidose liquid device incorporating a standard spray pump with 120 doses.
Reproduced with permission from [Citation48,Citation132].
![Figure 4. Breath Powered™ Bi-Directional™ nasal delivery devices.Multiuse Breath Powered powder device incorporating a standard size 3 capsule in disposable nosepiece unit and the Breath Powered multidose liquid device incorporating a standard spray pump with 120 doses.Reproduced with permission from [Citation48,Citation132].](/cms/asset/5e5c6ca1-84d7-4ee2-9028-45d85caaa400/itde_a_12366113_f0004.jpg)
Initial regional gamma deposition (A) and horizontal distribution (B) 2 min after self-administration with conventional spray pump and Breath Powered™ Bi-Directional™ nasal powder device.
Reproduced with permission from [Citation48,Citation132].
![Figure 5. Initial regional gamma deposition data.Initial regional gamma deposition (A) and horizontal distribution (B) 2 min after self-administration with conventional spray pump and Breath Powered™ Bi-Directional™ nasal powder device.Reproduced with permission from [Citation48,Citation132].](/cms/asset/71c2e26a-600d-4ff9-86e6-35f5c42d52d1/itde_a_12366113_f0005.jpg)
Initial gamma deposition (0–2 min after delivery) in the same healthy subject following self-administration with a traditional spray and three Breath Powered™ device variants, the standard and the nose to brain variants of the multidose liquid device and the powder device shown in
N2B: Nose to brain.
Reproduced with permission from [Citation23,Citation48,Citation132].
![Figure 6. Initial gamma deposition in the same subject.Initial gamma deposition (0–2 min after delivery) in the same healthy subject following self-administration with a traditional spray and three Breath Powered™ device variants, the standard and the nose to brain variants of the multidose liquid device and the powder device shown in Figure 4.N2B: Nose to brain.Reproduced with permission from [Citation23,Citation48,Citation132].](/cms/asset/9269a38a-98de-4126-bf68-1af3bb47e173/itde_a_12366113_f0006.jpg)
Mean initial nasal gamma deposition in seven healthy subjects 2 min after self-administration with the Breath Powered™ nose to brain device variant. Approximately 30% and 80% of the delivered dose was deposited in the upper third and upper two-thirds, respectively. Approximately 15% was found in the lower third and a small fraction (∼5%) was deposited or had been cleared to the nasopharynx 2 min after delivery.
Reproduced with permission from [Citation23,Citation48].
![Figure 7. Breath Powered™ nose to brain powder device variant; initial horizontal distribution.Mean initial nasal gamma deposition in seven healthy subjects 2 min after self-administration with the Breath Powered™ nose to brain device variant. Approximately 30% and 80% of the delivered dose was deposited in the upper third and upper two-thirds, respectively. Approximately 15% was found in the lower third and a small fraction (∼5%) was deposited or had been cleared to the nasopharynx 2 min after delivery.Reproduced with permission from [Citation23,Citation48].](/cms/asset/c341bdad-e7e4-4405-a51e-bc8c4e1fcf2c/itde_a_12366113_f0007.jpg)
Financial & competing interests disclosure
All three authors are employees of and hold share options in OptiNose. Per G Djupesland is one of the founders of OptiNose, a member of the board of directors and owns shares in OptiNose. The authors have no other relevant affiliations or financial involvement with any organization or entity with a financial interest in or financial conflict with the subject matter or materials discussed in the manuscript apart from those disclosed.
No writing assistance was utilized in the production of this manuscript.
References
- Nutt DJ Attridge J . CNS drug development in Europe – past progress and future challenges . Neurobiol. Dis.61 , 6 – 20 ( 2013 ).
- Abbott A . Schizophrenia. The drug deadlock . Nature468 ( 7321 ), 158 – 159 ( 2010 ).
- Pardridge WM . Drug transport across the blood–brain barrier . J. Cerebr. Blood F. Met.32 ( 11 ), 1959 – 1972 ( 2012 ).
- Pinzon-Daza ML Campia I Kopecka J Garzon R Ghigo D Rigant C . Nanoparticle- and liposome-carried drugs: new strategies for active targeting and drug delivery across blood–brain barrier . Curr. Drug Metab.14 ( 6 ), 625 – 640 ( 2013 ).
- Lochhead JJ Thorne RG . Intranasal delivery of biologics to the central nervous system . Adv. Drug Deliv. Rev.64 ( 7 ), 614 – 628 ( 2012 ).
- Chapman CD Frey WH 2nd Craft S et al. Intranasal treatment of central nervous system dysfunction in humans . Pharm. Res.30 ( 10 ), 2475 – 2484 ( 2012 ).
- Bastir M Rosas A Gunz P et al. Evolution of the base of the brain in highly encephalized human species . Nat. Commun.2 , 588 ( 2011 ).
- Merkus FW Van Den Berg MP . Can nasal drug delivery bypass the blood-brain barrier?: questioning the direct transport theory . Drugs R. D.8 ( 3 ), 133 – 144 ( 2007 ).
- Abbott NJ . Blood-brain barrier structure and function and the challenges for CNS drug delivery . J. Inherit. Metab. Dis.36 ( 3 ), 437 – 449 ( 2013 ).
- Laterra J Keep R Betz LA Goldstein GW . Blood–cerebrospinal fluid barrier . In : Basic Neurochemistry: Molecular, Cellular and Medical Aspects.6th Edition . In : SiegelGJAgranoffBWAlbersRWet al. (Eds). Lippincott-Raven , PA, USA ( 1999 ).
- Pardridge WM . Drug transport in brain via the cerebrospinal fluid . Fluids Barriers CNS8 ( 1 ), 7 ( 2011 ).
- Landis MS Boyden T Pegg S . Nasal-to-CNS drug delivery: where are we now and where are we heading? An industrial perspective . Ther. Deliv.3 ( 2 ), 195 – 208 ( 2012 ).
- De Lange EC Danhof M . Considerations in the use of cerebrospinal fluid pharmacokinetics to predict brain target concentrations in the clinical setting: implications of the barriers between blood and brain . Clin. Pharmacokinet.41 ( 10 ), 691 – 703 ( 2002 ).
- Iliff JJ Wang M Liao Y et al. A paravascular pathway facilitates CSF flow through the brain parenchyma and the clearance of interstitial solutes, including amyloid beta . Sci. Transl. Med.4 ( 147 ), 147ra111 ( 2012 ).
- Chen Y Liu L . Modern methods for delivery of drugs across the blood–brain barrier . Adv. Drug Deliv. Rev.64 ( 7 ), 640 – 665 ( 2012 ).
- Banks W . Drug transport into the central nervous system: using newer findings about the blood–brain barriers . Drug Deliv. Transl. Res.2 , 152 – 159 ( 2012 ).
- Pardridge WM Boado RJ . Reengineering biopharmaceuticals for targeted delivery across the blood–brain barrier . Methods Enzymol.503 , 269 – 292 ( 2012 ).
- Abdelbary GA Tadros MI . Brain targeting of olanzapine via intranasal delivery of core-shell difunctional block copolymer mixed nanomicellar carriers: in vitro characterization, ex vivo estimation of nasal toxicity and in vivo biodistribution studies . Int. J. Pharm.452 ( 1–2 ), 300 – 310 ( 2013 ).
- Mistry A Stolnik S Illum L . Nanoparticles for direct nose-to-brain delivery of drugs . Int. J. Pharm.379 ( 1 ), 146 – 157 ( 2009 ).
- Kanazawa T Akiyama F Kakizaki S Takashima Y Seta Y . Delivery of siRNA to the brain using a combination of nose-to-brain delivery and cell-penetrating peptide-modified nano-micelles . Biomaterials34 ( 36 ), 9220 – 9226 ( 2013 ).
- Pardeshi CV Belgamwar VS . Direct nose to brain drug delivery via integrated nerve pathways bypassing the blood-brain barrier: an excellent platform for brain targeting . Expert Opin. Drug Deliv.10 ( 7 ), 957 – 972 ( 2013 ).
- Patel MM Goyal BR Bhadada SV Bhatt JS Amin AF . Getting into the brain: approaches to enhance brain drug delivery . CNS Drugs23 ( 1 ), 35 – 58 ( 2009 ).
- Djupesland PG . Nasal drug delivery devices: characteristics and performance in a clinical perspective-a review . Drug Deliv. Transl. Res.3 ( 1 ), 42 – 62 ( 2013 ).
- Djupesland PG Mahmoud RA Messina JC . Accessing the brain: the nose may know the way . J. Cerebr. Blood F. Met.33 , 793 – 794 ( 2013 ).
- Danielyan L Schafer R Von Ameln-Mayerhofer A et al. Therapeutic efficacy of intranasally delivered mesenchymal stem cells in a rat model of Parkinson disease . Rejuvenation Res.14 ( 1 ), 3 – 16 ( 2011 ).
- Johnson NJ Hanson LR Frey WH . Trigeminal pathways deliver a low molecular weight drug from the nose to the brain and orofacial structures . Mol. Pharm.7 ( 3 ), 884 – 893 ( 2010 ).
- Van Woensel M Wauthoz N Rosiere R et al. Formulations for intranasal delivery of pharmacological agents to combat brain disease: a new opportunity to tackle GBM? Cancers 5 ( 3 ), 1020 – 1048 ( 2013 ).
- Freiherr J Hallschmid M Frey WH 2nd et al. Intranasal insulin as a treatment for Alzheimer's disease: a review of basic research and clinical evidence . CNS Drugs27 ( 7 ), 505 – 514 ( 2013 ).
- Westin UE Bostrom E Grasjo J Hammarlund-Udenaes M Bjork E . Direct nose-to-brain transfer of morphine after nasal administration to rats . Pharm. Res.23 ( 3 ), 565 – 572 ( 2006 ).
- Dhuria SV Hanson LR Frey WH 2nd. Intranasal drug targeting of hypocretin-1 (orexin-A) to the central nervous system . J. Pharm. Sci.98 ( 7 ), 2501 – 2515 ( 2009 ).
- Stevens J Ploeger BA Van Der Graaf PH Danhof M De Lange EC . Systemic and direct nose-to-brain transport pharmacokinetic model for remoxipride after intravenous and intranasal administration . Drug Metab. Dispos.39 ( 12 ), 2275 – 2282 ( 2011 ).
- Shingaki T Inoue D Furubayashi T et al. Transnasal delivery of methotrexate to brain tumors in rats: a new strategy for brain tumor chemotherapy . Mol. Pharm.7 ( 5 ), 1561 – 1568 ( 2010 ).
- Lu W Jiang W Chen J Yin M Wang Z Jiang X . Modulation of brain delivery and copulation by intranasal apomorphine hydrochloride . Int. J. Pharm.349 ( 1–2 ), 196 – 205 ( 2008 ).
- Wang D Gao Y Yun L . Study on brain targeting of raltitrexed following intranasal administration in rats . Cancer Chemother. Pharmacol.57 ( 1 ), 97 – 104 ( 2006 ).
- Wang C Quan LH Guo Y Liu CY Liao YH . Uptake and biodistribution of rizatriptan to blood and brain following different routes of administration in rats . Int. J. Pharm.337 ( 1–2 ), 155 – 160 ( 2007 ).
- Liu XF Fawcett JR Thorne RG Frey WH 2nd . Non-invasive intranasal insulin-like growth factor-I reduces infarct volume and improves neurologic function in rats following middle cerebral artery occlusion . Neurosci. Lett.308 ( 2 ), 91 – 94 ( 2001 ).
- Liu XF Fawcett JR Thorne RG Defor TA Frey WH 2nd . Intranasal administration of insulin-like growth factor-I bypasses the blood-brain barrier and protects against focal cerebral ischemic damage . J. Neurol. Sci.187 ( 1–2 ), 91 – 97 ( 2001 ).
- Topkoru BC Altay O Duris K Krafft PR Yan J Zhang JH . Nasal administration of recombinant osteopontin attenuates early brain injury after subarachnoid hemorrhage . Stroke44 ( 11 ), 3189 – 3194 ( 2013 ).
- Gomez D Martinez JA Hanson LR Frey WH 2nd Toth CC . Intranasal treatment of neurodegenerative diseases and stroke . Front. Biosci.4 , 74 – 89 ( 2012 ).
- Thorne RG Hanson LR Ross TM Tung D Frey WH 2nd . Delivery of interferon-beta to the monkey nervous system following intranasal administration . Neuroscience152 ( 3 ), 785 – 797 ( 2008 ).
- Thorne RG Pronk GJ Padmanabhan V Frey WH 2nd . Delivery of insulin-like growth factor-I to the rat brain and spinal cord along olfactory and trigeminal pathways following intranasal administration . Neuroscience127 ( 2 ), 481 – 496 ( 2004 ).
- Danielyan L Schafer R Von Ameln-Mayerhofer A et al. Intranasal delivery of cells to the brain . Eur. J. Cell Biol.88 ( 6 ), 315 – 324 ( 2009 ).
- Deadwyler SA Porrino L Siegel JM Hampson RE . Systemic and nasal delivery of orexin-A (Hypocretin-1) reduces the effects of sleep deprivation on cognitive performance in nonhuman primates . J. Neurosci.27 ( 52 ), 14239 – 14247 ( 2007 ).
- Guastella AJ Macleod C . A critical review of the influence of oxytocin nasal spray on social cognition in humans: evidence and future directions . Horm. Behav.61 ( 3 ), 410 – 418 ( 2012 ).
- Veening JG Olivier B . Intranasal administration of oxytocin: behavioral and clinical effects, a review . Neurosci. Biobehav. Rev.37 ( 8 ), 1445 – 1465 ( 2013 ).
- Schioth HB Craft S Brooks SJ Frey WH 2nd Benedict C . Brain insulin signaling and Alzheimer's disease: current evidence and future directions . Mol. Neurobiol.46 ( 1 ), 4 – 10 ( 2012 ).
- Born J Lange T Kern W Mcgregor GP Bickel U Fehm HL . Sniffing neuropeptides: a transnasal approach to the human brain . Nat. Neurosci.5 ( 6 ), 514 – 516 ( 2002 ).
- Djupesland PG Skretting A . Nasal deposition and clearance in man: comparison of a bidirectional powder device and a traditional liquid spray pump . J. Aerosol Med. Pulm. Drug Deliv.25 ( 5 ), 280 – 289 ( 2012 ).
- Djupesland PG Skretting A Winderen M Holand T . Breath actuated device improves delivery to target sites beyond the nasal valve . Laryngoscope116 ( 3 ), 466 – 472 ( 2006 ).
- Van Den Berg MP Merkus P Romeijn SG Verhoef JC Merkus FW . Uptake of melatonin into the cerebrospinal fluid after nasal and intravenous delivery: studies in rats and comparison with a human study . Pharm. Res.21 ( 5 ), 799 – 802 ( 2004 ).
- Merkus P Guchelaar HJ Bosch DA Merkus FW . Direct access of drugs to the human brain after intranasal drug administration?Neurology60 ( 10 ), 1669 – 1671 ( 2003 ).
- Hashizume R Ozawa T Gryaznov SM et al. New therapeutic approach for brain tumors: Intranasal delivery of telomerase inhibitor GRN163 . Neuro. Oncol.10 ( 2 ), 112 – 120 ( 2008 ).
- Ozduman K Wollmann G Piepmeier JM Van Den Pol AN . Systemic vesicular stomatitis virus selectively destroys multifocal glioma and metastatic carcinoma in brain . J. Neurosci.28 ( 8 ), 1882 – 1893 ( 2008 ).
- Balyasnikova IV Prasol MS Ferguson SD et al. Intranasal delivery of mesenchymal stem cells significantly extends survival of irradiated mice with experimental brain tumors . Mol. Ther. doi:10.1038/mt.2013.199 ( 2013 ) (Epub ahead of print) .
- Da Fonseca CO Schwartsmann G Fischer J et al. Preliminary results from a Phase I/II study of perillyl alcohol intranasal administration in adults with recurrent malignant gliomas . Surg. Neurol.70 ( 3 ), 259 – 266 ; discussion266 – 257 ( 2008 ).
- Da Fonseca CO Masini M Futuro D Caetano R Gattass CR Quirico-Santos T . Anaplastic oligodendroglioma responding favorably to intranasal delivery of perillyl alcohol: a case report and literature review . Surg. Neurol.66 ( 6 ), 611 – 615 ( 2006 ).
- Reitz M Demestre M Sedlacik J et al. Intranasal delivery of neural stem/progenitor cells: a noninvasive passage to target intracerebral glioma . Stem Cells Transl. Med.1 ( 12 ), 866 – 873 ( 2012 ).
- Aboody KS Najbauer J Metz MZ et al. Neural stem cell-mediated enzyme/prodrug therapy for glioma: preclinical studies . Sci. Transl. Med.5 ( 184 ), 184ra159 ( 2013 ).
- Wu S Li K Yan Y et al. Intranasal Delivery of Neural Stem Cells: A CNS-specific, non-invasive cell-based therapy for experimental autoimmune encephalomyelitis . J. Clin. Cell. Immunol.4 ( 3 ), ( 2013 ).
- Aboody KS Najbauer J Danks MK . Stem and progenitor cell-mediated tumor selective gene therapy . Gene Ther.15 ( 10 ), 739 – 752 ( 2008 ).
- Hadaczek P Yamashita Y Mirek H et al. The “perivascular pump” driven by arterial pulsation is a powerful mechanism for the distribution of therapeutic molecules within the brain . Mol. Ther.14 ( 1 ), 69 – 78 ( 2006 ).
- Thorne RG Emory CR Ala TA Frey WH 2nd . Quantitative analysis of the olfactory pathway for drug delivery to the brain . Brain Res.692 ( 1–2 ), 278 – 282 ( 1995 ).
- Leopold DA Hummel T Schwob JE Hong SC Knecht M Kobal G . Anterior distribution of human olfactory epithelium . Laryngoscope110 ( 3 Pt 1 ), 417 – 421 ( 2000 ).
- Kaur P Kim K . Pharmacokinetics and brain uptake of diazepam after intravenous and intranasal administration in rats and rabbits . Int. J. Pharm.364 ( 1 ), 27 – 35 ( 2008 ).
- Forbes AR Gamsu G . Lung mucociliary clearance after anesthesia with spontaneous and controlled ventilation . Am. Rev. Respir. Dis.120 ( 4 ), 857 – 862 ( 1979 ).
- Cervin A Lindberg S Mercke U . Effects of halothane on mucociliary activity in vivo . Otolaryngol. Head Neck Surg.112 ( 6 ), 714 – 722 ( 1995 ).
- Kesimci E Bercin S Kutluhan A Ural A Yamanturk B Kanbak O . Volatile anesthetics and mucociliary clearance . Minerva Anestesiol.74 ( 4 ), 107 – 111 ( 2008 ).
- Catani M Dell’acqua F Thiebaut De Schotten M . A revised limbic system model for memory, emotion and behaviour . Neurosci. Biobehav. Rev.37 ( 8 ), 1724 – 1737 ( 2013 ).
- Hummel T Livermore A . Intranasal chemosensory function of the trigeminal nerve and aspects of its relation to olfaction . Int. Arch. Occup. Environ. Health.75 ( 5 ), 305 – 313 ( 2002 ).
- Ivanusic JJ Kwok MM Jennings EA . Peripheral targets of 5-HT(1D) receptor immunoreactive trigeminal ganglion neurons . Headache51 ( 5 ), 744 – 751 ( 2011 ).
- Ivanusic JJ Kwok MM Ahn AH Jennings EA . 5-HT(1D) receptor immunoreactivity in the sphenopalatine ganglion: implications for the efficacy of triptans in the treatment of autonomic signs associated with cluster headache . Headache51 ( 3 ), 392 – 402 ( 2011 ).
- Shemesh E Rudich A Harman-Boehm I Cukierman-Yaffe T . Effect of intranasal insulin on cognitive function: a systematic review . J. Clin. Endocrinol. Metab.97 ( 2 ), 366 – 376 ( 2012 ).
- Hollander E Bartz J Chaplin W et al. Oxytocin increases retention of social cognition in autism . Biol. Psychiatry61 ( 4 ), 498 – 503 ( 2007 ).
- Hollander E Novotny S Hanratty M et al. Oxytocin infusion reduces repetitive behaviors in adults with autistic and Asperger's disorders . Neuropsychopharmacology28 ( 1 ), 193 – 198 ( 2003 ).
- Guastella AJ Hickie IB Mcguinness MM et al. Recommendations for the standardisation of oxytocin nasal administration and guidelines for its reporting in human research . Psychoneuroendocrinology38 ( 5 ), 612 – 625 ( 2013 ).
- Cole P Savard P Miljeteig H Haight JS . Resistance to respiratory airflow of the extrapulmonary airways . Laryngoscope103 ( 4 Pt 1 ), 447 – 450 ( 1993 ).
- Sahin-Yilmaz A Naclerio RM . Anatomy and physiology of the upper airway . Proc. Am. Thorac. Soc.8 ( 1 ), 31 – 39 ( 2011 ).
- Cole P . The four components of the nasal valve . Am. J. Rhinol.17 ( 2 ), 107 – 110 ( 2003 ).
- Haight JS Cole P . The site and function of the nasal valve . Laryngoscope93 ( 1 ), 49 – 55 ( 1983 ).
- Nodelman V Ultman JS . Longitudinal distribution of chlorine absorption in human airways: comparison of nasal and oral quiet breathing . J. Appl. Physiol.86 ( 6 ), 1984 – 1993 ( 1999 ).
- Schroeter JD Garcia GJ Kimbell JS . A computational fluid dynamics approach to assess interhuman variability in hydrogen sulfide nasal dosimetry . Inhal. Toxicol.22 ( 4 ), 277 – 286 ( 2010 ).
- Mann DG Sasaki CT Fukuda H Mann DG Suzuki M Hernandez JR . Dilator naris muscle . Ann. Otol. Rhinol. Laryngol.86 ( 3 Pt 1 ), 362 – 370 ( 1977 ).
- Hairfield WM Warren DW Hinton VA Seaton DL . Inspiratory and expiratory effects of nasal breathing . Cleft Palate J.24 ( 3 ), 183 – 189 ( 1987 ).
- Djupesland PG Skatvedt O Borgersen AK . Dichotomous physiological effects of nocturnal external nasal dilation in heavy snorers: the answer to a rhinologic controversy?Am. J. Rhinol.15 ( 2 ), 95 – 103 ( 2001 ).
- Cole P . Stability of nasal airflow resistance . Clin. Otolaryngol. Allied Sci.14 ( 2 ), 177 – 182 ( 1989 ).
- Dahl R Mygind N . Anatomy, physiology and function of the nasal cavities in health and disease . Adv. Drug Deliv. Rev.29 ( 1–2 ), 3 – 12 ( 1998 ).
- Halama AR Decreton S Bijloos JM Clement PA . Density of epithelial cells in the normal human nose and the paranasal sinus mucosa. A scanning electron microscopic study . Rhinology28 ( 1 ), 25 – 32 ( 1990 ).
- Jones N . The nose and paranasal sinuses physiology and anatomy . Adv. Drug Deliv. Rev.51 ( 1–3 ), 5 – 19 ( 2001 ).
- Einer-Jensen N Larsen L . Local transfer of diazepam, but not of cocaine, from the nasal cavities to the brain arterial blood in rats . Pharmacol. Toxicol.87 ( 6 ), 276 – 278 ( 2000 ).
- Einer-Jensen N Larsen L Deprez S Starns E Schwartz S . Intranasal absorption of sumatriptan and naratriptan: no evidence of local transfer from the nasal cavities to the brain arterial blood in male rats . Biopharm. Drug Dispos.22 ( 5 ), 213 – 219 ( 2001 ).
- Dhuria SV Hanson LR Frey WH 2nd . Intranasal delivery to the central nervous system: mechanisms and experimental considerations . J. Pharm. Sci.99 ( 4 ), 1654 – 1673 ( 2010 ).
- Daiber P Genovese F Schriever VA Hummel T Mohrlen F Frings S . Neuropeptide receptors provide a signalling pathway for trigeminal modulation of olfactory transduction . Eur. J. Neurosci.37 ( 4 ), 572 – 582 ( 2013 ).
- Husner A Frasnelli J Welge-Lussen A Reiss G Zahnert T Hummel T . Loss of trigeminal sensitivity reduces olfactory function . Laryngoscope116 ( 8 ), 1520 – 1522 ( 2006 ).
- Feron F Perry C Mcgrath JJ Mackay-Sim A . New techniques for biopsy and culture of human olfactory epithelial neurons . Arch. Otolaryngol. Head Neck Surg.124 ( 8 ), 861 – 866 ( 1998 ).
- Waddell AN Patel SK Toma AG Maw AR . Intranasal steroid sprays in the treatment of rhinitis: is one better than another?J. Laryngol. Otol.117 ( 11 ), 843 – 845 ( 2003 ).
- Suman JD Laube BL Dalby R . Validity of in vitro tests on aqueous spray pumps as surrogates for nasal deposition, absorption, and biologic response . J. Aerosol. Med.19 ( 4 ), 510 – 521 ( 2006 ).
- Si XA Xi J Kim J Zhou Y Zhong H . Modeling of release position and ventilation effects on olfactory aerosol drug delivery . Respir. Physiol. Neurobiol.186 ( 1 ), 22 – 32 ( 2013 ).
- Kundoor V Dalby RN . Effect of formulation- and administration-related variables on deposition pattern of nasal spray pumps evaluated using a nasal cast . Pharm. Res.28 ( 8 ), 1895 – 1904 ( 2011 ).
- Kundoor V Dalby RN . Assessment of nasal spray deposition pattern in a silicone human nose model using a color-based method . Pharm. Res.27 ( 1 ), 30 – 36 ( 2010 ).
- Guo Y Laube B Dalby R . The effect of formulation variables and breathing patterns on the site of nasal deposition in an anatomically correct model . Pharm. Res.22 ( 11 ), 1871 – 1878 ( 2005 ).
- Fung MC Inthavong K Yang W Lappas P Tu J . External characteristics of unsteady spray atomization from a nasal spray device . J. Pharm. Sci.102 ( 3 ), 1024 – 1035 ( 2013 ).
- Inthavong K Tian ZF Tu JY Yang W Xue C . Optimising nasal spray parameters for efficient drug delivery using computational fluid dynamics . Comput. Biol. Med.38 ( 6 ), 713 – 726 ( 2008 ).
- Scheibe M Bethge C Witt M Hummel T . Intranasal administration of drugs . Otolaryngol. Head Neck Surg.134 ( 6 ), 643 – 646 ( 2008 ).
- Al-Ghananeem AM Sandefer EP Doll WJ Page RC Chang Y Digenis GA . Gamma scintigraphy for testing bioequivalence: a case study on two cromolyn sodium nasal spray preparations . Int. J. Pharm.357 ( 1–2 ), 70 – 76 ( 2008 ).
- Mcinnes FJ O’mahony B Lindsay B et al. Nasal residence of insulin containing lyophilised nasal insert formulations, using gamma scintigraphy . Eur. J. Pharm. Sci.31 ( 1 ), 25 – 31 ( 2007 ).
- Bleier BS Debnath I Harvey RJ Schlosser RJ . Temporospatial quantification of fluorescein-labeled sinonasal irrigation delivery . Int. Forum Allergy Rhinol.1 ( 5 ), 361 – 365 ( 2011 ).
- Rudman KL O’brien EK Leopold DA . Radiographic distribution of drops and sprays within the sinonasal cavities . Am. J. Rhinol. Allergy25 ( 2 ), 94 – 97 ( 2011 ).
- Aggarwal R Cardozo A Homer JJ . The assessment of topical nasal drug distribution . Clin. Otolaryngol. Allied Sci.29 ( 3 ), 201 – 205 ( 2004 ).
- Suman JD Laube BL Dalby R . Comparison of nasal deposition and clearance of aerosol generated by nebulizer and an aqueous spray pump . Pharm. Res.16 ( 10 ), 1648 – 1652 ( 1999 ).
- Moller W Saba GK Haussinger K Becker S Keller M Schuschnig U . Nasally inhaled pulsating aerosols: lung, sinus and nose deposition . Rhinology49 ( 3 ), 286 – 291 ( 2011 ).
- Vecellio L De Gersem R Le Guellec S et al. Deposition of aerosols delivered by nasal route with jet and mesh nebulizers . Int. J. Pharm.407 ( 1–2 ), 87 – 94 ( 2011 ).
- Laube BL . Devices for aerosol delivery to treat sinusitis . J. Aerosol Med.20 ( Suppl. 1 ), S5 – S17 ; discussionS17 – S18 ( 2007 ).
- Giroux M . Controlled Particle Dispersion: Effective Nasal Delivery from a Versatile, Flexible Delivery Platform.ON drug Delivery Ltd , East Sussex, UK , 13 – 15 ( 2005 )
- Craft S Baker LD Montine TJ et al. Intranasal insulin therapy for Alzheimer disease and amnestic mild cognitive impairment: a pilot clinical trial . Arch. Neurol.69 ( 1 ), 29 – 38 ( 2012 ).
- Reger MA Watson GS Green PS et al. Intranasal insulin improves cognition and modulates beta-amyloid in early AD . Neurology70 ( 6 ), 440 – 448 ( 2008 ).
- Djupesland PG . Intranasal insulin improves cognition and modulates beta-amyloid in early AD . Neurology71 ( 11 ), 864 ; author reply864 ( 2008 ).
- Kurve Technology . www.kurvetech.com
- Hankin CS Cox L Lang D et al. Medical costs and adherence in patients receiving aqueous versus pressurized aerosol formulations of intranasal corticosteroids . 33 ( 3 ), 258 – 264 ( 2012 ).
- Suman J . Current understanding of nasal morphology and physiology as a drug delivery target . Drug Deliv. Transl. Res.3 ( 1 ), 4 – 15 ( 2013 ).
- Berger WE Mohar DE Laforce C et al. A 26-week tolerability study of ciclesonide nasal aerosol in patients with perennial allergic rhinitis . Am. J. Rhinol. Allergy26 ( 4 ), 302 – 307 ( 2012 ).
- Mohar D Berger WE Laforce C et al. Efficacy and tolerability study of ciclesonide nasal aerosol in patients with perennial allergic rhinitis . Allergy Asthma Proc.33 ( 1 ), 19 – 26 ( 2012 ).
- Impelpharma . www.impelpharma.com
- Hoekman JD Ho RJ . Enhanced analgesic responses after preferential delivery of morphine and fentanyl to the olfactory epithelium in rats . Anesth. Analg.113 ( 3 ), 641 – 651 ( 2011 ).
- University TechWATCH . http://utechwatch.com/2013/05/impel-neuropharma-succeeds-in-nose-to-brain-drug-delivery-clinical-trial
- Obaidi M Offman E Messina J Carothers J Djupesland PG Mahmoud RA . Improved pharmacokinetics of sumatriptan with breath powered nasal delivery of sumatriptan powder . Headache53 ( 8 ), 1323 – 1333 ( 2013 ).
- Hansen FS Djupesland PG Fokkens WJ . Preliminary efficacy of fluticasone delivered by a novel device in recalcitrant chronic rhinosinusitis . Rhinology48 ( 3 ), 292 – 299 ( 2010 ).
- Djupesland PG Docekal P . Intranasal sumatriptan powder delivered by a novel breath-actuated bi-directional device for the acute treatment of migraine: a randomised, placebo-controlled study . Cephalalgia30 ( 8 ), 933 – 942 ( 2010 ).
- Vlckova I Navratil P Kana R Pavlicek P Chrbolka P Djupesland PG . Effective treatment of mild-to-moderate nasal polyposis with fluticasone delivered by a novel device . Rhinology47 ( 4 ), 419 – 426 ( 2009 ).
- Luthringer R Djupesland PG Sheldrake CD et al. Rapid absorption of sumatriptan powder and effects on glyceryl trinitrate model of headache following intranasal delivery using a novel bi-directional device . J. Pharm. Pharmacol.61 ( 9 ), 1219 – 1228 ( 2009 ).
- Djupesland PG Skretting A Winderen M Holand T . Bi-directional nasal delivery of aerosols can prevent lung deposition . J. Aerosol Med.17 ( 3 ), 249 – 259 ( 2004 ).
- Dale O Nilsen T Loftsson T et al. Intranasal midazolam: a comparison of two delivery devices in human volunteers . J. Pharm. Pharmacol.58 ( 10 ), 1311 – 1318 ( 2006 ).
- Djupesland PG Messina JC Mahmoud RA . Breath powered nasal delivery: a new route to rapid headache relief . Headache53 ( Suppl. 2 ), 72 – 84 ( 2013 ).
- Moller W Lubbers C Munzing W Canis M . Pulsating airflow and drug delivery to paranasal sinuses . Curr. Opin. Otolaryngol Head Neck Surg.19 ( 1 ), 48 – 53 ( 2011 ).
- Skretting A Djupesland PG . A new method for scintigraphic quantification of deposition and clearance in anatomical regions of the human nose . Nucl. Med. Commun.30 ( 8 ), 629 – 638 ( 2009 ).
- Charlton ST Whetstone J Fayinka ST Read KD Illum L Davis SS . Evaluation of direct transport pathways of glycine receptor antagonists and an angiotensin antagonist from the nasal cavity to the central nervous system in the rat model . Pharm. Res.25 ( 7 ), 1531 – 1543 ( 2008 ).
- Charlton ST Davis SS Illum L . Nasal administration of an angiotensin antagonist in the rat model: effect of bioadhesive formulations on the distribution of drugs to the systemic and central nervous systems . Int. J. Pharm.338 ( 1–2 ), 94 – 103 ( 2007 ).
- Alexander TH Davidson TM . Intranasal zinc and anosmia: the zinc-induced anosmia syndrome . Laryngoscope116 ( 2 ), 217 – 220 ( 2006 ).
- Graf PM . Rhinitis medicamentosa . J. Allergy Clin. Immunol.19 , 295 – 304 ( 2007 ).
- Illum L . Nasal drug delivery – recent developments and future prospects . J. Control. Release161 ( 2 ), 254 – 263 ( 2012 ).
- Dhuria SV Hanson LR Frey WH 2nd . Novel vasoconstrictor formulation to enhance intranasal targeting of neuropeptide therapeutics to the central nervous system . J. Pharmacol. Exp. Ther.328 ( 1 ), 312 – 320 ( 2009 ).
- Charlton ST Davis SS Illum L . Evaluation of effect of ephedrine on the transport of drugs from the nasal cavity to the systemic circulation and the central nervous system . J. Drug Target.15 ( 5 ), 370 – 377 ( 2007 ).