Abstract
Population studies suggest that greater dietary fiber intake may lower colorectal cancer (CRC) risk, possibly through the colonic bacterial fermentative production of butyrate. Butyrylated starch delivers butyrate to the colon of humans with potential to reduce CRC risk but high doses may exacerbate risk through promoting epithelial proliferation. Here we report the effects of increasing dietary butyrylated high amylose maize starch (HAMSB) on azoxymethane (AOM) induced distal colonic DNA damage, cell proliferation, mucus layer thickness and apoptosis in rats. Five groups of 15 rats were fed AIN-93G based diets containing 0–40% HAMSB for 4 weeks then injected with (AOM) and killed 6 hours later. Large bowel total SCFA, acetate and butyrate pools and hepatic portal venous plasma total SCFA, acetate and butyrate concentrations were higher with greater HAMSB intake. Distal colonic epithelial apoptotic index and colonic mucus thickness increased, while DNA single strand breaks decreased dose-dependently with greater HAMSB intake. Colonocyte proliferation rates were unaffected by diet. These data suggest that increasing large bowel butyrate may reduce the risk of CRC in a dose dependent manner by enhancing apoptotic surveillance in the colonic epithelium for damaged cells without promoting the risk of tumorigenesis through increased cell proliferation.
Abbreviations
AI | = | apoptotic index |
AOM | = | azoxymethane |
HAMS | = | high amylose maize starch |
HAMSB | = | butyrylated high amylose maize starch |
HAMSB0 | = | base diet containing 0% HAMSB |
HAMSB5 | = | base diet containing 5% HAMSB |
HAMSB10 | = | base diet containing 10% HAMSB |
HAMSB20 | = | base diet containing 20% HAMSB |
HAMSB40 | = | base diet containing 40% HAMSB |
RS | = | resistant starch |
SCFA | = | short chain fatty acid |
SSB | = | single-stranded DNA breaks |
Introduction
Colorectal cancer (CRC) is one of the most common cancers world-wide and there is convincing evidence that consumption of dietary fiber is protective.Citation1 Dietary fiber consists largely of indigestible polysaccharides of plant origin which may be fermented by the colonic microbiota with the production of short chain fatty acids (SCFA), mainly acetate, propionate and butyrate. Of these acids, butyrate has an important role maintaining the homeostasis of the colonic mucosa and is a preferred energy source for colonocytes.Citation2 Its anti-tumorigenic potential has been investigated extensively, particularly its ability to enhance apoptosis in colorectal cancer cell lines in vitro,Citation3 and in the colonic epithelium of rats in response to acute exposure to genotoxin.Citation4 Butyrate has also been reported to promote differentiationCitation5 and to inhibit proliferation of colon cancer cells in vitroCitation6 at physiological concentrations by inhibiting histone deacetylase activity or other pathways involving G-protein coupled receptors.Citation7
Acylated starches have been shown to be an effective means of delivering specific SCFA to the large bowel of humansCitation8,9 and animalsCitation10 and butyrylated high amylose maize starch (HAMSB) has been shown to protect the colonic mucosa of rats from DNA damage induced by high dietary proteinCitation11 and reduce both incidence and number of colonic tumors in the azoxymethane (AOM)-induced rat model of CRC.Citation12 Although butyrate plays a major role regulating apoptosis, differentiation and proliferation in the colonic epithelium,Citation13 some in vitro studies have demonstrated that CRC cells can escape from butyrate-induced apoptosis and become butyrate-resistant.Citation14,15 Human CRC cells, selected in vitro for this ability to grow in the presence of butyrate, produce tumors with more aggressive phenotypes than did unselected cells from the parental CRC cell line when introduced either subcutaneously or orthotopically into a mouse xenograft cancer model.Citation16 However, it remains unclear whether prolonged exposure to elevated concentrations of butyrate selects for resistance to butyrate-induced apoptotic deletion of cells in the colonic mucosa that have undergone acute DNA damage. It has also been suggested that enhanced large bowel fermentation can promote intestinal tumor formation in Apcmin/+ mice.Citation17
The aim of this study was to investigate the dose effects of butyrate delivered by dietary HAMSB on the acute apoptotic and proliferative responses of the distal colonic epithelium to genotoxic challenge with AOM. We have examined the effects of diets containing 0–40% HAMSB on the mucosal responses in relation to the level of colonic epithelial DNA damage as assessed by the COMET assay. In addition, changes to other biomarkers relevant to CRC risk were also examined.
Results
Animal and digesta measures
Final body weights and food intakes were unaffected by the dietary treatment (). Daily water intake increased with level of HAMSB ingestion with HAMSB20 and HAMSB40 rats drinking significantly more than HAMSB0 rats (P < 0.01).
Table 1. Composition of experimental dietsa
Table 2. Final measures of rats fed diets for 28 d containing HAMS and HAMSB and killed 6 hours after injection with AOM
Cecal digesta weight was increased proportionally with dietary HAMSB content and was increased substantially in the HAMSB40 group. This was accompanied by greater cecal tissue weight and lower cecal digesta pH ().
Colonic length increased dose dependently with the level of HAMSB intake and was greater in rats fed HAMSB40 than in those fed HAMSB20. The colons of rats in both of these groups were significantly longer than those in the HAMSB0, HAMSB5 and HAMSB10-fed rats. Distal colonic digesta and faecal pH decreased with dietary HAMSB intake. The small intestine was longer and digesta pH lower for HAMSB40-fed rats compared to all other diets (P < 0.05) ().
Faecal output was higher in proportion to the HAMSB content of the diet () with the output of the HAMSB20 and HAMSB40 rats being significantly higher than all other groups. There were no significant differences in urinary output between dietary groups. The cecal digesta and faecal moisture contents increased with dietary HAMSB intake () with HAMSB40 ingestion resulting in faecal moisture levels higher than any other group and over double the HAMSB0 group. The HAMSB40 diet resulted in intermittent diarrhea in several rats, which was reflected in their greater water intake and faecal moisture content.
Colonic mucus layer thickness
The colonic mucus layer became thicker with increased dietary HAMSB intake (). Correlation analysis showed that cecal butyrate pools were related positively to colonic mucus thickness (; r = 0.48, P < 0.0001).
Table 3. Correlations between selected indices and faecal and colonic parameters
Hepatic portal vein plasma, cecal and faecal SCFA concentrations and pools
Hepatic portal plasma total SCFA, acetate and butyrate concentrations increased with higher intakes of dietary HAMSB (). However, there was no difference in hepatic portal vein plasma propionate concentrations.
Figure 1. Short chain fatty acid levels in (A) hepatic portal vein plasma concentrations (μmol/L) (B) cecal pools (μmoles) (C) cecal concentrations (μmol/L) (D) faecal pools (μmoles). Columns within a graph not sharing the same letter are significantly different P< 0.05, mean±SEM , n = 15/group).
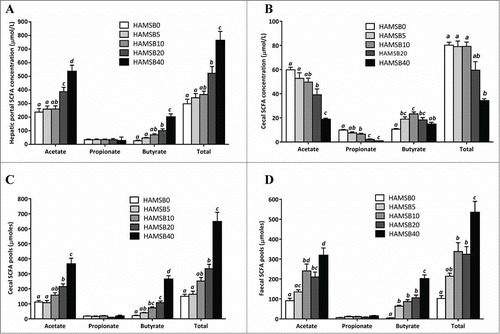
Cecal total SCFA, acetate and propionate concentrations decreased with increasing level of dietary HAMSB intake (). The rats fed HAMSB5–20 had higher cecal butyrate concentrations than HAMSB0-fed rats but were lower with high intakes of dietary HAMSB due to expansion of the digesta mass.
HAMSB10–20 fed rats had higher faecal acetate and total SCFA pools compared to HAMSB0 fed rats, and HAMSB5-20 groups had higher faecal butyrate pools than HAMSB0 rats. The butyrate and total SCFA pools of the HAMSB40 rats were higher than any other group (). There were no differences in faecal propionate pools.
Hepatic portal plasma butyrate concentrations were positively correlated with cecal butyrate pools but not concentrations (r = 0.79, P < 0.0001; r = 0.21, P = 0.07 respectively).
Distal colonic apoptosis and proliferation indices
Distal colonic AI increased with level of dietary HAMSB inclusion (). The AI of the HAMSB40 fed rats was over twice that in the HAMSB0-fed rats (P < 0.001) and correlated positively with hepatic portal acetate and butyrate concentration (r = 0.49, P < 0.0001 and r = 0.65, P < 0.0001 respectively) but not propionate.
Figure 2. Analysis of the distal colon of rats fed HAMSB and killed 6 hours after AOM injection. (A) AI as measured by morphological assessment of haematoxylin stained slides (n = 15/group) (B) Epithelial proliferation as measured by Ki67 immunohistochemistry (n = 15/group) (C) Genetic damage as measured by single-cell gel electrophoresis (comet) assay (n = 10/group). Columns within a graph not sharing the same letter are significantly different (P < 0.05, mean±SEM ).
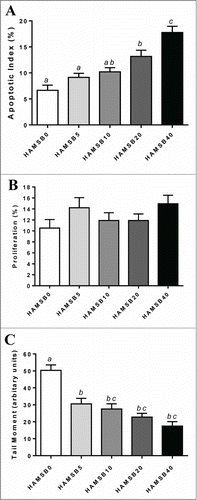
HAMSB increased the rate of apoptosis in a dose-related manner in cells in the distal colon in cellular positions 1 to 20, with the effect being most marked from the base of the crypt (position 1) to around position 15 ().
Figure 3. Crypt cell position of AOM-induced apoptosis measured by morphological assessment of distal colonic epithelium in distal colon of rats fed HAMSB. Data are mean±SEM (n = 15). a,b,cHAMSB0 different to HAMSB10, HAMSB20 and HAMSB40 respectively (P<0 .05).
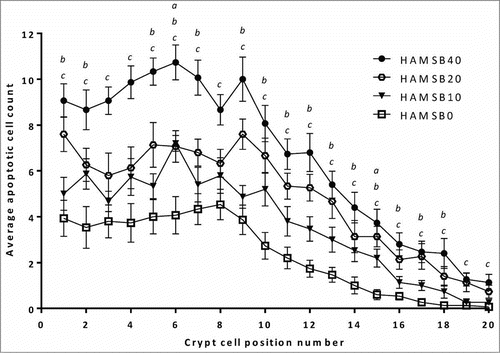
The rate of colonic epithelial proliferation was not influenced by dietary treatment ().
Colonocyte DNA damage
DNA damage (measured as SSB) was greatest when the diet did not contain HAMSB and decreased as the HAMSB content increased (). Colonic DNA damage correlated negatively with hepatic portal butyrate concentration (r = −0.64, P <0 .0001) and cecal butyrate pool (r = −0.66, P = 0.0001) ().
Large bowel ammonia
Cecal ammonia pools were lower in rats fed HAMSB5, and lower still in rats fed HAMSB40 than HAMSB0-fed rats, whereas distal colonic ammonia pools were lower in rats fed HAMSB20 and HAMSB40 than HAMSB0 rats (). Correlation analysis suggested a negative association between AI and cecal ammonia pool (r = −0.56, P < 0 .0001) and a positive association between level of DNA damage and cecal ammonia pool (r = 0.54, P = 0.009) ().
Discussion
This study demonstrates for the first time that elevation of butyrate levels in the large bowel of rodents by increasing dietary HAMSB dose-dependently lowers colonic epithelial DNA damage and increases rates of apoptotic deletion of carcinogen-challenged cells in the distal colon in rats. Importantly these dose-dependent effects are not associated with an increase in cellular proliferation. The relationships between cecal digesta butyrate pools and hepatic portal butyrate concentrations support our previous studies in which elevated large bowel butyrate enhanced apoptosisCitation4 and reduced tumor incidence and numberCitation12 in AOM-treated rats and lowered colonic DNA damage induced by a high protein diet.Citation11 DNA damage is linked closely to risk of carcinogenesisCitation18,19 so increasing the rate of apoptosis of damaged cells is likely to be an important contender for the CRC-protective effect of dietary HAMSB and other factors which increase large bowel butyrate. This present study suggests that at least one mechanism by which butyrate protects against colon carcinogenesis may involve increasing the stringency of apoptotic surveillance by reducing the threshold of DNA damage needed to induce apoptosis in colonocytes.
Butyrate induces apoptosis in cancer cells through various mechanismsCitation7 including alteration of gene transcription via histone deacetylase (HDAC) activityCitation20 and parallel activation of mitochondrial-dependent intrinsic and receptor mediated extrinsic pathways.Citation21,22 Through inhibition of HDAC and alteration of chromatin, butyrate induces expression of target genes that regulate proteins involved in apoptosis as well as cell cycle regulation and DNA repair.Citation6 Butyrate has also been shown to induce apoptosis via activation of G protein coupled receptor 43 (GPR43) which is frequently lost in colon cancer cells.Citation23 The genes encoding the various receptors and transporters involved in butyrate-induced apoptosis are often silenced in CRC cell lines.Citation24,25 For example the butyrate transporter SLC5A8 (SMCT1) gene is commonly silenced epigenetically by aberrant methylation early in human colonic neoplasiaCitation25, but facilitates butyrate-induced cellular apoptosis when re-expressed in the presence of butyrate.Citation26 Together these data suggest that the evasion of butyrate-induced apoptosis may be a key step in colorectal oncogenesis. Situations of extensive DNA damage and compromised repair may result in the accumulation of mutations or silencing of repair and apoptotic genes. Hence a facilitator like butyrate that enhances the deletion of genetically damaged cells would play a key role in maintaining a healthy colonic mucosa.
In the colon, stem cells proliferate at the base of the crypt producing both pluripotent stem cells and daughter cells which differentiate into mature goblet and absorptive cell types as they move from the bottom of the crypt to the lumen (for reviews, see Refs.Citation27–Citation29). The present demonstration that elevated large bowel digesta butyrate levels can dose-dependently promote apoptosis in the base and mid sections of the colonic crypts suggests that deletion of damaged stem and daughter cells may represent a key mechanism by which dietary butyrylated starch brought about a reduced incidence and number of colonic tumors in AOM treated rats.Citation12 Further, this may go some way to explaining how high daily intakes of dietary fiber, particularly fermentable carbohydrates like resistant starch, can reduce the risk of CRC.Citation1
In the current study, elevation of large bowel butyrate levels did not increase the rates of colonocyte proliferation over those observed within the colonic crypts of controls. This contrasts with observations in Apcmin/+mice where the feeding of fermentable fiber increased polyp size and, in morphologically normal regions of the colon, elevated epithelial cell proliferation and crypt fission.Citation17 Coupled with the discovery that human CRC cell lines selected for resistance to the apoptotic effects of butyrate displayed more aggressive tumor phenotypes in a mouse xenograft cancer modelCitation15 than their parental lines, this raised the possibility that prolonged elevation of butyrate levels in the large bowel may lead to loss of sensitivity to butyrate-induced apoptosis by the colonic epithelium when damaged acutely by genotoxins, resulting in greater risk of more numerous and aggressive tumors. However more recent studies have shown physiological concentrations of butyrate have been found to inhibit proliferation of apoptosis-resistant CRC cells, possibly by the activation of the MAPK signaling pathway.Citation6 In addition our previous study investigating the impact of dietary HAMSB on colorectal tumor formation using the AOM rat model showed a reduction of large bowel tumor number and incidence with no impact on tumor size when compared with LAMS-fed control animals.Citation12 Overall we concluded that, at least in situations when butyrate is delivered by acylated starch, the net effect of prolonged exposure to increased large bowel butyrate supply is greater protection against tumor formation.
Acylated starches were designed as a vehicle for the rapid and sustained delivery of butyrate to the colon.Citation10 Acylated HAMS is twice as effective in raising the concentration of the esterified SCFA as the parent starch.Citation30 The present study shows that although cecal butyrate pools increase dose-dependently with HAMSB consumption, cecal butyrate concentrations are lowered at high levels of HAMSB intake due to increased digesta bulk and moisture levels from cecal fermentation of the additional resistant starch. Nevertheless, at high levels of HAMSB intake SCFA concentrations in the hepatic portal vein plasma were closely correlated with cecal SCFA pools rather than concentrations suggesting the former are more accurate indicators of the amount of esterified SCFA in the large bowel available to the host tissues. This observation reflects the fact that butyrate uptake into colonocytes is driven by active mucosal transport mechanisms as well as passive diffusion along a concentration gradient so the butyrate pool is more critical than its concentration.
The genotoxicity of AOM could be affected by differences in digesta mass between the groups. Only a proportion of injected AOM is delivered via the digesta with the remainder being distributed to tissues via the circulatory system.Citation31 However, the relationship between cecal digesta weight and DNA damage appeared to plateau at relatively moderate cecal digesta weights with higher rates of accretion not affecting the level of DNA damage. Dietary sources of nonstarch polysaccharides (such as wheat bran) are most effective at increasing digesta faecal bulk, largely due to the passage of unfermented material, whereas resistant starch (including HAMSB) is generally of high fermentability and has a limited effect.Citation32 Protection from developing CRC is more closely associated with intake of fermentable rather than indigestible carbohydrates.Citation33
Ammonia is a recognized cytotoxic and carcinogenic agent.Citation34,35 Ammonia is in pH dependent equilibrium with its protonated ammonium ion form and ammonia, but not the ammonium ion, is able to passively diffuse into the cells of the colonic mucosaCitation36 and thence on into the circulation, for ultimate excretion via the kidneys. The lowering of the digesta pH resulting from release of butyrate from HAMSB and the fermentation of the resistant carrier starch favors ammonium ion formation, a faecal path for ammonia excretion and hence increased protection of the colonocytes from the toxic effects of this agent.
Increasing dietary HAMSB content was associated with a dose dependent increase in mucus layer thickness in the distal colon of the rats. The colonic mucosa provides a critical barrier to the entry of bacteria and toxins from the lumen into the tissues of the colon and the systemic circulation. The thickness of the mucus layer provides one important measure of gut barrier function.Citation37 The outer, non-adherent, mucus layer is thought to act by reducing shear stress to the mucosa while the inner mucus layer acts as a size exclusion barrier to potentially damaging luminal agents. Our observations here confirm several previous studies demonstrating that butyrate increases the mucin layer covering the colonic epitheliumCitation38 more effectively than other SCFA.Citation39 Our results are also consistent with in vitro studies that have shown butyrate to increase expression of the mucin-encoding gene, Muc2 in cultured cells.Citation40,41 Reduction of MUC2 expression is frequently seen in ulcerative colitis patientsCitation42 and other inflammatory bowel diseases where loss of barrier function is a prominent clinical feature.Citation43 Increased risk of CRC in IBD patients may relate in part to the compromised mucus layer allowing carcinogenic compounds greater access to the colonic mucosal cells. Hence it is likely that another part of the protective effect of HAMSB with respect to DNA damage in the current study and reduction in CRC burden in the long term AOM rat model may result from the enhanced mucus layer.
In conclusion, we have demonstrated that dietary HAMSB provides a dose-related reduction in the level of DNA damage and an increase in the rate of apoptotic deletion of damaged colonic epithelial cells after acute treatment with AOM. Rates of colonic epithelial proliferation were unaffected even at high intakes of HAMSB. Early removal of damaged cells may account, at least in part, for butyrate's observed ability to reduce the risk of AOM-induced CRC in rats. However it remains to be determined whether elevating butyrate concentration in the colon of humans has similar protective effects.
Materials and Methods
Animals and diets
Seventy-five 4 week old male Sprague Dawley rats were purchased from the Animal Resource Center, Murdoch University, Perth, Western Australia. After acclimatisation for one week in the CSIRO animal facility rats were allocated to one of 5 groups (n = 15/group) so that mean body weights were similar across those groups (180 ± 2 g; mean ± SEM). The rats were housed in wire-based cages to minimise coprophagy in rooms maintained at 23 ± 1ºC with a 12 h light/dark cycle. They were given free access to food and water and were weighed weekly throughout the study.
Each group of animals was fed one of 5 diets (). The diets were based on a balanced modification of the AIN-93G dietCitation44 containing α-cellulose as the (non-fermentable) fiber source. The amount of low amylose maize starch (Avon Maize Starch, New Zealand Starch Ltd, Auckland, New Zealand) in the diet was reduced to allow for the addition of 5, 10, 20, 40 % HAMSB. HAMSB was prepared for CSIRO by Ingredion Inc. (Bridgewater, New Jersey) and had a degree of substitution (DS) of 0.23 [i.e. 0.23 of the hydroxyl groups on each starch D-glucopyranosyl unit were derivatized or replaced by the substituent acidCitation45]. The diets were mixed, pelleted, and dried overnight at 45ºC and stored frozen at -20ºC until fed to the rats.
After 14 d of feeding the experimental diets rats (n = 8 per group) were placed in metabolic cages for 3 d to enable the accurate determination of food and water intakes and faecal and urinary outputs. After 28 days of being fed the experimental diets each rat was injected with 15 mg AOM/kg subcutaneously (Sigma Chemical Co., St Louis, MO). Six hours later the rats were anaesthetised with isoflorane (Isoflo, Abbott Animal Health, Abbott Park, IL) and blood collected from the hepatic portal vein and abdominal aorta. The length of the colon was recorded, 0.5 cm discarded from proximal and distal ends, and 1cm sections collected from both distal and proximal ends of the remaining colon. The tissues were fixed in 10% buffered formalin for 24 h, processed and then embedded in paraffin. Cross sections of 4 μm thickness were cut for all histological techniques. The cecum was removed, weighed and known weights of cecal and colonic digesta collected and homogenized in 3 × weight volume of SCFA internal standard solution (1.68 mM heptanoic acid diluted in Milli-Q water adjusted to pH 7) and vortexed for 30 seconds. The pH was measured and then the samples were frozen and stored at −20° C for SCFA analysis. Hepatic portal vein blood was collected into Vacuette tubes containing lithium heparin (Greiner Bio-One, Kremsmuenster, Austria) for plasma analyses. All blood samples were centrifuged at 2000 × g for 15 minutes and aliquots stored at −80°C until analyzed.
All procedures involving animals were approved by the Commonwealth Scientific and Industrial Research Organization (CSIRO) Animal, Food and Health Sciences Animal Ethics Committee and complied with the NHMRC Australian code of practice for the care and use of animals for scientific purposes 7th edition.Citation46
Colonic apoptosis/ haematoxylin
Samples of distal colonic tissue were processed and morphologically assessed for rates of epithelial apoptosis as described previously.Citation4 Briefly, sections (4 μm) were stained with haematoxylin (BDH Laboratory Supplies, UK) and evaluated under a light microscope (BX41, Olympus, Tokyo, Japan) for apoptotic cells. These cells were identified in 20 randomly chosen intact crypts by cell shrinkage, presence of condensed chromatin, and sharply delineated cell borders surrounded by a clear halo as described by Potten et al.Citation47 The apoptotic index (AI) was calculated as the mean number of apoptotic cells divided by the total number of cells in the crypt column multiplied by 100 (represented as percentage). The crypt height and position of apoptotic cells were also recorded. All slides used for assessment of rates of apoptosis and proliferation were encoded randomly and examined by an independent observer who was unaware of the dietary treatments.
Colonic cell proliferation
The rates of distal colonic epithelial proliferation were measured using a modified version of a Ki67 immunohistochemistry method as described previously.Citation4 The present study decreased the dilution of monoclonal antibody (Ki67, DAKO, Glostrup, Denmark) from 1/200 to 1/100 to increase the visibility of stain.
Colonic genotoxicity/Comet assay
A 6-cm segment of colon was removed from each rat at a point 3cm from the distal end of the colon and placed in a solution containing HBSS with 20mM EDTA and 10% DMSO and transferred to −80°C for storage. At the time of analysis the tissue was thawed, the colonic mucosa removed from the luminal surface of the tissue (ie epithelial cells collected by scraping mucosa with a glass microscope slide using even pressure) and the scrapings thoroughly dispersed for 2 minutes using a hand-held homogenizer (Wheaton scientific, Millville NJ, 0.1–0.15 mm gauge). Cells were then passed into a TV-10 tube through a 25 μm nylon filter (Millipore, Billerica, MA) held in a Swinnex filter (Millipore, Billerica, MA) to remove large aggregates of unseparated cells. A single cell suspension was then prepared as described by Thomas.Citation48 These cells were used for the measurement of DNA single-strand breaks (SSB) using the single-cell gel electrophoresis (COMET) assay as described previously using the COMET Assay Kit (Trevigen, Gaithersburg, MD).Citation49 COMET tail moment is the product of tail length and the fraction of DNA in that tail and was calculated for 50 cells from each of 3 slides per rat. The measure was calculated by CometScore Freeware v1.5 (TriTek Corporation, Summerduck, VA) analysis software.
Sampling and analysis procedures
SCFA concentration in faeces and cecal digesta and total ammonia nitrogen concentration in cecal and distal colonic digesta were determined as described previously.Citation30 The concentration of digesta ammonia (NH3) was calculated using the Henderson-Hasselbalch equation and the pKa of ammonia of 9.25. The digesta weights and ammonia concentrations were used to calculate the cecal and distal colonic ammonia pools. The samples were also freeze-dried to determine moisture content.
Colonic mucus layer thickness
A 1-cm segment of colon was collected at 2–3 cm from the distal end of the colon of each rat. The segment was cut open along the anti-mesenteric ridge and the mucosal surface washed gently with 0.15 M NaCl solution to remove digesta. The thickness of mucus lining the distal colon was determined by cutting the tissue into 1.6 mm lengths, illuminating and capturing images of the mucus layer to measure the thickness using an image analysis program.Citation50 For each animal 10 measurements were taken at different points along 4 tissue segments to give 40 thickness measurements in total.
Statistical analysis
All analyses were performed using GraphPad Prism Version 6.00 (GraphPad Software Inc.. San Diego, CA) and all data are expressed as mean ± standard error of the mean (SEM). One-way analysis of variance (ANOVA) with Tukey post-hoc tests (parametric data) and Kruskal-Wallis tests with Dunn's post-hoc test (non-parametric data) were used to compare data between treatment groups. The mean apoptotic cell count at crypt cell positions 1–17 in the distal colon of rats fed HAMSB were compared to LAMS counts using ANOVA with Dunnett's multiple comparison tests for parametric data. For cell positions 18–20 Kruskal-Wallis with Dunn's multiple comparison tests were used to compare the mean rank of each column with the mean rank of LAMS. The correlations between variables were undertaken using the Spearman's nonparametric correlation test for analyses involving AI, hepatic portal plasma and cecal SCFA pool and cecal ammonia pool as these data were non-parametrically distributed. Pearson's correlation tests were used for SSB and mucus thickness as these parameters were normally distributed. Differences were considered significant when P < 0.05.
Disclosure of Potential Conflicts of Interest
No potential conflicts of interest were disclosed.
Acknowledgments
The authors would like to thank Mr Simon Windsor for his technical assistance, Dr Ian Saunders of CSIRO Mathematical and Information Sciences (Urrbrae, South Australia) for his statistical advice. In addition we would like to thank Ingredion Inc. (formerly National Starch Food Chemical Co; Bridgewater, New Jersey) for supplying the HAMSB.
Funding
This work was supported by the Commonwealth Scientific and Industrial Research Organization Preventative Health National Research Flagship (PO Box 10041, Adelaide BC, Adelaide, South Australia 5000).
References
- World Cancer Research Fund. Continuous update project interim report summary: food, nutrition, and physical activity and the prevention of colorectal cancer. Washington, DC: American Institute for Cancer Research, 2011.
- Roediger WE, Heyworth M, Willoughby P, Piris J, Moore A, Truelove SC. Luminal ions and short chain fatty acids as markers of functional activity of the mucosa in ulcerative colitis. J Clin Pathol 1982; 35:323-6; PMID:7068924; http://dx.doi.org/10.1136/jcp.35.3.323
- Hague A, Manning AM, Hanlon KA, Huschtscha LI, Hart D, Paraskeva C. Sodium butyrate induces apoptosis in human colonic tumour cell lines in a p53-independent pathway: implications for the possible role of dietary fibre in the prevention of large-bowel cancer. Int J Cancer 1993; 55:498-505; PMID:8397167; http://dx.doi.org/10.1002/ijc.2910550329
- Clarke JM, Young GP, Topping DL, Bird AR, Cobiac L, Scherer BL, Winkler JG, Lockett TJ. Butyrate delivered by butyrylated starch increases distal colonic epithelial apoptosis in carcinogen-treated rats. Carcinogenesis 2012; 33:197-202; PMID:22080572; http://dx.doi.org/10.1093/carcin/bgr254
- Heerdt BG, Houston MA, Augenlicht LH. Potentiation by specific short-chain fatty acids of differentiation and apoptosis in human colonic carcinoma cell lines. Cancer Res 1994; 54:3288-93; PMID:8205551
- Fung KY, Ooi CC, Lewanowitsch T, Tan S, Tan HT, Lim TK, Lin Q, Williams DB, Lockett TJ, Cosgrove LJ, et al. Identification of potential pathways involved in induction of apoptosis by butyrate and 4-benzoylbutyrate in HT29 colorectal cancer cells. J Proteome Res 2012; 11:6019-29; PMID:23057685
- Bultman SJ. Molecular pathways: gene-environment interactions regulating dietary fiber induction of proliferation and apoptosis via butyrate for cancer prevention. Clin Cancer Res 2014; 20:799-803; PMID:24270685; http://dx.doi.org/10.1158/1078-0432.CCR-13-2483
- Clarke JM, Bird AR, Topping DL, Cobiac L. Excretion of starch and esterified short chain fatty acids by ileostomists after the ingestion of acylated starches. Am J Clin Nutr 2007; 86:1146-51; PMID:17921395
- Clarke JM, Topping DL, Christophersen CT, Bird AR, Lange K, Saunders I, Cobiac L. Butyrate esterified to starch is released in the human gastrointestinal tract. Am J Clin Nutr 2011; 94:1276-83; PMID:21940597; http://dx.doi.org/10.3945/ajcn.111.017228
- Annison G, Illman RJ, Topping DL. Acetylated, propionylated or butyrylated starches raise large bowel short-chain fatty acids preferentially when fed to rats. J Nutr 2003; 133:3523-8; PMID:14608068
- Bajka BH, Clarke JM, Cobiac L, Topping DL. Butyrylated starch protects colonocyte DNA against dietary protein-induced damage in rats. Carcinogenesis 2008; 29:2169-74; PMID:18684730; http://dx.doi.org/10.1093/carcin/bgn173
- Clarke JM, Topping DL, Bird AR, Young GP, Cobiac L. Effects of high amylose maize starch and butyrylated high amylose maize starch on azoxymethane-induced intestinal cancer in rats. Carcinogenesis 2008; 29:2190-4; PMID:18701436; http://dx.doi.org/10.1093/carcin/bgn192
- Cummings JH. Colonic absorption: the importance of short chain fatty acids in man. Scand J Gastroenterol Suppl 1984; 93:89-99; PMID:6374878
- Fung KY, Lewanowitsch T, Henderson ST, Priebe I, Hoffmann P, McColl SR, Lockett T, Head R, Cosgrove LJ. Proteomic analysis of butyrate effects and loss of butyrate sensitivity in HT29 colorectal cancer cells. J Proteome Res 2009; 8:1220-7; PMID:19195990; http://dx.doi.org/10.1021/pr8009929
- Mariadason JM, Velcich A, Wilson AJ, Augenlicht LH, Gibson PR. Resistance to butyrate-induced cell differentiation and apoptosis during spontaneous caco-2 cell differentiation. Gastroenterology 2001; 120:889-99; PMID:11231943; http://dx.doi.org/10.1053/gast.2001.22472
- Serpa J, Caiado F, Carvalho T, Torre C, Goncalves LG, Casalou C, Lamosa P, Rodrigues M, Zhu Z, Lam EW, et al. Butyrate rich colonic microenvironment is a relevant selection factor for metabolically adapted tumour cells. J Biol Chem 2010; 285:39211-23; PMID:20926374; http://dx.doi.org/10.1074/jbc.M110.156026
- Mandir N, Englyst H, Goodlad RA. Resistant carbohydrates stimulate cell proliferation and crypt fission in wild-type mice and in the Apc (Min+) mouse model of intestinal cancer, association with enhanced polyp development. Br J Nutr 2008; 100:711-21; PMID:18279550; http://dx.doi.org/10.1017/S0007114508901276
- Young GP, Hu Y, Le Leu RK, Nyskohus L. Dietary fibre and colorectal cancer: a model for environment–gene interactions. MolNutrFood Res 2005; 49:571-84; PMID:15864783
- Vogelstein B, Kinzler KW. The multistep nature of cancer. Trends in genetics: TIG 1993; 9:138-41; PMID:8516849; http://dx.doi.org/10.1016/0168-9525(93)90209-Z
- Wilson AJ, Chueh AC, Togel L, Corner GA, Ahmed N, Goel S, Byun DS, Nasser S, Houston MA, Jhawer M, et al. Apoptotic sensitivity of colon cancer cells to histone deacetylase inhibitors is mediated by an Sp1/Sp3-activated transcriptional program involving immediate-early gene induction. Cancer Res 2010; 70:609-20; PMID:20068171; http://dx.doi.org/10.1158/0008-5472.CAN-09-2327
- Wang L, Luo HS, Xia H. Sodium butyrate induces human colon carcinoma HT-29 cell apoptosis through a mitochondrial pathway. J Int Med Res 2009; 37:803-11; PMID:19589263; http://dx.doi.org/10.1177/147323000903700323
- Tang Y, Chen Y, Jiang H, Nie D. Short-chain fatty acids induced autophagy serves as an adaptive strategy for retarding mitochondria-mediated apoptotic cell death. Cell Death Differ 2011; 18:602-18; PMID:20930850; http://dx.doi.org/10.1038/cdd.2010.117
- Tang Y, Chen Y, Jiang H, Robbins GT, Nie D. G-protein-coupled receptor for short-chain fatty acids suppresses colon cancer. Int J Cancer 2011; 128:847-56; PMID:20979106; http://dx.doi.org/10.1002/ijc.25638
- Ganapathy V, Thangaraju M, Prasad PD. Nutrient transporters in cancer: relevance to Warburg hypothesis and beyond. Pharmacol Ther 2009; 121:29-40; PMID:18992769; http://dx.doi.org/10.1016/j.pharmthera.2008.09.005
- Li H, Myeroff L, Smiraglia D, Romero MF, Pretlow TP, Kasturi L, Lutterbaugh J, Rerko RM, Casey G, Issa JP, et al. SLC5A8, a sodium transporter, is a tumor suppressor gene silenced by methylation in human colon aberrant crypt foci and cancers. Proc Natl Acad Sci U S A 2003; 100:8412-7; PMID:12829793; http://dx.doi.org/10.1073/pnas.1430846100
- Thangaraju M, Cresci G, Itagaki S, Mellinger J, Browning DD, Berger FG, Prasad PD, Ganapathy V. Sodium-coupled transport of the short chain fatty acid butyrate by SLC5A8 and its relevance to colon cancer. J Gastrointest Surgery 2008; 12:1773-81; PMID:18661192; http://dx.doi.org/10.1007/s11605-008-0573-0
- Marshman E, Booth C, Potten CS. The intestinal epithelial stem cell. Bioessays 2002; 24:91-8; PMID:11782954; http://dx.doi.org/10.1002/bies.10028
- Stange DE, Clevers H. Concise review: the yin and yang of intestinal (cancer) stem cells and their progenitors. Stem Cells 2013; 31:2287-95; PMID:23836510; http://dx.doi.org/10.1002/stem.1475
- Hammoud SS, Cairns BR, Jones DA. Epigenetic regulation of colon cancer and intestinal stem cells. Curr Opin Cell Biol 2013; 25:177-83; PMID:23402869; http://dx.doi.org/10.1016/j.ceb.2013.01.007
- Bajka BH, Topping DL, Cobiac L, Clarke JM. Butyrylated starch is less susceptible to enzymic hydrolysis and increases large-bowel butyrate more than high-amylose maize starch in the rat. Br J Nutr 2006; 96:276-82; PMID:16923221; http://dx.doi.org/10.1079/BJN20061807
- Tan SL, Gerber JP, Cosgrove LJ, Lockett TJ, Clarke JM, Williams DB, Head RJ. Is the tissue persistence of O(6)-methyl-2'-deoxyguanosine an indicator of tumour formation in the gastrointestinal tract? Mutat Res 2011; 721:119-26; PMID:21295156; http://dx.doi.org/10.1016/j.mrgentox.2010.12.016
- Topping DL, Clifton PM. Short-chain fatty acids and human colonic function: roles of resistant starch and nonstarch polysaccharides. PhysiolRev 2001; 81:1031-64; PMID:11427691
- Cassidy A, Bingham SA, Cummings JH. Starch intake and colorectal cancer risk: an international comparison. BrJCancer 1994; 69:937-42; PMID:8180027
- Clinton SK, Bostwick DG, Olson LM, Mangian HJ, Visek WJ. Effects of ammonium acetate and sodium cholate on N-methyl-N'-nitro-N-nitrosoguanidine-induced colon carcinogenesis of rats. Cancer Res 1988; 48:3035-9; PMID:3365693
- Lin HC, Visek WJ. Colon mucosal cell damage by ammonia in rats. J Nutr 1991; 121:887-93; PMID:2033472
- Cohen RM, Stephenson RL, Feldman GM. Bicarbonate secretion modulates ammonium absorption in rat distal colon in vivo. Am J Physiol 1988; 254:F657-F67; PMID:3129949
- Brownlee IA, Havler ME, Dettmar PW, Adrian A, Pearson JP. Colonic mucus: secretion and turnover in relation to dietary fibre intake. Proc Nutr Soc 2003; 62:245-9; PMID:12756974; http://dx.doi.org/10.1079/PNS2003206
- Finnie IA, Dwarakanath AD, Taylor BA, Rhodes JM. Colonic mucin synthesis is increased by sodium butyrate. Gut 1995; 36:93-9; PMID:7890244; http://dx.doi.org/10.1136/gut.36.1.93
- Shimotoyodome A, Meguro S, Hase T, Tokimitsu I, Sakata T. Short chain fatty acids but not lactate or succinate stimulate mucus release in the rat colon. Comp Biochem Physiol A Mol Integr Physiol 2000; 125:525-31; PMID:10840229
- Gaudier E, Jarry A, Blottiere HM, de Coppet P, Buisine MP, Aubert J, Laboisse C, Cherbut C, Hoebler C. Butyrate specifically modulates MUC gene expression in intestinal epithelial goblet cells deprived of glucose. Am J Physiol Gastrointest Liver Physiol 2004; 287:G1168-74; PMID:15308471
- Hatayama H, Washita J, Kuwajima A, Abe T. The short chain fatty acid, butyrate, stimulates MUC2 mucin production in the human colon cancer cell line, LS174T. Biochem Biophys Res Commun 2007; 356:599-603; PMID:17374366; http://dx.doi.org/10.1016/j.bbrc.2007.03.025
- Einerhand AW, Renes IB, Makkink MK, Van der Sluis M, Buller HA, Dekker J. Role of mucins in inflammatory bowel disease: important lessons from experimental models. Eur J Gastroenterol Hepatol 2002; 14:757-65; PMID:12169985; http://dx.doi.org/10.1097/00042737-200207000-00008
- Jowett SL, Seal CJ, Pearce MS, Phillips E, Gregory W, Barton JR, Welfare MR. Influence of dietary factors on the clinical course of ulcerative colitis: a prospective cohort study. Gut 2004; 53:1479-84; PMID:15361498; http://dx.doi.org/10.1136/gut.2003.024828
- Reeves PG, Nielsen FH, Fahey GC, Jr. AIN-93 purified diets for laboratory rodents: final report of the American Institute of Nutrition ad hoc writing committee on the reformulation of the AIN-76A rodent diet. J Nutr 1993; 123:1939-51; PMID:8229312
- Thomas DJ, Atwell WA. Starches: Practical guides for the food industry. St. Paul, MN: Eagan Press, 1999.
- National Health and Medical Research Council. Australian code of practice for the care and use of animals for scientific purposes. 7th edition. Available from http://www.nhmrc.gov.au/publications/synopses/ea16syn.htm
- Potten CS, Li YQ, Oconnor PJ, Winton DJ. A possible explanation for the differential cancer incidence in the intestine, based on distribution of the cytotoxic effects of carcinogens in the murine large bowel. Carcinogenesis 1992; 13:2305-12; PMID:1473238; http://dx.doi.org/10.1093/carcin/13.12.2305
- Thomas P, Harvey S, Gruner T, Fenech M. The buccal cytome and micronucleus frequency is substantially altered in Down's syndrome and normal ageing compared to young healthy controls. Mutat Res 2008; 638:37-47; PMID:17920640; http://dx.doi.org/10.1016/j.mrfmmm.2007.08.012
- Olive PL, Banath JP. The comet assay: a method to measure DNA damage in individual cells. Nat Protoc 2006; 1:23-9; PMID:17406208; http://dx.doi.org/10.1038/nprot.2006.5
- Toden S, Bird AR, Topping DL, Conlon MA. Resistant starch attenuates colonic DNA damage induced by higher dietary protein in rats. Nutr Cancer 2005; 51:45-51; PMID:15749629; http://dx.doi.org/10.1207/s15327914nc5101_7