Abstract
The chemical nature of genetic drugs (e.g. antisense oligonucleotides, siRNA, vectors) requires a suitable carrier system to protect them from enzymatic degradation without changing their properties and enable efficient delivery into target cells. Lipid vectors for nucleic acid delivery that have been widely investigated for years can be very effective. As the majority of attempts made in the field of cancer gene therapy have focused on solid tumors, while blood cancer cells have attracted less attention, the latter became the subject of our investigation. The lipid carrier proposed here is based on liposomes constructed by others but the lipid composition is original. A liposome-coated lipoplex (L-cL) consists of a core arising from complexation of positively charged lipid and negatively charged oligodeoxynucleotide (ODN) or plasmid DNA coated by a neutral or anionic lipid bilayer. Moreover, our lipid vector demonstrates size stability and is able to retain a high content of enclosed plasmid DNA or antisense oligodeoxynucleotides (asODNs). Observed transfection efficacies of the tested preparation using a plasmid coding for fluorescent protein were up to 60-85% of examined leukemia cells (Jurkat T and HL-60 lines) in the absence or the presence of serum. When BCL‑2 asODN was encapsulated in the L-cL, specific silencing of this gene product at both the mRNA and protein level and also a markedly decreased cell survival rate were observed in vitro. Moreover, biodistribution analysis in mice indicates prolonged circulation characteristic for PEG-modified liposomal carriers. Experiments on tumor-engrafted animals indicate substantial inhibition of tumor growth.
Abbreviations
asODN | = | antisense oligodeoxynucleotide |
AML | = | acute myeloid leukemia |
Bcl-2 | = | B-cell lymphoma 2 protein |
CCL | = | coated cationic liposomes |
L-cL | = | liposome-coated lipoplex |
DC-CHOL | = | 3β-(N-[dimethylaminoethane]carbamoyl)cholesterol) DiD-1,1′, dioctadecyl-3,3,3′, 3′-tetramethylindodicarbocyanine |
DOPE | = | 1,2-dioleoyl-sn-glycero-3-phosphoethanolamine) |
DSPE | = | PEG-(1,2-distearoyl-sn-glycero-3-phosphoethanolamine-N-[amino(polyethylene glycol)2000] (ammonium salt) |
DOTAP | = | 1, 2-dioleoyl-3-trimethylammonium-propane) |
GFP | = | green fluorescent protein |
HPC | = | hydrogenated egg phosphatidylcholine) |
pDNA | = | plasmid DNA |
PE/PC | = | phosphatidylethanolamine and phosphatidylcholine liposomes |
siRNA | = | small interferingRNA TGI, tumor growth inhibition |
Introduction
Gene therapy is a promising strategy for the prevention and treatment of many diseases. The most effective carriers for genetic drugs seem to be viral vectors. Despite their high transfection efficiency, viral vectors have serious drawbacks since immunogenicity, insertional mutation or activation of certain oncogenes cannot be excluded.Citation1 Non-viral lipid carriers have been developed within recent years as an alternative to viral type gene vectors. They are mostly cationic lipid–based gene delivery systems which were developed as tools for gene delivery e.g.,Citation2,3 (for a review see ref.Citation4). Although they proved efficient in vitro, still many problems remain to be solved before using them as drug carriers. A gene transfer vector should be safe, stable, cost-effective to manufacture in clinically relevant quantities, and capable of efficient and tissue-specific delivery. Properly designed lipid-based carriers are able to fulfill all these conditions. Although there are a number of available promising results of in vitro tests, many obstacles must be overcome in order to improve selective and effective gene delivery into target cells and tissues. These include genetic drug protection against enzymatic degradation, biocompatibility of lipid carriers, specific delivery of the genetic drug–lipid carrier complex to target cells and tissues, and satisfactory pharmacokinetics in vivo. The main challenge for gene therapy as a strategy in therapeutic treatments is to obtain high transfection efficiency of targeted cells and to reduce side-effects such as toxicity for non-target cells and tissues.Citation5,6
The physicochemical nature of the genetic drugs and lipid compounds of the carrier, as well as the interactions between these molecules, is crucial for constructing a suitable and effective carrier system for gene delivery. The physicochemical profile of the synthetic non-viral vector determines its fate in biological fluids and after contact with a target cell (uptake and intracellular trafficking) and is important for improving the transfection efficiency. Recently, the efforts of many research groups have focused on development of new genetic drugs in order to improve their effectiveness and specificity, and to provide the possibility of full control of their action. Molecules such as antisense oligonucleotidesCitation7 or small inhibitory RNAs can be used to inhibit particular gene expression as sole therapeutics or in combination with other therapeutic approaches, to enhance the effectiveness of non-gene therapy (e.g., with cytostatic agents and/or radiation treatment for cancer diseasesCitation8).
As nucleic acid molecules are rather poorly internalized by cells, various carriers have been developed, of which the viral-derived vectors proved the most effective although the least safe.Citation1 The most promising results were obtained in transfection experiments using various cationic lipid supramolecular aggregates (for a review see refs.Citation4,9). These aggregates containing various cationic lipids exhibit a diversity of transfection efficacy, immunogenicity, toxicity and stability even in the presence of serum. The therapeutic agents in gene therapy can be plasmid DNA, antisense oligonucleotides or siRNA, which are not able to freely diffuse far from the injection site or to cross barriers such as endothelium or the blood-brain barrier, as they possess a high positive charge. Moreover, the net positive charge of these particles promotes opsonization and clearance from the circulation by the macrophage systems (for a review see ref.Citation5). Encapsulation of cationic lipid-nucleic acid complexes into the anionic liposomes was suggested.Citation10,11
In this report we present a lipid-based construction which uses the concept of coated cationic liposomes (CCL) of Stuart et al.Citation12 The lipid composition used in the construction of such liposomes allowed us to obtain a nucleic acid carrier characterized by high transfection efficacy toward cells of lymphoid and myeloid origin, low toxicity when not containing antisense asODN, lack of hemolytic activity, stability in the presence of serum, and stability in terms of particle size during long-term storage. As a model target gene, BCL-2 gene expression was chosen for silencing, as this gene was found overexpressedCitation13,14 in most of the leukemic cell types (for a review see refs.Citation15-18).
In this study we found that antisense ODNs against the gene encoding the B-cell lymphoma 2 protein (Bcl-2) encapsulated into a liposomal envelope named L-cL could be used as genotype-specific drugs that delay tumor growth in vivo.
Results
The lipid carrier proposed here is based on L-cL (Liposome-coated Lipoplex), first constructed by others,Citation12 but the lipid composition is original. L-cL consists of a core arising from complexation of positively charged lipid and negatively charged ODN or plasmid DNA coated by a neutral or anionic lipid bilayer. The core of the liposome was an asODN or plasmid DNA complex with DOTAP (1:20 w/w) while the bilayer coat was formed of HPC, DC-CHOL, DOPE, DSPE-PEG, in a ratio of 0.5:0.2:0.1:0.2 (w/w). The ratio of core DOTAP to the bilayer HPC was set to 0.7.
Lipid carrier characterization
The diameter of obtained liposomes L-cL was 116.3 ± 7.8 nm and their ζ-potential was 6.6 ± 0.9 mV (, respectively). The amount of encapsulated pDNA was in the order of 120 μg/ml (about 80–95% of DNA used for liposome preparation from 11.3 mg of lipid was entrapped inside the carrier). When stored as a suspension for up to 12 months their diameter, distribution, and ζ-potential were stable. The plasmid DNA or ODN content was also fairly stable, changing up to 25% during 6 months ().
Figure 1. Stability of L-cL liposomes upon storage as a suspension at 4°C during 12 months. Changes in liposome diameter (A) and ζ-potential (B), pDNA (C) or ODN (D) content. For further details see Materials and Methods section.
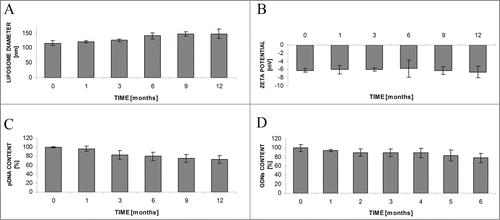
Another standard way to store the liposome preparations is to freeze-dry the suspension in the presence of a cryoprotectant (here sucrose at a ratio to the lipid of 5:1 (w/w) was used). In the changes of size, ζ-potential and pDNA or ODN content after rehydration during long-term storage (90 days) at −20°C are presented. Again, the stability of parameters seems acceptable.
Figure 2. Stability of liposomes upon storage as freeze-dried powder and subsequent rehydration. Changes in liposome diameter (A) and ζ-potential (B), pDNA (C) or ODN (D) content. For further details see Materials and Methods section.
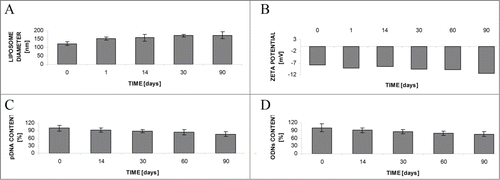
As mentioned above, one of the major criteria is the resistance of the liposomal preparation in the presence of serum. The obtained L-cL suspension was incubated for up to 30 h in the presence of 50% human serum. Next the liposomal fraction was separated from proteins on a Sepharose 4B microcolumn and the diameter () and ζ-potential (3B) measurements were performed. As shown in , prolonged (30 h) incubation of these liposomes at 37°C in the presence of human serum does not induce changes in the basic properties of L-cL liposomes.
Figure 3. Stability of L-cL liposomes upon incubation at 37°C in the presence of 50% human serum. Changes in liposome diameter (A) and ζ-potential (B) during 30 h incubation in the presence of 50% human serum at 37°C. Further details as in legend and Materials and Methods section.
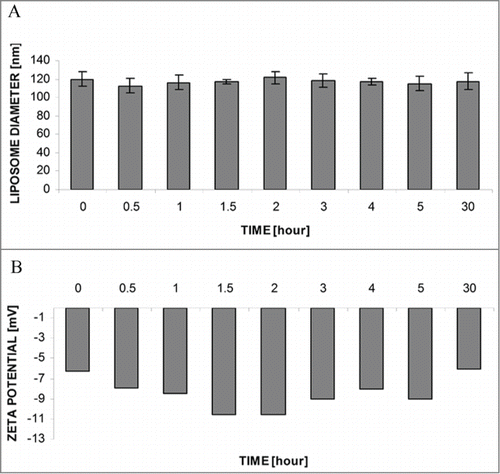
To test the ability of the construct to protect the encapsulated pDNA against soluble DNases and DNases present in human serum, electrophoretic analysis of the pDNA was performed after incubation with DNase I or human serum. The results are shown in . The data shown there suggest that after 10 minutes incubation of the encapsulated DNA is preserved from soluble DNase activity present outside liposomes. The same protective properties were confirmed after 8 h of incubation in 50% human serum ( right).
Figure 4. Effect of soluble DNase (left) or human serum (right) on pDNA encapsulated in L-cL liposomes. The agarose gel electrophoresis analysis of protective properties of L-cL liposomes toward encapsulated genetic drug (pDNA). NC – negative control (liposomes + 10% Triton X-100), PC – positive control (liposomes + 10% Triton X-100 + DNase I or 50% human serum), Ex – experimental sample (liposomes + DNase or 50% human serum-incubation 8 h + 10% Triton X-100).
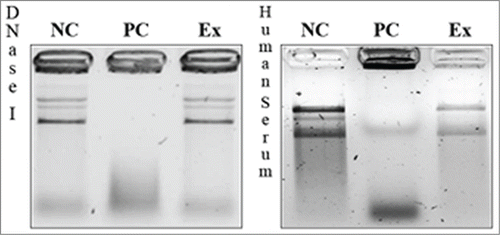
Toxicity
The obtained carrier essentially did not show hemolytic activity when tested on freshly prepared human erythrocytes. The maximal obtained values of hemolysis at the concentrations of L-cL used here were close to 5% ().
Figure 5. Hemolytic activity of L-cL liposomes against human red blood cells. Hemolytic activity was determined based the hemoglobin concentration released from 0.38% erythrocyte suspension in the presence of indicated concentrations of liposomes (lipid) after 30 min incubation at 37°C. Other details in Materials and Methods.
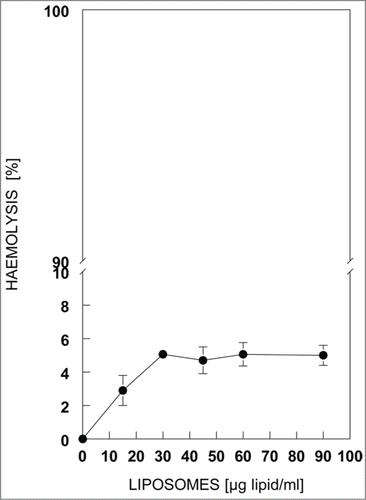
The observations based on the Trypan Blue exclusion test indicate that the proposed lipid carrier is essentially nontoxic for cells of the tested cell lines, such as Jurkat T and HL-60 in PBS, culture medium or serum-supplemented culture medium for 48 h. Some toxicity could be observed, however, for the highest doses ().
Figure 6. The effect of “neutral”(pDNA containing) L-cL liposomes on the survival rate of cells of several cell lines. Cell survival was determined by Trypan Blue exclusion test 72 h after addition of indicated concentration of liposome (lipid) suspension. The experiment was carried out in the presence of PBS buffer (A), or serum-free culture medium (B), or culture medium supplemented with 10% fetal bovine serum(C). Error bar = s.d.
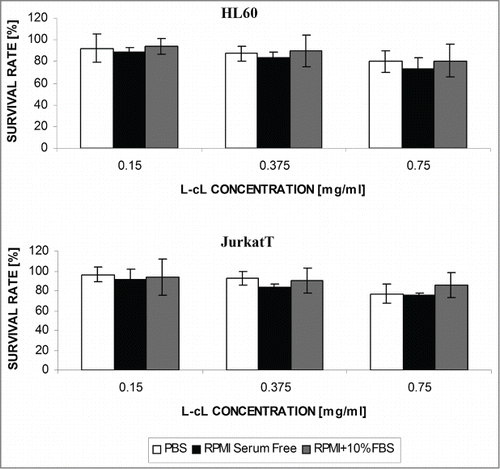
Functional properties of L-cL
As the basic physiochemical properties of the L-cL liposomes were acceptable, their functional properties were tested.
Transfection efficacy
Transfection efficiencies of the L-cL were assessed by encapsulating pDNA encoding GFP. The number of cells expressing fluorescent proteins were compared to the total number of cells. Transfection efficacy of L-cL toward 2 cell lines after 24 or 48 h varied from ∼25 up to even 85% for both cell lines depending on the concentration of the L-cL carrying pDNA and the medium in which the transfection was carried out (data not shown). contains data for HL60 and Jurkat T cells transfected for 24 and 48 h with various doses of L-cL in the culture medium supplemented with FBS. As can be seen, in these conditions (superior to those in the absence of serum or to those in the presence of PBS) the transfection efficacy is rather high, reaching more than 80% in the highest doses of L-cL used.
Table 1. Transfection efficacy of HL60 and Jurkat T cells in culture with coated cationic liposome carrier L-cL containing indicated concentration of pDNA encoding pGFP
Silencing of BCL-2 expression with BCL-2 directed antisense nucleotides (ODN)
Overexpression of the BCL-2 gene is a characteristic feature of most leukemia cells; therefore the effect of transfection of the cells with L-cL-encapsulated antisense ODN was tested. In the results of reverse transcriptase PCR (for Jurkat T) and in and C Western blot analysis of Jurkat T and Daudi cells treated with L-cL for up to 48 h are shown. As can be seen, the mRNA signal decays within 48 h after treatment with asODN-containing L-cL what results in a marked decrease of the Bcl-2 protein. This may suggest that these ODNs affect strongly protein synthesis. The fact that the effect on Bcl-2 protein level is qualitatively easily visible may again suggest that the transfection efficacy was high. Also, reasonable decrease of the Bcl-2 protein level can be observed in the case of Daudi cells (). It should be noted that “naked” asODN at the concentrations applied here remained without any effect on the BCL-2 expression in these cells (data not shown), as was the case for the L-Cl construct containing “scrambled” ODN ().
Figure 7. Treatment of Jurkat T and Daudi cells in culture with L-cL encapsulated as ODN directed against human BCL-2 gene. (A). Reverse transcriptase PCR analysis for Jurkat T cells treated with 10 μg/ml ODNs in L-cL for indicated time. The product of amplification on β-actin primers was electrophoresed accordingly to ensure that the quantitative differences were not due to the amount of cDNA loaded. (B and C). Western blot analysis with monoclonal mouse anti-human Bcl-2 antibodies and with polyclonal goat anti-human Actin antibodies followed by peroxidase-conjugated goat anti-mouse and donkey anti-goat IgG of Jurkat T and Daudi total cell lysate after 48 h treatment with indicated concentrations (μg/ml) of ODNs in L-cL. Blots were developed by chemiluminescent reaction. Lines C – cells not treated with asODN, line C' cells treated with scrambled ODN encapsulated in L-cL.
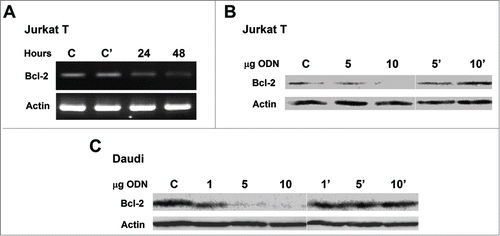
Transfected cell survival
To test whether silencing of the BCL-2 gene has an effect on cell survival, the experiment in which cultured cells were treated for up to 24 h with increasing concentrations of BCL-2 directed antisense ODN encapsulated in L-cL was performed. The results are shown in . They indicate that after 24 h of treatment with 2.5 μg/ml only ∼40% of Jurkat T and ∼50% of HL60 cells (), and about 59% in the case of Daudi cells (), survived. Data presented in this figure indicate that the effect is dose dependent. It should be noted that rather low toxicity of the proposed carrier has been observed. As shown in , the toxic effect of liposomes 750 μg/ml containing ∼10 μg/ml of neutral DNA did not exceed 20% when incubated as long as 72 h.
Figure 8. Survival of chosen line cells upon treatment with L-cL encapsulated antisense ODNs directed against BCL-2 gene. A. Jurkat T and HL60 assessed by Trypan Blue exclusion test. Abbreviations: HL-6, HL-12, HL-24, HL60 cells cultured for 6, 12 and 24 h with L-cL containing BCL-2 directed asODNs. JT= Jurkat T cells cultured with the same liposomes for 6,12 and 24 h. B. Daudi cells assessed by MTT assay.
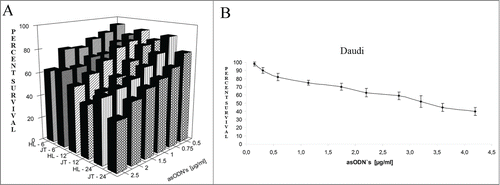
When the AML patient's white blood cells were treated in vitro, in culture conditions with up to 2.5 μg/ml of antisense ODNs in L-cL liposomes, 40–50% of cells survived after 24–48 h while ∼25% survived after 72 h following transfection. The percentage survival was proportional to the construct concentration ().
Figure 9. Survival of cultured AML-patient cells upon treatment with L-cL encapsulated antisense ODNs directed against BCL-2 gene assessed by Trypan Blue exclusion test. Cells were treated with L-cL liposomes at concentrations corresponding to the indicated concentrations of asODNs. The bars correspond to the time after which the test was carried out (legend on the right-side). Error bars = ± s.d. Significant differences from the control (untreated) culture values are marked with asterisks.
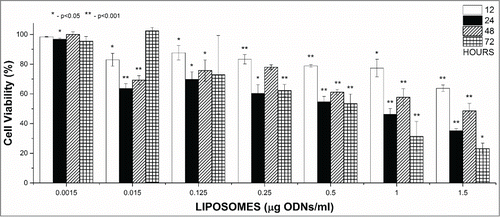
Biodistribution studies
In vivo imaging
Liposomes (L-cL) which were fluorescently labeled with DiD were injected in a dose of 50 mg of lipids/0.8 mg of asODNs/kg of body weight via the tail vein of mice. Liposome distribution was analyzed in vivo using the NightOwl II LB 983 apparatus (Berthold). At the indicated time mice were anesthetized with isoflurane and the fluorescence of labeled liposomes was analyzed.
Representative images taken at 2 and 8 h after liposome injection are shown in . PEG-modified L-cL liposomes were easily detectable in the body of the experimental animal at least for 8 h, in contrast to the control PE/PC liposomes, which became undetectable in the mouse body using this method within the first 2 h.
Figure 10. Circulation time and biodistribution of L-cL in experimental animal. A. In vivo imaging in BALB/c mice DiD labeled PE/PC and L-cL liposomes. L-cL remained in mice even 8 h after injection. Control liposomes (PE/PC) were cleared as fast as 2 h after administration. For details see Materials and methods. B. The prolonged circulation of L-cL liposome in a mouse's bloodstream. L-cL liposomes have remained in blood even 24 h after administration. The fluorescence intensity of DiD-labeled liposomes in serum was measured as described in Materials and methods. C. and D. Organ distribution of L-cL and control PE/PC liposomes in liver and spleen of experimental animal. The dependence of fluorescence intensity of L-cL liposomes accumulated in liver and spleen on time. Fluorescence intensity was measured as cts/pix*s, which means the integrated number of photons per 1 pixel of matrix per second.
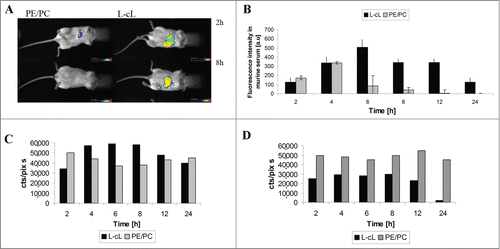
Blood circulation time
Mice were injected with DiD-labeled liposomes as described above. The blood samples were collected via the retro-orbital sinus at time intervals from 2 to 24 h after administration. The fluorescence intensity of liposomes circulating in blood was measured in a serum fraction by using a spectrofluorimeter. PEG-modified L-cL liposomes reached the highest concentration at 6–8 h and a high concentration (>60% maximal) was detected in circulating blood up to 12 h. Between 12 and 24 h after injection seems to be the approximate half time (τ1/2). After 24 h following carrier injection still ∼25% of fluorescence was detectable. The elimination of PE/PC liposomes was much faster, reaching maximum at 4 h and a half-time between 4 and 6 h following injection. After 12 h the fluorescence of PE/PC liposomes was essentially undetectable ().
Organ distribution
Accumulation of control and L-cL liposomes in the liver and spleen (in other organs, such as lung, kidney and brain, the fluorescence intensity of liposomes was very low – data not shown). As shown in , both liposomal formulations, control (PE/PC) and L-cL, were accumulated in these organs. The fluorescence intensity of labeled L-cL liposomes in the liver decreased 12 h after injection, and became comparable with the fluorescence intensity of control non-pegylated PE/PC liposomes. L-cL liposomes are probably trapped by the liver, especially 4–8 h after liposome injection, then a decrease, steeper in the case of L-cL in the spleen than in the liver, could be observed.
Therapeutic efficacy of liposomes containing asODN against BCL-2
The aim of this experiment was to determine the antitumor activity of our liposome formulation in the test model of the human Daudi Burkitt's lymphoma implanted subcutaneously into NOD/SCID mice. The preparations were administered intravenously as specified in the Materials and methods section. In the results of the measurements of the tumor volume during the time of the experiment are shown. L-cL (encapsulated asODNs) and L-cLsc (encapsulated scrambled sequence), both as monotherapy, were well tolerated, with minimal (<5%) loss in mean body weight (data not shown), and no significant clinical symptoms were observed. Antitumor effects were expressed as % tumor growth inhibition (TGI), dividing the tumor volumes from treatment groups with the control group (PBS). L-cL (liposomes carrying antisense oligonucleotides directed against BCL-2) and L-cLsc (liposomes carrying oligonucleotides of a scrambled sequence) therapy resulted in TGI values of 57% and 0.33% respectively on day 30 of treatment in mice receiving 50 mg/kg (). The highest tumor growth inhibition activity of L-cL formulation was observed on day 21 (TGI = 60.38%), and the activity of this formulation remained at 55%–60% until the end of the experiment ().
Table 2. Inhibition of tumor growth (%TGI) determined for L-cLsc and L-cL liposomes given at a dose of 50 mg/kg in the Daudi murine leukemia model during 30-day treatment. For details see Materials and methods section
Figure 11. The kinetics of tumor growth. Mice bearing tumor xenografts were treated with L-cL liposomes containing antisense oligonucleotides against the BCL-2 gene (L-cL asODN) or with control liposomes containing scrambled sequence oligonucleotides L-cL scODN, or PBS only. For further details see Materials and methods section.
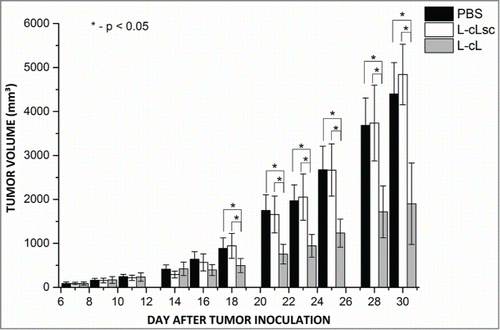
As can be seen, a substantial inhibitory effect of tumor growth was observed for the group treated with asODN-containing L-cL. The statistical analysis (Kruskal-Wallis ANOVA) showed that differences in tumor volumes between the group treated with asODN L-cL and control, scODN L-cL and PBS groups were statistically significant, indicating the possibility of use this construct to delay tumor growth.
In summary, results obtained from our experiments indicate that the proposed lipid carrier is stable for at least 12 months during storage as a suspension at 4°C, and maintains its properties for 3 months during storage in lyophilized form at −20°C. Moreover, our construct maintained a relatively high level of encapsulated pDNA, protected it from external DNases and appeared essentially nontoxic against erythrocytes or cells in the culture while not encapsulating specific ODN. The L-cL construct was stable in the presence of 50% human serum and showed high transfection efficiencies against human leukemia cells. Moreover, when containing specific asODNs it was shown to effectively silence target (BCL-2) gene expression at the mRNA as well as protein level. Finally, the construct presented here was shown to effectively inhibit target cell growth in vitro and moreover it markedly inhibited engrafted tumor growth in experimental animals.
Discussion
Antisense therapy is a promising strategy for the treatment of many neoplastic diseases. The target of our study is blood cancer cells. Controlled delivery of nucleic acids (plasmid DNA, ODN and siRNA) to the target cells remains a challenge. Lipofection is one of the main strategies among non-viral delivery tools. We have chosen several leukemia and lymphoma cell lines, among them Jurkat T, known to be particularly difficult to use for transfection. As the starting point we chose the procedure of Stuart et al.Citation10,12 to test the possibility of application of coated cationic liposomes for this purpose. There is a separate branch of literature concerning attempts to silence BCL-2 or other apoptosis-inhibiting proteins encoding gene expressionCitation19-22 in cancer cells.
The lipid carrier composition which was designed here was characterized by small size and good long-term (several months) stability in a suspension as well as in the form of freeze-dried powder. It was also stable for up to 30 h incubated in the presence of 50% human serum. Moreover, it efficiently condensed and encapsulated pDNA and asODN. It also protected test preparations of pDNA from breakdown catalyzed by external nucleases. The L-cL preparation did not induce substantial lysis of human erythrocytes and was not substantially toxic to the cultured cells of the cell lines studied here when irrelevant nucleic acids (plasmid DNA or scODNs) were used.
By using this vector it was possible to transfect the mentioned cell lines with high effectiveness reaching ∼80% at the most optimal conditions (presence of serum supplemented culture medium). This high level of transfection was also observed in the case of the same cells transfected with pDNA encoding siRNAs for other genes (Grzybek et al. unpublished observations). It seems that this construct may prove a useful tool for in vitro transfection of cells difficult to transfect by other means.
Moreover, when BCL-2 gene directed asODNs were encapsulated, efficient silencing of this gene could be observed at either the mRNA or the protein level what was tested in the case of Jurkat T cells (). This confirms the high efficacy of transfection which was also visible as marked decrease of Bcl-2 protein level in Daudi cells 48 h following treatment with L-cL (). It should be noted that control experiments in which “naked” ODNs or those of a “scrambled” sequence were used showed no decrease in BCL-2 expression at either mRNA or protein level. Silencing of the BCL-2 gene was accompanied by substantial toxicity of the proposed formulation to the target cells (overexpressing the BCL-2 gene) in culture. Also, myeloid cells from the AML patient's blood were sensitive to the treatment with L-cL-encapsulated BCL-2 directed ODN (). The above-presented properties of the obtained nanocarrier made a good starting point for the animal studies. As shown in , the biodistribution data indicated the properties characteristic for PEG-modified liposomal carriers, i.e. prolonged presence in blood and lower accumulation in liver and spleen than PE/PC liposomes (). The carrier presented here, L-cL, was easily detectable in blood as long as 24 h following injection. Even though both PE/PC and L-cL liposomes accumulated in liver and spleen at relatively high levels, the L-cL liposome concentration in the blood was maintained high for 12 h while the PE/PC liposome level in the blood was maintained at a similar level for only 4 h ().
The results of the experiment on engrafted tumor treatment with the L-cL asODN construct indicate that it is able to inhibit tumor growth by at least 50% and cause marked delay in its development. The data from tumor size measurements are generally consistent and statistically significant. Moreover, the obtained results show that the L-cL formulation exhibits significantly higher antitumor activity compared to the control formulation (TGI of the L-cL carrying scrambled ODNs during 30-day treatment was 4–170 times lower than observed for the L-cL carrying ODNs against BCL-2, administered in the same formulation and concentration).
This in our opinion is reasonable inhibition, particularly taking into consideration the fact that it was the sole treatment. Antisense nucleotide treatment does not preclude application of other therapies, such as cytostatics. In this case the effect should be even greater, as BCL-2 is an antiapoptotic gene.
Materials and Methods
Animals. All experimental procedures were approved by the Local Ethics Committee in Wroclaw. Adult, female 5–7-week-old BALB/c mice and male, 5–7-week-old NOD/SCID mice were used.
Cells. Daudi, HL60, K562 and Jurkat T cell lines were from the Institute of Immunology and Experimental Therapy, Polish Academy of Sciences at Wrocław. Primary AML cells were from one patient of the Haematology Clinic, Medical University at Wrocław; the blood sample was used according to permission no. KB -542/2011 of the Ethics Committee at the Medical University of Wrocław. Mononuclear cells were isolated as previously described.Citation23
Lipids: DOTAP (1,2-dioleoyl-3-trimethylammonium-propane), DOPE (1,2-dioleoyl-sn-glycero-3-phosphoethanolamine), HPC (hydrogenated egg phosphatidylcholine), DSPE-PEG (1,2-distearoyl-sn-glycero-3-phosphoethanolamine-N-[amino(polyethylene glycol)2000] (ammonium salt) and DC-CHOL (3β-(N-[dimethylaminoethane]carbamoyl)cholesterol) were either from Avanti Polar Lipids, Alabaster USA, or Northern Lipids, Vancouver, Canada. PCR primers and asODNs (based onCitation24-27), were synthesized by Oligo.pl, and the pEGFP plasmid was from Clontech.
Lipid carrier preparation
Lipid carrier preparation was based on the procedure described by others where asODNs or plasmid DNA is extracted into organic (chloroform) phase using cationic lipids. Briefly, plasmid DNA or ODN aqueous solution (100–150 μg in 150 μl) was mixed with a chloroform solution of DOTAP (3 mg in 150 μl). The next step was titration with methanol until a single phase arose. Subsequent addition of chloroform (600 μl) and water (600 μl) restores 2 phases. After short centrifugation (7 min, 800 x g) the upper phase was discarded. To the lower (chloroform) phase containing approximately 90% DNA or ODNCitation28 a chloroform solution of a mixture of coating lipids (4.3 mg HPC, 0.6 mg DC-CHOL, 1.5 mg DOPE, 1.7 mg DSPE-PEG) and 250 μl of PBS (5 mM NaH2PO4, 5 mM Na2HPO4, 2 mM KCl, 150 mM NaCl, pH 7.4) were added. The resulting suspension was sonicated (1 min, 3 W, 0°C, in a Microson sonifier) and organic solvents were removed by evaporation first under gaseous N2 followed by evaporation in a rotary evaporator. The obtained liposome suspension after dilution with PBS to the final volume of 1 ml was extruded at least 10 times first through 400, next 200 and finally through 100-nm Nuclepore filters (Whatman). The last 10 cycles of extrusion through a 100 nm Nuclepore filter was performed in one direction only to ensure sterility of the final preparation. The sterility of the preparation was also tested by plating of 10 μl of the final preparation onto a small agar plate containing L-B medium. Incubation was carried out up to 5 days at 37°C in a microbiological incubator. Liposome size and zeta-potential were measured using a Malvern Zetasizer 5000 Instrument.
The encapsulated nucleic acid content in such a carrier was evaluated by spectrophotometric assay (absorbance measurements at 260 nm) after extraction of lipids from the liposome suspension with 2 volumes of a chloroform:methanol (1:1) mixture.
Cell culture
All cell lines were grown in RPMI 1640 medium supplemented with 10% fetal bovine serum (FBS), glutamine and antibiotics and incubated in a humified atmosphere containing 5% CO2 at 37°C.
For transfection assays, Jurkat T or HL-60 cells were seeded in 24-well plates (0.2-1.0 × 106 cells per well in 0.5 ml of PBS, or RPMI 1640 in the presence or absence of serum). Following addition of the plasmid DNA-containing liposomes, cells were incubated for 3–8 h and cells were replaced into fresh PBS or RPMI medium with or without 10% FBS.
For determination of transfection efficiency, cells were incubated for 24–48 h and prepared for fluorescence microscope observations by fixation in 4% paraformaldehyde in PBS buffer. Transfection efficiency was evaluated by comparing the number of fluorescent cells to the number of all cells in the microscope field. Microscope fields containing at least 75–150 cells were evaluated.
Toxicity of liposomes
Viability: The viability assays were based on the 2.5% Trypan Blue exclusion test. In some cases the MTT test was used: Assays were performed in sterile 96-well plates. Cells in the logarithmic growth phase were seeded on the plate at 1*104 cells/well/100 μl of complete RPMI 1640. Cells were incubated for 24 h at 37°C, in 5% CO2. After 24 Ch cells were treated with an increasing concentration of liposomes. At the indicated time, 200 μg of blue formazan triazole (MTT) at a concentration of 4 mg/ml in PBS was added to each well and incubated for 4 h at 37°C, in 5% CO2. Then plates were centrifuged at 4000 g, for 10 minutes, at room temperature. The supernatant was carefully removed. The crystals of formed formazan were dissolved in 100 μl of DMSO, and absorbance at 570 nm was measured.
Lipid carrier hemolytic activity: Hemolytic activity was determined based on the concentration of hemoglobin (absorbance at 570 nm) released from a 0.38% erythrocyte suspension in 0.9% NaCl, 10 mM Tris-HCl, pH 7.4 in the presence or absence of liposome suspension (0–100 μg lipid/ml) after 30 min incubation at 37°C. Hundred percent hemolysis was determined after addition of Triton X-100 to achieve final concentration of 0.5% or by placement of the appropriate amount of erythrocyte suspension in water.
Stability of liposomes in the presence of serum
Liposomes were incubated in the presence of 50% human serum for time periods from 30 min up to 30 h at 37°C. After incubation, the liposome fraction was separated from the serum on a Sepharose 4 B column (0.5 × 10 cm).
Protective properties of L-cL liposomes toward encapsulated plasmid in the presence of DNase I or human serum
The enzyme DNase I was stored in buffer (50 mM Tris-HCl-pH 7.5, 10 mM CaCl2 and 50% (v/v) glycerol) at a concentration of 1000 units/ml. 15 μl of liposomal suspension (containing 1 μg pDNA) was incubated with active DNase I for 10 minutes at 37°C (0.1 U enzyme/1 μg DNA). After 10 minutes, DNase was inactivated by heating at 65°C for 10 min in the presence of 50 mM EDTA pH 7.5. Then the liposomes were disrupted by 10% Triton X-100 (v/v) to release encapsulated pDNA, and then pDNA was analyzed by electrophoresis in 1% agarose gel. For a positive control of DNA digestion by DNase, the liposomes were disrupted with Triton X-100 before incubation with that enzyme.
Similarly liposomes were incubated with 50% human serum. The protective properties of liposomes toward genetic drugs were examined after 8 h of incubation at a temperature of 37°C. Then the liposomes were fractioned on microcolumns filled with Sepharose 4 B, in order to remove serum proteins. Purified liposomes were disrupted with 10% Triton X-100 and analyzed by 1% agarose gel electrophoresis. For a positive control of human serum nucleolytic activity, the liposomes were treated with Triton X-100 before incubation with human serum.
Reverse transcriptase PCR and Western blot analyses
Total RNA isolation from cultured cells was performed using an RNeasy Mini Kit (Qiagen, Germany) according to the manual provided by the manufacturer. Obtained RNA was used for reverse transcriptase reaction by using a Robust T I RT-PCR kit, Finnzymes (Finland) according to the manufacturer's manual. The following primers were used: Bcl-2 forward, 5′ GTG GAG GAG CTC TTC AGG GA 3′; Bcl-2 reverse, 5′ AAG CAC CCA GGG TGA TGC AA 3′; and control ODNs of scrambled sequences. β-actin gene (ACTB) primers were of the following sequences: forward, 5′ TAC AAT GAG CTG CGT GTG GCT CCC G 3′; and reverse, 5′ AAT GGT GAT GAC CTG GCC GTC AGG C 3′.
For Western blot analysis cells were extracted with RIPA buffer (1% Triton X-100, 1% sodium deoxycholate, 0.1% SDS, 50 mM Tris-HCl, pH 7.4, 150 mM NaCl, 1 mM EDTA, 5 μg/ml aprotinin, 5 μg/ml leupeptin) for 20 min on ice and centrifuged at 14 000 rpm at 4°C. SDS-polyacrylamide (12%) gel electrophoresis of 15 μg supernatant protein was performed. Then separated proteins were transferred in a buffer: 25 mM Tris, 192 mM glycine, 20% methanol and 0.1% SDSCitation29 onto nitrocellulose membrane. After 3 h at 400 mA the membrane was blocked with 5% nonfat (dry) milk in TBST buffer (20 mM Tris-HCl pH 7.4, 137 mM NaCl, 0.05% Tween 20 and 0.1% bovine serum) for 4 h at room temperature and then with anti-human Bcl-2 mouse monoclonal antibodies and anti-human Actin goat polyclonal antibodies (Santa Cruz, USA) at 1:200 and 1:500 dilution in TBST for 3 h at room temperature or overnight at +4°C. After washing 3 times for 10 min in TBST the membrane was incubated in a 1:2000 dilution of horseradish peroxidase-conjugated goat anti-mouse and donkey anti-goat IgG in TBS buffer (composition as above but Tween was omitted) for 2 h. After washing 3 times with TBS, an enzymatic reaction was performed with a chemiluminescent substrate. Detection of chemiluminescence was carried out using the UVP Multispectral Imaging System.
Biodistribution studies
Liposomes which were fluorescently labeled by addition after the sonication step of DiD (see Lipid carrier preparation section above) to a final concentration of 0.1 mol % were injected in a dose of 50 mg of lipids and 0.8 mg of asODN/kg of body weight in a volume of 100 μl via the tail vein of mice. Liposome distribution was analyzed in vivo by using the NightOwl II LB 983 apparatus (Berthold) equipped with a CCD camera with the following matrix parameters: 1024×1024×13.6 μm, lens 25 mm, focal length 0.95. Berthold WinLight 32 software was used. At the indicated time mice were anesthetized with isoflurane (2-chloro-2-(difluoromethoxy)-1,1,1-trifluoro-ethane) and the fluorescence of labeled liposomes was analyzed.
For blood concentration DiD-labeled liposomes were injected into mice as described above. The blood serum was 10-fold diluted with PBS and fluorescence was measured in a Cary Eclipse spectrofluorimeter at λexc. and λem of 644 and 665 nm respectively.
Therapeutic efficacy of L-cL entrapped asODN against BCL-2
To determine the antitumor activity of our liposome formulation in the test model of human Daudi Burkitt's lymphoma, Daudi cells (5 × 106 cells/mouse), suspended in 200 μl of Hanks’ medium (Laboratory of General Chemistry, Institute of Immunology and Experimental Therapy) and BD MatrigelTM (BD, Immunogen, BD Matrigel Basement Membrane Matrix High Concentration, 10 ml vial, Cat No. 354248), mixed in a ratio of 1:1, were implanted subcutaneously (right side) into NOD/SCID mice (60 males). The measurement of tumor size started 3 days post-implantation, and tumor volume was calculated using the formula: tumor volume = (a2b)/2, where a is the transverse dimension, and b is the longitudinal dimension, both in mm. On day 7 of the experiment when the mean tumor volume was 87.6 ± 33.3 mm3, the mice were injected intravenously via the lateral tail vein with the liposomes. L-cL containing asODN or L-cL containing scODN (50 mg of liposomes containing 0.8 mg of ODNs/kg body weight in a volume of 10 μl per 1 g body weight) or PBS (placebo) were administered intravenously via the lateral tail vein. All preparations were administered once a week at days 7, 14, 21 and 28 of the experiment. The mice were monitored by checking their weight and tumor volume, 3 times per week. The experiment was terminated 30 days after the implantation of Daudi cells.
Tumor growth inhibition (TGI) was calculated as the percentage by which the treated group median tumor weight was inhibited compared to the control using the formula: TGI [%] = (VT / VC) × 100 – 100%, where VT is the median tumor volume found for the treated mice and VC is that of the untreated control animals.
The average body weight change (BWC) in all of the groups was calculated using the formula BWC = (ABWn/ABW1) × 100 – 100%, where ABWn is the average body weight on the nth day of the experiment (during treatment), and ABW1 is the average body weight on the first day of treatment.
Statistics
The statistical analysis of the animal experiment was carried out using STATISTICA software, version 10 (StatSoft Inc., USA). Data were analyzed by the non-parametric test of Kruskal-Wallis ANOVA and a Mann-Whitney test. P values less than 0.05 were considered as statistically significant. The other data were evaluated by Student's t test and P values less than 0.05 were considered significant.
Disclosure of Potential Conflicts of Interest
No potential conflicts of interest were disclosed.
Acknowledgments
The authors acknowledge the expert help of Prof. J Wietrzyk and her colleagues in the animal experiments.
Funding
This work was supported in part by Wroclaw Research Center EIT+ within the project "Biotechnologies and advanced medical technologies"-Biomed (POIG.01.01.02-02-003/08) Co-financed by the European Regional Development Found (Operational Program Innovative Economy, 1.1.2). Publication costs were covered by KNOW Programme for Wrocław Biotechnology Centre.
References
- Nienhuis AW. Development of gene therapy for blood disorders. Blood 2008; 111:4431-44; PMID:18441245; http://dx.doi.org/10.1182/blood-2007-11-078121
- Tagami T, Suzuki T, Matsunaga M, Nakamura K, Moriyoshi N, Ishida T, Kiwada H. Anti-angiogenic therapy via cationic liposome-mediated systemic siRNA delivery. Int J Pharm 2012; 422:280-9; PMID:22101286; http://dx.doi.org/10.1016/j.ijpharm.2011.10.059
- Kurosaki T, Kitahara T, Kawakami S, Higuchi Y, Yamaguchi A, Nakagawa H, Kodama Y, Hamamoto T, Hashida M, Sasaki H. Gamma-polyglutamic acid-coated vectors for effective and safe gene therapy. J Control Release 2010; 142:404-10; PMID:19931327; http://dx.doi.org/10.1016/j.jconrel.2009.11.010
- Zhao X, Pan F, Holt CM, Lewis AL, Lu JR. Controlled delivery of antisense oligonucleotides: a brief review of current strategies. Expert Opin Drug Deliv 2009; 6:673-86; PMID:19552611; http://dx.doi.org/10.1517/17425240902992894
- Wyrozumska P, Stebelska K, Grzybek M, Sikorski AF. Synthetic Vectors for the genetic drug delivery. In: Mozafari MR, ed. Nanocarriers Technologies: Frontiers of Nanotheraphy. Dordrecht, The Netherlands: Springer 2006:139-74.
- Oh YK, Park TG. siRNA delivery systems for cancer treatment. Adv Drug Deliv Rev 2009; 61:850-62; PMID:19422869; http://dx.doi.org/10.1016/j.addr.2009.04.018
- Grijalvo S, Avino A, Eritja R. Oligonucleotide delivery: a patent review (2010–2013). Expert Opin Ther Pat 2014; 24:801-19; PMID:24798406; http://dx.doi.org/10.1517/13543776.2014.915944
- Chang RS, Suh MS, Kim S, Shim G, Lee S, Han SS, Lee KE, Jeon H, Choi HG, Choi Y, et al. Cationic drug-derived nanoparticles for multifunctional delivery of anticancer siRNA. Biomaterials 2011; 32:9785-95; PMID:21937102; http://dx.doi.org/10.1016/j.biomaterials.2011.09.017
- Hoekstra D, Rejman J, Wasungu L, Shi F, Zuhorn I. Gene delivery by cationic lipids: in and out of an endosome. Biochem Soc Trans 2007; 35:68-71; PMID:17233603; http://dx.doi.org/10.1042/BST0350068
- Stuart DD, Allen TM. A new liposomal formulation for antisense oligodeoxynucleotides with small size, high incorporation efficiency and good stability. Biochim Biophys Acta 2000; 1463:219-29; PMID:10675501; http://dx.doi.org/10.1016/S0005-2736(99)00209-6
- Bartsch M, Weeke-Klimp AH, Hoenselaar EP, Stuart MC, Meijer DK, Scherphof GL, Kamps JA. Stabilized lipid coated lipoplexes for the delivery of antisense oligonucleotides to liver endothelial cells in vitro and in vivo. J Drug Target 2004; 12:613-21; PMID:15621687; http://dx.doi.org/10.1080/10611860400013519
- Stuart DD, Semple SC, Allen TM. High efficiency entrapment of antisense oligonucleotides in liposomes. Methods Enzymol 2004; 387:171-88; PMID:15172164; http://dx.doi.org/10.1016/S0076-6879(04)87011-3
- Shen JZ, Zhang YY, Fu HY, Wu DS, Zhou HR. Overexpression of microRNA-143 inhibits growth and induces apoptosis in human leukemia cells. Oncol Rep 2014; 31:2035-42; PMID:24626955
- Youle RJ, Strasser A. The BCL-2 protein family: opposing activities that mediate cell death. Nat Rev Mol Cell Biol 2008; 9:47-59; PMID:18097445; http://dx.doi.org/10.1038/nrm2308
- Robak T, Wierzbowska A. Current and emerging therapies for acute myeloid leukemia. Clin Ther 2009; 31 Pt 2:2349-70; PMID:20110045; http://dx.doi.org/10.1016/j.clinthera.2009.11.017
- Vitagliano O, Addeo R, D'Angelo V, Indolfi C, Indolfi P, Casale F. The Bcl-2/Bax and Ras/Raf/MEK/ERK signaling pathways: implications in pediatric leukemia pathogenesis and new prospects for therapeutic approaches. Expert Rev Hematol 2013; 6:587-97; PMID:24083449; http://dx.doi.org/10.1586/17474086.2013.827415
- Jurecekova J, Hatok J, Stefanikova A, Dobrota D, Racay P. Targeting of Bcl-2 family proteins for treatment of acute leukaemia. Gen Physiol Biophys 2011; 30 Spec No:S3-S12; PMID:21869449; http://dx.doi.org/10.4149/gpb_2011_SI1_3
- Buggins AG, Pepper CJ. The role of Bcl-2 family proteins in chronic lymphocytic leukaemia. Leuk Res 2010; 34:837-42; PMID:20359747; http://dx.doi.org/10.1016/j.leukres.2010.03.011
- Moreira JN, Santos A, Simoes S. Bcl-2-targeted antisense therapy (Oblimersen sodium): towards clinical reality. Rev Recent Clin Trials 2006; 1:217-35; PMID:18473975; http://dx.doi.org/10.2174/157488706778250050
- Milella M, Estrov Z, Kornblau SM, Carter BZ, Konopleva M, Tari A, Schober WD, Harris D, Leysath CE, Lopez-Berestein G, et al. Synergistic induction of apoptosis by simultaneous disruption of the Bcl-2 and MEK/MAPK pathways in acute myelogenous leukemia. Blood 2002; 99:3461-4; PMID:11964319; http://dx.doi.org/10.1182/blood.V99.9.3461
- Rong YP, Aromolaran AS, Bultynck G, Zhong F, Li X, McColl K, Matsuyama S, Herlitze S, Roderick HL, Bootman MD, et al. Targeting Bcl-2-IP3 receptor interaction to reverse Bcl-2's inhibition of apoptotic calcium signals. Mol Cell 2008; 31:255-65; PMID:18657507; http://dx.doi.org/10.1016/j.molcel.2008.06.014
- Fulda S. Inhibitor of apoptosis proteins in pediatric leukemia: molecular pathways and novel approaches to therapy. Front Oncol 2014; 4:3; PMID:24478984; http://dx.doi.org/10.3389/fonc.2014.00003
- Dubielecka PM, Jazwiec B, Potoczek S, Wrobel T, Miloszewska J, Haus O, Kuliczkowski K, Sikorski AF. Changes in spectrin organisation in leukaemic and lymphoid cells upon chemotherapy. Biochem Pharmacol 2005; 69:73-85; PMID:15588716; http://dx.doi.org/10.1016/j.bcp.2004.08.031
- Siwak DR, Tari AM, Lopez-Berestein G. Liposomal antisense oligonucleotides for cancer therapy. Methods Enzymol 2004; 387:241-53; PMID:15172168; http://dx.doi.org/10.1016/S0076-6879(04)87015-0
- Rudin CM, Otterson GA, Mauer AM, Villalona-Calero MA, Tomek R, Prange B, George CM, Szeto L, Vokes EE. A pilot trial of G3139, a bcl-2 antisense oligonucleotide, and paclitaxel in patients with chemorefractory small-cell lung cancer. Ann Oncol 2002; 13:539-45; PMID:12056703; http://dx.doi.org/10.1093/annonc/mdf124
- Rudin CM, Kozloff M, Hoffman PC, Edelman MJ, Karnauskas R, Tomek R, Szeto L, Vokes EE. Phase I study of G3139, a bcl-2 antisense oligonucleotide, combined with carboplatin and etoposide in patients with small-cell lung cancer. J Clin Oncol 2004; 22:1110-7; PMID:15020613; http://dx.doi.org/10.1200/JCO.2004.10.148
- Dai G, Chan KK, Liu S, Hoyt D, Whitman S, Klisovic M, Shen T, Caligiuri MA, Byrd J, Grever M, et al. Cellular uptake and intracellular levels of the bcl-2 antisense g3139 in cultured cells and treated patients with acute myeloid leukemia. Clin Cancer Res 2005; 11:2998-3008; PMID:15837754; http://dx.doi.org/10.1158/1078-0432.CCR-04-1505
- Reimer DL, Zhang Y, Kong S, Wheeler JJ, Graham RW, Bally MB. Formation of novel hydrophobic complexes between cationic lipids and plasmid DNA. Biochemistry 1995; 34:12877-83; PMID:7548044; http://dx.doi.org/10.1021/bi00039a050
- Towbin H, Staehelin T, Gordon J. Electrophoretic transfer of proteins from polyacrylamide gels to nitrocellulose sheets: procedure and some applications. Proc Natl Acad Sci U S A 1979; 76:4350-4; PMID:388439; http://dx.doi.org/10.1073/pnas.76.9.4350