Abstract
The radioprotective potential of histamine on healthy tissue has been previously demonstrated. The aims of this work were to investigate the combinatorial effect of histamine or its receptor ligands and gamma radiation in vitro on the radiobiological response of 2 breast cancer cell lines (MDA-MB-231 and MCF-7), to explore the potential molecular mechanisms of the radiosensitizing action and to evaluate the histamine-induced radiosensitization in vivo in a triple negative breast cancer model. Results indicate that histamine significantly increased the radiosensitivity of MDA-MB-231 and MCF-7 cells. This effect was mimicked by the H1R agonist 2-(3-(trifluoromethyl)phenyl)histamine and the H4R agonists (Clobenpropit and VUF8430) in MDA-MB-231 and MCF-7 cells, respectively. Histamine and its agonists enhanced radiation-induced oxidative DNA damage, DNA double-strand breaks, apoptosis and senescence. These effects were associated with increased production of reactive oxygen species, which correlated with the inhibition of catalase, glutathione peroxidase and superoxide dismutase activities in MDA-MB-231 cells. Histamine was able also to potentiate in vivo the anti-tumoral effect of radiation, increasing the exponential tumor doubling time. We conclude that histamine increased radiation response of breast cancer cells, suggesting that it could be used as a potential adjuvant to enhance the efficacy of radiotherapy.
Abbreviations
H2O2 | = | hydrogen peroxide |
ROS | = | reactive oxygen species |
GPx | = | glutathione peroxidase |
SOD | = | superoxide dismutase |
DCFH-DA | = | dichlorodihydrofluorescein diacetate |
U | = | unit |
SEM | = | standard error of mean |
ER | = | estrogen receptor |
Gy | = | gray |
SF | = | surviving fraction |
TUNEL | = | terminal deoxynucleotidyl transferase dUTP nick end labeling |
H1R | = | histamine receptor 1 |
H2R | = | histamine receptor 2 |
H3R | = | histamine receptor 3 |
H4R | = | histamine receptor 4 |
PBS | = | phosphate buffer saline |
3F-MPHA | = | 2-(3-(trifluoromethyl)phenyl)histamine |
FBS | = | fetal bovine serum |
BSA | = | bovine seroalbumine |
BrdU | = | 5-bromo-2′-deoxyuridine |
IgG | = | immunoglobuline G |
8-OHdG | = | 8-hydroxy-2′-deoxyguanosine |
HA | = | histamine |
Clob | = | clobenpropit |
γH2AX | = | phosphorylated histone H2AX |
Dapi | = | 4′-6-diamidino-2-phenylindole |
sc | = | subcutaneous |
Introduction
Breast cancer remains the most frequently diagnosed female cancer worldwide and the leading cause of cancer death. The global burden of breast cancer exceeds all other cancers, despite screening and improvements in adjuvant treatment.Citation1
Radiation is an effective therapy in patients with locally advanced breast cancer. Tumor control by radiotherapy requires the use of a maximum dose, which can be delivered while maintaining a tolerance risk of normal tissue toxicity.Citation2 The ratio of tumor response to normal-tissue damage is called the therapeutic index and can be manipulated by the use of drugs that preferentially either increase the tumor damage (radiosensitizers) or reduce the biological effects of ionizing radiation on normal tissue (radioprotectors). The clinical use of radiation protectors or radiosensitizers is limited due to their toxicity; thus, the development of effective and non-toxic agents is yet a challenge for oncologists and radiobiologists.Citation3,4 In this regard, it was reported that histamine significantly protects small intestine and bone marrow, from high doses of ionizing radiation, in 2 models of rodents.Citation5-7 In addition, histamine has the ability to prevent ionizing radiation-induced functional and histological alterations of salivary glands.Citation8 These features make histamine a suitable candidate as a radioprotector for patients undergoing radiotherapy.
Histamine [2-(4-imidazolyl)-ethylamine] is an endogenous biogenic amine and is a pleiotropic mediator in different (patho) physiological conditions. It exerts its effects through the activation of 4 different receptors H1, H2, H3 and H4 (H1R, H2R, H3R, H4R).Citation9 Considerable evidence has been accumulated indicating that histamine can modulate proliferation of different normal and malignant cells.Citation9,10 Histamine is involved in growth regulation, differentiation and functioning of mammary gland during development, pregnancy and lactation.Citation9 It has been previously demonstrated that the 4 histamine receptor subtypes are expressed in cell lines derived from human mammary gland.Citation9,11-14 In addition, it has been already reported that histamine is capable of modulating cell proliferation exclusively in the triple negative breast cancer MDA-MB-231 cells [lacking estrogen receptor (ER) α, progesterone and HER-2 receptors] while no effect on proliferation is observed in non-tumorigenic HBL-100 cells.Citation11 Furthermore, histamine acts as an anti-proliferative agent through the H4R in 2 different human breast cancer cells, MDA-MB-231 and MCF-7 (ER α+).Citation14 H4R agonists inhibited proliferation by 50%, increasing the exponential doubling time and the number of apoptotic and senescent cells.Citation14,15 Furthermore, the anti-tumoral effect of histamine and other H4R agonists (clozapine and JNJ28610244) was demonstrated in vivo in xenograft tumors of MDA-MB-231 cells developed in nude mice. Histamine also significantly increased median survival of tumor-bearing animals and tumoral apoptosis.Citation16
In the light of the above mentioned evidences, the aims of this work were: (1) to investigate the combinatorial effect of histamine or its receptor ligands and gamma radiation in vitro on the radiobiological response of 2 breast cancer cell lines with different malignant characteristics, (2) to explore the potential molecular mechanisms of the radiosensitizing action by evaluating the effects of histamine receptor ligands on breast cancer cell proliferation, apoptosis and senescence and also the regulation of reactive oxygen species (ROS) production, antioxidant enzyme modulation and DNA damage, and (3) to evaluate the histamine-induced radiosensitization in vivo in a triple negative breast cancer model.
Results
Histamine modulates the radiobiological parameters of MDA-MB-231 and MCF-7 cell lines
In order to evaluate the response to gamma radiation, cells were treated with histamine or different specific ligands for histamine receptor subtypes, and were irradiated 24 h after treatment with a single dose of gamma radiation. The radiobiological parameters that were obtained from the survival curves adjusted to the linear quadratic model, indicated that histamine and the H1R agonist (3F-MPHA) produced a radiosensitizing effect on MDA-MB-231 cells (SF2Gy: 0.06 ± 0.02 and 0.04 ± 0.01 vs. 0.22 ± 0.04, respectively) while this effect was blocked with the combined treatment of histamine and mepyramine, an H1R antagonist (). In addition, histamine and the H4R agonist clobenpropit enhanced the radiosensitivity of MCF-7 cell line (SF2Gy: 0.16 ± 0.01 and 0.06 ± 0.01 vs. 0.21 ± 0.02, respectively). The combined treatment with the H4R antagonist JNJ7777120 completely reversed the histamine effect (). Similar results (SF2Gy: 0.10 ± 0.02) were obtained with another H4R agonist, VUF8430 (data not shown). In agreement with these results, H1R agonist in MDA-MB-231 cells and H4R agonist in MCF-7 cells decreased the dose that reduces survival to 1% (Dose 0.01) and the one that reduces survival to 10% (Dose 0.10) (). No enhanced radiosensitivity was observed upon clobenpropit and 3F-MPHA treatments in MDA-MB-231 and MCF-7 cells, respectively (). Furthermore, the radiobiological parameters were not significantly modulated by either H2R or H3R agonists in these cell lines (data not shown).
Figure 1. Effect of histamine on the radiosensitivity of breast cancer cells. (A) MDA-MB-231 and (B) MCF-7 cells were cultured in presence or absence of histamine (HA), H1R Agonist (3F-MPHA), H4R Agonist (Clobenpropit) and/or H1R antagonist (Mepyramine), or H4R antagonist (JNJ7777120, JNJ77) and clonogenic survival was determined. Radiobiological parameters (SF 2Gy: fraction of surviving cells after exposure to 2 Gy dose; Dose 0.01: dose that reduces survival to 1%; Dose 0.10: dose that reduces survival to 10%) for (C) MDA-MB-231 and (D) MCF-7 cells were obtained from the survival curves adjusted to the linear quadratic model [SF = e−(αD+βD2)]. Values are means ± SEM of 3 independent experiments performed in triplicates. (ANOVA and Newman-Keuls post test, *P < 0.05; **P < 0.01 vs. Control. #P < 0.05; ##P < 0.01 vs. Histamine).
![Figure 1. Effect of histamine on the radiosensitivity of breast cancer cells. (A) MDA-MB-231 and (B) MCF-7 cells were cultured in presence or absence of histamine (HA), H1R Agonist (3F-MPHA), H4R Agonist (Clobenpropit) and/or H1R antagonist (Mepyramine), or H4R antagonist (JNJ7777120, JNJ77) and clonogenic survival was determined. Radiobiological parameters (SF 2Gy: fraction of surviving cells after exposure to 2 Gy dose; Dose 0.01: dose that reduces survival to 1%; Dose 0.10: dose that reduces survival to 10%) for (C) MDA-MB-231 and (D) MCF-7 cells were obtained from the survival curves adjusted to the linear quadratic model [SF = e−(αD+βD2)]. Values are means ± SEM of 3 independent experiments performed in triplicates. (ANOVA and Newman-Keuls post test, *P < 0.05; **P < 0.01 vs. Control. #P < 0.05; ##P < 0.01 vs. Histamine).](/cms/asset/a9ff00de-4a56-4281-ae04-d8bb58654c06/kcbt_a_987091_f0001_b.gif)
We further explored the effect of histamine receptor agonists on proliferation of 2 Gy dose irradiated cells. The incorporation of BrdU assay showed that gamma radiation reduced the proliferative capacity of both cell lines. In agreement with the results obtained from survival curves, histamine treatment significantly intensified the decrease in proliferation produced by ionizing radiation. Also, H1R agonist and H4R agonist mimicked histamine effect in MDA-MB-231 and MCF-7 cells, respectively ().
Figure 2. Effect of radiation and/or histamine on cell proliferation and cell cycle distribution. (A,B) MDA-MB-231 and (C,D) MCF-7 cells were cultured in presence or absence of histamine (HA), 3F-MPHA, clobenpropit (Clob) or were left untreated (Control, C), and were irradiated 24 h after treatment. (A,C) The incorporation of BrdU was determined as percentage of positive cells 24 h after irradiation (ANOVA and Newman-Keuls post test, **P < 0.01; ***P < 0.001 vs. Control; #P < 0.05; ###P < 0.001 vs. 2 Gy Control). (B,D) The percentage of cells in different phases of the cell cycle was monitored as a function of time using flow cytometry. Results represent the mean value of 3 independent experiments. Insets show the data of untreated (control) and histamine-treated and 24 h post-irradiated cells (HA 2 Gy). ANOVA and Newman-Keuls post test, ***P < 0.001 vs. Control (6 h post-irradiation); #P < 0.05; ###P < 0.001 vs. Control (24 h post-irradiation).
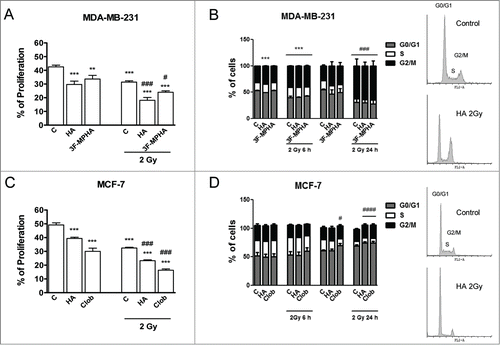
Flow cytometric analysis disclosed that 48 h treatment with histamine induced cell cycle arrest in MDA-MB-231 cells as shown by changes in the percentage of cells in each phase (). Furthermore, radiation induced cell cycle accumulation in G2/M phase in MDA-MB-231 cells, while enhanced cell cycle accumulation in G0/G1 phase in MCF-7 cells ().
Histamine increases apoptosis and senescence of irradiated cells
We next evaluated whether histamine inhibitory effect on proliferation could be associated with a modulation of the apoptotic cell death. Therefore, we investigated apoptosis by the TUNEL assay. Results demonstrated that histamine treatment did increase the number of apoptotic cells compared to the untreated cells in both cell lines (). This assay showed that the number of apoptotic cells increased in 2 Gy irradiated cells 24 h after being treated with the H1R agonist in MDA-MB-231 cells and the H4R agonist in MCF-7 cells (). Accordingly, these results were confirmed by evaluating the apoptotic cells by Annexin-V staining ().
Figure 3. Role of radiation and/or histamine in cell apoptosis and senescence. (A,B,C) MDA-MB-231 and MCF-7 cells were cultured in presence or absence of histamine (HA), 3F-MPHA, clobenpropit (Clob) or were left untreated (Control, C), and were irradiated 24 h after treatment. Percentage of apoptotic cells was determined 24 h after irradiation by the (A) TUNEL assay and (B) Annexin-V staining and flow cytometry (ANOVA and Newman-Keuls post test, *P < 0.05; **P < 0.01; ***P < 0.001 vs. Control; #P < 0.05; ###P < 0.001 vs. 2 Gy Control). (C) Percentage of senescent cells was determined 24 h after irradiation. (ANOVA and Newman-Keuls post test, **P < 0.01; ***P < 0.001 vs. Control; #P < 0.05; ###P < 0.001 vs. 2 Gy Control). Results are means ± SEM of 3 independent experiments.
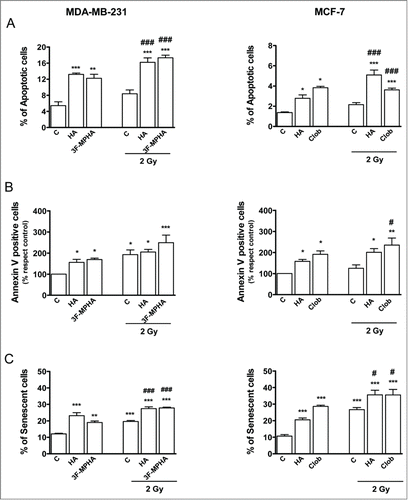
We have previously reported the ability of histamine and H4R agonists to induce cell senescence of tumoral cells.Citation14,17 Coincidently, histamine treatment significantly enhanced the number of senescent cells in irradiated and non-irradiated cells compared to the untreated ones (). This effect was mimicked by the H1R agonist in MDA-MB-231 cells and the H4R agonist in MCF-7 cells ().
Histamine modulates antioxidant enzymes' activity and ROS levels
The level of intracellular ROS was determined immediately after irradiation by flow cytometry.Citation11 Histamine and the H1R agonist increased ROS levels in irradiated and non-irradiated cells (). This outcome is consistent with the reduction of the activity of catalase, GPx and SOD in irradiated and non-irradiated MDA-MB-231 cells (). The decreased SOD activity was associated with a down regulation of CuZnSOD expression levels. However, non-significant modification of catalase and GPx protein expression was observed (). Catalase and GPx activities were not significantly modified in untreated MDA-MB-231 cells after irradiation (), while irradiation induced a reduction of SOD activity ().
Figure 4. Effect of radiation and/or histamine on ROS production. (A) MDA-MB-231 and (B) MCF-7 cells were cultured in presence or absence of histamine (HA), 3F-MPHA, clobenpropit (Clob) or were left untreated (Control, C), and were irradiated 24 h after treatment. Intracellular ROS levels were determined immediately after irradiation by flow cytometry using DCFH-DA fluorescent probe. Data represent the mean fluorescence intensity in percentage with respect to control values. (ANOVA and Newman-Keuls post test, *P < 0.05; ***P < 0.001 vs. Control; ###P < 0.001 vs. 2 Gy Control). Results are means ± SEM of 5 independent experiments. (C, D) Lcn2 expression levels were evaluated by Western blot in MDA-MB-231 (C) and MCF-7 (D) cells. Lanes: 1, untreated cells; 2, histamine-treated cells; 3, agonist-treated cells; 4, irradiated and untreated cells; 5, irradiated and histamine-treated cells; 6, irradiated and agonist-treated cells; positive control (+C, pig kidney). Data are representative of 2 independent experiments. β-actin was used as load control to normalize the expression levels of Lcn2. Semi-quantitative analysis of band intensities for Lcn2 is shown.
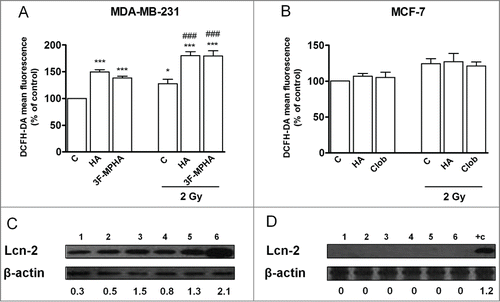
Figure 5. Role of radiation and/or histamine in the expression and activity of antioxidant enzymes. MDA-MB-231 and MCF-7 cells were cultured in presence or absence of histamine (HA), 3F-MPHA, clobenpropit (Clob) or were left untreated (Control, C), and were irradiated 24 h after treatment. (A) Catalase, (B) GPx and (C) SOD activities were evaluated by spectrophotometric techniques 6 h post-irradiation. Data represent the mean in percentage with respect to control values (ANOVA and Newman-Keuls post test, *P < 0.05; **P < 0.01; ***P < 0.001 vs. Control; #P < 0.05; ###P < 0.001 vs. 2 Gy Control). Values are means ± SEM of 3 independent experiments. (D) Catalase, GPx, CuZnSOD expression levels were evaluated by Western blot. MDA-MB-231 cells lanes: 1, untreated cells; 2, histamine-treated cells; 3, 3F-MPHA-treated cells; 4, irradiated and untreated cells; 5, irradiated and histamine-treated cells; 6, irradiated and 3F-MPHA-treated cells. MCF-7 cells lanes: 1, untreated cells; 2, histamine-treated cells; 3, clobenpropit-treated cells; 4, irradiated and untreated cells; 5, irradiated and histamine-treated cells; 6, irradiated and clobenpropit-treated cells. Data are representative of 3 independent experiments. β-actin was used as load control to normalize the expression levels of CuZnSOD. Semi-quantitative analysis of band intensities for CuZnSOD is shown.
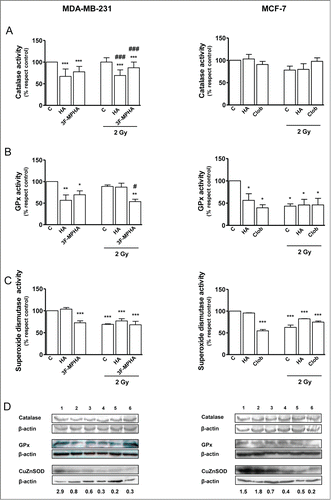
On the other hand, no modification was observed in the levels of ROS () and the activity and expression of catalase in the MCF-7 cells (), while decreased activities of GPx and SOD were seen in treated and/or irradiated MCF-7 cells (). The lower activity of SOD was related to a down regulation of the CuZnSOD enzyme expression after treatment with clobenpropit or irradiation ().
We also examined the Lcn-2 expression by western blot. Lcn-2 protein expression was detected only in MDA-MB-231 cells. Irradiation up-regulated Lcn-2 protein expression and histamine and H1R agonist increased Lcn-2 protein expression in non-irradiated and also in irradiated MDA-MB-231 cells ().
Histamine enhances radiation-induced DNA damage
We evaluated the formation of 8-OHdG as a marker of DNA oxidative damage by immunocytochemistry. Histamine significantly increased 8-OHdG production in non-irradiated MDA-MB-231 cells. Also histamine and H1R agonist enhanced radiation-induced 8-OHdG formation (). Similarly, histamine and the H4R agonist intensified radiation-induced 8-OHdG formation in MCF-7 cells ().
Figure 6. Effect of histamine on radiation-induced DNA damage. (A,C,E,G) MDA-MB-231 and (B,D,F,H) MCF-7 cells were cultured in presence or absence of histamine (HA), 3F-MPHA, clobenpropit (Clob) or were left untreated (Control, C), and were irradiated 24 h after treatment. (A,B) Oxidative DNA damage was evaluated as percentage of nuclear 8-OHdG positive cells 6 h after irradiation. (C,D,E,F) DNA double-strand breaks were evidenced by γH2AX foci formation. (C,D) The average number of foci per cell was determined 20 min after irradiation. Values are means ± SEM of 3 independent experiments (ANOVA and Newman-Keuls post test, *P < 0.05; **P < 0.01; ***P < 0.001 vs. Control; #P < 0.05; ##P < 0.01; ###P < 0.001 vs. 2 Gy Control). (E,F) Representative pictures are shown (630× magnification. Scale bar = 20 μm). (G, H) p53 expression levels were evaluated by Western blot in MDA-MB-231 (G) and MCF-7 (H) cells. Lanes: 1, untreated cells; 2, histamine-treated cells; 3, agonist-treated cells; 4, irradiated and untreated cells; 5, irradiated and histamine-treated cells; 6, irradiated and agonist-treated cells. Data are representative of 2 independent experiments. β-actin was used as load control to normalize the expression levels of p53. Semi-quantitative analysis of band intensities for p53 is shown.
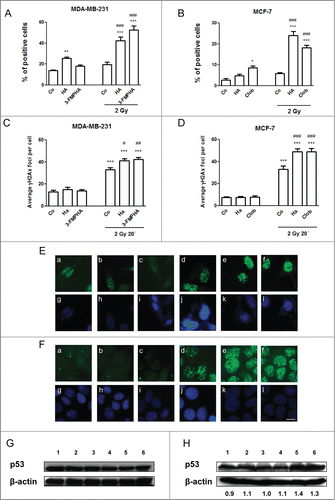
γH2AX was further investigated as a marker of DNA double-strand breaks. Radiation resulted in a significant increase in the number of γH2AX foci, compared with untreated control cells, and histamine receptor ligands significantly enhanced radiation-induced formation of γH2AX foci in MDA-MB-231 and MCF-7 cells () at 20 min. Results were also confirmed by flow cytometric analysis (data not shown).
Ionizing radiation induced expression of p53 and histamine receptor agonists enhanced this further in MCF-7 cells while p53 levels were not significantly modified in MDA-MB-231 cells ().
Histamine potentiates radiation effect on the MDA-MB-231 xenograft tumor
We further explored the effect of histamine in combination with radiation in vivo on the growth of triple negative breast tumors induced in nude mice with MDA-MB-231 cells. The results showed that histamine injected sc from 1 day before irradiation until the end of the experiment, potentiated radiation-induced anti-tumoral effect. Radiation administered as 3 doses of 2 Gy in consecutive days showed only a modest non-significant reduction of tumor size. On the other hand, histamine treatment significantly reduced size and increased exponential doubling time of irradiated tumors (). The histological analysis demonstrated that irradiation enhanced neovascularization with focal areas of extracellular matrix on the tumors of untreated animals. The tumors of the histamine 2 Gy group displayed reduced cellularity and large bands of extracellular matrix separating tumor tissue ().
Figure 7. Combined effect of radiation and histamine on the triple-negative breast tumor induced in nude mice. (A) Evaluation of relative tumor volume in untreated, untreated and 2 Gy irradiated and histamine-treated and 2 Gy irradiated animals. Tumor volumes were measured by day and a non-linear regression fit was performed to evaluate the exponential growth (*P < 0.05 vs. Untreated; #P < 0.05 vs. Untreated 2 Gy; T-test). (B) Comparison of tumor size at the end of the experimental period. Data are shown as means ± SEM of 2 independent experiments. (*P < 0.05 vs. Untreated; #P < 0.05 vs. Untreated 2 Gy; T-test). (C) Median tumor doubling time of each group is depicted numerically (**P < 0.01 vs. Untreated; #P < 0.05 vs. Untreated 2 Gy; ANOVA and Newman–Keuls Multiple Comparison Test). (D) Representative H&E stained images of paraffin-embedded tumor specimens. 630× original magnification. Scale bar = 20 μm.
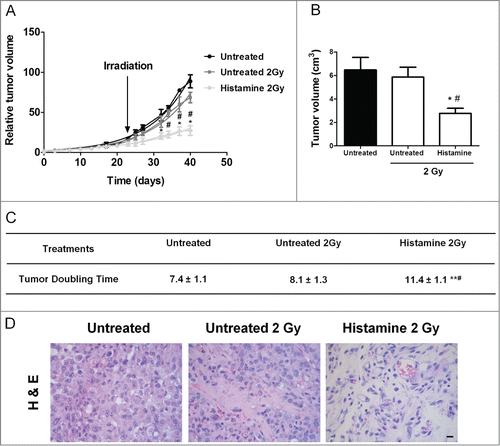
Discussion
Radiotherapy represents one of the cornerstones in the treatment of patients with breast cancer. Radiation reduces local recurrence rates and enhances survival in patients with early-stage cancer after breast-conserving surgery and in node-positive patients with mastectomy. However, it is associated with side effects including an increased risk of cardiovascular disease.Citation18,19 Finding agents that sensitize malignant cells to radiation would therefore increase tumor response allowing to minimize toxicity to surrounding organs by lowering effective therapeutic doses. Numerous conventional chemotherapeutics are known to sensitize cells to the effects of radiation. However, when combined with radiation, these agents also enhance toxicity to normal tissue.Citation4
Histamine was safely used in different experimental models as a radioprotective agent.Citation5-8 Furthermore, we have previously shown that histamine augments the radiosensitivity of the human breast cancer cell line MDA-MB-231 but that it has no effect on the radiosensitivity of the non-tumorigenic HBL-100 cells.Citation11 Therefore, we aimed to elucidate the mechanisms involved in histamine effect by exploring the role of this amine and its receptor agonists on the radiosensitivity of 2 breast cancer cell lines.
Our study provided evidence that histamine acts as a radiosensitizer of these 2 human breast cancer cell lines. This effect was exerted via the H1R in MDA-MB-231 cells and via the H4R in MCF-7 cells. In agreement with these results, a 2 Gy single dose of ionizing radiation produces a decrease in cell proliferation, an effect that was intensified when cells were treated with histamine or H1R agonist and histamine or H4R agonist in MDA-MB-231 and MCF-7 cells, respectively. Also, the radiation-induced growth suppression was associated with cell cycle arrest.
In order to investigate the mechanisms involved in histamine-induced radiosensitization, we evaluated whether histamine could modulate proliferation-associated processes such as cell apoptosis and senescence. Both seem to be involved in the molecular mechanism of radiosensitivity in cancer cells.Citation20 Breast tumor cells are relatively refractory to apoptosis in response to conventional therapies such as ionizing radiation.Citation21 Therefore, promotion of apoptosis is thought to be critical for the effectiveness of radiotherapy.
Results demonstrated that the combined treatment of histamine receptor ligands and ionizing radiation leads to enhanced apoptosis in both cell lines, an effect that can contribute to the increased radiosensitivity observed. In this regard, other radiosensitizers produced their action by increasing apoptosis of breast cancer cells.Citation22
Accumulating evidence suggests that apoptosis may not be the exclusive or even the primary mechanism whereby tumor cells lose their self-renewal capacity after radiation, particularly in the case of solid tumors.Citation20 Loss of reproductive capacity of the tumor cell in response to radiation can occur through alternative pathways, including a terminally arrested state similar to replicative senescence, which has been termed premature or accelerated senescence.Citation20 Furthermore, accelerated senescence appears to mediate the impact of ionizing radiation on self renewal capacity, having shown a close correspondence between the extent of radiation-induced senescence and radiation sensitivity.Citation22,23 Ionizing radiation significantly augmented the number of senescent cells compared with the non-irradiated control cells. Our results show that histamine and the H1R agonist in MDA-MB-231 cells and the H4R agonist in MCF-7 cells have the ability to enhance the number of senescent cells not only in irradiated but also in non-irradiated cells.
Together, these data demonstrate the effect of histamine in combination with radiation, which was effective in activating cell apoptosis and senescence, providing a potential mechanism of histamine-mediated radiosensitization of breast cancer cells with different malignant characteristics.
Radiation-induced damage is introduced into genome, the most sensitive target, by either a direct action or indirectly via formation of ROS. The latter mechanism, which accounts for about 75% of radiation-induced damage by photons, originates a pro-oxidant state which contributes to cell radiation injury and can activate apoptosis.Citation4,24 Furthermore, there is a large body of experimental evidence showing that a rise in the intracellular ROS contributes to cell senescence and may influence the overall tumor response to anticancer therapy.Citation25,26 The net intracellular concentration of ROS is the result of their production and the ability of antioxidants to remove them. Therefore, in order to investigate whether histamine-induced increase in cell apoptosis and senescence was associated with a modulation of ROS levels, we further examined ROS levels and the activity of some metabolizing antioxidant enzymes.
As previously shown, histamine produces a significant increase in ROS levels in MDA-MB-231 cells,Citation11 an effect that was mimicked by the H1R agonist. This effect can be related with a down modulation of catalase, GPx and SOD activities in this cell line. This is in agreement with previous studies that showed that histamine modulates antioxidant enzymes activity in WM35 melanoma cells.Citation17 In line with our results, recent data support that increasing the cellular levels of ROS by using hydrogen-peroxide-generating drugs may be an efficient way of killing cancer cells.Citation27
Lcn-2 is a member of the lipocalin superfamily, with diverse functions, including the induction of apoptosis, and is abnormally expressed in some malignant human cancers.Citation28-30 Previous studies indicated that the expression of Lcn-2 is induced under harmful conditions, where production of free radicals has been reported, and that it could be a useful biomarker for the detection of oxidative stress.Citation31
Results show that histamine and H1R agonist increased apoptosis and ROS levels while upregulated Lcn-2 expression in non-irradiated and irradiated MDA-MB-231 cells. Similar results were observed in HepG2 cells in which ionizing radiation exposure and H2O2 treatment induced Lcn-2 expression.Citation31
Interestingly, we were not able to show a significant modulation of ROS levels or catalase activity by ionizing radiation or histamine treatment in MCF-7 cells in the same experimental conditions. However, a significant reduction of GPx and SOD activities was observed in irradiated and non-irradiated MCF-7 cells that were treated with histamine receptor ligands. Apparently, the modulation of these enzymatic activities is not enough to produce an imbalance on ROS levels in MCF-7 cells. Furthermore, Lcn-2 was not detected at the protein level while was barely detected at the mRNA level (data not shown). These results are consistent with other studies, which showed that MDA-MB-231 cells express markedly higher Lcn-2 levels compared to MCF-7 cells.Citation30
DNA is the principal target for the biological effects of radiation, being the double-strand breaks the most relevant lesions that lead to biological insults, including cell killing.Citation4 Phosphorylation of histone H2AX (γH2AX) occurs rapidly in response to the presence of DNA double-strand breaks and thus, the analysis of γH2AX has the potential to provide useful information on tumor and normal cell response to ionizing radiation after exposure to clinically relevant doses of radiation.Citation32
The number of γH2AX foci per nucleus in MCF-7 and MDA-MB-231 cells increased upon ionizing radiation exposure, an effect that was enhanced by the combined treatment with histamine receptor ligands. After radiation exposure, the number of γH2AX foci reaches a peak at around 30 min and then diminishes after the repair of DNA double-strand breaks as it was previously described.Citation33
Oxidative damage from ROS including free radicals has been considered to play a vital role in ionizing radiation-induced biological effects. 8-OHdG is a major type of oxidative DNA damage, and is widely used as a marker of oxidative stress.Citation34 Therefore, formation of 8-OHdG was further investigated. Results demonstrate that histamine receptor ligands significantly increased 8-OHdG formation in both breast cancer cells. Therefore, these compounds enhance radiation-induced genotoxic activity evidenced by an enhanced number of γH2AX foci and also an increased 8-OHdG formation. p53 is a well-studied nuclear transcription factor that accumulates in response to cellular stress, including DNA damage, and is involved in processes such as cell cycle arrest, senescence and apoptosis.Citation35 Histamine and clobenpropit increased radiation-induced p53 expression in p53 wild-type MCF-7 cells while p53 levels were not modified in the p53 mutated MDA-MB-231 cells. It is interesting to point out that constitutive γH2AX expression is higher in the triple negative and p53 mutated MDA-MB-231 breast cancer cells compared to MCF-7 cells, as it has been previously demonstrated.Citation36
Results have shown that these agents produced a radiosensitizing action involving enhanced radiation-induced DNA damage, apoptosis and senescence in both human estrogen-dependent and p53 wild-type MCF-7 cells and estrogen-independent and p53 mutant MDA-MB-231 cells. Furthermore, in MDA-MB-231 cells these effects correlated with the increased intracellular ROS and inhibition of antioxidant enzymes activity, decreasing antioxidant defense. We hypothesize that the different phenotypical characteristics and differential growth factor requirements could be involved in the dissimilarities observed between MDA-MB-231 and MCF-7 cells.
Histamine dihydrochloride is currently safely used in clinical trials as an adjuvant for the potential treatment of different cancers, exhibiting no unexpected or irreversible side effects,Citation37 and therefore could be an attractive candidate to be used as adjuvant for breast cancer radiotherapy.
Radiotherapy is a locoregional treatment of all invasive breast cancers, including triple negative breast cancer, which accounts approximately for 15–20% of breast cancers subtypes.Citation38,39 It is associated with high proliferative rates, early recurrence and poor survival rates. It is also insensitive to widely used targeted therapies, highlighting the need to develop novel therapies and/or treatment strategies to reduce the mortality associated with this breast cancer subtype. In this regard, we have recently reported that in vivo H4R ligands' administration produced a significant decrease in tumor growth rate in a triple negative breast cancer experimental model.Citation16 Interestingly, histamine was able to potentiate in vivo the anti-tumoral effect of gamma radiation, increasing the exponential tumor doubling time, suggesting that histamine could also be investigated as a potential adjuvant to cancer radiotherapy.
Based on the presented evidence, we conclude that histamine through different receptor subtypes modulates radiosensitivity of breast cancer cell lines, suggesting that it qualifies as a promising radiosensitizer. Therefore, the combined use of histamine and radiation could be an attractive strategy to enhance the efficacy of radiotherapy for both estrogen-dependent and estrogen-independent breast cancers. Prospective clinical trials are warranted to confirm the selective and effective histamine-induced radio-potentiation of breast cancer tumors.
Materials and Methods
Cell culture and treatments
The human breast cancer cell lines MDA-MB-231 and MCF-7 (American Type Tissue Culture Collection, VA, USA) were cultured in RPMI 1640 supplemented with 10% (v/v) fetal bovine serum (FBS), 0.3 g·L−1 glutamine, and 0.04 g·L−1 gentamicin (Gibco BRL, NY, USA). Cells were maintained at 37°C in a humidified atmosphere containing 5% CO2. Histamine and receptor ligands were used at 10 μmol·L−1 concentration according to what was previously described.Citation11,14,15
Radiation dose-response curves
For the radiosensitivity studies, MCF-7 and MDA-MB-231 cells were seeded in 6-well plates and were treated with histamine (Fluka, MO, USA), VUF8430 (Tocris Bioscience, MO, USA), JNJ7777120 (H4R antagonists, Johnson & Johnson Pharmaceutical Research and Development, USA), clobenpropit (H3R antagonist and H4R agonist), 2-(3-(trifluoromethyl)phenyl)histamine (3F-MPHA, H1R agonist), mepyramine (H1R antagonist) (Sigma Chemical Co., MO, USA) or remained untreated. The radiobiological parameters (SF 2Gy: fraction of surviving cells after exposure to 2 Gy dose; Dose 0.01: dose that reduces survival to 1%; Dose 0.10: dose that reduces survival to 10%) were calculated from the clonogenic survival curves as it was previously described.Citation11
Cell proliferation assay
Quantification of cellular DNA synthesis was performed by BrdU (Sigma Chemical Co., MO, USA) incorporation using the previously described assay.Citation15 Briefly, treatments were added to cell cultures 24 h before irradiation with a single dose of 2 Gy, and were maintained up to 24 h after. After that, BrdU (30 μmol·L−1) was added for 2 h. The cells were then washed twice and fixed for 15 min in 4% (v/v) formaldehyde in PBS. To denature the DNA into single-stranded molecules, cells were incubated with 3 N HCl, 1% Triton X-100 (v/v) in PBS for 15 min at room temperature. Cells were washed in 1 ml of 0.1 mol·L−1 Na2B4O7 (Sigma Chemical Co., USA), 1% (v/v) Triton X-100 in PBS, pH 8.5. After inactivating the endogenous peroxidase activity with 3% (v/v) H2O2 in distilled water and blocking with 5% (v/v) FBS in PBS, cells were then incubated with anti-BrdU mouse monoclonal antibody diluted 1:100 in 1% BSA (w/v) in PBS (Sigma Chemical Co., USA) and 1:100 horseradish peroxidase-conjugated anti-mouse IgG and visualized by diamino-benzidine staining (Sigma Chemical Co., St. Louis, MO, USA). Light microscopy was performed on an Axiolab Karl Zeiss microscope (Göttingen, Germany). Photographs were taken at 630× magnifications using a Canon PowerShot G5 camera (Tokyo, Japan). At least 500 cells were scored for each determination.
Cell cycle analysis
Cells were plated, cultured for 24 h and serum-starved for an additional 24 h. Treatments were added to synchronized cell cultures 24 h before irradiation with a single dose of 2 Gy, and were maintained up to 24 h after. Cells were harvested at indicated time points by trypsinization, fixed with ice-cold methanol, centrifuged and resuspended in 0.5 ml of propidium iodide (PI) staining solution (50 μg·mL−1 in PBS containing 0.2 mg·mL−1 of DNase-free RNase A; Sigma Chemical Co., MO, USA). After incubation for 30 min at 37°C, samples were evaluated by flow cytometry. Cell cycle distribution was analyzed using Cylchred version 1.0.2 software (Cardiff University, UK).
Western blot analysis
Samples were processed and protein gel blot analysis was performed as previously described.Citation11,15 The primary antibodies were diluted as follows: mouse anti-catalase, mouse anti-β-actin, goat anti-glutathione peroxidase (GPx) (1:1,000, Sigma Chemical Co. MO, USA), sheep anti-copper-zinc-containing superoxide dismutase (1:1,000, CuZnSOD; Calbiochem, San Diego, CA, USA), mouse anti-P53 (1:500, Santa Cruz Biotechnology, Santa Cruz, CA, USA) and rabbit anti-lipocalin 2 (1:500, Lcn2, Millipore, Temecula, CA, USA). Immunoreactivity was detected by using horseradish peroxidase-conjugated anti-mouse, anti-sheep, anti-goat, anti-rabbit as appropriate (Sigma), and visualized by enhanced chemiluminescence (Amersham Biosciences, Arlington Heights, IL, USA). Densitometric analyses were performed using the software ImageJ 1.32J (NIH, Bethesda, MD, USA).
Senescence-associated β-galactosidase staining
Senescence-associated β-galactosidase-positive cells were detected using the method described by Dimri et al.Citation40 and also previously by us.Citation17 In brief, cells were treated and irradiated 24 h later and maintained up to 24 h. Cells were then fixed and incubated at 37°C for 6 h with 1 mg·mL−1 of 5-bromo-4-chloro-indolyl-b-galactoside (USB Corp., Cleveland, OH, USA) in an appropriate buffer. The percentage of β-galactosidase-positive cells was assessed under light microscopy (Axiolab Karl Zeiss, Göttingen, Germany). All photographs were taken at 630× magnification using a Canon PowerShot G5 camera (Tokyo, Japan).
Determination of apoptosis
Cells were treated and irradiated 24 h later and maintained up to 24 h. Apoptotic cells were determined by TdT-mediated UTP-biotin Nick End labeling (TUNEL) assay according to the manufacturer's instructions (CHEMICON International, CA, USA). Cells were visualized using Axiolab Karl Zeiss microscope (Göttingen, Germany).
Phosphatidylserine exposure on the surface of apoptotic cells was detected by flow cytometry after staining with Annexin V-FITC (BD biosciences, USA), and PI (50 μg·mL−1). Data were analyzed using WinMDI 2.8 software (Scripps Institute, CA, USA).
Measurement of intracellular ROS production
Cells untreated or treated with histamine or its receptor ligands and irradiated or not with a 2 Gy dose were incubated with 5 μmol·L−1 dichlorodihydrofluorescein diacetate (DCFH2-DA), a ROS sensitive fluorescent probe (Sigma Chemical Co., MO, USA) for 30 min at 37°C. Cells were then washed, detached by trypsinazation, and suspended in PBS. Levels of intracellular ROS were measured immediately by flow cytometry on a FACSCalibur flow cytometer (Becton Dickinson, CA, USA), and data analysis was performed using WinMDI 2.8 software (Scripps Institute, CA, USA).
Measurement of antioxidant enzymes activity
Cells treated or untreated, irradiated or not with a 2 Gy dose were washed, scraped and collected in phosphate buffer (50 mmol·L−1 KH2PO4/K2HPO4, pH 7.8). This was followed by sonic disruption and the suspensions were centrifuged at 10,000 g for 10 minutes at 4°C. Protein concentration was determined by Bradford assay.Citation41
Catalase activity was measured spectrophotometrically by monitoring the disappearance of hydrogen peroxide at 240 nm, as previously described.Citation17,42
GPx activity was examined indirectly by spectrophotometrically monitoring the oxidation of NADPH at 340 nm as previously described by Flohé and Gunzler.Citation43 The coupled assay system contained glutathione, glutathione reductase, and t-butyl hydroperoxide as the substrate. One unit of enzyme was defined as the oxidation of 1 nmol of NADPH per minute (ϵ = 6.22 mmol·L−1·cm−1).Citation42,43
Superoxide dismutase (SOD) activity was assayed by inhibition of adrenochrome formation rate at 480 nm. One unit in the SOD assay is determined as the amount of enzymatic protein required to inhibit 50% epinephrine auto-oxidation.Citation44
Immunocytochemistry
Cells were seeded on coverslips in 12-well plates and allowed to grow overnight. Cells were treated and irradiated 24 h after with a single dose of 2 Gy and were then fixed with methanol at −20°C for 10 min followed by washing with PBS. Fixed cells were treated with RNase (100 μg·mL−1) for 1 h at 37°C and proteinase K (10 μg·mL−1) (Sigma Chemical Co., MO, USA) for 10 min at room temperature. After rinsing with PBS, DNA was denatured by treatment with 4 nmol·L−1 HCl for 10 min followed by pH adjustment with 50 mmol·L−1 Tris (pH 10) for 5 min at room temperature. After blocking in 5% (w/v) BSA, cells were incubated overnight at 4°C in a humidified chamber with goat anti-8-hydroxy-2′-deoxyguanosine antibody (8-OHdG, 1:100, Millipore, Temecula, CA, USA). Cells were washed with PBS and incubated for 2 h with 1:400 fluorescein isothiocyanate (FITC)-conjugated anti-goat Immunoglobuline G (IgG) and 4′-6-diamidino-2-phenylindole at room temperature (Dapi, Sigma Chemical Co., MO, USA). Coverslips were mounted with FluorSaveTM Reagent (Calbiochem, USA) and fluorescence was observed under Axiolab Karl Zeiss microscope (Göttingen, Germany).
Immunofluorescent γH2AX staining
Cells were seeded on coverslips in 12-well plates and allowed to grow overnight. Cells were treated and irradiated 24 h after with a single dose of 2 Gy. Cells were washed and fixed with 4% (v/v) paraformaldehyde at 0 or 20 min after irradiation. After blocking in 10% normal blocking serum at room temperature for 10 min, slides were incubated with rabbit anti-phosphorilated histone H2AX antibody (γH2AX, Cell Signaling Technology, Beverly, MA) at 4˚C overnight and then incubated with goat anti-rabbit IgG conjugated with FITC and Dapi at room temperature (Sigma Chemical Co., MO, USA). Coverslips were mounted with FluorSaveTM Reagent (Calbiochem, USA) and fluorescence was observed under Axiolab Karl Zeiss microscope (Göttingen, Germany). For quantification of foci, a minimum of 100 cells were analyzed for each time point.
Animals and treatments
Histamine was diluted in saline solution. Specific pathogen-free athymic female nude (NIH nu/nu) mice were purchased from the Division of Laboratory Animal Production, School of Veterinary Sciences, University of La Plata, Buenos Aires (Argentina), and maintained in sterile isolated conditions. Mice were kept 3 per cage and maintained in our animal health care facility at 22 to 24°C and 50% to 60% humidity on a 12 h light/dark cycle with food and water available ad libitum. Animals with an age of 8–10 weeks and an average weight of 25–30 g were used. Animal procedures were in accordance with recommendations from the Guide for the Care and Use of Laboratory Animals of the National Research Council, USA, 1996, and protocols were approved by the Ethical Committee for the Use and Care of Laboratory Animals of the School of Pharmacy and Biochemistry. Tumors of MDA-MB-231 cells were developed as previously described.Citation16 When the tumor volumes reached 8 mm in diameter (on day 22 after surgery) xenografted mice were separated into 3 groups, the Untreated group received a subcutaneous (sc) daily injection of saline solution (n = 6), Untreated 2 Gy group received a sc daily injection of saline solution (untreated and irradiated animals, n = 6) or Histamine 2 Gy group received a sc daily injection of histamine 5 mg/kg (treated and irradiated, n = 6). One day after treatment began, animals were irradiated with a 2 Gy dose per day for 3 consecutive days. Mice were anesthetized with a combination of xylazine (10 mg/kg) and ketamine (100 mg/kg) and fixed on an acryl plate. Xenografts were locally irradiated with a 60Co γ-radiation source (Teradi 800; Hospital Municipal de Oncología “Marie Curie”), while other body parts were protected with lead blocks.
The length and width of the tumors were measured using a caliper 3 times a week. The tumor size was calculated as sphere volume according to the following formula: Tumor volume [mm3] = 4/3π × [(large diameter + small diameter)/4]Citation3. Tumor growth data analysis was carried out using GraphPad Prism version 5.00. Animals were euthanized by cervical dislocation 40 days after surgery to perform the ex vivo histological studies.
Histopathological studies
Tumors were excised, fixed with 10% (v/v) neutral buffered formalin, paraffin embedded and cut into 4 μm thick serial sections. Tumor morphology and histopathological characteristics were examined on tissue sections after hematoxylin-eosin staining. Light microscopy was performed on an Axiolab Karl Zeiss microscope (Göttingen, Germany). Photographs were taken at 630× magnifications using a Canon PowerShot G5 camera (Tokyo, Japan).
Statistical analysis
Results are presented as means with standard error of mean (SEM). Statistical evaluations were made by analysis of variance (ANOVA), which was followed by Newman-Keuls multiple comparison test or by Student's t-test. All statistical analyses were performed with GraphPad Prism version 5.00 software (San Diego, CA, USA).
Disclosure of Potential Conflicts of Interest
No potential conflicts of interest were disclosed.
Acknowledgment
We thank Miss Natalia Rivera for proofreading the manuscript.
Funding
This work has been supported by grants from the University of Buenos Aires, 20020110200253, 20020100100270, 20020130200148BA, 20020130100799BA, CONICET (PIP 11220110101121), and from the National Cancer Institute, Argentina.
References
- Jemal A, Siegel R, Xu J, Ward E. Cancer statistics. CA Cancer J Clin 2010; 60: 277-300; PMID:20610543; http://dx.doi.org/10.3322/caac.20073
- Henríquez Hernández LA, Lara PC, Pinar B, Bordón E, Rodríguez Gallego C, Bilbao C, Fernández Pérez L, Flores Morales A. Constitutive gene expression profile segregates toxicity in locally advanced breast cancer patients treated with high-dose hyperfractionated radical radiotherapy. Radiat Oncol 2009; 4: 4-17; http://dx.doi.org/10.1186/1748-717X-4-4
- Grdina DJ, Murley JS, Kataoka Y. Radioprotectans: current status and new directions. Oncology 2002; 63: 2-10; PMID:12466639; http://dx.doi.org/10.1159/000067146
- Hall EJ, Giaccia AJ. Hall EJ, Giaccia AJ, Eds, Radiobiology for Radiologists. Philadelphia, Lippincott Williams and Wilkins, 2012. ISBN 0781741513, 9780781741514
- Medina VA, Croci M, Mohamad NA, Massari N, Garbarino G, Cricco GP, Núñez MA, Martín GA, Crescenti EJ, Bergoc RM, et al. Mechanisms underlying the radioprotective effect of histamine on small intestine. Int J Radiat Biol 2007; 83: 653-63; PMID:17729160; http://dx.doi.org/10.1080/09553000701570238
- Medina VA, Croci M, Carabajal E, Bergoc RM, Rivera ES. Histamine protects bone marrow against cellular damage induced by ionising radiation. Int J Radiat Biol 2010; 86: 283-90; PMID:20353338; http://dx.doi.org/10.3109/09553000903564067
- Carabajal E, Massari N, Croci M, Martinel Lamas DJ, Prestifilippo JP, Bergoc RM, Rivera ES, Medina VA. Radioprotective potential of histamine on rat small intestine and uterus. Eur J Histochem 2012; 56: e48; PMID:23361244; http://dx.doi.org/10.4081/ejh.2012.e48
- Medina VA, Prestifilippo JP, Croci M, Carabajal E, Bergoc RM, Elverdin JC, Rivera ES. Histamine prevents functional and morphological alterations of submandibular glands induced by ionising radiation. Int J Radiat Biol 2011; 87: 284-92; PMID:21142703; http://dx.doi.org/10.3109/09553002.2010.533247
- Medina VA, Rivera ES. Histamine receptors and cancer pharmacology. British J Pharmacol 2010; 161: 755-67; http://dx.doi.org/10.1111/j.1476-5381.2010.00961.x
- Rivera ES, Cricco GP, Engel NI, Fitzsimons CP, Martín GA, Bergoc RM. Histamine as an autocrine growth factor: an unusual role for a widespread mediator. Semin Cancer Biol 2000; 10: 15-23; PMID:10888267; http://dx.doi.org/10.1006/scbi.2000.0303
- Medina V, Cricco G, Nuñez M, Martín G, Mohamad N, Correa-Fiz F, Sanchez-Jimenez F, Bergoc R, Rivera ES. Histamine-mediated signaling processes in human malignant mammary cells. Cancer Biol Ther 2006; 5: 1462-71; PMID:17012845; http://dx.doi.org/10.4161/cbt.5.11.3273
- Davio CA, Cricco GP, Andrade N, Bergoc RM, Rivera ES. H1 and H2 histamine receptors in human mammary carcinomas. Agents Actions 1993; 38: C172-74; http://dx.doi.org/10.1007/BF01996450
- Lemos B, Davio C, Gass H, Gonzalez P, Cricco G, Martin G, Bergoc R, Rivera E. Histamine receptors in human mammary gland, different benign lesions and mammary carcinomas. Inflamm Res 1995; 44: S68-9; PMID:8521007; http://dx.doi.org/10.1007/BF01674400
- Medina VA, Brenzoni PG, Lamas DJ, Massari N, Mondillo C, Nunez MA, Pignataro O, Rivera ES. Role of histamine H4 receptor in breast cancer cell proliferation. Front Biosci 2011; 18: 1042-60.
- Medina V, Croci M, Crescenti E, Mohamad N, Sanchez-Jiménez F, Massari N, Nuñez M, Cricco G, Martin G, Bergoc R, et al. The role of histamine in human mammary carcinogenesis: H3 and H4 receptors as potential therapeutic targets for breast cancer treatment. Cancer Biol Ther 2008; 7: 28-35; http://dx.doi.org/10.4161/cbt.7.1.5123
- Martinel Lamas DJ, Croci M, Carabajal E, Crescenti EJ, Sambuco L, Massari NA, Bergoc RM, Rivera ES, Medina VA. Therapeutic potential of histamine H4 receptor agonists in triple-negative human breast cancer experimental model. Br J Pharmacol 2013; 170: 188-99; PMID:23425150; http://dx.doi.org/10.1111/bph.12137
- Medina VA, Massari NA, Cricco GP, Martín GA, Bergoc RM, Rivera ES. Involvement of hydrogen peroxide in histamine-induced modulation of WM35 human malignant melanoma cell proliferation. Free Radic Biol Med 2009; 46: 1510-15; PMID:19285550; http://dx.doi.org/10.1016/j.freeradbiomed.2009.03.003
- Hooning MJ, Botma A, Aleman BM, Baaijens MH, Bartelink H, Klijn JG, Taylor CW, van Leeuwen FE. Long-term risk of cardiovascular disease in 10-year survivors of breast cancer. J Natl Cancer Inst 2007; 99: 365-75; PMID:17341728; http://dx.doi.org/10.1093/jnci/djk064
- Yang TJ, Ho AY. Radiation therapy in the management of breast cancer. Surg Clin North Am 2013; 93: 455-71; PMID:23464696; http://dx.doi.org/10.1016/j.suc.2013.01.002
- Gewirtz DA, Holt SE, Elmore LW. Accelerated senescence: an emerging role in tumor cell response to chemotherapy and radiation. Biochem Pharmacol 2008; 76: 947-57; PMID:18657518; http://dx.doi.org/10.1016/j.bcp.2008.06.024
- Gewirtz DA. Growth arrest and cell death in the breast tumor cell in response to ionizing radiation and chemotherapeutic agents which induce DNA damage. Breast Cancer Res Treat 2000; 62: 223-35; PMID:11072787; http://dx.doi.org/10.1023/A:1006414422919
- Jasinski P, Terai K, Zwolak P, Dudek AZ. Enzastaurin renders MCF-7 breast cancer cells sensitive to radiation through reversal of radiation-induced activation of protein kinase C. Eur J Cancer 2000; 44: 1315-22; http://dx.doi.org/10.1016/j.ejca.2008.03.024
- Podtcheko A, Ohtsuru A, Namba H, Saenko V, Starenki D, Palona I, Sedliarou I, Rogounovitch T, Yamashita S. Inhibition of ABL tyrosine kinase potentiates radiation-induced terminal growth arrest in anaplastic thyroid cancer cells. Radiat Res 2006; 165: 35-42; PMID:16392960; http://dx.doi.org/10.1667/RR3466.1
- Saha GP: Physics and Radiobiology of Nuclear Medicine, New York: Springer-Verlag Inc, 2003.
- Kim R, Emi M, Tanabe K. Role of apoptosis in cancer cell survival and therapeutic outcome. Cancer Biol Ther 2006; 52: 1424-29
- Roninson IB. Tumor cell senescence in cancer treatment. Cancer Res 2003; 63: 2705-15; PMID:12782571
- López-Lázaro M. Dual role of hydrogen peroxide in cancer: possible relevance to cancer chemoprevention and therapy. Cancer Lett 2007; 252: 1-8; http://dx.doi.org/10.1016/j.canlet.2006.10.029.
- L Devireddy LR, Teodoro JG, Richard FA, Green MR. Induction of apoptosis by a secreted lipocalin that is transcriptionally regulated by IL-3 deprivation. Science 2001; 293: 829-34; PMID:11486081; http://dx.doi.org/10.1126/science.1061075
- Chien MH, Ying TH, Yang SF, Yu JK, Hsu CW, Hsieh SC, Hsieh YH. Lipocalin-2 induces apoptosis in human hepatocellular carcinoma cells through activation of mitochondria pathways. Cell Biochem Biophys 2012; 64: 177-86; PMID:22707293; http://dx.doi.org/10.1007/s12013-012-9370-1
- Yang J, Moses MA. Lipocalin 2: a multifaceted modulator of human cancer. Cell Cycle 2009; 15: 2347-52; http://dx.doi.org/10.4161/cc.8.15.9224
- Roudkenar MH, Kuwahara Y, Baba T, Roushandeh AM, Ebishima S, Abe S, Ohkubo Y, Fukumoto M. Oxidative stress induced lipocalin 2 gene expression: addressing its expression under the harmful conditions. J Radiat Res 2007; 48: 39-44; PMID:17229997; http://dx.doi.org/10.1269/jrr.06057
- Olive PL, Banáth JP. Phosphorylation of histone H2AX as a measure of radiosensitivity. Int J Radiat Oncol Biol Phys 2004; 58: 331-35; PMID:14751500; http://dx.doi.org/10.1016/j.ijrobp.2003.09.028
- Mah LJ, Orlowski C, Ververis K, Vasireddy RS, El-Osta A, Karagiannis TC. Evaluation of the efficacy of radiation-modifying compounds using γH2AX as a molecular marker of DNA double-strand breaks. Genome Integr 2011; 2: 3; PMID:21261999; http://dx.doi.org/10.1186/2041-9414-2-3
- Kasai H. Analysis of a form of oxidative DNA damage, 8-hydroxy-2'-deoxyguanosine, as a marker of cellular oxidative stress during carcinogenesis. Mutat Res 1997; 387: 147-63; PMID:9439711; http://dx.doi.org/10.1016/S1383-5742(97)00035-5
- Farnebo M, Bykov VJ, Wiman KG. The p53 tumor suppressor: a master regulator of diverse cellular processes and therapeutic target in cancer. Biochem Biophys Res Commun 2010; 396: 85-9; PMID:20494116; http://dx.doi.org/10.1016/j.bbrc.2010.02.152
- Nagelkerke A, van Kuijk SJ, Sweep FC, Nagtegaal ID, Hoogerbrugge N, Martens JW, Timmermans MA, van Laarhoven HW, Bussink J, Span PN. Constitutive expression of γ-H2AX has prognostic relevance in triple negative breast cancer. Radiother Oncol 2011; 101: 39-45; PMID:21840613; http://dx.doi.org/10.1016/j.radonc.2011.07.009
- Romero AI, Thorén FB, Aurelius J, Askarieh G, Brune M, Hellstrand K. Post-consolidation immunotherapy with histamine dihydrochloride and interleukin-2 in AML. Scand J Immunol 2009; 70: 194-205; PMID:19703009; http://dx.doi.org/10.1111/j.1365-3083.2009.02303.x
- Brouckaert O, Wildiers H, Floris G, Neven P. Update on triple-negative breast cancer: prognosis and management strategies. Int J Womens Health 2012; 4: 511-20; PMID:23071421
- Amos KD, Adamo B, Anders CK. Triple-negative breast cancer: an update on neoadjuvant clinical trials. Int J Breast Cancer 2012; 2012: 385978; PMID:22461984; http://dx.doi.org/10.1155/2012/385978
- Dimri GP, Lee X, Basile G, Acosta M, Scott G, Roskelley C, Medrano EE, Linskens M, Rubelj I, Pereira-Smith O, et al. Biomarker that identifies senescent human cells in culture and in aging skin in vivo. Proc Natl Acad Sci USA 1995; 92: 9363-67; PMID:7568133; http://dx.doi.org/10.1073/pnas.92.20.9363
- Bradford MM. A rapid and sensitive method for the quantification of microgram quantities of protein utilizing the principle of protein-dye binding. Anal Biochem 1976; 72: 248-54; PMID:942051; http://dx.doi.org/10.1016/0003-2697(76)90527-3
- Policastro L, Molinari B, Larcher F, Blanco P, Podhajcer OL, Costa CS, Rojas P, Durán H. Imbalance of antioxidant enzymes in tumor cells and inhibition of proliferation and malignant features by scavenging hydrogen peroxide. Mol Carcinogenesis 2004; 39: 103-13; http://dx.doi.org/10.1002/mc.20001
- Flohé L, Gunzler WA. Assays of glutathione peroxidase. Methods Enzymol 1984; 105: 114-21; http://dx.doi.org/10.1016/S0076-6879(84)05015-1
- Misra HP, Fridovich I. The role of superoxide anion in the autoxidation of epinephrine and a simple assay for superoxide dismutase. J Biol Chem 1972; 247: 3170-75; PMID:4623845