Abstract
Mammalian oocyte maturation is distinguished by asymmetric division that is regulated primarily by cytoskeleton, including microtubules and microfilaments. Small Rho GTPase RhoA is a key regulator of cytoskeletal organization which regulates cell polarity, migration, and division. In this study, we investigated the roles of RhoA in mammalian oocyte meiosis and early embryo cleavage. (1) Disrupting RhoA activity or knock down the expression of RhoA caused the failure of polar body emission. This may have been due to decreased actin assembly and subsequent spindle migration defects. The involvement of RhoA in this process may have been though its regulation of actin nucleators ROCK, p-Cofilin, and ARP2 expression. (2) In addition, spindle morphology was also disrupted and p-MAPK expression decreased in RhoA inhibited or RhoA KD oocytes, which indicated that RhoA also regulated MAPK phosphorylation for spindle formation. (3) Porcine embryo development was also suppressed by inhibiting RhoA activity. Two nuclei were observed in one blastomere, and actin expression was reduced, which indicated that RhoA regulated actin-based cytokinesis of porcine embryo. Thus, our results demonstrated indispensable roles for RhoA in regulating porcine oocyte meiosis and cleavage during early embryo development.
Abbreviations
GVBD | = | germinal vesicle breakdown |
CGFD | = | cortical granule-free domain |
MII | = | metaphase II |
NPFs | = | nucleation promoting factors |
MT | = | microtubule |
KD | = | knock down |
COCs | = | cumulus oocyte complexs |
DOs | = | denuded oocytes |
Introduction
The meiotic maturation of mammalian oocytes is characterized by unique asymmetric cytokinesis during which chromosomes move from the center toward the cortex area of an oocyte. After germinal vesicle breakdown (GVBD), a meiotic spindle assembles around centrally positioned metaphase chromosomes and then moves peripherally to the cortex in an actin filament-dependent process. In addition, cortical reorganization occurs: an ectopic actin-rich actin cap and a cortical granule-free domain (CGFD) formed proximity to the cortex devoid of microvilli in a MOS-dependent manner.Citation1 Establishing cortical polarity, including spindle migration, positioning and cortical reorganization is critical for oocyte asymmetric divisions.Citation2 Then cytokinesis occurs and the polar body was extruded, forming a high polarized MII oocyte.Citation3,4
Recent studies suggested that several signaling molecules were involved in regulating the cytoskeleton to establish oocyte polarity. Actin nucleators Arp2/3 complex and the formin Formin2 were shown to be involved into this process. Knock down of Arp2/3 or its nucleation promoting factors (NPFs) caused the aberrant actin expression and the failure of polar body extrusion.Citation5,6 And depletion of Formin 2 caused the spindle migration defects and symmetric division.Citation7,8 Moreover, the Rho GTPases including Cdc42, Ran, and Rac1 were demonstrated to be upstream regulators of actin nucleators and be critical for oocyte spindle positioning. Cdc42 is considered to associate with actin assembly in sea urchin and Xenopus eggsCitation9,10 and is also confirmed to participate in regulating polar body extrusion in Xenopus and mouse oocytes.Citation11,12 This may be due to its regulatory roles on microtubule and actin filament dynamics.Citation13 In addition to Cdc42, Ran and Rac1 are also involved in controlling cortical polarity during polar body extrusion via the cytoskeleton-related regulation in mouse oocytes.Citation14,15 Additionally, a recent study showed that polar body extrusion requires the combined effects of RhoA and Cdc42 on actin filaments in Xenopus.Citation16
RhoA (24 kDa) is also a member of Rho GTPases, which belongs to the Ras superfamily of small GTP-binding proteinsCitation17 and has been characterized in a wide variety of animal cell types. Similar to most members of the Rho GTPases, RhoA acts as a molecular switch to trigger a wide variety of fundamental cellular processes in somatic cells, including cytoskeletal organization and cell cycle progression, survival, polarity, and migration. There are 2 possible functions of RhoA for regulating cell division, with the actin cytoskeleton as one of its main targets.Citation18 Several studies indicated that RhoA was also required for reorganization of the actin cytoskeleton, maintaining cleavage activities during embryo division and regulating actomyosin contractility.Citation19,20 In addition to its regulation on actin assembly, RhoA is also crucial for regulating microtubule (MT) organization and dynamics.Citation21 Inhibiting RhoA activity prevents the formation of stable Glu-MTs during cell polarization and migration.Citation22 Although RhoA protein has been implicated in multiple critical biological processes, their physiological functions and signaling cascade during mammalian oocyte meiosis and embryo development have not yet been established.
Here, we hypothesized that RhoA might be involved in porcine oocyte maturation and embryo development. For the porcine model, the maturation time of porcine oocyte is much longer compared with mouse system. And since the size of porcine oocyte is much larger, whereas the spindle is much smaller than mouse, a longer distance for actin-mediate spindle migration during porcine oocyte meiotic maturation is needed, and the asymmetry is more typical compared with mouse due to the time and space aspects. Moreover, the porcine model shares more similarities with human, which makes the porcine model more benefits for human reproduction compared to the mouse. And our current results indicated the critical roles of RhoA during porcine oocyte maturation and early embryo development via its regulatory roles for actin assembly and spindle formation.
Results
RhoA localization in porcine oocytes
We first examined the subcellular localization of RhoA at different stages during meiotic maturation of porcine oocytes by immunofluorescent staining. Oocytes were first cultured for 12, 26, or 44 h, which corresponded to the times to achieve GVBD, the MI and MII stages, respectively. As shown in , RhoA was primarily distributed uniformly at the cortex of porcine oocytes and was also concentrated at the microtubules of meiotic spindles during all meiotic stages. Using double staining for α-tubulin and RhoA, we confirmed the co-localization of RhoA and α-tubulin ().
Figure 1. RhoA protein localization in porcine oocytes. (A) Subcellular RhoA localization from GVBD to the MII stage of porcine oocyte meiotic maturation. RhoA accumulated at the cortex and spindle microtubules during all stages of meiosis. Blue, chromatin; red, RhoA. Bar = 20 μm (B) Immunofluorescent staining for co-localization of RhoA and α-tubulin in oocytes. RhoA co-localized with spindles at the MI stage. Blue, chromatin; red, RhoA; green, α-tubulin.
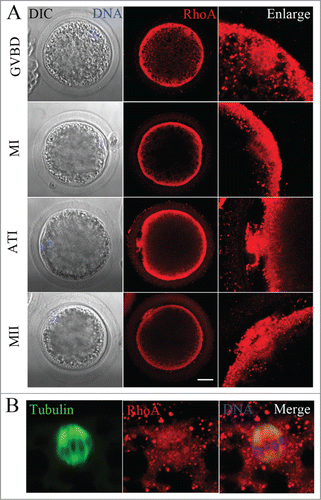
Disruption of RhoA blocks polar body extrusion in vitro
To assess the roles of RhoA during meiotic maturation, we inhibited RhoA activity using an inhibitor Rhosin and knocked down the expression of RhoA by RNAi. After RhoA siRNA microinjection, RhoA protein expression was significantly reduced as compared with controls (relative RhoA/α-tubulin intensity of 0.21 vs. 1 control; P < 0.01; ). For inhibitor treatment, COCs were treated with Rhosin at different doses for 44 h, after which meiotic maturation competence of control and Rhosin treated oocytes were compared. As shown in , the expansion of the peripheral layers cumulus achieved to more than 5 layers in control group, whereas it became progressively poor in Rhosin-treated COCs. Moreover, most control oocytes had extruded small polar bodies and were arrested at the MII stage, whereas Rhosin treatment suppressed polar body extrusion, the polar body extrusion defects was also confirmed by RhoA knock down (KD) groups. RhoA inhibition resulted in reduced polar body extrusion in a dose-dependent manner (). Rhosin treatment effectively inhibited polar body emission at a concentration of 100 μM. These results showed that 89.70 ± 1.67% (n = 227 COCs) of control oocytes had extruded polar bodies. However, with 100 μM and 150 μM Rhosin treatment, the rates of polar body extrusion were significantly reduced to 57.83 ± 1.91% (P < 0.05; n = 211 COCs) and 42.07 ± 1.31% (P < 0.01; n = 198 COCs), respectively. In addition, most oocytes failed to extrude a polar body with 200 μM Rhosin for which the rate of polar body extrusion was 27.93 ± 3.51% (P < 0.01; n = 211 COCs). The polar body extrusion defects were also found in RhoA KD groups. Only 30.46 ± 1.89% (n = 122) of RhoA depletion oocytes extruded first polar body compared to the control oocytes (77.04 ± 2.67%; n = 161; P < 0.01) (). These results suggested that RhoA was crucial for polar body extrusion in porcine oocytes. In this study, a Rhosin concentration of 200 μM was used for subsequent studies with oocytes.
Figure 2. RhoA inhibition and RhoA KD effect on porcine oocyte maturation. (A) Knowdown of endogenous RhoA protein expression after RhoA siRNA injection was verified by western blot analysis. RhoA protein expression was significantly decreased after siRNA injection. (B) RhoA inhibition and RhoA KD both affect meiotic maturation. For COCs, the expansion of the peripheral layers cumulus achieved to more than 5 layers in control group, whereas it became progressively poor in Rhosin-treated COCs; For oocytes, most oocytes extruded a first polar body (indicated by white arrow) in the control group. After RhoA inhibition or RhoA KD, a large proportion of oocytes failed to extrude polar bodies. Bar = 100 μm (C) RhoA inhibition results in a decreased rate of pbI extrusion among COCs. The effect of Rhosin on maturing oocytes was clearly dose dependent. The most suitable concentration for use with COCs was 200 μM. (D) RhoA KD results in a decreased rate of pbI extrusion among DOs. (E) RhoA inhibition results in a decreased rate of pbI extrusion among DOs. The effect of Rhsin on maturing oocytes was clearly dose dependent. The most suitable concentration for use with DOs was 50 μM, as higher concentrations resulted in oocyte death (data not shown).
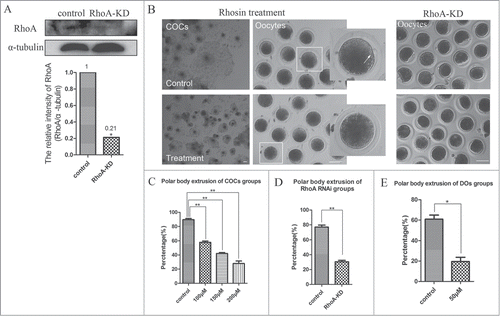
To exclude the effects of cumulus cells on oocyte maturation, denuded oocytes (DOs) were treated with 50 μM Rhosin (). Compared with the controls for which the rate of oocytes that extruded a polar body was 60.90 ± 4.01% (n = 88 DOs), this rate was significantly reduced to 19.55 ± 4.14% after Rhosin treatment (P < 0.05; n = 73 DOs). These results showed that RhoA directly affected meiotic nuclear maturation of porcine oocytes. For subsequent analyses, COCs were used to investigate the regulatory mechanisms of RhoA on porcine oocyte meiotic maturation.
Disruption of RhoA affects actin assembly and spindle positioning in porcine oocytes
To investigate the regulatory mechanism of RhoA during meiosis, actin expression was assessed using intact MI oocytes. As shown in , treatment with 200 μM Rhosin caused profound changes in the arrangements of the actin cytoskeleton. Staining F-actin with phalloidin showed that cortical actin had accumulated uniformly in control MI oocytes, and actin was also abundantly distributed in the cytoplasm of control oocytes. However, actin expression was drastically reduced in the cytoplasm and at the cortex of Rhosin treated MI oocytes. The cortical actin expression of RhoA KD oocytes also reduced, supporting for these results. To confirm the reduced actin expression, we also quantitatively determined the actin fluorescence intensity levels of control and treated oocytes. As shown in , as compared with controls, actin fluorescence intensity was significantly reduced at the cortex after Rhosin treatment. For controls, actin fluorescence intensity at the membrane was 86.77 ± 4.06 (n = 48), whereas it was only 35.77 ± 2.57 (P < 0.01) in the treated oocytes. Similarly, the intensity of cytoplasmic actin signals in treated oocytes was lower than that in control oocytes. Compared with 34.94 ± 3.89 for the control, it was 25.83± 2.02 (P < 0.05) for treated oocytes. A similar result was detected in RhoA KD oocytes. For controls, the fluorescence intensity of cortical actin was 90.75 ± 3.67, whereas, it was significantly reduced to 44.01 ± 3.48 (n = 24; P < 0.01) depleted of RhoA. Thus, RhoA was involved in regulating actin assembly during porcine oocyte meiosis.
Figure 3. RhoA inhibition and RhoA KD both affect actin assembly and spindle positioning in porcine oocytes. (A) Actin signals at the membrane and in the cytoplasm of MI oocytes. Oocytes that were treated with 200 μM Rhosin had profoundly decreased actin cytoskeleton expression. Cortical actin and cytoplasmic actin were not visible in oocytes. Depletion of RhoA confirmed the significant decreased of cortical actin expression. Red, actin. Bar = 20 μm (B) Actin fluorescence intensity at the membrane and in the cytoplasm of MI oocytes. Compared to control oocytes, actin fluorescence intensity was significantly decreased in inhibitor treated or RhoA KD oocytes. (C) Spindle localization in oocytes. For controls, spindles were located peripherally, whereas they were nearly centrally located in Rhosin treated and RhoA KD oocytes. Enlarged images below show the distances of spindles from the cortex. (D) Spindle stages and localization after 26 h in culture. For the controls, the number of oocytes with peripherally located spindles was significant higher than in the Rhosin treated and RhoA KD oocytes (P < 0.01). (E) Formation of actin caps and CGFD's. After RhoA inhibition, the actin cap over a chromosome disappeared. RhoA inhibition also disrupted CGFD formation. Red, actin; green, CGs; blue, chromatin. Bar = 20 μm
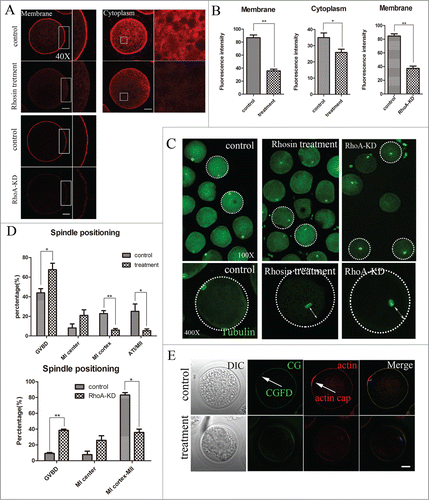
As noted above, porcine oocytes treated with Rhosin failed to extrude polar bodies. Thus, we focused on the effects of RhoA on spindle positioning. The spindle locations of MI oocytes were assessed using the immunofluorescent images of spindles that had been labeled with an anti-α-tubulin antibody. As shown in , spindles were located peripherally in control oocytes, whereas their migration to the oocyte cortex was disrupted in most RhoA inhibition and RhoA KD oocytes. The enlarged images below in this figure show a peripherally localized spindle in a control oocyte and a centrally localized spindle in both Rhosin treated and RhoA KD oocyte.
To further confirm this, the stage and localization of spindles were determined after culture for 26 h. As shown in , the rates of control oocytes that remained in the GVBD period or exhibited centrally located MI spindles were 43.92 ± 4.27% (n = 231) and 8.22 ± 4.13%, respectively, whereas these rates increased to 67.51 ± 6.58% (n = 201; P < 0.05) and 20.97 ± 5.71%, respectively, for treated oocytes. However, the percentage of oocytes with MI cortex spindles or in the AT/MII stages decreased after treatment. The rate of control oocytes with spindles at the cortex was 22.81 ± 2.95%, which was significantly higher than 6.07 ± 1.58% (P < 0.01) for treated oocytes. In addition, the proportion of control oocytes in the AT/MII stage was 25.04 ± 7.53%, whereas it declined to only 5.46 ± 1.83% (P < 0.05) after Rhosin treatment. Spindle positioning of RhoA KD oocytes also confirmed these. The percentage of RhoA KD oocytes in GVBD stage or with the MI center located spindles were 38.42 ± 1.58% and 25.79 ± 5.79%, which are higher than that of 9.17 ± 1.17% (P < 0.01) and 7.23 ± 4.28% for control oocytes, respectively; however, the rate of oocytes from exhibited MI cortex spindles to MII stages was sharply decreased to 35.79 ± 4.21% (P < 0.01) as compared with 83.11 ± 3.11% for controls. Thus, RhoA was essential for spindle positioning and the processes associated with meiosis.
To further confirm that RhoA inhibition affected oocyte polarity, actin cap formation and cortical granule free domain (CGFD), characteristic of successful spindle positioning, were also investigated. In control oocytes, actin cap formed normally, whereas with RhoA inhibition, actin cap over the chromosomes had disappeared (). RhoA inhibition also disrupted the formation of CGFD, as in control oocytes, cortical granules (CGs) were absent in the region where the actin cap formed over the chromosomes at the MI stage, whereas CGs were uniformly distributed throughout the entire cortex and resulted in CGFD formation failure after RhoA inhibition. Thus, these results confirmed that disrupting RhoA activity affected oocyte polarity formation, which further confirmed the failure of spindle migration.
Disruption of RhoA results in reduced ROCK, p-Cofilin, and ARP2 expression
To investigate a possible signaling pathway of RhoA for actin assembly during porcine oocytes meiosis, ROCK expression, a downstream effector of RhoA, was assessed after inhibiting RhoA activity. shows that after RhoA inhibition, ROCK protein expression was significantly reduced. The relative intensity of ROCK protein expression (ROCK/α-tubulin) also confirmed this. For control oocytes, the relative intensity of ROCK protein expression was 1.42 ± 0.13, whereas it was only 0.79 ± 0.13 (P < 0.01) after Rhosin treatment. The declined ROCK expression also confirmed in RhoA KD oocytes. (relative ROCK/α-tubulin intensity of 0.17 vs. 0.29 control; P < 0.05).Thus, RhoA was crucial for ROCK expression during meiosis.
Figure 4. RhoA inhibition affects ROCK, p-Cofilin, and ARP2 expression. (A) ROCK expression decreased after Rhosin treatment or RhoA KD, as the relative intensity of ROCK protein expression (ROCK/α-tubulin) was significantly reduced (P < 0.01). (B) p-Cofilin expression decreased after Rhosin treatment or RhoA KD, as the relative intensity of p-Cofilin protein expression (p-Cofilin/α-tubulin) was significantly reduced (P < 0.01). (C) p-Cofilin localization after Rhosin treatment or RhoA KD. p-Cofilin expression was barely detectable in treated oocytes. Blue, chromatin; Green, tubulin; Red, p-Cofilin. Bar = 5 μm (D) ARP2 expression was decreased after Rhosin treatment or RhoA KD, as the relative intensity of ARP2 protein expression (ARP2/α-tubulin) was significantly reduced (P < 0.01).
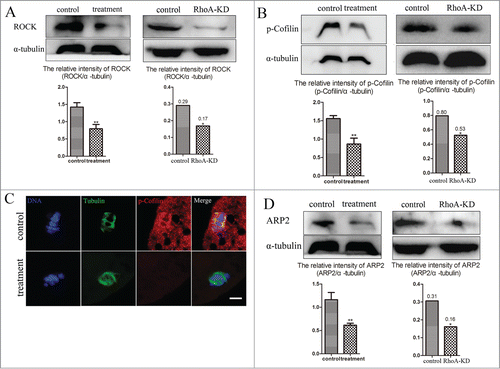
We next investigated P-Cofilin expression, a well-known downstream molecule of ROCK. p-Cofilin protein expression was also reduced after inhibiting RhoA activity or depleting RhoA expression (). The relative p-Cofilin protein expression (p-Cofilin/α-tubulin) confirmed this significant decrease. Compare with 1.56 ± 0.08 for control oocytes, the relative intensity was 0.87 ± 0.1 (P < 0.01) for Rhosin treated oocytes. The relative p-Cofilin/α-tubulin intensity is 0.53 in RhoA KD oocytes compared with 0.80 in controls (P < 0.05) .Moreover, using immunofluorescence staining, it was suggested that p-Cofilin was specifically found at the spindles of porcine oocytes, whereas p-Cofilin was barely expressed in treated oocytes (). Taken together, these results suggested that RhoA was required for Cofilin phosphorylation during meiosis.
We also assessed ARP2 expression, a nucleating component for regulating actin assembly. As shown in , compared with control oocytes, ARP2 was barely expressed in oocytes after RhoA inhibition or RhoA depletion. The relative intensity of ARP2 protein expression (ARP2/α-tubulin) also demonstrated this significant decrease. For controls, the relative intensity was 1.16 ± 0.15, whereas it sharply declined to 0.61 ± 0.04 (P < 0.01) in treated oocytes. The relative ARP2/α-tubulin intensity in RhoA KD groups also determined these (0.16 vs. 0.31 control; P < 0.05).Thus, this suggested that RhoA was essential for ARP2 expression during porcine oocyte meiosis.
Disruption of RhoA affects meiotic spindle morphology and p-MAPK expression
We next examined spindle morphology after disrupting RhoA activity. As shown in , after culture for 26 h, control oocytes in the MI stage had morphologically typical MI spindles. However, abnormal spindle formation was detected in both RhoA-inhibited and RhoA KD oocytes. To confirm this, the percentages of morphologically abnormal spindles were determined. For controls, the percentage of morphologically abnormal spindles was only 7.26 ± 6.68% (n = 230), while this increased to 38.21 ± 6.68% (n = 202) for 200 μM Rhosin treated oocytes (P < 0.01). In addition, compared with 5.54 ± 3.94% (n = 75) in control oocytes, the percentage of morphologically abnormal spindles in RhoA KD oocytes was significant increased to 42.78 ± 7.22% (n = 88; P < 0.01) (). Thus, these results indicated that RhoA was involved in meiotic spindle organization.
Figure 5. RhoA inhibition, RhoA KD affect meiotic spindle morphology and p-MAPK expression. (A) Meiotic spindle morphology in MI oocytes. Control oocytes had morphologically normal spindles, whereas spindles were abnormal in inhibitor treated and RhoA KD oocytes. Gray, DIC; Green, tubulin; blue, chromatin. Bar = 20 μm (B) Rates of abnormal spindles. For Rhosin treated oocytes, the percentage of abnormal spindle morphology was significant higher than that of controls (P < 0.01). RhoA depletion also showed a significant higher percentage of abnormal spindle morphology compared with controls (P < 0.01) (C) p-MAPK expression after RhoA inhibition and RhoA KD was significantly reduced (P < 0.05). (D) p-MAPK localization after RhoA inhibition. Green, tubulin; blue, chromatin. Red, p-MAPK. Bar = 5 μm. For control oocytes, p-MAPK accumulated at the spindle poles. This specific localization of p-MAPK was disrupted after RhoA inhibition.
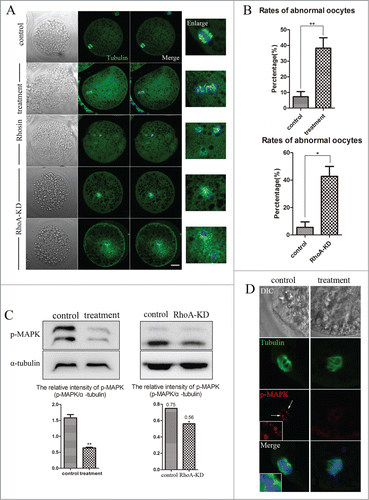
For determine the mechanism of RhoA for meiotic spindle assembly, the localization and expression of p-MAPK, a well-known regulator of microtubules, were determined. shows that p-MAPK expression was significantly reduced after Rhosin treatment or RhoA KD. The relative intensity of p-MAPK protein expression (p-MAPK/α-tubulin) confirmed the significant decrease in p-MAPK expression (Rhosin treated oocytes: 1.58 ± 0.10 vs. 0.64 ± 0.02; P < 0.01, RhoA KD oocytes: 0.75 vs. 0.56; P < 0.05). Fluorescence staining results showed that p-MAPK primarily accumulated at the spindle poles in control MI oocytes, whereas inhibiting RhoA activity resulted in the failure of p-MAPK specific localization, as only a few signals were on spindle microtubules (). Thus, RhoA was essential for the expression and localization of p-MAPK during meiosis.
RhoA localization in porcine embryos
To determine whether RhoA was expressed in embryos, parthenogenetically activated oocytes were used. RhoA protein localization was examined in the following samples: zygotes, and 2-, 4-, 8-cell and morula embryos. As shown in , RhoA was distributed in the nuclear area and, in particular, RhoA appeared to be enriched in the cortex region similar to the distribution of cortical actin filament layers irrespective of the embryo stage. An enlarged view showed RhoA accumulation at the cleavage furrow of a 2-cell embryo, which co-localized with actin.
Figure 6. RhoA protein localization in parthenogenetically activated oocytes, zygotes, and cleaving embryos. RhoA protein subcellular localization during porcine parthenogenetic embryo development. During mitotic cytokinesis, RhoA protein accumulated in the nuclear region. RhoA was also concentrated at the cortex, and co-localized with actin from the zygote to morula stage. White arrow indicates its localization in a cleavage furrow. Gray, DIC; green, RhoA; red, actin. Bar = 20 μm.
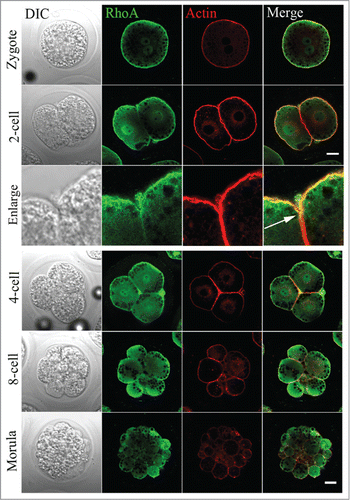
RhoA inhibition affects the early cleavage of porcine embryos
To investigate a role for RhoA on embryo development, zygotes that were produced by the parthenogenic activity of MII oocytes were immediately cultured in vitro in medium that was supplemented with Rhosin to monitor their ability to undergo cleavage divisions. These results showed that early porcine embryo development was disrupted after RhoA inhibition (). For controls, 95.02 ± 0.22% (n = 121) of MII oocytes developed to the 2-cell stage, whereas the developmental rates declined to 90.94 ± 1.04% (n = 122; P < 0.05), 71.14 ± 4.92% (n = 125; P < 0.05), and 14.93 ± 5.48% (n = 104; P < 0.01) with, respectively, 10 μM, 50 μM, and 100 μM Rhosin. In addition, 86.56 ± 2.71% of MII control oocytes developed to the 4-cell stage, whereas the developmental rates declined to 73.83 ± 3.18% (P < 0.05), 39.9 ± 1.74% (P < 0.01), and 4.51 ± 1.05% (P < 0.01) with, respectively, 10, 20, and 50 μM Rhosin. After culture for 72 h, 32.13 ± 1.61% of control MII oocytes developed to the 8-cell stage, whereas this declined sharply to 12.35 ± 4.44% (P < 0.05) and 0.94 ± 0.94% (P < 0.01) with 10 and 50 μM Rhosin. None of the zygotes that were cultured with 100 μM Rhosin cleaved to the 8- cell stage. These results showed that RhoA inhibition resulted in early embryo development failure in a dose-dependent manner. Thus, RhoA was critical for the early cleavage of porcine embryos.
Figure 7. RhoA inhibition affects embryos’ mitotic divisions. (A) Schematic diagram of first cleavage, second cleavage, and third cleavage in controls and undeveloped stage in treated embryos. RhoA inhibition affected the cleavage of zygotes. Most control embryos cleaved normally, whereas cleavage was blocked in treated embryos, particularly those treated with 100 μM inhibitor. Bar = 20 μm. (B) Morphology of embryos derived from zygotes treated with Rhosin. For embryos that were treated with Rhosin, 2 nuclei were found in each blastomere. RhoA inhibition resulted in aberrant cytokinesis rather than karyokinesis defects. Blue, chromatin; gray, DIC. Bar = 20 μm. (C) Rates of abnormal embryos after Rhosin treatment. The percentage of abnormal embryos was significantly increased for treated oocytes (P < 0.01).
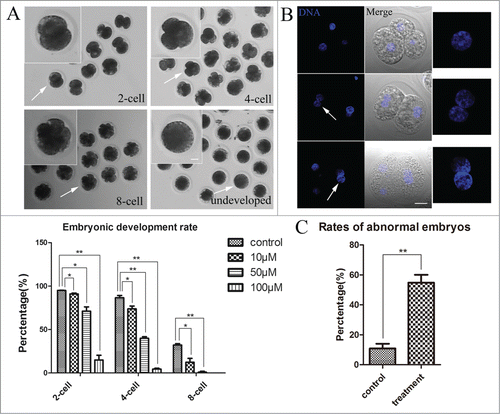
To determine a possible reason for these mitotic division defects in embryos, embryo morphology was assessed by immunofluorescence staining. As shown in , a nucleus was found in the cytoplasm of each cell in a control 4-cell embryo. However, although embryos derived from zygotes treated with Rhosin did not exhibit morphologically different nuclei from those of control embryos, 2 nuclei were found in the cytoplasm of one blastomere each of these arrested 2-cell and 4-cell embryos. Subsequently, we determined the abnormal rates of embryos, which showed that only 10.89 ± 3.12% of control zygotes had abnormal nuclei, whereas this rate was significantly increased to 54.80 ± 5.27% (P < 0.01) for treated zygotes (). These observations suggested that after RhoA inhibition, karyokinesis of mitotic division was successfully completed, whereas cytokinesis was severely disrupted. Thus, RhoA inhibition may have caused cytokinesis failure in dividing embryos.
RhoA inhibition effects on actin expression in embryos
To investigate a possible mechanism for cell division failure by porcine embryos, we investigated actin microfilaments, which are regulatory molecules involved in cytokinesis. The results in show that there were changes in actin expression in embryos that were cultured with Rhosin. For controls, actin cytoskeleton expression in cleaving embryos was relatively higher than that of treated embryos. An analysis of the fluorescence intensity curve for the line drawn across embryos also confirmed this (). These results demonstrated that RhoA was critical for actin assembly in porcine embryos.
Figure 8. RhoA inhibition affects actin cytoskeleton expression. (A) Embryos that were cultured with Rhosin exhibited pronounced changes in actin expression, as actin expression was reduced after RhoA inhibition. In control embryos, actin expression was normal both at the cortex and in the cytoplasm. After RhoA inhibition, actin expression was irregular and weakened both at the cortex and in the cytoplasm. Bar = 20 μm. (B) Actin fluorescence intensity curve for the line shown in oocytes. Actin fluorescence intensity was significantly weaker in the Rhosin treatment group. Red, actin. Bar = 20 μm.
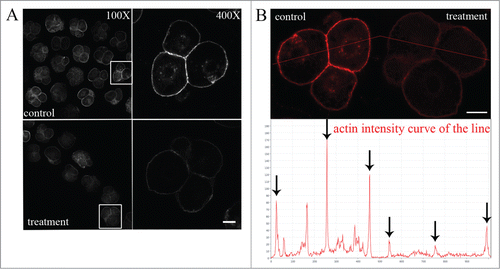
Discussion
Asymmetric cytokinesis is a unique characteristic of meiotic divisions in mammalian oocytes, and ultimately produces oocytes with the appropriate ploidy polar body size. Our present study employs the porcine system, which shares much similarity to human oocytes and is the better animal model for human reproduction to investigate the possible functions and mechanisms of a Rho GTPase, RhoA, during oocyte meiotic maturation and embryo development. The results showed that inhibiting RhoA activity affected polar body extrusion, spindle positioning, actin assembly, the formation of actin caps and CGFD's, and spindle organization. Our results suggest that RhoA is crucial for regulating the cytoskeleton mediated via ROCK/p-Cofilin, ARP2, and p-MAPK during porcine oocyte meiosis. Additionally, inhibiting RhoA activity affected embryos’ mitotic divisions and actin assembly. Thus, our results indicated important roles for RhoA in porcine oocytes and embryos.
RhoA plays pivotal roles in polar body emission and cytokinesis of porcine oocytes and embryos
Although it has been confirmed that RhoA is involved in establishing cell polarity and regulating cell divisions in somatic cells, its role and localization in porcine oocytes and embryonic cells has remained unclear. We first determined whether RhoA was expressed in porcine oocytes and embryos. Our results showed that RhoA exhibited specific localizations in porcine oocytes and embryos. In particular, RhoA accumulated exclusively at the cortex and also localized at the microtubules of meiotic spindles in fully-grown oocytes. We also found RhoA localization in the nucleus and the cortex as well as cleavage furrows in early embryos at mitosis. Similar localization patterns were reported in HeLa cells,Citation23 epithelial cells,Citation24 sea urchin eggs,Citation25 and Xenopus embryos.Citation26 Furthermore, the localization pattern of RhoA is similar with that in mouse oocyte concentrating in the midbody of telophase spindle.Citation27 The localization pattern of RhoA in porcine oocytes and embryos suggested that it was involved in actin and spindle-related cellular processes.
To confirm our hypothesis, we disrupted RhoA activity, which resulted in the cumulus expansion defects, the failure of oocyte polar body extrusion and embryo cleavage. Cumulus expansion is critical for the maturation of mammalian oocytes, and this might be one reason for the failure of oocyte polar body extrusion after RhoA inhibition. Depletion of RhoA also showed the polar body extrusion defects. These findings were somewhat expected because inhibiting RhoA in HeLa cells disrupted the completion of cytokinesis or ectopic cleavage,Citation28 and its inactivation also resulted in multinucleate cell formation in murine T lymphoma EL4 cells,Citation29 which suggested that RhoA was necessary for cytokinesis in somatic cells. In addition, it was proposed that RhoA was pivotal for GVBD in mouse oocytes,Citation30 indicating its roles on the following polar body extrusion. Therefore, we suggest that RhoA activity may positively affect polar body emission of porcine oocytes and mitotic divisions of embryos.
RhoA regulates actin-dependent spindle positioning through its effects on ROCK, p-Cofilin, and ARP2 expression in porcine oocytes
To determine a possible reason for polar body extrusion defects after inhibiting RhoA activity, we examined actin cytoskeleton expression, which has been proved to play critical roles in porcine oocyte polarity formation and is critical for cytokinesis in animal cells.Citation31 A previous study confirmed that Rho GTPases were regulators of the cytoskeleton for supporting cellular morphological changes.Citation32 It was also recently reported that increased RhoA activity resulted in rearrangements of the cortical actin cytoskeleton, which is likely to be significant for chromosome segregation and the positioning of the cytokinetic furrow during cytokinesis.Citation33 In porcine oocytes, we found a significant block of actin cytoskeleton assembly of cytoplasmic actin coupled with cortical actin after RhoA activity inhibition and RhoA depletion, with similar results observed in porcine embryos. It was previously demonstrated that the cortical polarity of oocytes required Ran GTPase mediated actin regulation.Citation34 In addition to Ran, the Cdc42 protein regulates actin polymerization to promote polar body emission during meiosis.Citation35 Our results indicated that RhoA regulated porcine oocyte maturation and early embryo development through its regulation of actin assembly.
To further investigate the mechanisms of RhoA in porcine oocytes, we examined spindle positioning during oocyte meiotic maturation, as actin filaments are involved in peripheral meiotic spindle positioning during oocyte meiotic maturation.Citation4 A recent study confirmed that an actin flow drove spindles to migrate from the central to cortical area of oocytes, which resulted in oocyte asymmetric division.Citation34 Our results showed that those oocytes treated with Rhosin or knocked down of RhoA exhibited spindle positioning defects, which resulted in centrally arrested spindles. These results also indicated that the formation of actin caps and CGFD's, both features of oocyte polarization resulting from spindle migration, had failed after Rhosin treatment. Similar results were found with the Rho GTPases Rac and Cdc42, which were involved in regulating spindle positioning and polar body extrusion during mouse oocyte meiosis.Citation36 Furthermore, the effects of RhoA on spindle migration were similar to those other signaling molecules, including actin nucleation factor Arp2/3 complexes and Formin-2Citation37 in mouse oocytes. These molecules are downstream regulators of small GTPases and have been shown to regulate polar body extrusion and cytokinesis by regulating actin assembly during mouse oocyte meiosis.Citation38 Thus, our results suggest that RhoA participates in porcine oocyte polar body emission through regulating actin-mediated spindle positioning.
To determine a possible signaling pathway for RhoA activity inhibition on porcine polar body extrusion defects, we examined the expressions of ROCK, p-Cofilin, and ARP2, which were shown to regulate actin assembly in mouse oocyte.Citation6,39 Our results showed that ROCK, p-Cofilin, and ARP2 protein expressions all significantly decreased after inhibiting RhoA activity or depleting RhoA. Cofilin, a downstream molecule of ROCK, is a key regulator of actin filament dynamics and reorganization by stabilizing actin filament networks during mitosis.Citation40 Arp2/3 complexes are the most highly characterized nucleating components for regulating actin assembly.Citation41 Our previous work showed the involvement of ROCK, Arp2/3 in regulation actin assembly during porcine oocyte maturation.Citation42,43 The present study indicates that RhoA acts as the upstream regulator of ROCK, p-Cofilin and Arp2/3 in regulating actin assembly in porcine oocyte. Therefore, it suggests that RhoA might regulate actin assembly through 2 classes of substrates: ROCK-Cofilin and Arp2/3 complex.
RhoA affects meiotic spindle by regulating p-MAPK expression in porcine oocytes
To further investigate how RhoA inhibition perturbed polar body extrusion in porcine oocytes, we also examined meiotic spindle organization. In migrating fibroblasts, RhoA induces the formation of stable Glu-microtubules during cell migration . As a novel regulator of the MT cytoskeleton, RhoA selectively stabilizes MTs by modulating the dynamic and stable states of MTs during cell polarization.Citation22 It was also recently shown that Rho acted as a microtubule-dependent signal and regulated cytokinesis by post-mitotic spindle structures.Citation44 We showed that RhoA inhibition and RhoA KD both induced significantly increased rates of abnormalities in spindle morphology, which indicated disruption of meiotic spindle organization. The Rho GTPase Ran was confirmed to play crucial roles during spindle formation in vertebrate oocytes.Citation45 Rac and Cdc42 were also found to be involved in controlling spindle dynamics, as with the Ran GTPase.Citation12 Thus, our results suggest that RhoA affects spindle organization, which regulates polar body extrusion during porcine oocyte maturation.
To further investigate the mechanism of RhoA inhibition for spindle organization during meiosis, we examined the distribution and expression of p-MAPK. We found that p-MAPK expression and localization were disrupted after RhoA inhibition. RhoA KD oocytes also showed decreased p-MAPK expression. Mitogen-activated protein kinase (MAPK) is an important regulator of spindle formation during mouse and porcine oocyte maturation,Citation46.Previous study showed that RhoA signaling could activate MAPK.Citation47 Therefore, our results indicate that RhoA might regulate p-MAPK for its involvement in spindle formation during porcine oocyte meiosis.
In summary, RhoA regulates the expressions of ROCK, p-Cofilin, ARP2, and p-MAPK for its involvement in the actin assembly and spindle formation, which further affects porcine oocyte spindle positioning, polar body extrusion, and early embryo cleavage. Our results provided the mechanistic regulation roles of RhoA in mammalian oocytes and provided novel understanding and information for human reproduction.
Materials and Methods
Antibodies and chemicals
Rabbit polyclonal anti-RhoA antibody and Rabbit polyclonal anti-ARP2 antibody was from Santa Cruz Biotechnology Inc. (Santa Cruz, CA, USA). Rabbit polyclonal anti-phospho-MAPK antibody was purchased from Cell Signaling Technology (Beverly, MA, USA). Phalloidin-TRITC, Lectin-FITC, and mouse monoclonal anti-α-tubulin-FITC antibody were from Sigma-Aldrich Corp. Alexa Fluor 488 and 568 goat anti-rabbit IgG were purchased from Zhongshan Golden Bridge Biotechnology Co., Ltd. (Beijing). Rhosin was from Calbiochem (Darmstadt, Germany). Basic maturation culture medium was tissue culture medium (TCM-199) (St. Louis, MO, USA). PBS was obtained from Life Technologies (Invitrogen, Carlsbad, CA, USA). All other chemicals and reagents were from Sigma-Aldrich Corp. unless otherwise stated. Porcine follicular fluid (pFF) was aspirated from 3–6 mm follicles of ovaries. The fluid was collected and centrifuged at 2000 rpm for 30 min at 4°C, then stored at 20°C under sterile conditions before use.
Porcine oocyte isolation and culture
Porcine ovaries were collected from prepubertal gilts at a local slaughterhouse and transported to the laboratory in sterile saline (0.9% NaCl) containing 75 mg penicillin G/ml and 50 mg streptomycin sulfate/ml maintained at 37°C. Cumulus-oocyte complexes (COCs) were aspirated from medium-sized follicles (3–6 mm in diameter) of ovaries with a 20-gauge needle and a syringe. The COCs surrounded by a compact cumulus mass and also with uniform ooplasm were isolated from the cellular debris. After rinsesing 4 times in maturation medium: TCM-199 supplemented with 0.1% polyvinyl alcohol, 0.57 mM cysteine, 0.91 mM sodium pyruvate, 3.05 mM glucose, 75 mg/L of penicillin, 50 mg/L of streptomycin, 10% (v/v) pig follicular fluid (pFF), 10 ng/ml epidermal growth factor (EGF), 10 IU PMSG /ml, 10 IU hCG/ml. A group of 80 oocytes was cultured in 500 μl maturation medium in a 4-well dish (NUNC) at 38.5°C in an atmosphere of 5% CO2 and saturated humidity.
For evaluation of oocyte maturation, COCs were cultured for 44 h. After culturing, the COCs were treated with 0.02% (w/v) hyaluronidase (in TCM-199) for 5 min at 38°C. The oocytes were then separated from surrounding cumulus cells completely by pipetting gently with a fine-bore pipette. After 3–4 rinses, the cumulus-free oocytes were used for experiments. For the judgment of the maturation rate, a group of cumulus-free porcine oocytes were gathered together in a 100 μl TCM199 droplet, and then each oocyte was rotated several times one by one under a Nikon Diaphot ECLIPSE TE300 inverted microscope (Nikon UK. Ltd) for observation the polar body. Oocytes with clearly extruded polar bodies were judged as matured oocytes.
Parthenogenetic activation for production porcine embryos in vitro
For obtaining the cleaving embryos at desired stages of development, matured MII oocytes were applied for electrical stimulation (EST) to product the parthenogenetic activation embryos in vitro. The denuded MII oocytes were placed in a chamber filled with activation medium (0.3 M mannitol, 0.05 mM CaCl2 and 0.1 mM MgCl2 and 0.1% BSA) for 3 min. Ten to 15 oocytes were then moved into an electrode wire chamber each time; an electrical pulse of 0.63 kV/cm, 80 μs, and 1 DC was employed to activate oocytes. After electrical activation, oocytes were washed 3 times and finally transferred to 500 μl of porcine zygote medium 3 (PZM-3) containing 0.4% BSA in a 4-well for embryo culture. Two-cell embryos were obtained 36–42 h, 4-cell embryos 48–62 h and 8-cell embryos 63–67 h after EST.
Treatment of porcine oocytes and embryos with Rhosin in vitro
RhoA inhibitor, Rhosin was employed to inhibit the activity of intracellular RhoA during oocytes meiosis. Rhosin was diluted to 50 mM stock solution in dimethyl sulfoxide (DMSO) and stored at −20°C. For each culture, the stock solution was diluted with TCM199 to reach final concentrations of 50, 100 and 200 μM, and oocytes were treated with the maturation medium containing Rhosin for 44 h to obtain the MII oocytes. Then oocytes were washed 3 times and used for immunofluorescent staining. Control oocytes were treated with the same concentration of DMSO in the medium before examination. The GV oocytes were incubated in maturation medium containing 200 μM Rhosin for 26 h to obtain the MI stage oocytes for the examination of actin assembly, spindle assembly and positioning.
The 50 mM stock solution was diluted with PZM-3 to get final concentrations of 10, 50 and 100 μM. After EST, 30–40 parthenogenetic activation oocytes were cultured in 500 μl of PZM-3 medium containing Rhosin at 38.5°C in humidified air with 5% CO2. The developmental capability of embryos was recorded at Day 1, 2 or 3 when embryos should reach the 2-cell, 4-cell or 8-cell stage. After completion of culture, the distribution of RhoA in the embryos and the formation of microfilaments were examined by immunofluorescence staining described below. The control parthenogenetic activation embryos were cultured with the same concentration of DMSO.
RhoA siRNA injection
For depleting RhoA, 3 target-specific small interfering double-stranded RNA oligomers (siRNAs) (Gene Pharma, Shanghai, China) were used for RNAi. The target region of each potential siRNA was blasted against all available porcine sequences in GenBank. COCs were selected and denuded, subsequently; the microinjection was performed with 50 mM RhoA siRNA using an Eppendorf FemtoJet (Eppendorf AG) with a Nikon Diaphot ECLIPSE TE300 inverted microscope (Nikon UK. Ltd) equipped with a Narishige MM0–202 N hydraulic 3-dimensional micromanipulator (Narishige Inc. Tokyo, Japan). Approximately 10–20 pl of RhoA siRNA solution was microinjected into the cytoplasm of denuded GV porcine oocyte. The same amount of negative control siRNA was microinjected as control. Approximately 250 oocytes were injected in each group.
Microinjected oocytes were rinsed with TCM-199, then culture in TCM-199 maturation medium supplemented with 5 μM milrinone for 24 h to facilitate RhoA mRNA translation KD. Subsequently, The GV porcine oocytes was washed 3 times (2 min each wash) in milrinone-free fresh TCM-199 medium, and then transferred to fresh TCM-199 maturation medium and cultured another 26 or 44 h to determine the MI stage or the maturation status (pbI extrusion) at 38.5°C in a 5% CO2 atmosphere, respectively. Actin expression, spindle and chromosome morphology, and chromosome localization were examined.
Immunofluorescent staining and confocal microscopy
For immunofluorescence staining of RhoA, α-tubulin, actin, p-MAPK, p-Cofilin, cumulus-free oocytes or embryos at various culture times or after activation treatment were fixed in 4% paraformaldehyde (in PBS) for 30 min at room temperature and then permeabilized in permeabilization solution (1% Triton X-100 in PBS) for 8–12 h at room temperature. To suppress nonspecific binding of IgG, oocytes were blocked in blocking buffer for 1 h at room temperature (1% BSA-supplemented PBS), For RhoA staining, they were incubated overnight with 1:100 dilution rabbit polyclonal anti-RhoA antibodies (1:100 p-MAPK, 1:100 p-Cofilin) at 4°C. After 3 washes (2 min each) in washing buffer (0.1% Tween 20 and 0.01% Triton X-100 in PBS), they were labeled with 1:100 dilution Alexa Fluor 488/568 goat-anti-rabbit IgG for 1 h at room temperature. For α-tubulin-FITC staining, the oocytes were incubated with 1:400 dilution anti-α-tubulin-FITC antibodies for 2 h at room temperature. For actin staining, the oocytes or embryos were incubated with Phalloidin-TRITC (5 μg/ml in PBS) for 1 h at room temperature. After 3 additional washes in washing buffer, they were co-stained with Hoechst 33342 (10 μg/ml in PBS) 10 min for chromosome examination.
After staining, the oocytes were mounted on glass slides and examined with a Confocal Laser-Scanning Microscope (Zeiss LSM 700 META, Germany).
Fluorescence and western band intensity analysis
Actin fluorescence intensity was analyzed by the Image J software. The samples of control and treatment groups were mounted on the same glass slide. After immunofluorescent staining, the average fluorescence intensity per unit area within the region of interest (ROI) of immunofluorescence images was determined. Independent measurements using identically sized ROIs were taken for the cell membrane and cytoplasm. The average values of all measurements were used to compare the final average intensities between control and treatment groups.
For quantification of the western blot results, intensity values of bands were measured using the Image J. The intensity of control bands (ROCK:α-Tubulin, ARP2:α-Tubulin,p-MAPK:α-Tubulin or p-Cofilin:α-Tubulin) was defined was set to 1. Three different replicates were used for the analysis.
Protein extraction and protein gel blot analysis
A total of 100 porcine oocytes at MI stage were collected, lysed in Laemmli sample buffer (SDS sample buffer and 2-Mercaptoethanol) and boiled at 100°C for 10 min. After being cooled on ice and centrifuged at 12 000 × g for 4 min, samples were frozen at −20°C until use. Proteins were separated by sodium dodecyl sulfate-polyacrylamide gel electrophoresis (SDS-PAGE) with a Criterion precast gel (Bio-Rad, Richmond, CA, USA). After electrophoretic separation, proteins were electrophoretically transferred onto a polyvinylidene fluoride membrane (Millipore, Billerica, MA) for 1.5 h at 100 V at 4°C using a semidry blotting system. To avoid nonspecific binding, membranes were blocked 2.5 h with Tris-buffered saline (TBS) containing 0.1% (w/w) Tween 20 (TBST) and 5% (w/v) nonfat dry milk powder except 5% BSA of p-Cofilin for 2 h at room temperature. The membrane was simultaneously incubated with rabbit polyclonal anti-RhoA (1:200, Santa Cruz, USA), rabbit polyclonal anti-ROCK (1:200, Santa Cruz, USA), rabbit polyclonal anti-ARP2 (1:200, Santa Cruz, USA), rabbit anti-p-MAPK (1:2000; Cell Signaling Technology, Danvers, MA, USA) or rabbit monoclonal anti-p-Cofilin (1:1000; Cell Signaling Technology, Danvers, MA, USA) primary antibodies at overnight 4°C, respectively. The incubation buffer was 2% BSA in TBST except 5% BSA of p-Cofilin. Subsequently, after being washed 3 times in TBST (10 min each), membranes were treated in horseradish peroxidase (HRP) conjugated anti-rabbit IgG (1:2000; Cell Signaling Technology, Beverly, MA, USA) for 2 h at room temperate. Finally, the membranes were washed 3 times in TBST and then the specific proteins were visualized using chemiluminescence reagent (Millipore, Billerica, MA). Equal protein loading was confirmed by the detection of α-tubulin (rabbit monoclonal anti-α-tubulin antibody;1:2000;Cell Signaling Technology, Danvers, MA, USA). This experiment was repeated at least 3 times using different samples.
Data Analysis
Each experiment was performed at least 3 times and at least 30 oocytes or embryos were examined each time. Analysis of variance (ANOVA) was adopted for statistical analyses. Differences between treated groups were evaluated with the Duncan multiple comparison test. Data are expressed as mean ± SEM's. A value of P < 0.05 is considered to be statistically significant.
Disclosure of Potential Conflicts of Interest
No potential conflicts of interest were disclosed.
Funding
This work was supported by the National Basic Research Program of China (2014CB138503), the Natural Science Foundation of Jiangsu Province (BK20130671, BK20140030), and the Biogreen 21 Program (PJ009594, PJ009080 and PJ00909801), RDA, Republic of Korea.
References
- Deng M, Kishikawa H, Yanagimachi R, Kopf GS, Schultz RM, Williams CJ. Chromatin-mediated cortical granule redistribution is responsible for the formation of the cortical granule-free domain in mouse eggs. Dev Biol 2003; 257:166-76; PMID:12710965; http://dx.doi.org/10.1016/S0012-1606(03)00045-9
- Brunet S, Verlhac MH. Positioning to get out of meiosis: the asymmetry of division. Hum Reprod Update 2011; 17:68-75; PMID:20833637; http://dx.doi.org/10.1093/humupd/dmq044
- Verlhac MH, Dumont J. Interactions between chromosomes, microfilaments and microtubules revealed by the study of small GTPases in a big cell, the vertebrate oocyte. Mol Cell Endocrinol 2008; 282:12-7; PMID:18191018; http://dx.doi.org/10.1016/j.mce.2007.11.018
- Sun SC, Kim NH. Molecular mechanisms of asymmetric division in oocytes. Microsc Microanal 2013; 19:883-97; PMID:23764118; http://dx.doi.org/10.1017/S1431927613001566
- Sun SC, Xu YN, Li YH, Lee SE, Jin YX, Cui XS, Kim NH. WAVE2 regulates meiotic spindle stability, peripheral positioning and polar body emission in mouse oocytes. Cell Cycle 2011; 10:1853-60; PMID:21543895; http://dx.doi.org/10.4161/cc.10.11.15796
- Sun SC, Wang ZB, Xu YN, Lee SE, Cui XS, Kim NH. Arp2/3 complex regulates asymmetric division and cytokinesis in mouse oocytes. PLoS One 2011; 6:e18392; PMID:21494665; http://dx.doi.org/10.1371/journal.pone.0018392
- Leader B, Lim H, Carabatsos MJ, Harrington A, Ecsedy J, Pellman D, Maas R, Leder P. Formin-2, polyploidy, hypofertility and positioning of the meiotic spindle in mouse oocytes. Nat Cell Biol 2002; 4:921-8; PMID:12447394; http://dx.doi.org/10.1038/ncb880
- Dumont J, Million K, Sunderland K, Rassinier P, Lim H, Leader B, Verlhac MH. Formin-2 is required for spindle migration and for the late steps of cytokinesis in mouse oocytes. Dev Biol 2007; 301:254-65; PMID:16989804; http://dx.doi.org/10.1016/j.ydbio.2006.08.044
- Nishimura Y, Mabuchi I. An IQGAP-like protein is involved in actin assembly together with Cdc42 in the sea urchin egg. Cell Motil Cytoskeleton 2003; 56:207-18; PMID:14584023; http://dx.doi.org/10.1002/cm.10146
- Benink HA, Bement WM. Concentric zones of active RhoA and Cdc42 around single cell wounds. J Cell Biol 2005; 168:429-39; PMID:15684032; http://dx.doi.org/10.1083/jcb.200411109
- Ma C, Benink HA, Cheng D, Montplaisir V, Wang L, Xi Y, Zheng P-P, Bement WM, Liu XJ. Cdc42 activation couples spindle positioning to first polar body formation in oocyte maturation. Curr Biol 2006; 16:214-20; PMID:16431375; http://dx.doi.org/10.1016/j.cub.2005.11.067
- Na J, Zernicka-Goetz M. Asymmetric positioning and organization of the meiotic spindle of mouse oocytes requires CDC42 function. Curr Biol 2006; 16:1249-54; PMID:16782018; http://dx.doi.org/10.1016/j.cub.2006.05.023
- Cui XS, Li XY, Kim NH. Cdc42 is implicated in polarity during meiotic resumption and blastocyst formation in the mouse. Mol Reprod Dev 2007; 74:785-94; PMID:17154294; http://dx.doi.org/10.1002/mrd.20571
- Deng M, Suraneni P, Schultz RM, Li R. The Ran GTPase mediates chromatin signaling to control cortical polarity during polar body extrusion in mouse oocytes. Dev Cell 2007; 12:301-8; PMID:17276346; http://dx.doi.org/10.1016/j.devcel.2006.11.008
- Halet G, Carroll J. Rac activity is polarized and regulates meiotic spindle stability and anchoring in mammalian oocytes. Dev Cell 2007; 12:309-17; PMID:17276347; http://dx.doi.org/10.1016/j.devcel.2006.12.010
- Zhang X, Ma C, Miller AL, Katbi HA, Bement WM, Liu XJ. Polar body emission requires a RhoA contractile ring and Cdc42-mediated membrane protrusion. Dev Cell 2008; 15:386-400; PMID:18804436; http://dx.doi.org/10.1016/j.devcel.2008.07.005
- Aepfelbacher M, Heesemann J. Modulation of Rho GTPases and the actin cytoskeleton by Yersinia outer proteins (Yops). Int J Med Microbiol 2001; 291:269-76; PMID:11680787; http://dx.doi.org/10.1078/1438-4221-00130
- Etienne-Manneville S, Hall A. Rho GTPases in cell biology. Nature 2002; 420:629-35; PMID:12478284; http://dx.doi.org/10.1038/nature01148
- Kishi K, Sasaki T, Kuroda S, Itoh T, Takai Y. Regulation of cytoplasmic division of Xenopus embryo by rho p21 and its inhibitory GDP/GTP exchange protein (rho GDI). J Cell Biol 1993; 120:1187-95; PMID:8436590; http://dx.doi.org/10.1083/jcb.120.5.1187
- Bement WM, Benink HA, von Dassow G. A microtubule-dependent zone of active RhoA during cleavage plane specification. J Cell Biol 2005; 170:91-101; PMID:15998801; http://dx.doi.org/10.1083/jcb.200501131
- Wojnacki J, Quassollo G, Marzolo MP, Caceres A. Rho GTPases at the crossroad of signaling networks in mammals: Impact of Rho-GTPases on microtubule organization and dynamics. Small GTPases 2014; 5:e28430; PMID:24691223
- Cook TA, Nagasaki T, Gundersen GG. Rho guanosine triphosphatase mediates the selective stabilization of microtubules induced by lysophosphatidic acid. J Cell Biol 1998; 141:175-85; PMID:9531557; http://dx.doi.org/10.1083/jcb.141.1.175
- Yuce O, Piekny A, Glotzer M. An ECT2-centralspindlin complex regulates the localization and function of RhoA. J Cell Biol 2005; 170:571-82; PMID:16103226; http://dx.doi.org/10.1083/jcb.200501097
- Yonemura S, Hirao-Minakuchi K, Nishimura Y. Rho localization in cells and tissues. Exp Cell Res 2004; 295:300-14; PMID:15093731; http://dx.doi.org/10.1016/j.yexcr.2004.01.005
- Nishimura Y, Nakano K, Mabuchi I. Localization of Rho GTPase in sea urchin eggs. FEBS letters 1998; 441:121-6; PMID:9877178; http://dx.doi.org/10.1016/S0014-5793(98)01531-2
- Drechsel DN, Hyman AA, Hall A, Glotzer M. A requirement for Rho and Cdc42 during cytokinesis in Xenopus embryos. Curr Biol 1997; 7:12-23; PMID:8999996; http://dx.doi.org/10.1016/S0960-9822(06)00023-6
- Zhong ZS, Huo LJ, Liang CG, Chen DY, Sun QY. Small GTPase RhoA is required for ooplasmic segregation and spindle rotation, but not for spindle organization and chromosome separation during mouse oocyte maturation, fertilization, and early cleavage. Mol Reprod Dev 2005; 71:256-61; PMID:15791586; http://dx.doi.org/10.1002/mrd.20253
- O'Connell CB, Wheatley SP, Ahmed S, Wang YL. The small GTP-binding protein rho regulates cortical activities in cultured cells during division. J Cell Biol 1999; 144:305-13; PMID: 9922456; http://dx.doi.org/10.1083/jcb.144.2.305
- Moorman JP, Bobak DA, Hahn CS. Inactivation of the small GTP binding protein Rho induces multinucleate cell formation and apoptosis in murine T lymphoma EL4. J Immunol 1996; 156:4146-53; PMID:8666781
- Cheon YP, Kim SW, Kim SJ, Yeom YI, Cheong C, Ha KS. The role of RhoA in the germinal vesicle breakdown of mouse oocytes. Biochem Biophys Res Commun 2000; 273:997-1002; PMID:10891361; http://dx.doi.org/10.1006/bbrc.2000.3052
- Robinson DN, Spudich JA. Towards a molecular understanding of cytokinesis. Trends Cell Biol 2000; 10:228-37; PMID:10802538; http://dx.doi.org/10.1016/S0962-8924(00)01747-5
- Condeelis J. Life at the leading edge: the formation of cell protrusions. Annu Rev Cell Biol 1993; 9:411-44; PMID:8280467; http://dx.doi.org/10.1146/annurev.cb.09.110193.002211
- Maddox AS, Burridge K. RhoA is required for cortical retraction and rigidity during mitotic cell rounding. J Cell Biol 2003; 160:255-65; PMID:12538643; http://dx.doi.org/10.1083/jcb.200207130
- Yi KX, Li R. Actin cytoskeleton in cell polarity and asymmetric division during mouse oocyte maturation. Cytoskeleton 2012; 69:727-37; PMID:22753278; http://dx.doi.org/10.1002/cm.21048
- Leblanc J, Zhang X, McKee D, Wang ZB, Li R, Ma C, Sun QY, Liu XJ. The small GTPase Cdc42 promotes membrane protrusion during polar body emission via ARP2-nucleated actin polymerization. Mol Hum Reprod 2011; 17:305-16; PMID:21511720; http://dx.doi.org/10.1093/molehr/gar026
- Dehapiot B, Carriere V, Carroll J, Halet G. Polarized Cdc42 activation promotes polar body protrusion and asymmetric division in mouse oocytes. Dev Biol 2013; 377:202-12; PMID:23384564; http://dx.doi.org/10.1016/j.ydbio.2013.01.029
- Dumont J, Million K, Sunderland K, Rassinier P, Lim H, Leader B, Verlhac MH. Formin-2 is required for spindle migration and for the late steps of cytokinesis in mouse oocytes. Dev Biol 2007; 301:254-65; PMID:16989804; http://dx.doi.org/10.1016/j.ydbio.2006.08.044
- Yi K, Rubinstein B, Unruh JR, Guo F, Slaughter BD, Li R. Sequential actin-based pushing forces drive meiosis I chromosome migration and symmetry breaking in oocytes. J Cell Biol 2013; 200:567-76; PMID:23439682; http://dx.doi.org/10.1083/jcb.201211068
- Duan X, Liu J, Dai XX, Liu HL, Cui XS, Kim NH, Wang ZB, Wang Q, Sun SC. Rho-GTPase effector ROCK phosphorylates cofilin in actin-meditated cytokinesis during mouse oocyte meiosis. Biol Reprod 2014; 90:37; PMID:24429217; http://dx.doi.org/10.1095/biolreprod.113.113522
- Kaji N, Muramoto A, Mizuno K. LIM kinase-mediated cofilin phosphorylation during mitosis is required for precise spindle positioning. J Biol Chem 2008; 283:4983-92; PMID:18079118; http://dx.doi.org/10.1074/jbc.M708644200
- Pollard TD, Cooper JA. Actin, a central player in cell shape and movement. Science 2009; 326:1208-12; PMID:19965462; http://dx.doi.org/10.1126/science.1175862
- Zhang Y, Duan X, Xiong B, Cui XS, Kim NH, Rui R, Sun SC. ROCK inhibitor Y-27632 prevents porcine oocyte maturation. Theriogenology 2014; 82:49-56; PMID:24681214; http://dx.doi.org/10.1016/j.theriogenology.2014.02.020
- Wang F, An GY, Zhang Y, Liu HL, Cui XS, Kim NH, Sun SC. Arp2/3 complex inhibition prevents meiotic maturation in porcine oocytes. PLoS One 2014; 9:e87700; PMID:24498171; http://dx.doi.org/10.1371/journal.pone.0087700
- Kamijo K, Ohara N, Abe M, Uchimura T, Hosoya H, Lee JS, Miki T. Dissecting the role of Rho-mediated signaling in contractile ring formation. Mol Biol Cell 2006; 17:43-55; PMID:16236794; http://dx.doi.org/10.1091/mbc.E05-06-0569
- Wollman R, Cytrynbaum EN, Jones JT, Meyer T, Scholey JM, Mogilner A. Efficient chromosome capture requires a bias in the 'search-and-capture' process during mitotic-spindle assembly. Curr Biol 2005; 15:828-32; PMID:15886100; http://dx.doi.org/10.1016/j.cub.2005.03.019
- Fan HY, Sun QY. Involvement of mitogen-activated protein kinase cascade during oocyte maturation and fertilization in mammals. Biol Reprod 2004; 70:535-47; PMID:14613897; http://dx.doi.org/10.1095/biolreprod.103.022830
- Frost JA, Xu SC, Hutchison MR, Marcus S, Cobb MH. Actions of Rho family small G proteins and p21-activated protein kinases on mitogen-activated protein kinase family members. Mol Cell Biol 1996; 16:3707-13; PMID:8668187