Abstract
Tetraspanin CD151 interacts with laminin-binding integrins (i.e., α3β1, α6β1 and α6β4) and other cell surface molecules to control diverse cellular and physiological processes, ranging from cell adhesion, migration and survival to tissue architecture and homeostasis. Here, we report a novel role of CD151 in maintaining the branching morphogenesis and activity of progenitor cells during the pubertal development of mammary glands. In contrast to the disruption of laminin-binding integrins, CD151 removal in mice enhanced the tertiary branching in mammary glands by 2.4-fold and the number of terminal end buds (TEBs) by 30%, while having minimal influence on either primary or secondary ductal branching. Consistent with these morphological changes are the skewed distribution of basal/myoepithelial cells and a 3.2-fold increase in proliferating Ki67-positive cells. These novel observations suggest that CD151 impacts the branching morphogenesis of mammary glands by upregulating the activities of bipotent progenitor cells. Indeed, our subsequent analyses indicate that upon CD151 removal the proportion of CD24HiCD49fLow progenitor cells in the mammary gland increased by 34%, and their proliferating and differentiating activities were significantly upregulated. Importantly, fibronectin, a pro-branching extracellular matrix (ECM) protein deposited underlying mammary epithelial or progenitor cells, increased by >7.2-fold. Moreover, there was a concomitant increase in the expression and nuclear distribution of Slug, a transcription factor implicated in the maintenance of mammary progenitor cell activities. Taken together, our studies demonstrate that integrin-associated CD151 represses mammary branching morphogenesis by controlling progenitor cell activities, ECM integrity and transcription program.
Abbreviations
ECM | = | extracellular matrix |
TEB | = | terminal end bud |
LB | = | laminin-binding |
EMT | = | epithelial-mesenchymal transition |
Introduction
Distinct from other branched organs, the development of mammary organs in mice is largely a postnatal process.Citation1-3 Shortly after birth, epithelial stem and/or progenitor cells within the mammary gland actively divide, differentiate, and invade into the fat pad to form a tree-like tubular network consisting of hierarchized ductal and lobular systems.Citation2 While the primary ducts extend through the continuous bifurcations of terminal end buds (TEBs), the lobular structures, comprised of secondary and tertiary ducts or branches, are derived from the lateral branching of trailing ducts.Citation1,2 All ductal and lobular structures consist of an inner layer of luminal cells and an outer layer of basal or myoepithelial cells. The actively proliferating cap/basal cells in TEBs are potential progenitors for myoepithelial cells, while the body cells within the TEB are the precursors of luminal cells.Citation4 Upon reproduction, the pouched structures at the end of lobules further develop into milk-producing alveoli structures. Thus, mammary gland development in mice is accompanied by extensive branching morphogenesis and activation of pluripotent or bipotent stem/progenitor cells.
Cell-extracellular matrix (ECM) interactions are crucial for the branching morphogenesis or outgrowth of mammary glands.Citation3,5,6 It is of no surprise that integrins, heterodimer receptors for ECMs, have been extensively implicated in regulating mammary gland development.Citation6–8 Notably, removal of β1 integrin in the basal cell compartment leads to complete abrogation of branching morphogenesis.Citation9 In contrast, deletion of β1 integrin in luminal epithelial cells only impairs cellular differentiation into lobuloalveolar structures.Citation10,11 Consistent with these observations are the similar defects in the mammary glands found in mice depleted of fibronectin, a ligand for α5β1 integrin, or major downstream signaling effectors such as FAK and ILK.Citation8,12,13 However, given the fact that β1 subunit associates with more than 10 different α subunits to constitute functional adhesion receptors,Citation14 the effects of disrupting β1 integrin or its downstream effectors may actually reflect the combined effects of multiple impaired integrins. In addition, members of the integrin family are functionally distinct from each other or their roles vary with cell or tissue type.Citation7,14 Thus, more studies are needed to define the specific roles of individual integrins during mammary gland development.
Despite being crucial for maintaining the basal or myoepithelial cell interaction with the underlying basement membrane, laminin-binding (LB) integrins, consisting of α3β1, α6β1 and α6β4, have received limited attention for their roles in mammary gland development. This is because mice with targeted deletion of α3 or α6 integrin are postnatally lethal.Citation15–17 In fact, early studies on these integrins involving the transplantation of rudimentary mammary glands from the embryos of gene-targeted mice revealed little change in the outgrowth of mammary gland.Citation18 In a recent study, however, mice with a mammary gland-specific deletion of α3 integrin under the control of CK5 promoter exhibited a marked defect in the function of myoepithelial cells and lactation.Citation19 In addition, α6 integrin, also known as CD49f, is a potential contributor to mammary gland development, as it has been extensively linked to the dynamics of mammary epithelial stem cells.Citation20,21 Moreover, transplantation of a CD24MedCD49fHi subpopulation, which has a medium level of CD24 but a high level of CD49f expression, has been shown to be capable of regenerating the entire mammary gland in recipient mice, while the CD24HiCD49fLow fraction only gives rise to lobuloalveolar structures.Citation20,22 Hence, available studies consistently support crucial roles of LB integrins in the development of mammary glands.
We and others have recently shown that CD151, a member of the tetraspanin family, is a master regulator of LB integrins at the molecular, cellular and tissue levels.Citation23–26 Structurally, CD151 is physically associated with the α3 or α6 subunit of LB integrins via protein-protein interactions.Citation27 At the cellular level, CD151 mediates LB integrin-dependent cell-ECM or cell-cell adhesion, motility, survival and invasion.Citation23,25 Targeted deletion or genetic mutation of CD151 partially impairs LB integrin-mediated tissue architecture and physiological functions.Citation28–30 Such in vivo function of CD151 appears to be linked to its ability to regulate branching morphogenesis and ECM dynamics in multiple cell types, including epithelial and endothelial cells.Citation24,31 While mice carrying the whole-body deletion of either α3 or α6 integrin are postnatally lethal,Citation7 the mice with whole-body deletion of the CD151 gene are viable and fertile, and exhibit a mild strain-dependent defect in kidney function.Citation24 Consequently, the availability of CD151-targeted mice provides a unique opportunity to evaluate how CD151 regulates tissue morphogenesis in vivo, particularly during normal mammary gland development.
Here, we describe a novel role of CD151 in mammary gland development and the dynamics of progenitor cells. We discovered that loss of CD151 led to a marked increase in tertiary branching in mammary glands and enhanced the proliferating and differentiating activities of bipotent progenitor cells. Consistent with these changes are the marked increase in the deposition of ECM proteins, particularly fibronectin, and the elevated expression of transcription factor Slug. Our study, for the first time, provides evidence that CD151 contributes to mammary gland development by maintaining the integrity of ECM niches and by sustaining the quiescence of progenitor cells at both cellular and transcriptional levels.
Results
CD151 removal alters the branching morphogenesis of the mammary gland
In our recent study, mice expressing rat ErbB2 oncogene exhibited a marked alteration in the architecture of mammary glands upon CD151 removal.Citation24 Following this observation, we subsequently investigated the role of CD151 in the pubertal development of mammary glands by conducting whole-mount analysis of CD151- knockout mice. As shown in , the outgrowth of mammary glands was markedly enhanced in 6 week-old CD151-KO mice compared to those of control littermates, validating our contention that CD151 is a critical player in mammary gland development.Citation24 Notably, there was a 2-fold increase in tertiary branching in the absence of CD151 (). In addition, the number of TEBs increased by 24% in CD151-deficient mice (). Changes in secondary branching or ductal elongation did not reach statistical significance upon CD151 removal (). Consistent with these observations were the similar changes in the mammary glands of 7 and 7.5 week old mice as well as H&E analyses of mammary tissue sections (). Particularly, CD151-deficient 7.5 week old mice exhibited an earlier appearance of lobuloalveolar-like structures, compared to their control counterparts (). Moreover, the changes in the mammary branching morphogenesis observed with CD151-targeted FVB mice are also apparent in mice carrying deletion of single CD151 allele or CD151 KO mice on mixed Sv129/FVB background (data not shown). In contrast, mice with the deletion of TSPAN12, another tetraspanin present in mammary tissues,Citation32,33 revealed minimal difference in the outgrowth of mammary gland (data not shown). Together, our whole-mount analyses demonstrate a specific repressing role of tetraspanin CD151 during the pubertal branching morphogenesis of mammary glands in mice.
Figure 1. (A) Role of CD151 in the branching morphogenesis of mammary glands in mice. (A) Representative images of whole-mounted inguinal mammary glands collected from 6 week old CD151 wild-type (WT) and knock-out (KO) female virgin mice. CD151-targeted FVB mice were used to generate mouse littermates. Scale bar: 1 mm. (B) Changes in ductal lengthand number of secondary and tertiary branches in mammary glands upon CD151 removal (values: mean ± SEM, n = 4). *: P value <0.05. (C) Representative images of whole-mounted mammary glands collected from 7 and 7.5 week old female virgin mice. Scale bar: 1 mm. (D) Typical H&E staining of mammary tissue sections from 7 week old female virgin mice. Scale bar: 500 μm. For (C and D), 2–3 mice per genotype were analyzed at each time point.
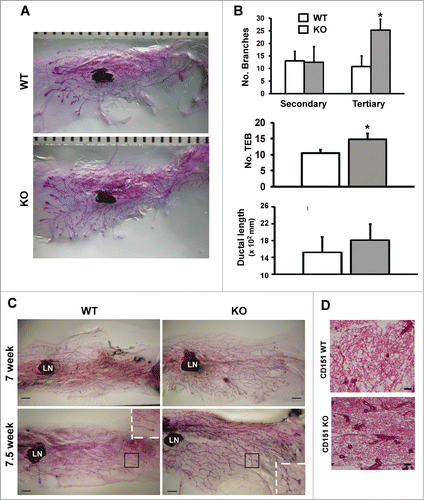
Loss of CD151 skews the distribution of basal or myoepithelial cells in the mammary gland
We next evaluated the changes in cellular composition or subpopulations in mammary tissues upon CD151 removal. As shown in , expression of CK5 and CK14, 2 well-recognized markers for basal or myoepithelial cells,Citation34 exhibited 2.5 to 8.6-fold increase in the absence of CD151. By comparison, estrogen receptor (ER), a representative marker for luminal cells, showed little change. Together, these observations imply the presence of excessive basal epithelial cells in CD151-deficient mammary glands. Indeed, upon CD151 removal the percentages of CK14- and CK5-positive cells in mammary glands increased from about 45% to 84%, according to IHC analyses (p < 0.05) (). Furthermore, the number of Ki67-positive cells in the TEBs of CD151-deficient mammary glands increased by nearly 3-fold (), while there was minimal change in cell survival as assessed by IHC analyses with an antibody against cleaved Caspase-3 (data not shown). Moreover, our staining of consecutive mammary tissue sections revealed that intense Ki-67 staining largely occurred in body cells in the TEBCitation2 (), as evidenced by the expression of β-catenin at cell-cell contacts (). A small number of cap or basal cells, which resided outside body cells,Citation2 were also Ki67-positive (). Together, these results indicate that the increased branching in mammary glands upon CD151 removal is coupled with increased proliferative activities of progenitor cells within the TEB structure.
Figure 2. CD151 removal skews the distribution and proliferation of body and basal/cap or myoepithelial cells in murine mammary glands. (A) Expression of epithelial cell lineage-related genes in mammary tissues. Values indicate the relative fold changes in protein expression as determined by densitometry. Blotting for β-actin protein served as a loading control. (B) Typical IHC images of mammary tissue sections were stained with antibodies against cytokeratin 5 (CK5) or cytokeratin 14 (CK14). Staining is shown for CK5 (a-d) and CK14 (e-h) for WT (a, c, e and g) and CD151-null (b, d, f and h), respectively. (C) Representative images of IHC staining of Ki67 antigen in TEBs (a-b). Ki67 staining for WT (a) and CD151 KO mice (b). Consecutive sections of CD151-null mammary tissues were stained for Ki67 (c) and β-catenin (d), respectively. Bottom panel: Percentages of Ki67-positive cells. Values were determined by estimating the average numbers of Ki67-positive cells in 5 representative fields of stained tissue section (mean ± SEM, n = 3). *: P value <0.05. For (A–C), 7 week-old mice per genotype (n = 3) were analyzed. Scale bars for B & C: 50 μm.
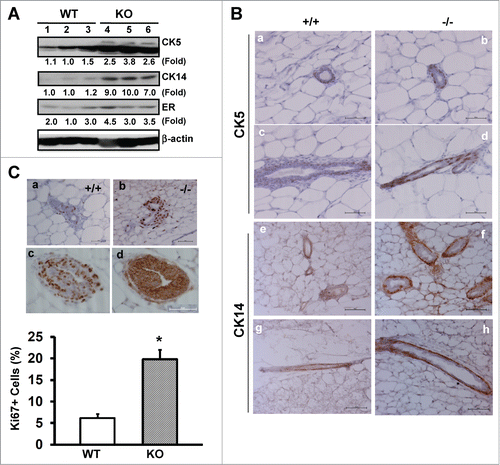
A role of CD151 in maintenance of mammary progenitor cells
There is growing evidence that CD24MedCD49fHi and CD24HiCD49fLow subpopulations are enriched in stem and progenitor cells, contributing to the branching morphogenesis of mammary glands.Citation34 To gain mechanistic insight into the impact of CD151 ablation on mammary branching morphogenesis, we isolated these 2 subpopulations from purified lineage-negative (Lin−) populations in murine mammary glands by flow cytometry (). While little change occurred in the CD24MedCD49fHi fraction upon CD151 deletion, the proportion of CD24HiCD49fLow fraction within the Lin− population increased by 30% (), implying a marked effect of CD151 removal on the dynamics of luminal progenitor cells. Following this lead, we next examined the impact of CD151 removal on the acinar structure-forming capabilities of mammary luminal progenitor cells in 3D culture. As shown in , the number and dynamics of acinar-like structures generated by CD24HiCD49fLow luminal progenitor cells were markedly altered in the absence of CD151. Particularly, the portion of hollow colonies, which reflects the differentiation potential of progenitor cells, exhibited a nearly 2-fold increase, while the average size of acinar-like structures was reduced (). By comparison, there was little change in the formation of mammosphere (data not shown). Furthermore, there was a marked increase in the portion of Ki67-positive cells in the acinar-like colonies formed by CD151-deficient CD24HiCD49fLow cells (). Similar changes were also detected for SMA- and CK5-positive cells (). In addition, we detected a significant increase in the proportion of Ki67-positive cells in tumors arising from mammary progenitor cells in MMTV-Wnt1 mice upon CD151 removal (data not shown). Together, these novel observations indicate that the proliferating and differentiating activities of mammary luminal progenitor cells in TEBs are simultaneously affected upon CD151 removal, implying a potential disruption of the quiescent state of mammary progenitor cells.
Figure 3. For figure legend, see page 2713.Figure 3 (See opposite page). Effect of CD151 removal on distribution, proliferation and differentiation of luminal progenitor cells in mammary glands. (A) Left panel: Typical plots of cell subpopulations within the Lin− population residing in mammary glands. Lin− populations were prepared from10–14 week old FVB/Sv129 mice and then sorted on flow cytometry using a combination of FITC or APC-conjugated monoclonal antibodies against CD24 and CD49f antigens. Right panel: Average portions of CD24MedCD49fHi and CD24HiCD49fLow subpopulations within Lin− populations prepared from CD151 WT and null mouse littermates (mean ± SEM, n = 3). (B) Left panel: Representative images of acinar-like colonies formed by CD24Hi CD49fLow subpopulation after 3 weeks of 3D culture. Right panel: Changes in the size, number and percentage of solid and hollow colonies (n = 5). (C and D) Staining of Ki67 or smooth muscle actin (SMA) or cytokeratin 5 (CK5) in the acinar-like colonies formed by the CD24HiCD49fLow fraction prepared from CD151 WT or KO mice. Green/Red: Antibody staining. Blue: DAPI for nuclei. Percentages of Ki67-positive cells were determined microscopically in multiple representative fields. For (B and C), values (mean ± SEM) were calculated from 3 independent experiments. For (A–C), *: P value <0 .05, **: P value <0.01. All images and measurements in (B–D) are representative of at least 3 independent experiments. Scale bars for (B–D): 50 μm.
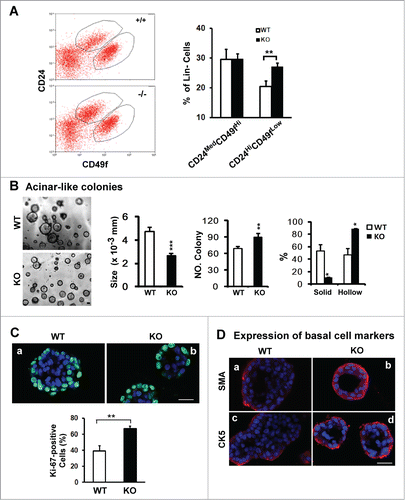
CD151 removal alters ECM composition and FAK activation in mammary tissues
To gain mechanistic insight into the alterations at both tissue and cellular levels (), we evaluated the impact of CD151 removal on integrin-mediated cell-ECM interactions, as they are consistently implicated in driving the formation of lobuloalveolar structures during mammary gland development.Citation35,36 As shown in , expression of fibronectin, and to a lesser extent, tenascin C, exhibited a marked increase in mammary tissues upon CD151 removal. Also, the expression of fibronectin in TEBs was markedly elevated (, bottom panel). A similar change in fibronectin expression was detected in CD151-deficient luminal progenitor cells under 3D culture, as well as human myoepithelial cells (MCF-10A) or carcinoma cells (SUM-149) with CD151 knockdown under 2D or 3D culture (). In addition, the level of laminin-511 (LN-10) was markedly elevated in MCF-10A cells. By comparison, the deposition of laminin-332 (LN-5) only exhibited a small decrease in the absence of CD151 (). In line with these changes in the ECM composition was an increased activation of FAK, as reflected by a 2-fold increase in the phosphorylation at the Y397 residue (). In comparison, there was little change in Src kinase activation, expression or distribution of α3β1 and α5β1 integrins upon CD151 deletion (). Together, these results suggest that CD151 contributes to mammary gland development by maintaining ECM-based niches of epithelial progenitor cells, but not by controlling the expression or distribution of α3 or α5 integrin.
Figure 4. For figure legend, see page 2715.Figure 4 (See opposite page). Impact of CD151 removal on the ECM expression and distribution in murine mammary glands and progenitor cells. (A) ECM proteins in murine mammary glands. Top panel: Protein analyses were performed with mammary tissues from CD151 wild-type (+/+) or null (−/−) mice (n = 3) and fold changes were indicated. Bottom panel: IHC staining of fibronectin in mammary tissues of CD151 WT and KO mice. (B) Distribution of ECM proteins surrounding acinar-like structures formed by murine mammary CD24HiCD49fLow subpopulations under 3D culture. Fibronectin (FN): a-b. laminin-332 (LN-5): c-d. CD151: e-f. Red: Antibody staining; Blue: DAPI for nuclei. CD151 WT: a, c and e; CD151 KO: b, d and f. The CD24HiCD49fLow subpopulation was prepared from 10–14 week old mice as described in Figure 3. (C) Changes in expression and distribution of ECM proteins, i.e., laminin-332 (LN-5), laminin-511 (LN-10), collagen I or fibronectin (FN) and integrins in cultured human breast epithelial cell lines (SUM-149, MCF-10A). Left panel, immunoblotting of FN, CD151, α3 integrin and β-actin in SUM-149 and MCF-10A cell lines with or without CD151 knockdown (CT, KD). Right panel, CD151 knockdown effect on ECM composition in MCF-10A cells. All IF staining of ECM molecules (red) or DAPI (blue) for nuclei were visualized and imaged under confocal microscope. Scale bars for (A–C): 50 μm.
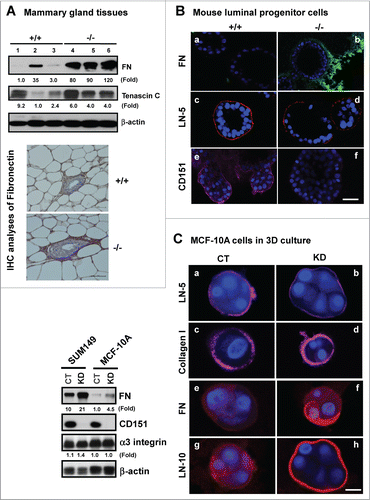
Figure 5. Effect of CD151 deletion on integrin expression and signaling in murine mammary glands. (A and B), Lysates of mammary tissues from 3 individual mice per genotype were analyzed with antibodies against phospho-specific or total protein for FAK or Src kinases (A) or against α3 or α5 integrin (B). Fold changes in the ratio of Y397/total FAK protein or pY416/total Src or integrins were calculated on the basis of densitometry measurements. (C)Representative images of α3 and α5 integrin distribution in mouse mammary tissues. IHC analyses were conducted with rabbit polyclonal antibodies against α3 (a-b) or α5 integrin (c-d) with mammary tissues from CD151 WT (a, c) or KO mice (b, d) (n = 3). Scale bar: 50 μm.
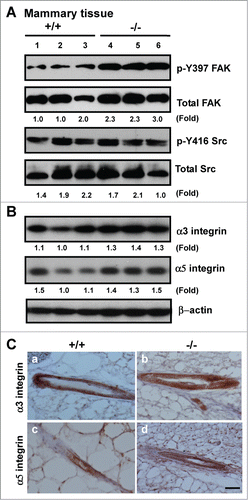
CD151 removal coincides with the induction of transcription factor Slug
Increased fibronectin expression and FAK activation frequently reflect epithelial-mesenchymal transition (EMT)-like changes during normal development or tumor progression.Citation36–38 We therefore evaluated changes in the expression of EMT-inducing transcription factors upon CD151 removal, particularly Snail and Slug, which have been previously linked to CD151 function as well as the dynamics of mammary epithelial stem/progenitor cells or mammary gland development.Citation39,40 As shown in , expression of Slug, but not Snail, increased more than 3-fold at the protein level in the absence of CD151. IHC analyses showed that the number of cells with the nuclear expression of Slug protein increased by 2.5-fold in TEBs (). Slug staining was also restricted to the cap or basal cell layer (), consistent with a recent report on the distribution of this molecule in murine mammary tissue.Citation39 Collectively, these results implicate an intrinsic link between CD151 and Slug in mammary epithelial progenitor cells.
Figure 6. For figure legend, see page 2718.Figure 6 (See previous page). Suppression of the expression and activation of transcription factor Slug and TGF-β signaling by CD151. (A) Expression of Snail and Slug proteins and TGF-β-mediated signaling in mammary tissues. Mammary tissues from 3 individual mice per genotype were blotted with the indicated antibodies. MMP-2 served as a loading control. (B) Representative images of Slug distribution in mammary glands. IHC staining was conducted with tissues from CD151 WT (a, c) or KO (b, d) mice (n = 3). Scale bar: 50 μm. (C) Changes in Slug expression in human mammary epithelial cells upon CD151 ablation. Human mammary epithelial cells (HCC-1187 and SUM-149) with (KD) or without CD151 knockdown (CT) were lysed and blotted for Slug, CD151, α3 integrin and β-actin. (D) Effect of CD151 removal on Slug protein stability. Top panel, Scatter plot of fold changes in Slug protein over time. MCF-10A cells with or without CD151 knockdown were treated with 20 μg/mL cycloheximide over the indicated periods of time and followed by lysing in RIPA buffer and blotting with indicated antibody. (E) Proliferation indices of human mammary progenitor-like epithelial cells (HCC-1187, SUM-149) with (KD) or without CD151 knockdown (CT) over 48 hr period of culture (n = 4). *: P value <0.05. (A–D): Fold changes were calculated on the basis of densitometry measurements.
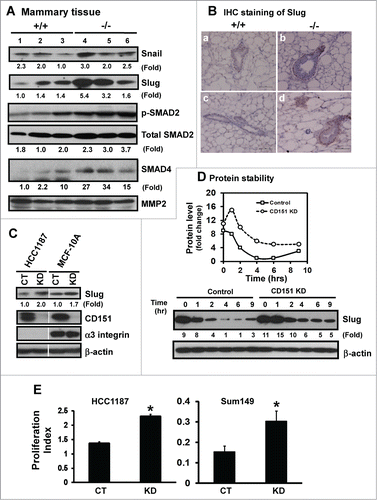
Next, we evaluated whether CD151 directly regulates Slug expression by knocking down CD151 in mammary myoepithelial cell-derived non-tumorigenic (MCF-10A) and tumorigenic (HCC-1187) cancer cell lines. As shown in , CD151 ablation led to a nearly 2-fold increase in the Slug protein in both MCF-10A and HCC-1187 lines. Furthermore, our cycloheximide-based pulse-chase analyses showed that the half-life of the Slug protein was doubled in the absence of CD151 (). Also, the proliferation of human HCC-1187 and SUM-149 cells were markedly enhanced upon CD151 ablation (), consistent with the role of Slug in the self-renewal of mammary progenitor cells.Citation39 These observations indicate that CD151 may restrict the tertiary branching of mammary glands largely by repressing the expression and/or activation of Slug.
In addition to Slug, TGF-β has recently been linked to the EMT-like changes resulted from CD151 removal.Citation41 Consistent with this notion was a marked increase in the active form of SMAD2, as well as expression of total SMAD4, upon CD151 removal (). However, there was little difference in the nuclear localization of phopho-SMAD2, according to IHC analyses of mammary epithelial tissues (data not shown). In addition, CD151 absence appeared to enhance the sensitivity of mammary epithelial cells to TGF-β stimulation, rather than constitutively elevating TGF-β-mediated signaling (data not shown). Collectively, our available observations support a direct role of Slug, rather than TGF-β-mediated signaling, in CD151-mediated mammary gland development and maintenance of progenitor cells.
The impact of CD151 removal on the branching morphogenesis of mammary glands is associated with diminished expression of Ron
We then explored the possibility that CD151 may collaborate with receptor tyrosine kinase Ron during mammary gland development, as deletion of this molecule has a similar impact on the branching morphogenesis as seen in CD151-targeted mice.Citation42 As shown in , expression of Ron protein was markedly diminished in mammary tissues in the absence of CD151. In line with this observation was a marked decrease in the phosphorylation at the Y654 residue of β-catenin upon CD151 removal as described in a prior study,Citation42 while activation of GSK-3β, crucial for Wnt signaling, remained unaffected Citation43,44 (). Similarly, we found that signaling of Ron through Erk1/2 and Akt in human mammary carcinoma cells was markedly impaired upon CD151 ablation (). In contrast, expression of Slit-2 and Robo-1, both shown to enhance branching morphogenesis in mice upon deletion as seen in CD151-targeted mice,Citation45 exhibited a minimal change (). Together, these results indicate that CD151 may collaborate with Ron to affect the pubertal development of mammary glands in mice.
Figure 7. (A) Link between CD151 and receptor tyrosine kinase Ron in mammary epithelial cells. (A) Expression of Ron, Slit2, Robo1 and related signaling molecules in mammary glands. Blotting was conducted with tissues collected from CD151 WT (+/+) and KO (−/−) mice (n =3). (B) Altered signaling of Ron kinase. Human breast carcinoma cell lines (HCC-1937 and T47D), with and without CD151 ablation, were starved and subsequently stimulated with 30 ng/mL MSP, a ligand for Ron, followed by lysing in RIPA buffer and blotting with indicated signaling antibodies. (C) A working model of CD151 function during pubertal development of mammary glands.
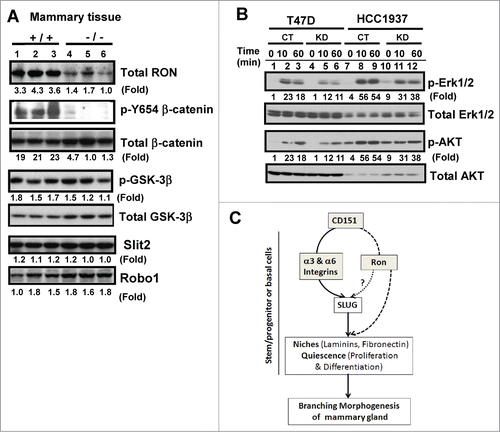
Discussion
Here, we provide evidence that CD151, a member of the tetraspanin family, regulates the normal development of the mammary gland and dynamics of progenitor cells in mice during puberty. Rather than disrupting various integrins or their signaling effectors or ligands,Citation7 deletion of CD151 in mice enhanced branching morphogenesis, particularly the tertiary branching and led to the earlier development of the lobuloalveolar structure. Such developmental alterations were strongly associated with increased number of progenitor cells in TEBs, as well as skewed distribution of basal or myoepithelial cells in the gland. In support of these changes were the enhanced differentiating and proliferating activities of progenitor cells enriched in CD24HiCD49Low subpopulation in the absence of CD151. In addition, there was an increased deposition of fibronectin and a significant increase in the expression and nuclear presence of Slug in CD151-deficient mammary tissues. Moreover, expression and signaling of Ron was impaired in the absence of CD151. Together, these observations support a model in which CD151 suppresses branching morphogenesis of mammary glands by controlling the ECM niches and activity of luminal progenitor cells, as well as activation of Ron and Slug ().
Involvement of LB integrins in CD151-mediated branching morphogenesis of mammary gland
Even though data from our current study support a strong role of CD151 in mammary gland development, the extent of LB integrin involvement in this process remains unclear. Several lines of observation implicate a limited role of these receptors in CD151-mediated outgrowth of mammary glands. First, CD151 deletion had only a minimal effect on the survival of mammary epithelial cells, which has been linked to the function of α6 integrin by prior studies.Citation24,46 Second, we detected little change in mammary stem cell fraction upon CD151 removal (), even though α3 integrin has recently been implicated in regulating the niches of adult epithelial stem cells.Citation47 Third, while targeted deletion of α3 integrin affects both the contractile activity of myoepithelial cells and related lactation processes,Citation19 removal of CD151 appears to have minimal impact on lactation or litter survival (data not shown). Lastly, there was little change in the expression and distribution of α3 integrin upon CD151 removal (). In these regards, our data implicate a minimal influence of α3 or α6 or both integrins on the outgrowth of CD151-deficient mammary glands as implicated in a prior transplantation-based analysis.Citation18
On the other hand, our data on the changes in non-laminin ligands, such as fibronectin, and FAK activation upon CD151 removal (), suggest that the impact of CD151 removal on mammary gland development is linked to the abrogation of the α3 integrin-dependent transdominancy over other integrins.Citation48 In fact, non-LB integrins, such as α2β1, αvβ3 and α5β1integrins, have been implicated in driving the branching morphogenesis of mammary glands.Citation7 Intriguingly, in the absence of CD151, there was little or minimum change in the expression or distribution of α5 integrin, a primary receptor for fibronectin (). Perhaps, the phenotypic change in mammary gland upon CD151 removal is only associated with the altered signaling of integrins, rather than their expression or distribution.
CD151 as a potential functional regulator of mammary epithelial progenitor cells
One of the surprising observations in our current study is the intrinsic link between CD151 and the dynamics of mammary epithelial luminal progenitor cells featured as CD24HiCD49fLow subpopulation. Such functional connection is likely related to the ability of CD151 to maintain the integrity of the ECM niche, while repressing Slug activation (). In fact, both fibronectin and Slug have been consistently implicated in driving luminal progenitor cell proliferation and lobuloaveolar structure formation as part of the development of the mammary gland.Citation35,39,49 Based on these observations, we speculate that CD151 removal induces Slug expression in the luminal progenitor cells, and subsequently increases the expression of Slug target genes, including fibronectin and tenascin C. As a result of such genetic re-programing, progenitor cells escape from ECM-mediated constraint or quiescent state to acquire greater proliferating and differentiating activities, thereby enhancing tertiary branching of the mammary gland. In this manner, CD151 negatively regulates the formation of lobuloalveolar structures during mammary gland development, in contrast to the effect of disrupting fibronectin, FAK or β1 integrin.Citation11,35,37
CD151 mediates normal development of mammary glands by repressing EMT-like changes
Another unexpected finding in our study is the potential role of CD151 in EMT-like changes, as reflected by the increased expression of fibronectin and Slug upon CD151 removal (). The effect on Slug activation is quite intriguing, as this transcription factor is not only known to induce the EMT Citation40 but also regulate the expression of LB integrins.Citation50 More recently, Slug has been strongly implicated in driving the proliferation of luminal progenitor cells during the outgrowth of the mammary gland,Citation39 consistent with our findings on the impact of CD151 removal (). These available observations raise a strong possibility that there is a reciprocal interaction between CD151-LB integrin complexes and Slug in mammary luminal progenitor cells.
The impact of CD151 removal on the EMT-like process during mammary development may also be attributed to the marked upregulation in TGF-β-mediated signaling detected in our study. This observation is consistent with a recent study on the in vivo effect of CD151 removal in another epithelial tissue.Citation41 Our unpublished data, however, do suggest that altered TGF-β-mediated signaling in mammary tissues may not stem from a direct effect of CD151. Rather, it may result from CD151-mediated activation of Slug.
A potential functional link of Ron kinase to CD151-mediated mammary gland development
Results from our current study also raise the likelihood that CD151 may mediate mammary gland development through Ron. A recent study has reported that Ron represses branching morphogenesis in the mammary gland,Citation42 largely reminiscent of the role of CD151 described in current study (). However, our Brij-96 detergent-based co-IP analyses failed to recapture the physical complexes between CD151 and Ron in murine mammary tissues (data not shown), despite a physical link between Ron and LB integrins reported previously.Citation51 Nonetheless, our available data favor the notion that CD151 acts collaboratively or along the same pathway with Ron to regulate the normal development of mammary glands and the dynamics of progenitor cells. The ultimate validation of the close relationship between CD151 and Ron during mammary glands development, however, may come from the analyses of the compound mice deleted of both genes.
Our data also reveal a minimal influence of CD151 deletion on the expression of Slit-2 or Robo-1 in mammary tissues (), even though both of these molecules have recently been shown to repress the pubertal outgrowth of mammary glands.Citation45 In this regard, the skewed distribution of basal or myoepithelial cells detected in CD151-targeted mice may be partially attributed to the altered function of Ron, rather than Slit-2/Robo-1 receptor complexes ().
Implications for a potential role of CD151 in human breast cancer
The unexpected suppressive roles of CD151 in the branching morphogenesis in the mammary gland, as well as the dynamics of progenitor cells, ignite the possibility of CD151 as a tumor suppressor of human triple-negative breast cancer, particularly for those arising from basal or luminal progenitor cells. Such implication is in sharp contrast with CD151's tumor-promoting role reported by many previous studies,Citation26,52 in which CD151 appears to drive breast cancer progression largely through promoting cell survival in the context of constitutively active Ras/MAPK pathways.Citation53,54 In this regard, the tumor-suppressing function of CD151 may take place in an oncogenic context-dependent manner. Furthermore, the notion of CD151 as a tumor suppressor is supported by multiple clinical analyses of this molecule in several other types of human cancer, including bladder, endometrial and possibly colon cancer.Citation55–57 Hence, as a multi-faceted molecule, the functional role of CD151 during cancer development is likely linked to the cellular origin or oncogenic context of tumor-initiating cells as implicated for many adhesion molecules including integrins and their downstream effectors.Citation58–61
In summary, our current study demonstrates that tetraspanin CD151 contributes to the pubertal development of mammary glands by maintaining the normal activity of adult epithelial progenitor cells and ECM homeostasis. Our results also indicate that CD151 may regulate ECM niches, as well as proliferating and differentiating activities of progenitor cells, by collaborating with Ron and repressing Slug. For the first time, we demonstrate a direct functional link of a tetraspanin molecule to mammary gland development and dynamics of progenitor cells at both the signaling and transcriptional levels. Such findings also provide novel implications for the function of CD151-integrin complexes in the onset and development of stem/progenitor cell-originated breast cancer.
Materials and Methods
Mice
CD151-targeted mice on Sv129 background were obtained from Dr. Martin Hemler (Dana-Farber Cancer Institute and Harvard Medical School, Boston MA).Citation62 Mouse mammary tumor virus (MMTV)-Wnt1 mice were purchased from The Jackson Laboratory (Bar Harbor, ME). TSPAN12-targeted FVB mice were obtained from the Mutant Mouse Regional Resource Center (UC Davis, CA). Mouse littermates used for whole-mount experiments included the offspring of the intercrossing of CD151+/− FVB/Sv129 mice which were then backcrossed onto FVB background for 3 to 8 consecutive generations as described in our prior study.Citation24 Genotyping was conducted at the beginning and end of in vivo experiments for CD151 or TSPAN12 or Wnt1 gene. Mice were humanely sacrificed by CO2 inhalation. All experiments were performed in accordance to protocols approved by the Institutional Animal Care and Use Committee of the University of Kentucky.
Antibodies, reagents and cell culture
Biotinylated or fluorescence-conjugated rat monoclonal antibodies against mouse CD31, CD45, Ter-119, CD24, Sca1, CD29 and CD49f, were purchased from BD Biosciences (San Jose, CA) or R&D Systems (Minneapolis, MN). The biotinylated rat anti-mouse CD140a antibody was obtained from eBioscience (San Diego, CA). Antibodies for Cytokeratin (CK) 5/6 and CK-14 were purchased from Covance (Princeton, NJ) and CK-18 from Abcam (Cambridge, MA). The antibodies against smooth muscle actin (SMA) and Ki67 were obtained from Sigma-Aldrich (St. Louis, MO) and Thermo Fisher Scientific (Waltham, MA), respectively. Antibodies to Snail, Slug, cleaved Caspase 3 and β-catenin, as well as to total and/or phosphorylated forms of GSK-3β, AKT and Erk1/2, were obtained from Cell Signaling Technology (Danvers, MA). The antibody to Y654-β-catenin was purchased from Antibodies-Online (Atlanta, GA). Antibodies for Slit2 and ROBO1 were obtained from Abcam (Cambridge, MA). The antibody against Ron receptor kinase was obtained from Santa Cruz Biotechnology (Santa Cruz, CA). Antibodies to human integrins and tetraspanins were raised in house.Citation63 Antibodies against α5 integrin- or human laminin α5 chains were purchased from Millipore (Billerica, MA). Rabbit polyclonal antibody against human α3 cytoplasmic tail was raised in house.Citation64 Regular and Growth Factor-Reduced Matrigel were purchased from BD Biosciences (San Jose, CA). Growth factors and hormones, including bFGF, EGF and prolactin, were obtained from R&D Systems (Minneapolis, MN).
All epithelial cells prepared from mouse mammary glands were cultured in mammary epithelial basal medium (MEBM) (Lonza, Basel, Switzerland) supplemented with B27 mixture (Life Technologies, Carlsbad, CA), bFGF and EGF. Immortalized human myoepithelial (MCF-10A) and breast cancer cell lines (Sum-149, T47D, HCC-1187 and HCC-1937) were purchased from ATCC (Manassas, VA) and cultured in defined medium Citation63 or RPMI-1640 medium (Life Technologies, Carlsbad, CA) supplemented with 10% FBS. All cell cultures were performed at 37°C and 5% CO2.
Isolation of epithelial subpopulations from murine mammary glands by cell sorting
The lineage negative (Lin−) population was prepared essentially as described.Citation20,21,65 In brief, mammary glands from 8–12 week-old CD151 WT and KO virgin mouse littermates were surgically removed, minced, then digested for 2 h at 37°C in 10 mL of 5% FBS-containing DMEM/F12 medium supplemented with 300 U/ml type I collagenase (Worthington Biochemical, Lakewood, NJ) and 100 U/ml hyaluronidase (Sigma-Aldrich, St. Louis, MO). The resultant organoid mixtures were washed with 2% FBS and 2 mM EDTA in HBSS (HF medium) (Life Technologies, Carlsbad, CA). After centrifugation, cell pellets were re-suspended in 2–3 mL of pre-warmed 0.25% Trypsin/EDTA (Life Technologies, Carlsbad, CA) and incubated at RT for 2–3 min with frequent pipetting prior to being neutralized with 10 ml of cold HF medium and centrifugation. Newly obtained cell pellets were suspended in HF medium containing 5 mg/mL dispase (Life Technologies, Carlsbad, CA) and 0.1 mg/mL DNAase for 5 min at RT and followed by lysis of red-blood cells in AKC buffer. The resultant single cell suspension was subsequently blocked with 10 μg/mL Fc receptor-specific antibody at 4°C for 20 min prior to incubation with a cocktail of biotin-labeled rat mAb cocktails against CD45, Ter119, CD31 and CD140a at 10 μg/mL. The cell-antibody mixtures were then incubated with streptavidin-conjugated magnetic beads (Life Technologies, Carlsbad, CA) at 4°C for 45 min to remove unwanted cells. The resulting Lin− cells were collected and then incubated with a combination of CD24-FITC, CD49f-APC or CD29-PE conjugated or Sca1-PE. The stained Lin− cells were then fractioned into subpopulations using a MoFlo cell sorter (Becton Dickinson). During sorting, all gates were set to exclude 99.9% of cells labeled with isoform-matched control antibodies conjugated with the corresponding fluorochromes.
Mammosphere formation and cell proliferation and differentiation assays under 3D culture
To assay self-renewal capacity, purified CD24HiCD49fLow cells were seeded into 24-well ultra-low attachment plates at a density of 10000 cells/well. Cells were allowed to develop into mammospheres (sphere-like structures) over a span of 5–7 daysCitation65 before imaged microscopically. Cell proliferation and differentiation capacities of progenitor cells were determined by initially growing cells under 3D culture according to the protocol previously described.Citation21,66 In brief, sorted mouse mammary epithelial cells were seeded at 10000 cells/well and grown under growth factor-reduced Matrigel for 7 d and followed by staining with antibodies against Ki67 or various lineage-related markers prior to imaging under confocal microscope. The size, number and morphologies of mammospheres or colonies were quantified by analyzing the photographed representative fields using Nikon NIS-Elements Advanced Analysis Software as described in our prior study.Citation24 Proliferation of human HCC-1187 or SUM-149 cells varying in CD151 expression was evaluated by MTT assay.
Whole-mount analyses of mammary gland and tissue collection
Fourth and/or ninth inguinal mammary glands from 6 week old female virgin CD151+/+ and CD151−/− mice were surgically removed for morphological and developmental analyses. Mammary glands were mounted onto glass slides and then fixed in Carnoy's solution for 4 h or overnight at RT. Glands were rehydrated in a graded series of ethanol and then transferred into carmine alum stain for 48 h at RT. Glands were washed in a graded series of ethanol and then cleared in xylenes (Sigma-Aldrich, St. Louis, MO). Glands were transferred into methyl salicylate (Sigma-Aldrich, St. Louis, MO) prior to photographic documentation. Glands were viewed under a dissecting microscope and images were captured by a Sony Cyber-shot DSC-H20 digital camera. Ductal length and quantity of secondary and tertiary branches were quantified from the images of representative whole-mounted inguinal mammary glands with Nikon NIS-Elements AR software. Ductal elongation was measured as the distance between the center of the lymph node (LN) and the most distal terminal end bud (TEB) at the leading edge of the mammary fat pad. For branching analyses, all secondary and tertiary ducts bifurcating from the longest primary duct either directly above or below the lymph node, depending upon visibility, were measured. For quantification purposes, a secondary duct was defined as any duct that bifurcated from the primary duct, whereas a tertiary duct was defined as any duct that bifurcated from a secondary duct.
Additional tissues from the third and/or fifth axillary mammary glands and kidney were also removed and frozen for immunoblotting analyses or fixed in 10% neutral formalin (Sigma-Aldrich, St. Louis, MO). Fixed kidney tissues were then rinsed and stored in 70% ethanol for hematoxylin and eosin (H&E) or immunohistochemistry (IHC) analyses to verify the CD151 phenotype.
Cell sorting, flow cytometry, transfection, immunofluorescence (IF), and IHC analyses
Stable knockdown of >90% of CD151 proteins in SUM-149 and HCC-1937 cells were carried out as previously describedCitation63 and confirmed by subsequent flow cytometry or immunoblotting with anti-CD151 monoclonal antibodies (5C11 and 1A5). To evaluate the expression of cell surface or intracellular proteins, cells grown under 3D culture, cells remained intact or fixed in 4% paraformaldehyde prior to staining with antibodies.Citation63 For IF analyses of ECM proteins, cells were stained alive on ice with antibodies and then fixed in 2% paraformaldehyde, according to protocols previously described.Citation63,67
IHC analyses were carried out according to the protocol previously described.Citation63 In brief, after antigen retrieval in 0.1% EDTA, tissue slices were stained with primary antibodies and biotin-conjugated secondary antibodies, followed by incubation with Avidin and detection (DAB Substrate kit; BD Biosciences, San Jose, CA). All immunofluorescence or IHC images were taken with a confocal microscope or a Nikon Automated Eclipse Ti-E inverted microscope.
Signaling and immunoblot analyses
For signaling analyses, cells were seeded onto 6-well plates at 2 × 10Citation5 cells per well and starved overnight prior to stimulation. Cell lysates or homogenized mammary gland tissue samples were lysed in RIPA buffer supplemented with 1 mM NaV3O4, PMSF and protease inhibitor cocktail (Roche, Basel, Switzerland).Citation63,64 Protein concentrations were quantified by use of DC Protein Assay Kit (Bio-Rad, Hercules, CA). Western blotting was carried out by separating equal amount of proteins on SDS-PAGE and transferring samples onto nitrocellulose membrane prior to detection with the indicated antibodies and Amersham ECL Prime Western Blotting Detection Reagent kit (GE Healthcare Life Sciences, Piscataway, NJ). To evaluate differences in protein expression among samples, ratios of densitometry values of individual protein to β-actin were calculated. These values were then divided by the smallest value within the group to obtain fold change.
Disclosure of Potential Conflicts of Interest
No potential conflicts of interest were disclosed.
Additional information
Funding
References
- Watson CJ, Khaled WT. Mammary development in the embryo and adult: a journey of morphogenesis and commitment. Development 2008; 135:995-1003; PMID: 18296651; http://dx.doi.org/10.1242/dev.005439
- Sternlicht MD. Key stages in mammary gland development - The cues that regulate ductal branching morphogenesis. Breast Cancer Res 2006; 8:201; PMID: 16524451; http://dx.doi.org/10.1186/bcr1368
- McNally S, Martin F. Molecular regulators of pubertal mammary gland development. Ann Med 2011; 43:212-34; PMID: 21417804; http://dx.doi.org/10.3109/07853890.2011.554425
- Asselin-Labat ML, Vaillant F, Shackleton M, Bouras T, Lindeman GJ, Visvader JE. Delineating the epithelial hierarchy in the mouse mammary gland. Cold Spring Harb Symp Quant Biol 2008; 73:469-78; PMID: 19022771; http://dx.doi.org/10.1101/sqb.2008.73.020
- Lu P, Takai K, Weaver VM, Werb Z. Extracellular matrix degradation and remodeling in development and disease. Cold Spring Harb Perspect Biol 2011; 3:25; PMID: 21917992; http://dx.doi.org/10.1101/cshperspect.a005058
- Muschler J, Streuli CH. Cell-matrix interactions in mammary gland development and breast cancer. Cold Spring Harb Perspect Bio 2010; 2:a003202; PMID: 20702598; http://dx.doi.org/10.1101/cshperspect.a003202
- Raymond K, Faraldo MM, Deugnier MA, Glukhova MA. Integrins in mammary development. Semin Cell Dev Biol 2012; 23:599-605; PMID: 22430758; http://dx.doi.org/10.1016/j.semcdb.2012.03.008
- Rooney N, Streuli CH. How integrins control mammary epithelial differentiation: a possible role for the ILK-PINCH-Parvin complex. FEBS Lett 2011; 585:1663-72; PMID: 21570968; http://dx.doi.org/10.1016/j.febslet.2011.05.014
- Taddei I, Deugnier MA, Faraldo MM, Petit V, Bouvard D, Medina D, Fassler R, Thiery JP, Glukhova MA. beta 1 integrin deletion from the basal compartment of the mammary epithelium affects stem cells. Nat Cell Biol 2008; 10:716-22; PMID: 18469806; http://dx.doi.org/10.1038/ncb1734
- Li N, Zhang Y, Naylor MJ, Schatzmann F, Maurer F, Wintermantel T, Schuetz G, Mueller U, Streuli CH, Hynes NE. beta 1 integrins regulate mammary gland proliferation and maintain the integrity of mammary alveoli. Embo J 2005; 24:1942-53; PMID: 15889143; http://dx.doi.org/10.1038/sj.emboj.7600674
- Naylor MJ, Li N, Cheung J, Lowe ET, Lambert E, Marlow R, Wang P, Schatzmann F, Wintermantel T, Schuetz G, et al. Ablation of beta1 integrin in mammary epithelium reveals a key role for integrin in glandular morphogenesis and differentiation. J Cell Biol 2005; 171:717-28; PMID: 16301336; http://dx.doi.org/10.1083/jcb.200503144
- Nagy T, Wei H, Shen TL, Peng X, Liang CC, Gan B, Guan JL. Mammary epithelial-specific deletion of the focal adhesion kinase gene leads to severe lobulo-alveolar hypoplasia and secretory immaturity of the murine mammary gland. J Biol Chem 2007; 282:31766-76; PMID: 17716968; http://dx.doi.org/10.1074/jbc.M705403200
- Akhtar N, Marlow R, Lambert E, Schatzmann F, Lowe ET, Cheung J, Katz E, Li W, Wu C, Dedhar S, et al. Molecular dissection of integrin signalling proteins in the control of mammary epithelial development and differentiation. Development 2009; 136:1019-27; PMID: 19211680; http://dx.doi.org/10.1242/dev.028423
- Hynes RO. Integrins: Bidirectional, allosteric signaling machines. Cell 2002; 110:673-87; PMID: 12297042; http://dx.doi.org/10.1016/S0092-8674(02)00971-6
- Georges-Labouesse E, Messaddeq N, Yehia G, Cadalbert L, Dierich A, Le Meur M. Absence of integrin alpha 6 leads to epidermolysis bullosa and neonatal death in mice. Nat Genet 1996; 13:370-3; PMID: 8673141; http://dx.doi.org/10.1038/ng0796-370
- DiPersio CM, Hodivala-Dilke KM, Jaenisch R, Kreidberg JA, Hynes RO. alpha3beta1 Integrin is required for normal development of the epidermal basement membrane. J Cell Biol 1997; 137:729-42; PMID: 9151677; http://dx.doi.org/10.1083/jcb.137.3.729
- Kreidberg JA, Donovan MJ, Goldstein SL, Rennke H, Shepherd K, Jones RC, Jaenisch R. Alpha 3 beta 1 integrin has a crucial role in kidney and lung organogenesis. Development 1996; 122:3537-47; PMID: 8951069
- Klinowska TC, Alexander CM, Georges-Labouesse E, Van der Neut R, Kreidberg JA, Jones CJ, Sonnenberg A, Streuli CH. Epithelial development and differentiation in the mammary gland is not dependent on alpha 3 or alpha 6 integrin subunits. Dev Biol 2001; 233:449-67; PMID: 11336507; http://dx.doi.org/10.1006/dbio.2001.0204
- Raymond K, Cagnet S, Kreft M, Janssen H, Sonnenberg A, Glukhova MA. Control of mammary myoepithelial cell contractile function by alpha3beta1 integrin signalling. EMBO J 2011; 30:1896-906; PMID: 21487391; http://dx.doi.org/10.1038/emboj.2011.113
- Stingl J, Eirew P, Ricketson I, Shackleton M, Vaillant F, Choi D, Li HI, Eaves CJ. Purification and unique properties of mammary epithelial stem cells. Nature 2006; 439:993-7; PMID: 16395311
- Jeselsohn R, Brown NE, Arendt L, Klebba I, Hu MG, Kuperwasser C, Hinds PW. Cyclin D1 kinase activity is required for the self-renewal of mammary stem and progenitor cells that are targets of MMTV-ErbB2 tumorigenesis. Cancer Cell 2010; 17:65-76; PMID: 20129248; http://dx.doi.org/10.1016/j.ccr.2009.11.024
- Shackleton M, Vaillant F, Simpson KJ, Stingl J, Smyth GK, Asselin-Labat ML, Wu L, Lindeman GJ, Visvader JE. Generation of a functional mammary gland from a single stem cell. Nature 2006; 439:84-8; PMID: 16397499; http://dx.doi.org/10.1038/nature04372
- Hemler ME. Tetraspanin functions and associated microdomains. Nat Rev Mol Cell Biol 2005; 6:801-11; PMID: 16314869; http://dx.doi.org/10.1038/nrm1736
- Deng X, Li Q, Hoff J, Novak M, Yang H, Jin H, Erfani SF, Sharma C, Zhou P, Rabinovitz I, et al. Integrin-associated CD151 drives ErbB2-evoked mammary tumor Onset and metastasis. Neoplasia 2012; 14:678-89; PMID: 22952421
- Yang XWH, Richardson AL, Torres-Arzayus MI, Zhou PC, Sharma C, Kazarov AR, Andzelm MM, Strominger JL, Brown M, Hemler ME. CD151 accelerates breast cancer by regulating alpha(6) integrin function, signaling, and molecular organization. Cancer Res 2008; 68:3204-13; PMID: 18451146; http://dx.doi.org/10.1158/0008-5472.CAN-07-2949
- Stipp CS. Laminin-binding integrins and their tetraspanin partners as potential antimetastatic targets. Expert RevMol Med 2010; 12:e3; PMID: 20078909; http://dx.doi.org/10.1017/S1462399409001355
- Kazarov AR, Yang X, Stipp CS, Sehgal B, Hemler ME. An extracellular site on tetraspanin CD151 determines alpha 3 and alpha 6 integrin-dependent cellular morphology. J Cell Biol 2002; 158:1299-309; PMID: 12356873; http://dx.doi.org/10.1083/jcb.200204056
- Sachs N, Kreft M, van den Bergh Weerman MA, Beynon AJ, Peters TA, Weening JJ, Sonnenberg A. Kidney failure in mice lacking the tetraspanin CD151. J Cell Biol 2006; 175:33-9; PMID: 17015618; http://dx.doi.org/10.1083/jcb.200603073
- Crew VK, Burton N, Kagan A, Green CA, Levene C, Flinter F, Brady RL, Daniels G, Anstee DJ. CD 151, the first member of the tetraspanin (TM4) superfamily detected on erythrocytes, is essential for the correct assembly of human basement membranes in kidney and skin. Blood 2004; 104:2217-23; PMID: 15265795; http://dx.doi.org/10.1182/blood-2004-04-1512
- Baleato RM, Guthrie PL, Gubler MC, Ashman LK, Roselli S. Deletion of Cd151 results in a strain-dependent glomerular disease due to severe alterations of the glomerular basement membrane. Am J Pathol 2008; 173:927-37; PMID: 18787104; http://dx.doi.org/10.2353/ajpath.2008.071149
- Zhang XA, Kazarov AR, Yang X, Bontrager AL, Stipp CS, Hemler ME. Function of the tetraspanin CD151-a6b1 integrin complex during cellular morphogenesis. Mol Biol Cell 2004; 15
- Xu D, Sharma C, Hemler ME. Tetraspanin12 regulates ADAM10-dependent cleavage of amyloid precursor protein. Faseb J 2009; 23:3674-81; PMID: 19587294; http://dx.doi.org/10.1096/fj.09-133462
- Knoblich K, Wang HX, Sharma C, Fletcher AL, Turley SJ, Hemler ME. Tetraspanin TSPAN12 regulates tumor growth and metastasis and inhibits beta-catenin degradation. Cell Mol Life Sci 2013; 71:1305-14; PMID: 23955570
- Van Keymeulen A, Rocha AS, Ousset M, Beck B, Bouvencourt G, Rock J, Sharma N, Dekoninck S, Blanpain C. Distinct stem cells contribute to mammary gland development and maintenance. Nature 2011; 479:189-93; PMID: 21983963; http://dx.doi.org/10.1038/nature10573
- Liu K, Cheng L, Flesken-Nikitin A, Huang L, Nikitin AY, Pauli BU. Conditional knockout of fibronectin abrogates mouse mammary gland lobuloalveolar differentiation. Dev Biol 2010; 346:11-24; PMID: 20624380; http://dx.doi.org/10.1016/j.ydbio.2010.07.001
- Park J, Schwarzbauer JE. Mammary epithelial cell interactions with fibronectin stimulate epithelial-mesenchymal transition. Oncogene 2013; 33(13):1649-57.
- Guan JL. Integrin signaling through FAK in the regulation of mammary stem cells and breast cancer. IUBMB Life 2010; 62:268-76; PMID: 20101634; http://dx.doi.org/10.1002/iub.311
- Zeisberg M, Neilson EG. Biomarkers for epithelial-mesenchymal transitions. J Clin Invest 2009; 119:1429-37; PMID: 19487819; http://dx.doi.org/10.1172/JCI36183
- Nassour M, Idoux-Gillet Y, Selmi A, Come C, Faraldo ML, Deugnier MA, Savagner P. Slug controls stem/progenitor cell growth dynamics during mammary gland morphogenesis. PloS One 2012; 7:e53498; PMID: 23300933; http://dx.doi.org/10.1371/journal.pone.0053498
- Guo W, Keckesova Z, Donaher JL, Shibue T, Tischler V, Reinhardt F, Itzkovitz S, Noske A, Zurrer-Hardi U, Bell G, et al. Slug and Sox9 cooperatively determine the mammary stem cell state. Cell 2012; 148:1015-28; PMID: 22385965; http://dx.doi.org/10.1016/j.cell.2012.02.008
- Tsujino K, Takeda Y, Arai T, Shintani Y, Inagaki R, Saiga H, Iwasaki T, Tetsumoto S, Jin Y, Ihara S, et al. Tetraspanin CD151 protects against pulmonary fibrosis by maintaining epithelial integrity. Am J Respir Crit Care Med 2012; 186:170-80; PMID: 22592804; http://dx.doi.org/10.1164/rccm.201201-0117OC
- Meyer SE, Zinser GM, Stuart WD, Pathrose P, Waltz SE. The Ron receptor tyrosine kinase negatively regulates mammary gland branching morphogenesis. Dev Biol 2009; 333:173-85; PMID: 19576199; http://dx.doi.org/10.1016/j.ydbio.2009.06.028
- Wagh PK, Gray JK, Zinser GM, Vasiliauskas J, James L, Monga SP, Waltz SE. β-Catenin is required for Ron receptor-induced mammary tumorigenesis. Oncogene 2011; 30:3694-704; PMID: 21423209; http://dx.doi.org/10.1038/onc.2011.86
- Wu ZQ, Li XY, Hu CY, Ford M, Kleer CG, Weiss SJ. Canonical Wnt signaling regulates Slug activity and links epithelial-mesenchymal transition with epigenetic Breast Cancer 1, Early Onset (BRCA1) repression. Proc Nat Acad Sci U S A 2012; 109:16654-9; PMID: 23011797; http://dx.doi.org/10.1073/pnas.1205822109
- Macias H, Moran A, Samara Y, Moreno M, Compton JE, Harburg G, Strickland P, Hinck L. SLIT/ROBO1 signaling suppresses mammary branching morphogenesis by limiting basal cell number. Dev Cell 2011; 20:827-40; PMID: 21664580; http://dx.doi.org/10.1016/j.devcel.2011.05.012
- Weaver VM, Lelievre S, Lakins JN, Chrenek MA, Jones JC, Giancotti F, Werb Z, Bissell MJ. beta4 integrin-dependent formation of polarized three-dimensional architecture confers resistance to apoptosis in normal and malignant mammary epithelium. Cancer Cell 2002; 2:205-16; PMID: 12242153; http://dx.doi.org/10.1016/S1535-6108(02)00125-3
- Sachs N, Secades P, van Hulst L, Kreft M, Song JY, Sonnenberg A. Loss of integrin alpha3 prevents skin tumor formation by promoting epidermal turnover and depletion of slow-cycling cells. Proc Nat Acad Sci U S A 2012; 109(52):21468-73.
- Hodivala-Dilke KM, DiPersio CM, Kreidberg JA, Hynes RO. Novel roles for alpha 3 beta 1 integrin as a regulator of cytoskeletal assembly and as a trans-dominant inhibitor of integrin receptor function in mouse keratinocytes. J Cell Biol 1998; 142:1357-69; PMID: 9732295; http://dx.doi.org/10.1083/jcb.142.5.1357
- Proia TA, Keller PJ, Gupta PB, Klebba I, Jones AD, Sedic M, Gilmore H, Tung N, Naber SP, Schnitt S, et al. Genetic predisposition directs breast cancer phenotype by dictating progenitor cell fate. Cell Stem Cell 2011; 8:149-63; PMID: 21295272; http://dx.doi.org/10.1016/j.stem.2010.12.007
- Turner FE, Broad S, Khanim FL, Jeanes A, Talma S, Hughes S, Tselepis C, Hotchin NA. Slug regulates integrin expression and cell proliferation in human epidermal keratinocytes. J Biol Chem 2006; 281:21321-31; PMID: 16707493; http://dx.doi.org/10.1074/jbc.M509731200
- Santoro MM, Gaudino G, Marchisio PC. The MSP receptor regulates alpha6beta4 and alpha3beta1 integrins via 14-3-3 proteins in keratinocyte migration. Dev Cell 2003; 5:257-71; PMID: 12919677; http://dx.doi.org/10.1016/S1534-5807(03)00201-6
- Romanska HM, Berditchevski F. Tetraspanins in human epithelial malignancies. J Pathol 2011; 223:4-14; PMID: 20938929; http://dx.doi.org/10.1002/path.2779
- Sadej R, Romanska H, Kavanagh D, Baldwin G, Takahashi T, Kalia N, Berditchevski F. Tetraspanin CD151 regulates transforming growth factor beta signaling: implication in tumor metastasis. Cancer Res 2010; 70:6059-70; PMID: 20570898; http://dx.doi.org/10.1158/0008-5472.CAN-09-3497
- Novitskaya V, Romanska H, Dawoud M, Jones JL, Berditchevski F. Tetraspanin CD151 regulates growth of mammary epithelial cells in three-dimensional extracellular matrix: implication for mammary ductal carcinoma in situ. Cancer Res 2010; 70:4698-708; PMID: 20501858; http://dx.doi.org/10.1158/0008-5472.CAN-09-4330
- Minner S, De Silva C, Rink M, Dahlem R, Chun F, Fisch M, Hoppner W, Wagner W, Bokemeyer C, Terracciano L, et al. Reduced CD151 expression is related to advanced tumour stage in urothelial bladder cancer. Pathology 2012; 44:448-52; PMID: 22772340; http://dx.doi.org/10.1097/PAT.0b013e32835576ee
- Semenza GL. Does loss of CD151 expression promote the metastasis of hypoxic colon cancer cells? Clinical Cancer Res 2008; 14:7969-70; PMID: 19088010; http://dx.doi.org/10.1158/1078-0432.CCR-08-2417
- Voss MA, Gordon N, Maloney S, Ganesan R, Ludeman L, McCarthy K, Gornall R, Schaller G, Wei W, Berditchevski F, et al. Tetraspanin CD151 is a novel prognostic marker in poor outcome endometrial cancer. Brit J Cancer 2011; 104:1611-8; PMID: 21505452; http://dx.doi.org/10.1038/bjc.2011.80
- Raymond K, Kreft M, Song JY, Janssen H, Sonnenberg A. Dual Role of alpha6beta4 integrin in epidermal tumor growth: tumor-suppressive versus tumor-promoting function. Mol Biol Cell 2007; 18:4210-21; PMID: 17699601; http://dx.doi.org/10.1091/mbc.E06-08-0720
- Keely PJ. Mechanisms by which the extracellular matrix and integrin signaling act to regulate the switch between tumor suppression and tumor promotion. J Mammary Gland Biol Neoplasia 2011; 16:205-19; PMID: 21822945; http://dx.doi.org/10.1007/s10911-011-9226-0
- Izumchenko E, Singh MK, Plotnikova OV, Tikhmyanova N, Little JL, Serebriiskii IG, Seo S, Kurokawa M, Egleston BL, Klein-Szanto A, et al. NEDD9 promotes oncogenic signaling in mammary tumor development. Cancer Res 2009; 69:7198-206; PMID: 19738060; http://dx.doi.org/10.1158/0008-5472.CAN-09-0795
- Seo S, Nakamoto T, Takeshita M, Lu J, Sato T, Suzuki T, Kamikubo Y, Ichikawa M, Noda M, Ogawa S, et al. Crk-associated substrate lymphocyte type regulates myeloid cell motility and suppresses the progression of leukemia induced by p210Bcr/Abl. Cancer Sci 2011; 102:2109-17; PMID: 21848808; http://dx.doi.org/10.1111/j.1349-7006.2011.02066.x
- Takeda Y, Kazarov AR, Butterfield CE, Hopkins BD, Benjamin LE, Kaipainen A, Hemler ME. Deletion of tetraspanin Cd151 results in decreased pathologic angiogenesis in vivo and in vitro. Blood 2007; 109:1524-32; PMID: 17023588; http://dx.doi.org/10.1182/blood-2006-08-041970
- Yang XH, Richardson AL, Torres-Arzayus MI, Zhou P, Sharma C, Kazarov AR, Andzelm MM, Strominger JL, Brown M, Hemler ME. CD151 accelerates breast cancer by regulating alpha 6 integrin function, signaling, and molecular organization. Cancer Res 2008; 68:3204-13; PMID: 18451146; http://dx.doi.org/10.1158/0008-5472.CAN-07-2949z
- Yang XH, Flores LM, Li Q, Zhou P, Xu F, Krop IE, Hemler ME. Disruption of laminin-integrin-CD151-focal adhesion kinase axis sensitizes breast cancer cells to ErbB2 antagonists. Cancer Res 2010; 70:2256-63; PMID: 20197472; http://dx.doi.org/10.1158/0008-5472.CAN-09-4032
- Dontu G, Abdallah WM, Foley JM, Jackson KW, Clarke MF, Kawamura MJ, Wicha MS. In vitro propagation and transcriptional profiling of human mammary stem/progenitor cells. Genes Dev 2003; 17:1253-70; PMID: 12756227; http://dx.doi.org/10.1101/gad.1061803
- Debnath J, Muthuswamy SK, Brugge JS. Morphogenesis and oncogenesis of MCF-10A mammary epithelial acini grown in three-dimensional basement membrane cultures. Methods 2003; 30:256-68; PMID: 12798140; http://dx.doi.org/10.1016/S1046-2023(03)00032-X
- Yang XH, Kovalenko OV, Kolesnikova TV, Andzelm MM, Rubinstein E, Strominger JL, Hemler ME. Contrasting effects of EWI proteins, integrins, and protein palmitoylation on cell surface CD9 organization. J Biol Chem 2006; 281:12976-85; PMID: 16537545; http://dx.doi.org/10.1074/jbc.M510617200