Abstract
In the fungal pathogen Fusarium oxysporum, vegetative hyphal fusion triggers nuclear mitotic division in the invading hypha followed by migration of a nucleus into the receptor hypha and degradation of the resident nucleus. Here we examined the role of autophagy in fusion-induced nuclear degradation. A search of the F. oxysporum genome database for autophagy pathway components identified putative orthologs of 16 core autophagy-related (ATG) genes in yeast, including the ubiquitin-like protein Atg8, which is required for the formation of autophagosomal membranes. F. oxysporum Foatg8Δ mutants were generated in a strain harboring H1-cherry fluorescent protein (ChFP)-labeled nuclei to facilitate analysis of nuclear dynamics. The Foatg8Δ mutants did not show MDC-positive staining in contrast to the wild type and the FoATG8-complemented (cFoATG8) strain, suggesting that FoAtg8 is required for autophagy in F. oxysporum. The Foatg8Δ strains displayed reduced rates of hyphal growth, conidiation, and fusion, and were significantly attenuated in virulence on tomato plants and in the nonvertebrate animal host Galleria mellonella. In contrast to wild-type hyphae, which are almost exclusively composed of uninucleated hyphal compartments, the hyphae of the Foatg8Δ mutants contained a significant fraction of hyphal compartments with 2 or more nuclei. The increase in the number of nuclei per hyphal compartment was particularly evident after hyphal fusion events. Time-lapse microscopy analyses revealed abnormal mitotic patterns during vegetative growth in the Foatg8Δ mutants. Our results suggest that autophagy mediates nuclear degradation after hyphal fusion and has a general function in the control of nuclear distribution in F. oxysporum.
Abbreviations:
- Atg, autophagy-related
- BLAST, basic local alignment search tool
- CFW, calcofluor white
- ChFP, cherry fluorescent protein
- DIC, differential interference contrast
- gDNA, genomic DNA
- GFP, green fluorescent protein
- HygR, hygromycin resistant
- MDC, monodansylcadaverine
- ORF, open reading frame
- PCR, polymerase chain reaction
- PDA, potato dextrose agar
- PDB, potato dextrose broth
- PMSF, phenylmethylsulfonyl fluoride
- SM, synthetic medium
- WT, wild-type
Introduction
Genetic analyses performed in the yeast species Saccharomyces cerevisiae, Hansenula polymorpha, and Pichia pastoris identified 38 autophagy-related (ATG) genes, of which 17 are components of the core autophagic machinery and 19 are involved in selective autophagy or the induction of specific autophagy-related pathways in response to different physiological conditions.Citation1 For a review see ref.Citation2 One of the key components of autophagy is Atg8, a highly conserved ubiquitin-like protein that, after cleavage and lipidation, is associated with the phagophore and autophagosome membrane and has been used as a marker of autophagy.Citation3 In filamentous fungi such as Aspergillus oryzae, autophagy mediates degradation of peroxisomes, mitochondria, and nuclei and has been suggested as a strategy to obtain nutrients under starvation conditions by recycling their own cellular components.Citation4
Recent evidence, however, suggests that autophagy in filamentous fungi is not only involved in nutrient homeostasis but also in developmental processes such as cell differentiation, secondary metabolism, and pathogenicity.Citation5-8 Mutants of A. oryzae or Fusarium graminearum lacking Atg8 show defects in conidiation and aerial hyphal growth.Citation9,10 In the rice blast pathogen Magnaporthe oryzae, MoAtg8 mediates autophagic cell death and is required for differentiation of infection structures.Citation11 Likewise, disruption of ATG8 in Ustilago maydis leads to autophagosome accumulation in the vacuoles and impaired virulence.Citation12 In the coprophilic ascomycete Sordaria macrospora, SmAtg8 is required for fruiting-body development, vegetative growth, and ascospore germination.Citation13 In the insect pathogen Metarhizium robertsii, Atg8 is essential for fungal development, lipid storage, and virulence.Citation14
The soil-borne plant pathogen Fusarium oxysporum provokes vascular wilt in a wide diversity of cropsCitation15 and can cause severe infections on immunocompromised patients.Citation16,17 Hyphae of F. oxysporum frequently undergo vegetative fusion, also called anastomosis.Citation18-21 Although not essential for plant infection, anastomosis may facilitate colonization of the host root surface.Citation22 Vegetative hyphal fusion in F. oxysporum initiates with directed growth of a hypha (fusing hypha) toward another one (receptor hypha). After hyphal contact, the apical nucleus of the fusing hypha undergoes mitosis, and one of the daughter nuclei migrates into the receptor hypha, thereby triggering the disintegration of the resident nucleus.Citation23 The mechanism underlying nuclear degradation is unknown. Here we examined the importance of autophagy in post-fusion nuclear degradation by generating deletion mutants in the F. oxysporum FoATG8 gene. We provide evidence for a previously unreported role of autophagy as a key mechanism of vegetative hyphal fusion and postfusion nuclear events. We propose that autophagy contributes to controlling the number of nuclei per hyphal compartment during vegetative growth. We further show, for the first time in a single species, the importance of autophagy in virulence on plants and animal hosts.
Results
The F. oxysporum genome contains a battery of autophagy-related genes
A protein BLAST (BLASTP) search of autophagy pathway components in the genome sequence database of the tomato pathogen F. oxysporum f. sp. lycopersici strain 4287 identified predicted orthologs of most core Atg proteins in S. cerevisiae. These include the ubiquitin-like protein Atg8 which is required for the formation of autophagosomal membranes, as well as 9 putative Atg proteins involved in the induction of autophagy and selective autophagy (). The F. oxysporum ATG8 ortholog FOXG_12665 encodes a predicted protein of 120 amino acids and a calculated molecular mass of 14,037 kDa, which shares significant identiy with the Atg8 proteins of F. graminearum (98.3%), A. niger (98.3%), Neurospora crassa (97.5%) Podospora anserina (95.8%), Gaeumannomyces graminis (94.2%), M. oryzae (93.3%), U. maydis (85.6%), and S. cerevisiae (78.6%) (Fig. S1). In S. cerevisiae, as well as in the filamentous ascomycete S. macrospora, the Atg8 precursor is cleaved after the glycine 116 residue by the protease Atg4 to yield mature Atg8.Citation13,24 Likewise, F. oxysporum FoAtg8 has a predicted Atg4 cleavage site at the same amino acid position 116 (Fig. S1).
Table 1. Autophagy-related genes in Fusarium oxysporum.
Deletion of FoATG8 impairs autophagy in F. oxysporum
To study FoAtg8 function, targeted gene replacement was performed in a F. oxysporum strain harboring the H1-ChFP fusion protein, thereafter called wild typeCitation23 (Table 2; Fig. S2A). Hygromycin-resistant (HygR) transformants were selected and initially analyzed by polymerase chain reaction (PCR) amplification of the insertion flanking regions (Fig. S2B). Putative deletion mutants were further confirmed by Southern blot. Three transformants (Foatg8Δ H1-ChFP #3, #9 and #10, hereafter named Foatg8Δ #3, Foatg8Δ #9 and Foatg8Δ #10, respectively) showed the expected shift in the hybridizing band from 3.9 kb to 6.8 kb, indicating homologous replacement of the FoATG8 gene (Fig. S2C). Complementation of Foatg8Δ was achieved by cotransformation with the FoATG8 gene and the NatR cassette as selective marker. NatR transformants carrying a wild-type FoATG8 allele (cFoATG8) were identified by PCR analysis using a primer pair specific for the FoATG8 coding region (; Fig. S2D).
Table 2. Fungal strains used in this study.
Table 3. Oligonucleotides used in this study.
We tested the ability of the different strains to undergo autophagy when transferred to minimal medium lacking a nitrogen source. To this aim, staining with monodansylcadaverine (MDC), an indicator of autophagic activity that accumulates in vacuoles,Citation25,26 was performed in the presence or absence of phenylmethylsulfonyl fluoride (PMSF), an inhibitor of vacuolar serine proteases.Citation27 Nitrogen starvation triggered autophagy in the wild type and the cFoATG8 strains as indicated by the presence of fluorescence in the cytoplasm and vacuoles (). Interestingly, most hyphal compartments staining positive for MDC contained either degrading or no nuclei. Importantly, no MDC staining was observed in the nitrogen-starved mycelium of the Foatg8Δ mutants. We conclude that in F. oxysporum, FoAtg8 is necessary for starvation-induced autophagy.
Figure 1. FoAtg8 is required for autophagy in F. oxysporum. Micrographs showing hyphae of the indicated strains subjected to nitrogen starvation in the presence (+) or absence (-) of PMSF, and stained with monodansylcadaverine (MDC). White arrows point to hyphal compartments containing degraded nuclei. Bars = 20 μm.
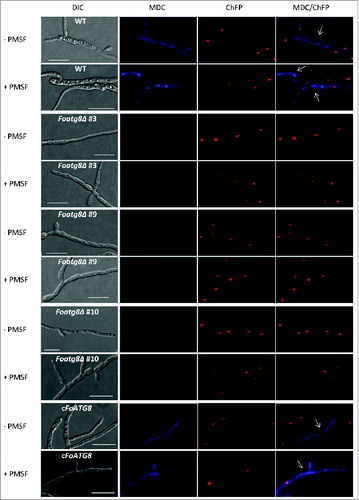
Autophagy is required for proper vegetative growth and conidiation
Defective autophagy results in severe impairment of conidiation and colony growth in several fungi.Citation5,9,10,14,28,29 We therefore determined vegetative growth and conidiation on solid and in liquid media, both in rich and minimal medium. Under high-nutrient conditions, colonies of the Foatg8Δ mutants showed slightly reduced radial growth () and decreased development of aerial mycelium in comparison with the wild-type strain (). Moreover, the number of microconidia recovered from colonies of the Foatg8Δ mutants was more than 10-fold lower than in the wild-type strain (; P < 0.05). Likewise, in liquid cultures the Foatg8Δ mutants produced approximately 5 times less microconidia than the wild type (; P < 0.05). Genetic complementation of the FoAtg8Δ mutant fully restored the wild-type phenotype. Colonies grown under mildly nutrient-limiting conditions (synthetic medium, SM), were highly irregular (Fig. S3A). Additionally, those grown under severely nutrient-limiting conditions (SM diluted 1:1000) that induced autophagy, produced very faint mycelia that originated faster growing colonies with increased diameter but with no detectable aerial hyphae, probably as a response to the severe starvation conditions encountered in the growth medium (Fig. S4A). Under both conditions the Foatg8Δ mutants displayed reduced radial growth compared to the wild-type or the cFoATG8 strains (Figs. S3B, S4B). The number of conidia produced by colonies of the Foatg8Δ mutants on SM and SM-diluted 1:1000 plates was only 18% and 0.4%, respectively, of that produced by the wild-type or the cFoATG8 strain (Figs. S3C and S4C). A severe reduction in conidiation of the Foatg8Δ mutants was also observed in liquid SM and SM diluted 1:1000 cultures (Figs. S3D and S4D).
Figure 2 (See previous page). Hyphal growth and conidiation are reduced in Foatg8Δ mutants. (A) Freshly obtained microconidia (103) of the indicated strains were spotted onto PDA plates and incubated at 28°C. Colony diameter was measured daily during 10 d, starting on the second day to avoid the lag time. Graph represents growth curves of the indicated strains. Bars indicate the standard error from 3 independent replicates. (B) Representative images of the indicated strains 6 and 10 d after inoculation. (C) Total number of conidia recovered from PDA plates after 10 d growth at 28°C. Bars indicate the standard error from 3 independent replicates. Columns with the same letter are not significantly different (Duncan, P < 0.05). (D) Total number of conidia recovered from liquid cultures grown for 2 d with shaking at 28°C. Bars indicate the standard error from 3 independent replicates. Columns with the same letter are not significantly different (Duncan, P < 0.05).
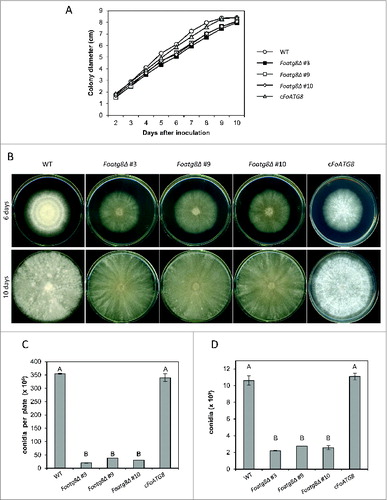
FoAtg8-mediated autophagy controls dynamics and cellular distribution of nuclei
During vegetative growth of F. oxysporum, mitotic activity occurs exclusively in the apical hyphal compartments.Citation23 We thus hypothesized that the decreased growth rates observed in the Foatg8Δ mutants could be related to defects in cell division during apical extension. To test this idea, we stained the hyphal cell walls in the different strains with the chitin-binding dye calcofluor white (CFW) to determine the number of nuclei per hyphal compartment. In contrast to the wild type, which had almost exclusively uninucleate hyphal compartments, between 12% and 15% (P < 0.05) of the hyphal compartments in the Foatg8Δ mutants contained 2 or more nuclei (). Multinucleate hyphal compartments in these mutants were more frequently observed in the sub-apical region than in the apical compartment (). Interestingly, some hyphal compartments in the Foatg8Δ mutants appeared to contain nuclei with increased size compared to the wild-type strain, as deduced from enhanced fluorescence (), suggesting anomalies in mitotic division. Indeed, time-lapse microscopy analysis of the Foatg8Δ mutants revealed aberrant nuclear divisions originating more than 2 nuclei within the same hyphal compartment ( and Movies S1 and S2). By contrast, the mitotic pattern in the cFoATG8 strain was similar to that of the wild-type strain. These findings suggest that autophagy contributes to the control of the number of nuclei per hyphal compartment during vegetative growth of F. oxysporum.
Figure 3. Hyphae from Foatg8Δ mutants contain multinucleated compartments. (A) Percentage of hyphal compartments containing 2 or more nuclei (n = 1000). Bars indicate the standard error from 3 independent replicates. Columns with the same letter are not significantly different (Duncan, P < 0.05). (B) Micrographs showing hyphae of the indicated strains subjected to nitrogen starvation and stained with calcofluor white (CFW). Arrows point to hyphal compartments containing more than one nucleus. Bars = 20 μm.
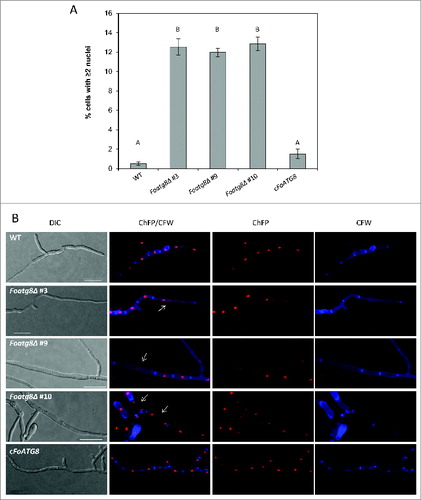
Figure 4. Aberrant nuclear divisions in the Foatg8Δ mutants originate multinucleated hyphal compartments. (A) Series of images of a developing hypha from Movie S1 showing an example of an aberrant nuclear division. An apical nucleus displaying increased fluorescence (white arrow) divides and originates 4 nuclei with normal fluorescence intensity. After septum formation, one daughter nucleus remains in the new sub-apical compartment, while the other 3 are located within the new apical compartment (black arrow). Bars = 20 μm. (B) Series of images of a developing hypha from Movie S2, showing an example of aberrant nuclear division. An apical nucleus displaying increased fluorescence (white arrow) divides and originates 2 nuclei. One of the daughter nuclei (green arrow) divides again before a new septum is formed (yellow arrow). After the second mitotic event, 3 nuclei are located within the same compartment (black arrow). Completion of cytokinesis then originates a new sub-apical compartment containing 2 nuclei (asterisk). Bars = 20 μm.
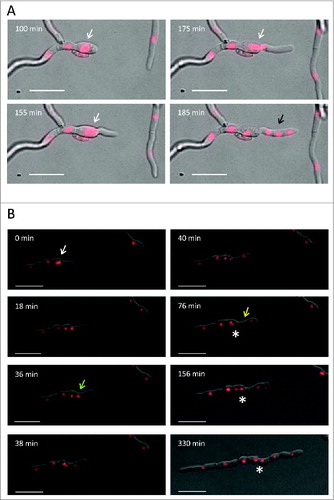
Autophagy regulates hyphal fusion and postfusion nuclear degradation events
Vegetative hyphal fusion in fungi is a highly regulated developmental process that involves complex cell-cell recognition and signaling events.Citation30 In F. oxysporum, hyphal fusion requires defined signaling components such as the mitogen-activated protein kinase Fmk1 or the WW domain protein Fso1.Citation22 The rate of hyphal fusion in the Foatg8Δ hyphae was approximately 10-fold lower (P < 0.05) than in the wild-type or the cFoATG8 strain (). A significant reduction in the fusion rate was also detected when Foatg8Δ hyphae were combined with wild-type hyphae (P < 0.05). Thus, efficient hyphal fusion in F. oxysporum requires FoAtg8 function.
Figure 5. FoAtg8 is required for nuclear degradation after vegetative hyphal fusion. (A) Percentage of fusion events observed among germlings from the indicated strains (n = 900). Bars indicate the standard error from 3 independent replicates. Columns with the same letter are not significantly different (Duncan, P < 0.05). (B) Micrographs showing formation of anastomosis tubes between hyphae from the indicated strains. Arrows point to fused hyphal compartments. Bars = 20 μm.
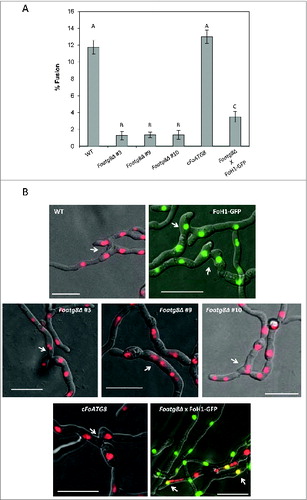
Hyphal fusion in F. oxysporum involves different nuclear events such as mitosis in the invading hypha and migration of the daughter nucleus into the receptor hypha, followed by degradation of the resident nucleus.Citation23 To examine the role of autophagy in fusion-induced nuclear degradation we determined the number of nuclei per hyphal compartment in hyphae undergoing hyphal fusion. In the wild-type strain the fused compartments almost always contained either 2 or 3 nuclei (), a result expected from the previously reported nuclear dynamics during hyphal fusion.Citation23 Two nuclei originate from prefusion nuclear division or postfusion nuclear migration and degradation of the resident nucleus, while 3 nuclei result from postfusion nuclear division and migration. Remarkably, in the rare cases where hyphal fusion was detected in the Foatg8Δ mutants, the fused hyphal compartments almost invariably contained more than 3 nuclei (). The number of nuclei in hyphal fusion compartments of the cFoATG8 strain was similar to that observed in the wild-type strain. Taken together, these results demonstrate that nuclear dynamics after hyphal fusion is severely impaired in the Foatg8Δ mutants.
Autophagy is required for virulence of F. oxysporum on plant and animal hosts
Tomato plants inoculated with the wild-type or the cFoATG8 strain showed progressive wilt symptoms followed by death of all plants after 27 d (). However, plants inoculated with Foatg8Δ mutants showed a significant delay in symptom development, and 60 to 70% were still alive and healthy 30 d after inoculation (P < 0.0022).
Figure 6. FoAtg8 contributes to virulence on plant and animal hosts. (A) Groups of 10 plants (cultivar Monika) were inoculated by immersing the roots into a suspension of 5 × 106 freshly obtained microconidia ml−1 of the wild-type strain (WT), the Foatg8Δ mutants #3, #9, and #10 or the cFoATG8 strain, planted in minipots and incubated at 28°C. Uninoculated plants were used as control. Percentage survival was recorded after different time points. All experiments were performed at least 3 times with similar results. The data shown are from 1 representative experiment. (B) Mantel-Cox plots of Galleria mellonella larvae survival after injection of 1.6 × 105 microconidia of the indicated strains into the hemocoel and incubation at 30°C. Larvae inoculated with phosphate-buffered saline were used as control. Experiments were performed 3 times with similar results. Data shown are from one representative experiment.
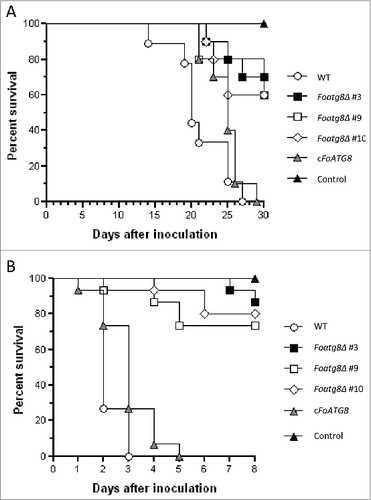
We next examined virulence in the greater wax moth Galleria mellonella, a nonvertebrate model used to study F. oxysporum infection on animal hosts.Citation31 Injection of microconidia of the wild-type or the cFoATG8 strain into the hemocoel of G. mellonella caused death of all larvae 3 d after inoculation (). By contrast, the Foatg8Δ mutants showed a significant reduction in killing (P < 0.0001), and more than 50% of the larvae survived 9 d after inoculation. We conclude that FoAtg8-mediated autophagy is required for full virulence of F. oxysporum both on plant and animal hosts.
The GFP-FoAtg8 fusion protein accumulates in autophagosomal compartments during autophagy-induced nuclear degradation
To investigate the subcellular localization of FoAtg8 in F. oxysporum, the gene encoding green fluorescent protein was fused in frame to the 5’-end of the FoATG8 coding region driven by its native promoter (Fig. S5). This construct, together with the NatR cassette, was used to cotransform the Foatg8Δ strain, resulting in GFP-FoAtg8 tagged strains that recovered wild-type growth and conidiation rates (data not shown), demonstrating the functionality of the construct. Microscopic analysis of the transformants revealed a strong fluorescence accumulating mainly in autophagosome-like structures located in close proximity to nuclei of nitrogen-starved hyphal compartments that were initiating the autophagy process, but were absent in MDC-positive stained compartments where nuclei were either absent or still being degraded (). These results suggest that FoAtg8 is actively involved in the initial steps of nuclear degradation during starvation-induced autophagy in F. oxysporum, and rapidly disintegrates in later stages of the process, as reported for Atg8 in A. nidulans where GFP-Atg8 fluorescence decays after autophagosome fusion to the vacuolar membrane.Citation32
Figure 7. The GFP-FoAtg8 fusion protein disappears rapidly during autophagy-induced nuclear degradation. Micrographs showing hyphae of the GFP-FoAtg8 strain harboring H1-ChFP protein subjected to nitrogen starvation and stained with monodansylcadaverine (MDC). Note the absence of autophagosome-like structures (white arrows) in MDC-stained compartments containing either nondegraded (A) or degrading (B) nuclei (red arrows). Bars = 20 μm.
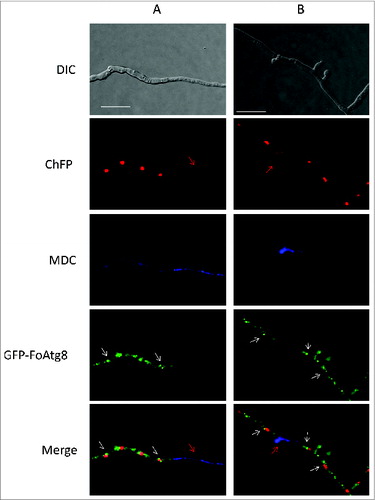
Discussion
Previous studies in the filamentous fungus F. oxysporum revealed that hyphae exhibit an acropetal pattern during vegetative growth. Therefore F. oxysporum can be considered as a mononucleated- compartmented mycelial organism.Citation23 This suggests the presence of a mechanism that precisely controls cell volume, compartmentalization, and cell cycle, thereby maintaining the uninucleate status of hyphal compartments during vegetative growth and fusion.
In this work a reverse genetic approach was followed to evaluate the role of autophagy in this cellular and nuclear maintenance process. We found that the F. oxysporum genome encodes most of the known Atg proteins for nonselective macroautophagy. This result is similar to those reported in other filamentous ascomycetes and highlights the importance of this conserved process in fungal cell survival and viability.Citation2,33,34 However, F. oxsporum only has currently identified orthologs for a portion of the Atg proteins required for selective autophagy in yeast, suggesting that organism-specific forms of selective autophagy may have evolved in fungi.Citation34 In S. cerevisae and S. macrospora, the C terminus of Atg8 is post-translationally modified by the cysteine protease Atg4, generating Atg8G116, which is then bound to the phospholipid phosphatidylethanolamine by Atg7 and Atg3.Citation13,24,35,36 The domain containing residue G116 is conserved between Atg8 orthologs of F. oxysporum and S. cerevisae, suggesting analogous functions of the two proteins.
One of the key roles of autophagy is the recycling of cellular elements to ensure survival under adverse conditions. We found that nitrogen depletion triggers autophagy in F. oxysporum, as shown previously in F. graminearum.Citation9 The absence of MDC positive staining in nitrogen-starved Foatg8Δ mutants strongly suggests that FoAtg8 is essential for autophagy in F. oxysporum. Endogenous recycling of the cytosol and organelles by autophagy is suggested to be important for nutrient trafficking along hyphal filaments as well as for the development of aerial hyphae bearing conidiophores.Citation29,37 In line with this idea, Foatg8Δ mutants exhibited reduced aerial hyphae and conidiation, as reported in other filamentous fungi.Citation5,910,14,28,29 In fungal pathogens, successful infection might depend on the recycling of macromolecules to support cellular activity under nutrient-limiting conditions in the host. We found that virulence of Foatg8Δ mutants on both tomato plants and G. melonella larvae was significantly attenuated. Among the three most important fungal pathogens of humans, Cryptococcus neoformans requires autophagy for infectionCitation38 whereas this process is dispensable for virulence in Candida albicansCitation6 and Aspergillus fumigatus.Citation29 The differential requirement of autophagy during infection of animal hosts is striking and highlights the unique interaction of each fungal pathogen with its host.
We noted that Foatg8Δ mutants undergo aberrant mitotic events that cause a significant increase in the fraction of hyphal compartments which contain more than one nucleus. In the multinucleated fungus A. oryzae autophagy mediates degradation of entire nuclei in aged basal hyphal compartments of the colony, suggesting that filamentous fungi may use nuclei as nutrient pools for supporting hyphal tip growth via autophagy.Citation4 However, while such a mechanism makes sense in organisms with multinucleated hyphal compartments, it would result in rapid cell death in fungi containing mostly uninucleate hyphal compartments such as F. oxysporum. It cannot be excluded that the increased rate of multinucleated hyphal compartments in the Foatg8Δ mutants is caused by defects in septum formation after nuclear division.
The Foatg8Δ mutants show a dramatically reduced rate of hyphal fusion, a phenotype that has not been described previously in a filamentous ascomycete. Because hyphal fusion occurs preferentially under nutrient-limited conditions,Citation22,23 we speculate that autophagy-mediated recycling of nutrients might be important during fusion events. Alternatively, autophagy might be required for hyphal recognition and differentiation during fusion, which is a highly regulated developmental process.Citation39 The developmental program that triggers fusion-competent hyphae is currently unknown. It has been hypothesized that mutual hyphal attraction during fusion is mediated by diffusible chemoattractant molecules that induce relocalization of the Spitzenkörper and reorientation of hyphal tip growth.Citation39 Importantly, mitogen-activated protein kinase pathways that control different aspects of fungal development are essential for hyphal fusion in different fungal species.Citation22,40,41
Strikingly, the Foatg8Δ mutants contained significantly more nuclei in the rare hyphal compartments that had undergone hyphal fusion. This is the first reported evidence for a role of autophagy in the regulation of nuclear number in a mononucleated filamentous organism. While degradation of nuclear components was previously shown to be an autophagy-mediated process in budding yeast, it remained restricted to preribosomes and the nuclear envelope.Citation42 In mammalian cells autophagy is used to degrade damaged DNA and nuclear proteins after translocation to the cytoplasm.Citation43 In addition to the above-mentioned case of A. oryzae, there are a few more examples of degradation of whole nuclei mediated by autophagy in multinucleated organisms. The rice blast fungus M. oryzae requires autophagy for nuclear degradation during appressorium formation.Citation11 In the ciliated protozoan Tetrahymena thermophila, ATG8 is required for programmed nuclear death during sexual reproduction.Citation44 The disparate effects of autophagy on nuclear degradation might reflect divergences in adaptation of the different organisms to cellular physiology and development. Further studies are needed to understand the exact role of autophagy during nuclear division and cytokinesis, and its effects on hyphal growth, development, and pathogenicity of F. oxysporum.
Materials and Methods
Fungal isolates and culture conditions
F. oxysporum f.sp lycopersici strains utilized in this study are listed in . Fungal strains were stored at −80°C with glycerol as a microconidial suspension. The pathotype of the isolates was periodically confirmed by plant infection assays. For extraction of DNA and microconidia production, cultures were grown in potato dextrose broth (PDB; Scharlau, 02-483) at 28°C as described previously.Citation45
For phenotypic analysis of colony growth and conidiation, water droplets containing 103 freshly obtained microconidia were spotted onto potato dextrose agar (PDA) plates and on plates containing synthetic minimal medium (SM45) [MgSO4.7H2O (0.2 g/L); KH2PO4 (0.2 g/L); KCl (0.2 g/L); NH4NO2 (1 g/L); FeSO4 (0.01 g/L); ZnSO4 (0.01 g/L); MnSO4 (0.01 g/L); glucose (10 g/L)] or SM diluted 1000 fold and incubated at 28°C for several days, before being photographed. The area of colonies grown on SM plates was calculated by image processing using the software ImageJ (U. S. National Institute of Health, Bethesda, Maryland, USA, http://imagej.nih.gov/ij/). Conidia from colonies grown for 10-13 d were harvested and counted. For analysis of conidiation in liquid cultures 1.25 × 108 microconidia were inoculated into 25 ml PDB, SM and SM diluted 1:1000 and incubated at 28°C for 2 to 7 d with shaking at 170 rpm. Conidia were then harvested and counted as described previously.Citation45 Data were analyzed with the software SPSS 15.0 for Windows® (LEAD Technologies, Inc..). ANOVA was performed and the Duncan post-hoc test was executed to assess the differences among strains at P ≤ 0.05.
For calcofluor white staining 5 × 107 freshly obtained microconidia were grown at 28°C for 14 h in 5 mL PDB with shaking at 170 rpm and incubated in the dark for 5 min with 10 μM CFW (Fluka, 18909). For fusion assays 50 μL water droplets containing 6.2 × 105 freshly obtained microconidia from the different strains were transferred to 1% (w/v) agarose plates containing fusion medium.Citation23 After 12 h incubation at 28°C agarose blocks were excised from the plates and samples were observed and photographed by optical and epifluorescence microscopy as described below. The rate of hyphal fusion was calculated as the number of fused hyphal compartments among a total of 1,000 hyphae observed in each strain combination. Experiments were performed 3 times. Data were analyzed with the software SPSS 15.0 for Windows® (LEAD Technologies, Inc.). ANOVA was performed and the Duncan post hoc test was executed to assess the differences among strains at P ≤ 0.05.
Nucleic acid manipula-tions and sequence analysis
Genomic DNA (gDNA) was extracted from F. oxysporum mycelium according to previously reported protocols.Citation46,47 Southern analyses and probe labeling were carried out as described previouslyCitation45 using the nonisotopic digoxigenin labeling kit (Roche Applied Science, 11175033910). DNA and protein sequence databases were searched using the BLASTP algorithmCitation48 at the National Center for Biotechnology Information (Bethesda, MD, USA). Amino acid sequences were aligned using the CLUSTALW algorithm Citation49 with the Bioedit 7.0.0 program.Citation50
Targeted deletion of FoATG8 and complemen-tation of the mutants
Targeted gene replacement was performed using the split marker technique.Citation51 Two overlapping gene deletion constructs were generated (Fig. S2A). One contained the promoter sequence of FoATG8 gene, obtained by amplification with the specific primer pair ATG8-5 and ATG8-2 (), fused to the 3´-end (approx. 75%) of the HygR cassette,Citation52 amplified using primer pair trpC-8B and hyg-G (). The second fragment contained the terminator region of the target gene, obtained by amplification with the specific primer pair ATG8-3 and ATG8-6 (), fused to the 5`-end (aprox. 75%) of the HygR cassette, amplified using primer pair gpdA-15B and hyg-Y (). These 2 overlapping DNA fragments were used to transform protoplasts of F. oxysporum strain harboring the H1-ChFP fusion proteinCitation23 (), as reported previously.Citation45 The resulting HygR transformants were initially identified by PCR (Fig. S2B), and the homologous recombination events were confirmed by Southern analysis of selected transformants using a terminator gene fragment as probe (Fig. S2C).
For complementation of the Foatg8Δ mutant, a 4.2 kb DNA fragment containing the complete FoATG8 gene was amplified from genomic DNA using primers ATG8-1 and ATG8-4 () and Pfu DNA polymerase (Promega, M774B). A 1.24 kb fragment containing the nourseothricin resistance (NatR) cassette was amplified from plasmid pDNat1Citation53 using primers M13for and M13rev (). Both fragments were used in the proportion 1:1 to cotransform protoplast of strain Foatg8Δ #10. The resulting NatR transformants were analyzed by PCR amplification of an internal fragment of the FoATG8 coding region, using primers ATG8-9 and ATG8-10 (, Fig. S2D). Nourseothricin (Werner BioAgents, CAS#96736-11-7) was used at a final concentration of 50 μg/mL on solid medium or 2.5 μg/mL in liquid cultures.
GFP-tagging of FoAtg8 protein
F. oxysporum cDNA encompassing the complete FoATG8 ORF was amplified from total RNA using primers ATG8-11N corresponding to the 7 initial codons of the ORF plus the NsiI restriction site and an additional cytosine, and ATG8-12X corresponding to the reverse complement of the last 7 codons of the ORF plus the XmaI restriction site and an additional cytosine (). The resulting amplified band was cloned into pGEMT vector (Promega, A3600) and then subcloned in frame into the appropriate sites of the Aspergillus nidulans vector p1902 (kindly provided by Dr. M.A. Peñalva, CIB-CSIC, Spain),Citation32 resulting in a GFP-FoAtg8 fusion protein under the transcriptional control of the gpdA promoter and terminator (Fig. S5). This plasmid was used as template for PCR amplification, using primers gfp-atg8-5E corresponding to the first 8 codons of the gfp ORF plus an EcoRI restriction site, and tgpda-1 corresponding to the reverse complement of the 5’ end of the of gpdA terminator (). The amplified DNA fragment was cloned into the pGEMT vector, resulting in plasmid gfp-FoATG8/pGEMT. On the other hand, a 1,879 bp fragment corresponding to the FoATG8 native promoter region was amplified from F. oxysporum gDNA using primer pairs ATG8-17E and ATG8-18E, both containing an EcoRI restriction site, cloned into pGEMT and then subcloned in frame into the appropriate EcoRI restriction site of the vector gfp- FoATG8/pGEMT. A 3.4 kb fragment containing the GFP-FoAtg8 fusion under the control of the FoATG8 native promoter and the gpdA terminator was obtained by PCR amplification using primer pair M13for and M13rev () and used for protoplast transformation. Sequencing of both DNA strands of all the obtained clones was performed at the STAB VIDA (Portugal) using the Dyedeoxy Terminator Cycle Sequencing Kit (Applied Biosystems, Foster City-CA, USA) on an ABI Prism 377 Genetic Analyzer apparatus (Applied Biosystems, Foster City, CA USA).
Analysis of autophagy
In order to visualize autophagy in F. oxysporum strains, 2.5 × 108 microconidia from the different strains were grown on PDB for 15 h at 28°C, washed with sterile water and transferred into SM lacking a nitrogen source in the presence or absence of 4 mM PMSF (Sigma, P7626). After 1 h of starvation, mycelium was stained with the fluorescent dye MDC (Sigma, D4008) at a final concentration of 50 μM for 30 min in the dark, washed with water and observed under differential interference contrast (DIC) and epifluorescence microscopy.
Optical and epifluorescence microscopy
For optical and epifluorescence microscopy analyses cell aliquots were embedded in 1% agarose blocks, and observed using the Nomarsky technique or the appropriate filter set, respectively, in a Zeiss Axio Imager M2 Dual Cam microscope (Carl Zeiss MicroImaging GmbH, Göttingen, Germany). Examination using epifluorescence was performed with UV-light 340 to 380 nm and the following filter blocks: MDC and CFW staining (G 365, FT 395, LP 420), GFP (BP 450-490, FT 510, LP 515), ChFP (BP 560/40, FT 585, BP 630/75). Images were captured with an Evolve Photometrics EM512 digital camera (Photometrics Technology, Tucson, AZ, USA) using the Axiovision 4.8 software. Images were processed using Adobe Photoshop CS3 (Adobe Systems, Mountain View, CA USA).
For fluorescence video microscopy analysis, 20-μl droplets containing a mixture of 2.5 × 106 spores/mL were inoculated on glass slides provided with a cavity of 15 to 18 mm diameter (depth 0.6 to 0.8 mm) (Marienfeld, 13 200 00) filled with 150 μL fusion medium containing 1% agarose, covered with a coverslip, and incubated at 28°C for up to 10 h. Images were taken every 5 min using the microscope mentioned above and time-lapse picture series were converted to QuickTime movies.
Plant and animal infection assays
Tomato root inoculation assays were performed as described,Citation45 using 2-wk-old tomato seedlings (cultivar Monika) (Syngenta, 10946359) and F. oxysporum strains, by immersing the roots in a suspension of 5 × 106 spores/mL for 30 min, planted in vermiculite and maintained in a growth chamber. Ten plants were used for each treatment. Severity of disease symptoms and plant survival was recorded daily for 30 d as previously described.Citation54 Virulence experiments were performed 3 times with similar results.
Galleria mellonella larvae in the final larval stage (Animal Center S.C.P., Valencia, Spain), were inoculated using a Burkard Auto Microapplicator (0.1–10 μL) (Burkard Manufacturing Co. Limited, Hertfordshire, UK) as previously described.Citation31 Fifteen larvae per treatment between 0.2 to 0.3 g in weight were inoculated, with a 1 mL syringe to inject 8 μL of a microconidial suspension containing 1.5 × 105 spores. Larvae injected with phosphate-buffered saline (137 mM NaCl; 2.7 mM KCl; 10 mM Na2HPO4; 1.8 mM KH2PO4) served as controls. Larvae were incubated in glass containers at 30°C, and the number of dead larvae was scored daily. Larvae were considered dead when they displayed no movement in response to touch. The Mantel-Cox method was used to assess statistical significance of differences in survival among groups. Data was plotted using Graph Pad Prism software version 4 for Windows. Differences showing a P value < 0.05 were considered significant. Experiments were performed 3 times with similar results. Data presented are from one representative experiment.
Disclosure of Potential Conflicts of Interest
No potential conflicts of interest were disclosed.
Supplemental Material
Supplemental data for this article can be accessed on the publisher's website.
KAUP_A_994413_Supplements.zip
Download Zip (8.8 MB)Acknowledgments
The authors gratefully acknowledge Esther Martínez Aguilera and Isabel Caballero for technical assistance.
Funding
This research was supported by Junta de Andalucía (grant CVI-7319) and the Spanish Ministerio de Ciencia e Innovación (grant BIO2013-47870 and the Ramón y Cajal Program).
References
- Inoue Y, Klionsky DJ. Regulation of macroautophagy in Saccharomyces cerevisiae. Semin Cell Dev Biol 2010; 21:664-70; PMID:20359542; http://dx.doi.org/10.1016/j.semcdb.2010.03.009
- Voigt O, Poggeler S. Self-eating to grow and kill: autophagy in filamentous ascomycetes. Appl Microbiol Biotechnol 2013; 97:9277-90; PMID:24077722; http://dx.doi.org/10.1007/s00253-013-5221-2
- Xie Z, Nair U, Klionsky DJ. Atg8 controls phagophore expansion during autophagosome formation. Mol Biol Cell 2008; 19:3290-8; PMID:18508918; http://dx.doi.org/10.1091/mbc.E07-12-1292
- Shoji JY, Kikuma T, Arioka M, Kitamoto K. Macroautophagy-mediated degradation of whole nuclei in the filamentous fungus Aspergillus oryzae. PLoS One 2010; 5:e15650; PMID:21187926; http://dx.doi.org/10.1371/journal.pone.0015650
- Bartoszewska M, Kiel JA. The role of macroautophagy in development of filamentous fungi. Antiox Redox Signal 2011; 14:2271-87; PMID:20712412; http://dx.doi.org/10.1089/ars.2010.3528
- Palmer GE, Kelly MN, Sturtevant JE. Autophagy in the pathogen Candida albicans. Microbiology 2007; 153:51-8; PMID:17185534; http://dx.doi.org/10.1099/mic.0.2006/001610-0
- Pollack JK, Harris SD, Marten MR. Autophagy in filamentous fungi. Fungal Genet Biol 2009; 46:1-8; PMID:19010432; http://dx.doi.org/10.1016/j.fgb.2008.10.010
- Khan IA, Lu JP, Liu XH, Rehman A, Lin FC. Multifunction of autophagy-related genes in filamentous fungi. Microbiol Res 2012; 167:339-45; PMID:22554685; http://dx.doi.org/10.1016/j.micres.2012.01.004
- Josefsen L, Droce A, Sondergaard TE, Sorensen JL, Bormann J, Schafer W, Giese H, Olsson S. Autophagy provides nutrients for nonassimilating fungal structures and is necessary for plant colonization but not for infection in the necrotrophic plant pathogen Fusarium graminearum. Autophagy 2012; 8:326-37; PMID:22240663; http://dx.doi.org/10.4161/auto.18705
- Kikuma T, Ohneda M, Arioka M, Kitamoto K. Functional analysis of the ATG8 homologue Aoatg8 and role of autophagy in differentiation and germination in Aspergillus oryzae. Eukaryotic Cell 2006; 5:1328-36; PMID:16896216; http://dx.doi.org/10.1128/EC.00024-06
- Veneault-Fourrey C, Barooah M, Egan M, Wakley G, Talbot NJ. Autophagic fungal cell death is necessary for infection by the rice blast fungus. Science 2006; 312:580-3; PMID:16645096; http://dx.doi.org/10.1126/science.1124550
- Nadal M, Gold SE. The autophagy genes atg8 and atg1 affect morphogenesis and pathogenicity in Ustilago maydis. Mol Plant Pathol 2010; 11:463-78; PMID:20618705; http://dx.doi.org/10.1111/j.1364-3703.2010.00620.x
- Voigt O, Poggeler S. Autophagy genes Smatg8 and Smatg4 are required for fruiting-body development, vegetative growth and ascospore germination in the filamentous ascomycete Sordaria macrospora. Autophagy 2013; 9:33-49; PMID:23064313; http://dx.doi.org/10.4161/auto.22398
- Duan Z, Chen Y, Huang W, Shang Y, Chen P, Wang C. Linkage of autophagy to fungal development, lipid storage and virulence in Metarhizium robertsii. Autophagy 2013; 9:538-49; PMID:23380892; http://dx.doi.org/10.4161/auto.23575
- Dean R, Van Kan JA, Pretorius ZA, Hammond-Kosack KE, Di Pietro A, Spanu PD, Rudd JJ, Dickman M, Kahmann R, Ellis J, et al. The Top 10 fungal pathogens in molecular plant pathology. Mol Plant Pathol 2012; 13:414-30; PMID:22471698; http://dx.doi.org/10.1111/j.1364-3703.2011.00783.x
- Nucci M, Anaissie E. Cutaneous infection by Fusarium species in healthy and immunocompromised hosts: implications for diagnosis and management. Clin Infect Dis 2002; 35:909-20; PMID:12355377; http://dx.doi.org/10.1086/342328
- Ortoneda M, Guarro J, Madrid MP, Caracuel Z, Roncero MI, Mayayo E, Di Pietro A. Fusarium oxysporum as a multihost model for the genetic dissection of fungal virulence in plants and mammals. Infect Immun 2004; 72:1760-6; PMID:14977985; http://dx.doi.org/10.1128/IAI.72.3.1760-1766.2004
- Correll JC, Puhalla JE, Schneider RW, Kraft JM. Differentiating races of Fusarium oxysporum f. sp. pisi based on vegetative compatibility. Phytopathology 1985; 75:1347
- Jacobson DJ, Gordon TR. Vegetative compatibility and self-incompatibility within Fusarium oxysporum f. sp. melonis. Phytopathology 1988; 78:668-72; http://dx.doi.org/10.1094/Phyto-78-668
- Mesterhazy A. The morphology of an undescribed form of anastomosis in Fusarium. Mycologia 1973; 65:916-9; PMID:4755866; http://dx.doi.org/10.2307/3758525
- Puhalla JE. Classification of strains of Fusarium oxysporum on the basis of vegetative compatibility. Can J Bot 1985; 63:179-83; http://dx.doi.org/10.1139/b85-020
- Prados Rosales RC, Di Pietro A. Vegetative hyphal fusion is not essential for plant infection by Fusarium oxysporum. Eukaryot Cell 2008; 7:162-71; PMID:18039941; http://dx.doi.org/10.1128/EC.00258-07
- Ruiz-Roldan MC, Kohli M, Roncero MI, Philippsen P, Di Pietro A, Espeso EA. Nuclear dynamics during germination, conidiation, and hyphal fusion of Fusarium oxysporum. Eukaryot Cell 2010; 9:1216-24; PMID:20543061; http://dx.doi.org/10.1128/EC.00040-10
- Kirisako T, Ichimura Y, Okada H, Kabeya Y, Mizushima N, Yoshimori T, Ohsumi M, Takao T, Noda T, Ohsumi Y. The reversible modification regulates the membrane-binding state of Apg8/Aut7 essential for autophagy and the cytoplasm to vacuole targeting pathway. J Cell Biol 2000; 151:263-76; PMID:11038174; http://dx.doi.org/10.1083/jcb.151.2.263
- Klionsky DJ, Abdalla FC, Abeliovich H, Abraham RT, Acevedo-Arozena A, Adeli K, Agholme L, Agnello M, Agostinis P, Aguirre-Ghiso JA, et al. Guidelines for the use and interpretation of assays for monitoring autophagy. Autophagy 2012; 8:445-544; PMID:22966490; http://dx.doi.org/10.4161/auto.19496
- Biederbick A, Kern HF, Elsasser HP. Monodansylcadaverine (MDC) is a specific in vivo marker for autophagic vacuoles. Eur J Cell Biol 1995; 66:3-14; PMID:7750517
- Takeshige K, Baba M, Tsuboi S, Noda T, Ohsumi Y. Autophagy in yeast demonstrated with proteinase-deficient mutants and conditions for its induction. J Cell Biol 1992; 119:301-11; PMID:1400575; http://dx.doi.org/10.1083/jcb.119.2.301
- Nitsche BM, Burggraaf-van Welzen AM, Lamers G, Meyer V, Ram AF. Autophagy promotes survival in aging submerged cultures of the filamentous fungus Aspergillus niger. Appl Microbiol Biotechnol 2013; 97:8205-18; PMID:23700238; http://dx.doi.org/10.1007/s00253-013-4971-1
- Richie DL, Fuller KK, Fortwendel J, Miley MD, McCarthy JW, Feldmesser M, Rhodes JC, Askew DS. Unexpected link between metal ion deficiency and autophagy in Aspergillus fumigatus. Eukaryot Cell 2007; 6:2437-47; PMID:17921348; http://dx.doi.org/10.1128/EC.00224-07
- Leeder AC, Palma-Guerrero J, Glass NL. The social network: deciphering fungal language. Nat Rev Microbiol 2011; 9:440-51; PMID:21572459; http://dx.doi.org/10.1038/nrmicro2580
- Navarro-Velasco GY, Prados-Rosales RC, Ortíz-Urquiza A, Quesada-Moraga E, Di Pietro A. Galleria mellonella as model host for the trans-kingdom pathogen Fusarium oxysporum. Fungal Genet Biol 2011; 48:1124-9; PMID:21907298; http://dx.doi.org/10.1016/j.fgb.2011.08.004
- Pinar M, Pantazopoulou A, Penalva MA. Live-cell imaging of Aspergillus nidulans autophagy: RAB1 dependence, Golgi independence and ER involvement. Autophagy 2013; 9:1024-43; PMID:23722157; http://dx.doi.org/10.4161/auto.24483
- Kiel JA, van der Klei IJ. Proteins involved in microbody biogenesis and degradation in Aspergillus nidulans. Fungal Genet Biol 2009; 46(Suppl 1):S62-71; PMID:18694841; http://dx.doi.org/10.1016/j.fgb.2008.07.009
- Meijer WH, van der Klei IJ, Veenhuis M, Kiel JA. ATG genes involved in non-selective autophagy are conserved from yeast to man, but the selective Cvt and pexophagy pathways also require organism-specific genes. Autophagy 2007; 3:106-16; PMID:17204848; http://dx.doi.org/10.4161/auto.3595
- Ichimura Y, Kirisako T, Takao T, Satomi Y, Shimonishi Y, Ishihara N, Tanida I, Kominami E, Ohsumi M, Noda T, et al. A ubiquitin-like system mediates protein lipidation. Nature 2000; 408:488-92; PMID:11100732; http://dx.doi.org/10.1038/35044114
- Geng J, Klionsky DJ. The Atg8 and Atg12 ubiquitin-like conjugation systems in macroautophagy. 'Protein modifications: beyond the usual suspects' review series. EMBO Rep 2008; 9:859-64; PMID:18704115; http://dx.doi.org/10.1038/embor.2008.163
- Shoji JY, Arioka M, Kitamoto K. Possible involvement of pleiomorphic vacuolar networks in nutrient recycling in filamentous fungi. Autophagy 2006; 2:226-7; PMID:16874107; http://dx.doi.org/10.4161/auto.2695
- Hu G, Hacham M, Waterman SR, Panepinto J, Shin S, Liu X, Gibbons J, Valyi-Nagy T, Obara K, Jaffe HA, et al. PI3K signaling of autophagy is required for starvation tolerance and virulenceof Cryptococcus neoformans. J Clin Invest 2008; 118:1186-97; PMID:18259613; http://dx.doi.org/10.1172/JCI32053
- Glass NL, Rasmussen C, Roca MG, Read ND. Hyphal homing, fusion and mycelial interconnectedness. Trends Microbiol 2004; 12:135-41; PMID:15001190; http://dx.doi.org/10.1016/j.tim.2004.01.007
- Wei H, Requena N, Fischer R. The MAPKK kinase SteC regulates conidiophore morphology and is essential for heterokaryon formation and sexual development in the homothallic fungus Aspergillus nidulans. Mol Microbiol 2003; 47:1577-88; PMID:12622813; http://dx.doi.org/10.1046/j.1365-2958.2003.03405.x
- Pandey A, Roca MG, Read ND, Glass NL. Role of a mitogen-activated protein kinase pathway during conidial germination and hyphal fusion in Neurospora crassa. Eukaryot Cell 2004; 3:348-58; PMID:15075265; http://dx.doi.org/10.1128/EC.3.2.348-358.2004
- Roberts P, Moshitch-Moshkovitz S, Kvam E, O'Toole E, Winey M, Goldfarb DS. Piecemeal microautophagy of nucleus in Saccharomyces cerevisiae. Mol Biol Cell 2003; 14:129-41; PMID:12529432; http://dx.doi.org/10.1091/mbc.E02-08-0483
- Park YE, Hayashi YK, Bonne G, Arimura T, Noguchi S, Nonaka I, Nishino I. Autophagic degradation of nuclear components in mammalian cells. Autophagy 2009; 5:795-804; PMID:19550147; http://dx.doi.org/10.4161/auto.8901
- Liu ML, Yao MC. Role of ATG8 and autophagy in programmed nuclear degradation in Tetrahymena thermophila. Eukaryot Cell 2012; 11:494-506; PMID:22366125; http://dx.doi.org/10.1128/EC.05296-11
- Di Pietro A, Roncero MIG. Cloning, expression, and role in pathogenicity of pg1 encoding the major extracellular endopolygalacturonase of the vascular wilt pathogen Fusarium oxysporum. Mol Plant-Micro Interact 1998; 11:91-8; PMID:9450333; http://dx.doi.org/10.1094/MPMI.1998.11.2.91
- Aljanabi SM, Martinez I. Universal and rapid salt-extraction of high quality genomic DNA for PCR-based techniques. Nucleic Acids Res 1997; 25:4692-3; PMID:9358185; http://dx.doi.org/10.1093/nar/25.22.4692
- Raeder U, Broda P. Rapid preparation of DNA from filamentous fungi. Lett App Microbiol 1985; 1:17-20; http://dx.doi.org/10.1111/j.1472-765X.1985.tb01479.x
- Altschul SF, Gish W, Miller W, Myers EW, Lipman DJ. Basic Local Alignment Search Tool. J Mol Biol 1990; 215:403-10; PMID:2231712; http://dx.doi.org/10.1016/S0022-2836(05)80360-2
- Thompson JD, Higgins DG, Gibson TJ. Clustal W improving the sensitivity of progressive multiple sequence alignment through sequence weighting, position specific gap penalties and weight matrix choice. Nucleic Acids Res 1994; 22:4673-80; PMID:7984417; http://dx.doi.org/10.1093/nar/22.22.4673
- Hall T. BioEdit. <http://wwwmbioncsuedu/BioEdit/bioedithtml> 2004.
- Catlett NL, Lee BN, Poder OC, Turgeon G. Split-Marker recombination for efficient targeted deletion of fungal genes. Fungal Genet Newslett 2003; 50:9-11.
- Carroll AM, Sweigard JA, Valent B. Improved vectors for selecting resistance to hygromycin. Fungal Genet Newslett 1994; 41:22.
- Kück U, Hoff B. Application of the nourseothricin acetyltransferase gene (nat1) as dominant marker for the transformation of filamentous fungi. Fungal Genet Newslett 2006; 53:9-11.
- Lopez-Berges MS, Capilla J, Turra D, Schafferer L, Matthijs S, Jochl C, Cornelis P, Guarro J, Haas H, Di Pietro A. HapX-mediated iron homeostasis is essential for rhizosphere competence and virulence of the soilborne pathogen Fusarium oxysporum. Plant Cell 2012; 24:3805-22; PMID:22968717; http://dx.doi.org/10.1105/tpc.112.098624