Abstract
It is well-known from the model dicotyledonous plants, Arabidopsis and tomato, that jasmonates (JAs) act as defense hormones in planta due to their potent ability to mediate defensive responses against insect/pathogen attacks or harsh environmental conditions. JA is also required for various developmental processes such as male fertility, seed maturation, root extension, and leaf senescence. In our recently published Plant Cell paper, the multiple roles of JA in the monocotyledonous agro-economically important model plant, maize, were investigated by comprehensive analysis of JA-deficient double mutant disrupted in the two oxophytodienoate reductase genes, OPR7 and OPR8. These two genes are the closest orthologs of the Arabidopsis JA-producing OPR3 and are the only maize OPRs required for JA biosynthesis. With this mutant, we previously showed that JA is essential for both male and female reproductive development, and required for the regulation of brace root pigmentation, leaf senescence, and defense against oomycete Pythium aristosporum, and beet armyworm (Spodoptera exigua). In this addendum, we expanded the investigation into the function of JA in elongation of sheaths, leaves, and roots, and its involvement in photomorphogenesis of seedlings.
Abbreviations
JAs | = | jasmonates |
MeJA | = | methyl jasmonate |
OPDA | = | 12-oxo-phytodienoic acid |
OPR | = | 12-oxo-phytodienoate reductase |
In plants, diverse classes of oxidized lipids, collectively known as oxylipins, are essential compounds for the regulation of developmental processes and plant adaptation to diverse biotic and abiotic stresses. Jasmonic acid (JA) and its derivatives including methyl jasmonate (MeJA) and JA-Isoleucine (JA-Ile), referred to as jasmonates (JAs), are undeniably the best understood subfamily of hundreds of molecular species of plant oxylipins. These compounds are extensively studied in dicotyledonous plants such as Arabidopsis and tomato (Solanum lycopersicum), where they were to shown to play pivotal roles in many biological processes, such as seed maturation, reproduction, root growth, tendril coiling, senescence, and defense responses to biotic and abiotic stresses.Citation1 A diverse collection of JA biosynthesis and signaling mutants in Arabidopsis (e.g., fad3/7/82, opr3/dde13, aos/dde24, coronatine insensitive 1 (coi1), Citation5 jin4/jar1, Citation6 and jin1/myc2 Citation7 and in tomato such as def1, Citation8 spr1 Citation9, spr2 Citation10 and jai1/spr6 Citation11) have been identified and widely utilized for JA functional analyses.
Despite the tremendous economic significance of monocot plants such as rice and maize, JA research in these species significantly lags behind research in dicots. Only 2 JA biosynthesis mutants of monocots (hebiba in rice and tasselseed1 (ts1) in maize) have been reported so far. The hebiba mutant is impaired in wound induction of JA, and its mesocotyl and coleoptile have an altered response to red light.Citation12 In ts1, the bisexual tassel primordia develop into pistillate florets, instead of the normally staminate inflorescence, due to reduced JA biosynthesis in tassel primordia.Citation13 TS1 was map-cloned as a 13-lipoxygenase involved in JA biosynthesis.Citation13 More recently, maize JA-deficient double-mutant opr7opr8 Citation14 was constructed, in which oxo-phytodienoate reductase genes, OPR7 and OPR8, were knocked out by insertion of transposon Mutators. The opr7opr8 double-mutant has a systemic JA-deficiency and showed a number of altered phenotypes such as unusual ear shoot elongation, absence of pigmentation in brace roots, delayed leaf senescence, and susceptibility to insects and soil pathogens, as well as tasselseed phenotype.Citation14 These phenotypes helped uncovering diverse JA function in maize in both developmental processes and defense responses. With further analyses of opr7opr8, in this short communication we report additional regulatory roles of JA in maize during growth.
Endogenous JA Acts as a Negative Growth Regulator in Leaves and Roots
Methyl jasmonic acid (MeJA) was first isolated in 1962 as a floral scent compound from the aromatic oil of Jasminum grandiflorum. Citation15 However, the physiological effects of MeJA or its free acid JA remained unknown until the 1980s when a senescence-promoting effect of JA in oat leavesCitation16 In addition, MeJA was found to inhibit growth of rice,Citation17 or Vicia faba Citation18 seedlings. Since then, JA has been widely recognized as a potent plant growth regulator. The ability of JA to dramatically inhibit root length was subsequently exploited in many forward genetic screens for discovery of mutants with decreased sensitivity to JA or MeJA. These screens yielded a number of (Me)JA-insensitive mutants such as jasmonic acid–resistant1 (jar1) with decreased sensitivity to MeJA,Citation19 and coronatine-insensitive1 (coi1) with extreme insensitivity to the bacterial virulence factor and the functional and structural mimic of JA, coronatine, as well as MeJA.Citation5 Now it is known that the JAR1 enzyme catalyzes JA-amino conjugation, including the production of the bioactive JA-signaling ligand, jasmonoyl-isoleucine (JA-Ile),Citation6 and that COI1 functions as a JA receptor.Citation20
The role of JAs in inhibiting growth was demonstrated with exogenous application of JAs on diverse plants. However, the role of endogenous JAs as natural regulators of plant growth and development has been demonstrated by the functional analyses of diverse JA biosynthesis or signaling mutants. Wound-induced JA caused stunted plant growth of wild type but not JA-signaling mutants coi1 or myc2,Citation21 indicating that wound-induced JA signaling suppresses growth of wounded plants. However, to the best of our knowledge, there is no report showing that basal JA levels (which are present at low concentrations) in plants affect growth. In this study, we observed repeatedly that opr7opr8 double mutant grows taller than WT during early developmental stages. Measuring the size of different organs of young seedlings, we found opr7opr8 has significantly longer coleoptile than WT (), indicating that JA plays a negative role in the coleoptile elongation. This phenotype was exacerbated when seedlings were grown under darkness compared with seedlings grown under red light (). These observations suggest that JA is involved in the normal response of the maize coleoptile to light. Additionally, opr7opr8 had a significantly longer sheath of the first leaf compared to WT (). However, such difference was not obserbved for the second leaf sheath and all subsequent leaves, indicating JA inhibits leaf sheath growth of the youngest leaf. Similarly, opr7opr8 had significantly longer leaf blades of the first and second leaves compared with WT (). Similarly to leaf sheath, the third and following leaves showed no significant difference between mutant and WT (data not shown). These results provide strong genetic evidence for the negative role of basal JA in early development of leaf sheath and blades in maize.
Figure 1. JA-deficient mutant opr7opr8 has larger seedling size than WT. (A) opr7opr8 showed longer coleoptile and sheath of first leaf than WT. (B) opr7opr8 showed longer first and second leaf blades than WT. (C) The length measurements of coleoptiles and first and second leaf sheaths and blades of opr7opr8 and WT.
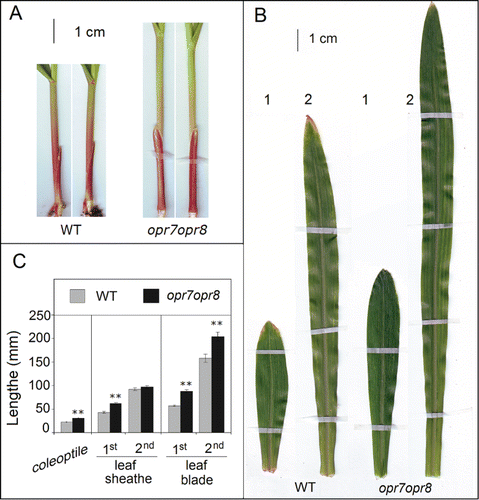
Figure 2. JA inhibits lateral root growth in maize seedlings. WT (A) and Mutant (B) seeds were grown on germination paper at 25°C under white light. Images were taken at sixth day after planting. opr7opr8 (B) showed more and longer lateral roots (indicated by arrows) than WT (A), and similar length of primary roots with WT. Scale bar indicates 1 cm.
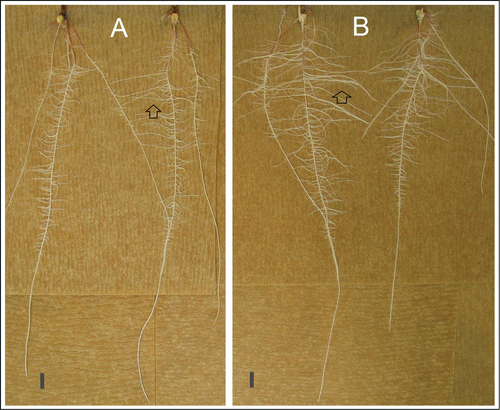
Figure 3. JA is involved in photomorphogenesis of maize coleoptile, indicated by the distinct phenotype of opr7opr8 mutant in darkness (A, B) and red light (C, D). Germinated under darkness at 32°C for 7 days, the mesocotyl of opr7opr8 (B) is much longer than WT (A); germinated under continuous red light at 32°C for 5 days, the mesocotyl and coleoptyl of opr7opr8 (D) is significantly longer than WT (C), while the leaf shoot of the mutant (D) is shorter than WT (C). The arrows indicate the coleoptile node and the scale bar represents 1 cm.
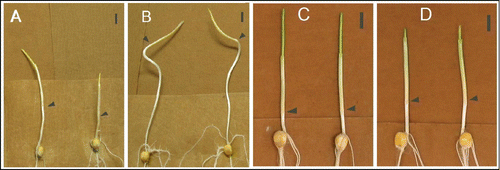
In below-ground tissue, we observed that opr7opr8 seedlings have much longer lateral roots on the primary root compared with WT (). Unexpectedly, the lengths of the primary and secondary roots were similar for both genotypes (). These results indicate that basal JA a negatively regulates formation of lateral root, but not primary or secondary root growth. Combining the previously reported phenotype of “ear-stalk elongation” of opr7opr8, Citation14 we conclude that basal JA functions as a growth inhibitor which is required for normal development of both above- and below-ground organs, including the ear stalk, coleoptile, leaf blade, leaf sheath, and root.
The molecular mechanism of JA inhibition on plant growth remains to be elucidated, but several reports proposed components of the machinery. JA signaling was reported to antagonistically interact with auxin through auxin-signaling element AXR1Citation22 and with GA through GA-signaling protein DELLACitation23 in Arabidopsis. In maize, opr7opr8 was found to contain less ethylene (ET), abscisic acid (ABA), and cytokinin (KT) in senescing leaves,Citation14 indicating a global involvement of JA in the regulation of biosynthesis of several diverse phytohormones that are classically associated with the physiological processes such as leaf senescence, seedling growth and flower fertility.
Endogenous JA is Involved in Photomorphogenesis of Maize
Seeds germinate and establish seedlings under both darkness and light. In darkness, seedlings undergo skotomorphogenesis and develop into “etiolated seedlings” characterized by an elongated hypocotyl, an apical hook with folded cotyledons with undifferentiated chloroplasts (proplastids), and a limited root system.Citation24 Under light, however, seedlings undergo un-etiolated growth, called photomorphogenesis, resulting in green seedlings with a short hypocotyl, 2 opened and expanded cotyledons (in dicots) containing photosynthetically active chloroplasts, and strong root system.Citation24 In the natural environment, soil-buried seedlings use skotomorphogenesis to emerge rapidly through soil in search of light, and then switch to photomorphogenesis to establish photosynthesis.
The light signal is the overarching factor for determining conversion from skotomorphogenesis to photomorphogenesis. Plants perceive light by 4 classes of photoreceptors,Citation25 namely phytochromes, cryptochromes, phototropins, and the UVB (280–320nm) receptor, UVR8. Phytochromes (PHYA to PHYE in Arabidopsis), are red/far-red (FR) light (650–700nm/700–750nm) receptors that constitute the major regulators of photomorphogenesis.Citation24,25 Cryptochromes (CRY1 and CRY2 in Arabidopsis) and phototropins (PHOT1 and PHOT2 in Arabidopsis) perceive UV-A/blue wavelengths (320–500nm). CRYs mediate light control of stem elongation, leaf expansion, photoperiodic flowering, and the circadian rhythm,Citation26 while PHOTs act predominantly in phototropism, chloroplast migration, and stomata opening.Citation27
Many protein factors called phytochrome-interacting proteins (PIPs), such as PIFs (phytochrome-interacting factors), HFR1 (long hypocotyl in far-red1), and FIN219 (far-red insensitive 219), act downstream of phytochromes and associate with distinct photomorphogenic processes.Citation24 For example, PIFs are active in the dark and regulate gene expression to promote skotomorphogenic growth; under light, PIFs interact directly with light-activated phytochromes (in Pfr form) and result in phosphorylation and subsequent degradation of PIFs by the proteasome, promoting photomorphogenesis.Citation28 In addition to PIPs, there is a group of proteins in plants, termed CONSTITUTIVE PHOTOMORPHOGENI-C/DE-ETIOLATED/FUSCA (COP/DET/FUS) that are central repressors of photomorphogenesis.Citation28 In darkness, COP/DET/FUS proteins, such as COP1, work in concert with COP9 signalosome (CSN) and COP10–DET1–DDB1 (CDD) complex for ubiquitylation of photomorphogenesis-promoting transcription factors, such as HY5 and HYH, for proteasome-mediated degradation, preventing photomorphogenesis. In the presence of light, however, the COP/DET/FUS proteins are repressed by signals from the light-activated phytochromes and probably cryptochromes, leading to the accumulation of HY5/HYH and photomorphogenesis.Citation28
Plant hormones including auxin (IAA), gibberellins (GA), ABA, KT, ET, and brassinosteroids (BR) have been shown to be associated with light-signaling steps of photomorphogenesis.Citation24 A good example for the role of auxin came from the studies of ABCB19, a member of a large family of ABC transporters, that was shown to play a role in IAA distribution along the hypocotyl.Citation25 Mutants of ABCB19 were hypersensitive to blue light, and loss of ABCB19 function strongly suppressed phyB and cry1 long hypocotyl phenotypes,Citation25 suggesting proper auxin distribution in the hypocotyl is essential for photomorphogenesis. For GAs, DELLA family proteins, the negative regulators of GA signaling, which are degraded by GA-signaling complex — SCFGID2/SLY1 upon high GA levels, play a central role in GA-IAA photomorphogenesis interaction.Citation25
Jasmonates were found to bear a number of roles that are essential for many developmental and defense responses of plants.Citation14 However, their roles in photomorphogenesis have only begun to be elucidated in recent years. Several lines of evidence have shown that JA acts as an important signal involved in photomorphogenesis. The first indication of a link between JA and light signaling came from the discovery that JA signaling core machinery SCFCOI1 complex interacts physically with one of the light-signaling complexes, COP9 signalosome. This connection was discovered through the analyses of a mutation, which interfered with the expression of COI1-dependent JA-responsive genes.Citation29 far-red-insensitive 219 (fin219), which was originally screened as a repressor of constitutive photomorphogenesis1 (cop1), is allelic to jar1. In addition, all alleles of jar1 are insensitive to FR light with respect to hypocotyl growth, similar to fin219. Citation30 MYC2, a key transcriptional factor in JA signaling pathway, was independently found as a repressor of blue light-mediated photomorphogenesis.Citation31 In rice, coleoptile photomorphogenesis2 (cpm2) and hebiba were found to be defective in the gene encoding allene oxide cyclase (OsAOC) by map-based cloning and complementation assays. Both of these mutants showed long coleoptiles under continuous red light,Citation32 a typical phenotype of photomorphogenesis mutants in monocots, suggesting JA is required for photomorphogenesis growth in monocots. In addition, a knockout mutation in rice homolog JAR1 renders seedlings insensitive to FR and blue light with respect to coleoptile elongation,Citation33 indicating that JA bioactivity is important for photomorphogenesis.
Corresponding to the hypocotyl of dicots, the maize mesocotyl is regarded as the organ responsible for responding to photomorphogenesis. Here we showed that JA-deficient mutant opr7opr8 displayed extremely long mesocotyl compared to WT under darkness (). Moreover, the first leaf of WT emerged from the coleoptile at 7 d after imbibition, but opr7opr8 did not yet emerge at this time (). This result indicates that the JA-deficient mutant undergoes exaggerated skotomorphogenesis in darkness. Under continuous red light, opr7opr8 seedlings had significantly longer mesocotyls and coleoptyle but a shorter leaf bundle compared to WT (), indicating that opr7 opr8 is insensitive to red light, which is required for normal photomorphogenesis. Putting the results from dark and red light experiments together, we conclude that JA is a signal required for photomorphogenesis in maize.
Disclosure of Potential Conflicts of Interest
No potential conflicts of interest were disclosed.
Acknowledgments
We wish to express our appreciation to Dr. Scott Finlayson for his help with these experiments.
Funding
This work was supported by National Science Foundation grant IOS-0925561 to M.K.
References
- Avanci NC, Luche DD, Goldman GH, Goldman MH. Jasmonates are phytohormones with multiple functions, including plant defense and reproduction. Genet Mol Res 2010; 9:484-505; PMID:20391333; http://dx.doi.org/10.4238/vol9-1gmr754
- McConn M, Browse J. The critical requirement for linolenic acid is pollen development, not photosynthesis, in an Arabidopsis mutant. Plant Cell 1996; 8:403-16; PMID:12239389; http://dx.doi.org/10.1105/tpc.8.3.403
- Stintzi A, Browse J. The Arabidopsis male-sterile mutant, opr3, lacks the 12-oxophytodienoic acid reductase required for jasmonate synthesis. Proc Natl Acad Sci USA 2000; 97:10625-30; PMID:10973494; http://dx.doi.org/10.1073/pnas.190264497
- Park JH, Halitschke R, Kim HB, Baldwin IT, Feldmann KA, Feyereisen R. A knock-out mutation in allene oxide synthase results in male sterility and defective wound signal transduction in Arabidopsis due to a block in jasmonic acid biosynthesis. Plant J 2002; 31:1-12; PMID:12100478; http://dx.doi.org/10.1046/j.1365-313X.2002.01328.x
- Feys B, Benedetti CE, Penfold CN, Turner JG. Arabidopsis mutants selected for resistance to the phytotoxin coronatine are male sterile, insensitive to methyl jasmonate, and resistant to a bacterial pathogen. Plant Cell 1994; 6:751-9; PMID:12244256; http://dx.doi.org/10.1105/tpc.6.5.751
- Staswick PE, Tiryaki I. The oxylipin signal jasmonic acid is activated by an enzyme that conjugates it to isoleucine in Arabidopsis. Plant Cell 2004; 16:2117-27; PMID:15258265; http://dx.doi.org/10.1105/tpc.104.023549.
- Lorenzo O, Chico JM, Sanchez-Serrano JJ, Solano R. jasmonate-insensitive1 encodes a MYC transcription factor essential to discriminate between different jasmonate-regulated defense responses in Arabidopsis. Plant Cell 2004; 16:1938-50; PMID:15208388; http://dx.doi.org/10.1105/tpc.022319
- Howe GA, Lightner J, Browse J, Ryan CA. An octadecanoid pathway mutant (JL5) of tomato is compromised in signaling for defense against insect attack. Plant Cell 1996; 8:2067-77; PMID:8953771; http://dx.doi.org/10.1105/tpc.8.11.2067
- Lee GI, Howe GA. The tomato mutant spr1 is defective in systemin perception and the production of a systemic wound signal for defense gene expression. Plant J 2003; 33:567-76; PMID:12581314; http://dx.doi.org/10.1046/j.1365-313X.2003.01646.x
- Li C, Liu G, Xu C, Lee GI, Bauer P, Ling H-Q, Ganal MW, Howe GA. The tomato suppressor of prosystemin-mediated responses2 gene encodes a fatty acid desaturase required for the biosynthesis of jasmonic acid and the production of a systemic wound signal for defense gene expression. Plant Cell 2003; 15:1646-61; PMID:12837953; http://dx.doi.org/10.1105/tpc.012237
- Li L, Zhao Y, McCaig BC, Wingerd BA, Wang J, Whalon ME, Pichersky E, Howe GA. The tomato homolog of coronatine-insensitive1 is required for the maternal control of seed maturation, jasmonate-signaled defense responses, and glandular trichome development. Plant Cell 2004; 16:126-43; PMID:14688297; http://dx.doi.org/10.1105/tpc.017954
- Riemann M, Muller A, Korte A, Furuya M, Weiler EW, Nick P. Impaired induction of the jasmonate pathway in the rice mutant hebiba. Plant Physiol 2003; 133:1820-30; PMID:14605232; http://dx.doi.org/10.1104/pp.103.027490
- Acosta IF, Laparra H, Romero SP, Schmelz E, Hamberg M, Mottinger JP, Moreno MA, Dellaporta SL. Tasselseed1 is a lipoxygenase affecting jasmonic acid signaling in sex determination of maize. Science 2009; 323:262-5; PMID:19131630; http://dx.doi.org/10.1126/science.1164645
- Yan Y, Christensen S, Isakeit T, Engelberth J, Meeley R, Hayward A, Emery RJ, Kolomiets MV. Disruption of OPR7 and OPR8 reveals the versatile functions of jasmonic acid in maize development and defense. Plant Cell 2012; 24:1420-36; PMID:22523204; http://dx.doi.org/10.1105/tpc.111.094151
- Demole E, Lederer E, Mercier D. Isolement et détermination de la structure du jasmonate de méthyle, constituant odorant caractéristique de l’essence de jasmin. Helvetica Chimica Acta 1962; 45:675-85; http://dx.doi.org/10.1002/hlca.19620450233
- Ueda J, Kato J. Isolation and identification of a senescence-promoting substance from wormwood (Artemisia absinthium L.). Plant Physiol 1980; 66:246-9; PMID:16661414; http://dx.doi.org/10.1104/pp.66.2.246
- Yamane H, Sugawara J, Suzuki Y, Shimamura E, Takahashi N. Syntheses of jasmonic acid related-compounds and their structure-activity-relationships on the growth of tice seedings. Agr Biol Chem 1980; 44:2857-64; http://dx.doi.org/10.1271/bbb1961.44.2857
- Dathe W, Ronsch H, Preiss A, Schade W, Sembdner G, Schreiber K. Endogenous plant hormones of the broad bean, Vicia faba L. (-)-jasmonic acid, a plant growth inhibitor in pericarp. Planta 1981; 153:530-5; PMID:24275871; http://dx.doi.org/10.1007/BF00385537
- Staswick PE, Su W, Howell SH. Methyl jasmonate inhibition of root growth and induction of a leaf protein are decreased in an Arabidopsis thaliana mutant. Proc Natl Acad Sci USA 1992; 89:6837-40; PMID:11607311; http://dx.doi.org/10.1073/pnas.89.15.6837
- Katsir L, Schilmiller AL, Staswick PE, He SY, Howe GA. COI1 is a critical component of a receptor for jasmonate and the bacterial virulence factor coronatine. Proc Natl Acad Sci USA 2008; 105:7100-5; PMID:18458331; http://dx.doi.org/10.1073/pnas.0802332105
- Zhang Y, Turner JG. Wound-induced endogenous jasmonates stunt plant growth by inhibiting mitosis. PLoS ONE 2008; 3:e3699; PMID:19002244; http://dx.doi.org/10.1371/journal.pone.0003699
- Tiryaki I, Staswick PE. An Arabidopsis mutant defective in jasmonate response is allelic to the auxin-signaling mutant axr1. Plant Physiol 2002; 130:887-94; PMID:12376653; http://dx.doi.org/10.1104/pp.005272
- Cheng H, Song S, Xiao L, Soo HM, Cheng Z, Xie D, Peng J. Gibberellin acts through jasmonate to control the expression of MYB21, MYB24, and MYB57 to promote stamen filament growth in Arabidopsis. PLoS Genet 2009; 5:e1000440; PMID:19325888; http://dx.doi.org/10.1371/journal.pgen.1000440
- Han YJ, Song PS, Kim JI. Phytochrome-mediated photomorphogenesis in plants. J Plant Biol 2007; 50:230-40; http://dx.doi.org/10.1007/BF03030650
- Arsovski AA, Galstyan A, Guseman JM, Nemhauser JL. Photomorphogenesis. The Arabidopsis Book American Society of Plant Biologists 2012; 10:e0147
- Yu X, Liu H, Klejnot J, Lin C. The cryptochrome blue light receptors. The Arabidopsis Book American Society of Plant Biologists 2010; 8:e0135; http://dx.doi.org/10.1199/tab.0135
- Briggs WR, Christie JM. Phototropins 1 and 2: versatile plant blue-light receptors. Trends Plant Sci 2002; 7:204-10; PMID:11992825; http://dx.doi.org/10.1016/S1360-1385(02)02245-8
- Lau OS, Deng XW. Plant hormone signaling lightens up: integrators of light and hormones. Curr Opin Plant Biol 2010; 13:571-7; PMID:20739215; http://dx.doi.org/10.1016/j.pbi.2010.07.001
- Feng SH, Ma LG, Wang XP, Xie DX, Dinesh-Kumar SP, Wei N, Deng XW. The COP9 signalosome interacts physically with SCFCOI1 and modulates jasmonate responses. Plant Cell 2003; 15:1083-94; PMID:12724535; http://dx.doi.org/10.1105/tpc.010207
- Chen IC, Huang IC, Liu MJ, Wang ZG, Chung SS, Hsieh HL. Glutathione S-transferase interacting with far-red insensitive 219 is involved in phytochrome A-mediated signaling in Arabidopsis. Plant Physiol 2007; 143:1189-202; PMID:17220357; http://dx.doi.org/10.1104/pp.106.094185
- Yadav V, Mallappa C, Gangappa SN, Bhatia S, Chattopadhyay S. A basic helix-loop-helix transcription factor in Arabidopsis, MYC2, acts as a repressor of blue light–mediated photomorphogenic growth. Plant Cell 2005; 17:1953-66; PMID:15923349; http://dx.doi.org/10.1105/tpc.105.032060
- Riemann M, Haga K, Shimizu T, Okada K, Ando S, Mochizuki S, Nishizawa Y, Yamanouchi U, Nick P, Yano M, et al. Identification of rice allene oxide cyclase mutants and the function of jasmonate for defence against magnaporthe oryzae. The Plant J 2013; 74:226-38; http://dx.doi.org/10.1111/tpj.12115
- Riemann M, Riemann M, Takano M. Rice jasmonate resistant1 is involved in phytochrome and jasmonate signalling. Plant Cell Environ 2008; 31:783-92; PMID:18266905; http://dx.doi.org/10.1111/j.1365-3040.2008.01790.x