Abstract
Over the past decades, several studies indicate a correlation between the phytohormone auxin and cell division. The molecular players of this signaling pathway are now being uncovered. DNA Binding Protein1 from Arabidopsis (AtDBP1) is an auxin-inducible gene able to bind DNA non-specifically. In this work the tissue-expression pattern of this gene was investigated. Promoter-GUS analysis demonstrated that the AtDBP1 promoter is active in regions exhibiting intense cell division such as meristems and nematode feeding sites. Also, the promoter expression was modulated upon incubation with cell cycle blockers, indicating a potential role in cell division for this gene. Lastly, AtDBP1 antisense plants presented a higher insensitivity to auxin, and interfered negatively with auxin–induced callus formation and reduced apical dominance.
Abbreviation
AtDBP1 | = | DNA binding protein1 from Arabidopsis thaliana |
Since the first attempts to culture plant cell or tissues in vitro, studies with the phytohormone auxin indicated that it plays a role in cell division (reviewed in refs.Citation1,2). Auxin is necessary for the stimulation of cell division in plant tissue culture and experimental data suggest that it provides competence for the G1/S transition.Citation3,4
Here, we present a functional analysis of Arabidopsis DNA Binding Protein1 (AtDBP1). Previous work has shown that AtDBP1 is ubiquitously expressed with higher transcript levels detected in the inflorescence zone of peduncles and flowers. In situ hybridization experiments conducted in roots indicate that AtDBP1 expression is induced in the division zone compared to the elongation zone. Also, AtDBP1 transcript levels were up-regulated in the presence of auxin. AtDBP1 protein expressed in bacteria was able to bind DNA nonspecifically.Citation5 Yamada and collaboratorsCitation6 have shown that AtDBP1 and its close homolog AtDBP2 physically interact in yeast and in vitro with ARR4. ARR4 is a transcriptional repressorCitation7 that promotes shoot formation in the presence of cytokinin.Citation8
In order to study the expression pattern of AtDBP1, a translational fusion with the GUS gene was obtained. The final construct contains 1.85 Kb from the promoter region, the 5′ untranslated sequence and the first 9 amino acids of AtDBP1 fused to the coding region of the GUS gene. This construct was used to transform Arabidopsis plants. Ten Arabidopsis independent transgenic lines presenting the same expression pattern were obtained.
In Arabidopsis, pAtDBP1:GUS expression can be observed in plantlets analyzed 7 days after germination, in which the promoter was active in the shoot apical meristem, root vasculature and root meristem. Also, expression could be observed at the tip of the cotyledons, a known site of auxin accumulationCitation9 (). During the development of the first leaves the expression in shoot meristems was increased being also detected on the vascular tissues of hypocotyls (). In the root system of mature plants, the AtDBP1 promoter was active in the root meristem, lateral root initiation and vascular tissues (). The meristem expression pattern of AtDBP1 promoter hints at a potential role in cell division.
Figure 1. Expression and localization of AtDBP1. (A) 8 DAG seedlings, (B) 15 DAG shoot apice, (C) hypocotyl-root transition zone showing lateral roots, (D) cross section of inflorescence axis, (E) flower bud, (F) early stage of open flower, (G) late stage of open flower, (H) silique base, (I) mature embryo, (J and K) 6-day post-infection root—(J) infection with Meloidogyne incognita, (K) Heterodera schachtii, (L–N) 2 week-old roots incubated for 16-20 hours with cell-cycle blockers—(L) control, (M) 100 μg/ml hydroxyurea, (N) 15 μM oryzalin, (O, P, U, and V) SAM sections—(O) anti-AtDBP1, (P) DAPI staining, (U) pre-immune serum, (V) anti-AtDBP1 of previously blocked tissue with bacteria-expressed AtDBP1, (Q and R) vascular bundle—(Q) anti-AtDBP1, (R) DAPI staining, (S and T) leaf primordial—(S) anti-AtDBP1, (T) DAPI staining.
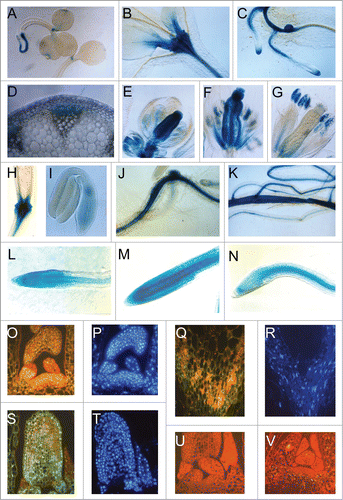
In the Inflorescence axis of Arabidospis the gene was expressed specifically on phloem tissues (). During flower development, the activity of AtDBP1 promoter was shown to be highly modulated. The GUS gene was highly expressed in the ovaries and stigma from flower buds () to open flowers (). In mature flowers, the expression in ovary tissues and stigma was lost (). In the anther, the expression was detected in pollen grains (). During fruit development, the expression of the GUS gene became restricted to the basal part of siliques () and to the hypocotyl-root axis of immature embryos ().
Taken the fact that AtDBP1 is an auxin-induced DNA binding proteinCitation5, highly expressed in shoot and root meristems, which are target sites for auxin action and also areas of intense cell division (reviewed in ref.Citation10), the AtDBP1 promoter activity in non-meristematic cells stimulated to cell division was investigated. In Arabidopsis, cytological observations and molecular markers assays have shown that both root-knot and cyst nematode infection induces large multinucleated feeding cells in the roots, where the parasites induce cycles of cell divisions and DNA endoreduplication.Citation11,12
Transgenic plants transformed with AtDBP1 promoter-GUS construct were infected with Meloidogyne incognita and Heterodera schachtii and GUS expression observed after infection. With both parasites, an increase in GUS expression could be detected 2 days after infection (). This expression is still observed 6 days post inoculation (data not shown). These results indicate that AtDBP1 promoter is expressed not only in meristems but also in non-meristematic cells upon induction of cell division.
In order to verify the possible regulation of AtDBP1 promoter during the progression of the cell cycle, its expression in roots treated with cell cycle blockers was analyzed. Two week-old plants were transferred to medium containing either hydroxyurea which blocks cycling cells at the onset of S phase or to medium containing oryzalin, which arrests the cell cycle at G2/prophase transition.Citation13,12 After 18 hours of incubation, the plants were submitted to GUS assays. Each assay was repeated 5 times with 5 homozygous transgenic lines. When compared with the controls without inhibitor (), hydroxyurea treatment increased GUS expression (), while oryzalin reduced the promoter activity () of root apices. These results indicate that the transcriptional activity of AtDBP1 promoter oscillates during the cell cycle progression, being more active in the S phase than at G2/M transition. This cell-cycle regulation of expression corroborates the proposition of AtDBP1 being involved in cell division.
Polyclonal antibodies against AtDBP1 were prepared using a protein fusion expressed in E. coli.Citation5 Since the DNA binding activity of AtDBP1 has been demonstrated previously,Citation5 we decided to examine the possible nuclear localization of this protein.
Fluorescence immunolocalization experiments were performed in order to confirm the predicted nuclear localization of AtDBP1. These experiments were conducted on the tissues where AtDBP1 promoter shows the highest transcriptional activities. Intense staining could be observed in the cross sections of shoot meristem (), vascular tissues () and young leaves (). Controls with pre-immune serum () and with antibodies pre-incubated with purified bacterial expressed AtDBP1 () confirm the specificity of the staining. DAPI staining of the same sections () indicates a co-localization of nucleus and the AtDBP1 immunostaining, confirming that AtDBP1 is a nuclear protein. The nuclear localization of AtDBP1, together with it in vitro DNA binding properties, further supports the proposition of it presenting an in vivo property of DNA binding protein.
In order to investigate the functional role of AtDBP1, 2four transgenic lines carrying an antisense construct of this gene were obtained. Those lines were analyzed through protein blots as a manner to select the lines presenting undetectable levels of the AtDBP1 protein. The protein blot of exemplary lines is shown (). Lines with extremely reduced AtDBP1 levels or that could not be detected in the protein blot were used for further functional analysis.
Figure 2. Phenotypic analysis of AtDBP1 antisense plants. (A) Western blot of antisense plants with anti-AtDBP1. C24—wild-type plant, AS#1 and AS#2–2 independent AtDBP1 antisense lines, rec ptn—bacteria-expressed AtDBP1, (B) 8 week-old plants presenting loss of apical dominance phenotype of the AtDBP1 antisense plants, (C–F) effect of 2,4-D on 3 DAG plants grown of the hormone for 30 (C and D) or 45 (E and F) days. (C and D) plants grown on 1 μM 2,4-D, (E and F) plants grown on 0.5 μM 2,4-D—(C and E) wild-type C24 plants, (D and F) AtDBP1 antisense plants.
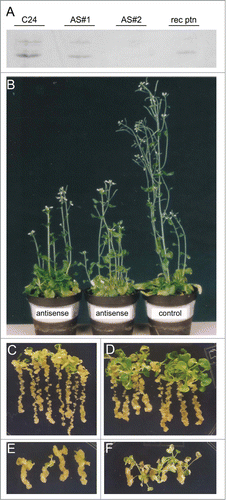
Apical dominance is lost in antisense plants corroborating the role of AtDBP1 gene in auxin signaling (). This loss indicates a lower auxin sensitivity of AtDBP1 antisense plants and suggests that auxin signaling is partially impaired in these plants.
Through experiments using the synthetic auxin 2,4-D, an auxin resistance phenotype in the aerial parts of antisense plants could be observed. Wild type plants incubated for 30 days on 1 μM () or for 45 days on 0.5 μM () of 2,4-D presented formation of undifferentiated tissue on their leaves and roots. On the other hand, antisense plants under the same conditions presented undifferentiated tissue restricted to the roots (), indicating that their aerial parts are resistant to auxin and AtDBP1 is necessary for 2,4-D-induced callus. This result corroborates the proposition that auxin signaling is affected in AtDBP1 antisense plants. Taken together, AtDBP1 plays a role in auxin-related developmental processes affecting vegetative and reproductive organ production.
Material and Methods
Plant growth conditions were performed according to Mangeon et al.Citation14 Transformation procedures were performed according to Clarke et al.Citation15 Histochemical GUS assays were performed according to Mangeon et al.Citation16 Nematode inoculation was conducted as described in Almeida-Engler et al.Citation12 Five to 10 plants were examined for the presence of GUS activity. Hydroxyurea and Oryzalin treatments were performed as previously describedCitation12 with the following modifications: concentration of hydroxyurea and oryzalin was 100 μg/mL and 15μM, respectively, and incubation time was 16-20 hours. Ten plants were examined for the presence of GUS activity. For the auxin sensitivity experiments, 3 DAG plants were transferred for medium containing different concentrations of 2,4-D (1 or 0.5 μM) and grown for 30 or 45 days.
The genomic clone of AtDBP1 was previously isolated from an Arabidopsis thaliana genomic library.Citation5 A BamHI–HincII fragment containing 1.85 Kb promoter region, the 5′ untranslated sequence and the first 9 aminoacids of AtDBP1 was isolated from plasmid pATC-10.Citation5 The GUS gene was isolated from the pHW16 vector after digestion with Asp718, klenow treatment and PstI digestion. These 2 fragments were fused together with a PstI—BamHI fragment from the pLK33 vector, resulting in the recombinant plasmid pAtDBP1-GUS. pAtDBP1-GUS was digested with XbaI, treated with klenow and digested with SmaI. After ligation with HindIII adaptors, the fragment was cloned as a HindIII fragment in the plant transformation vector pGSFR760A (Plant Genetic Systems N.V.) giving rise to the plasmid pTDO2.
The cDNA clone of AtDBP1 was previously isolated from an Arabidopsis thaliana cDNA library.Citation5 A BamHI fragment was isolated from plasmid pATC1ACitation5 and ligated to pGSJ780A (Plant Genetic Systems N.V.) T-DNA vector under the control of CaMV 35S promoter. The clones were checked for cDNA antisense orientation giving rise to the plasmid pTDO3.
Immunolocalization experiments were performed according to Clément et al.Citation17 with the following modifications: the dilution of primary antibody was 50-fold and the secondary antibody was FITC-labeled goat anti-rabbit (polyclonal AB). Anti-DBP polyclonal antibodies were prepared against a purified fusion protein expressed in Escherichia coli as previous described.Citation5 After a pre-purification with H2SO4 extraction, the AtDBP1 fusion protein was purified in S-Sepharose column. Fusion proteins purified after preparative SDS-PAGE were used for immunisation of rabbits. For nucleus identification, sections were washed with PBS buffer and then incubated with DAPI 1 μg/mL diluted in PBS buffer for 1 min at room temperature. The samples were washed 3 times in PBS buffer for 5 min and kept in the dark at 4°C until microscope analysis.
Disclosure of Potential Conflicts of Interest
No potential conflicts of interest were disclosed.
Acknowledgments
The authors would like to thank to Luiza da Silva for technical support (FAPERJ Technical Assistant Fellowship).
Funding
A.B was supported by a CNPq-Rhae fellowship, A.M. was supported by Postdoctoral fellowship from CNPq and PNPD-Capes. This work was supported by grants from the Conselho Nacional de Desenvolvimento Científico e Tecnológico (CNPq) and the Fundação Carlos Chagas Filho de Amparo à Pesquisa do Estado do Rio de Janeiro (FAPERJ) to G.S.M.
References
- Benjamins R, Scheres B. Auxin: the looping star in plant development. Annu Rev Plant Biol 2008; 59:443-65; PMID:18444904; http://dx.doi.org/10.1146/annurev.arplant.58.032806.103805
- Mockaitis K, Estelle M. Auxin receptors and plant development: a new signaling paradigm. Annu Rev Cell Dev Biol 2008; 24:55-80; PMID:18631113; http://dx.doi.org/10.1146/annurev.cellbio.23.090506.123214
- Stals H, Inzé D. When plant cells decide to divide. Trends Plant Sci 2001; 6:359-64; PMID:11495789; http://dx.doi.org/10.1016/S1360-1385(01)02016-7
- Inze D, De Veylder L. Cell cycle regulation in plant development. Annu Rev Genet 2006; 40:77-105; PMID:17094738; http://dx.doi.org/10.1146/annurev.genet.40.110405.090431
- Alliotte T, Tiré C, Engler G, Peleman J, Caplan A, Van Montagu M, Inzé D. An auxin-regulated gene of Arabidopsis thaliana encodes a DNA-binding protein. Plant Physiol 1989; 89:743-52; PMID:16666616; http://dx.doi.org/10.1104/pp.89.3.743
- Yamada H, Hanaki N, Imamura A, Ueguchi C, Mizuno T. An Arabidopsis protein that interacts with the cytokinin-inducible response regulator, ARR4, implicated in the His-Asp phosphorylay signal transduction. FEBS Lett 1998; 436:76-80; PMID:9771897; http://dx.doi.org/10.1016/S0014-5793(98)01103-X
- Hwang I, Sheen J. Two-component circuitry in Arabidopsis cytokinin signal transduction. Nature 2001; 413:383-9; PMID:11574878; http://dx.doi.org/10.1038/35096500
- Osakabe Y, Miyata S, Urao T, Seki M, Shinozaki K, Yamaguchi-Shinozaki K. Overexpression of Arabidopsis response regulators, ARR4/ATRR1/IBC7 and ARR8/ATRR3, alters cytokinin responses differentially in the shoot and in callus formation. Biochem Biophys Res Comm 2002; 293:806-15; PMID:12054542; http://dx.doi.org/10.1016/S0006-291X(02)00286-3
- Benková E, Michniewicz M, Sauer M, Teichmann T, Seifertová D, Jürgens G, Friml J. Local, efflux-dependent auxin gradients as a common module for plant organ formation. Cell 2003; 115:591-602; http://dx.doi.org/10.1016/S0092-8674(03)00924-3
- Perrot-Rechenmann C. Cellular responses to auxin: division versus expansion. Cold Spring Harb Perspect Biol 2010; 2:a001446; PMID:20452959; http://dx.doi.org/10.1101/cshperspect.a001446
- Niebel A, de Almeida Engler J, Hemerly A, Ferreira P, Inzé D, Van Montagu M, Gheysen G. Induction of cdc2a and cyc1At expression in Arabidopsis thaliana during early phases of nematode-induced feeding cell formation. Plant J 1996; 10:1037-43; PMID:9011085; http://dx.doi.org/10.1046/j.1365-313X.1996.10061037.x
- Almeida-Engler J, De Vleesschauwer V, Burssens S, Celenza JL Jr, Inzé D, Van Montagu M, Engler G, Gheysen G. Molecular markers and cell cycle inhibitors show the importance of cell cycle progression in nematode-induced galls and syncytia. Plant Cell 1999; 11:793-808; PMID:10330466; http://dx.doi.org/10.1105/tpc.11.5.793
- Segers G, Gadisseur I, Bergounioux C, de Almeida Engler J, Jacqmard A, Van Montagu M, Inzé D. The Arabidopsis cyclin-dependent kinase gene cdc2bAt is preferentially expressed during S and G2 phases of the cell cycle. Plant J 1996; 10:601-12; http://dx.doi.org/10.1046/j.1365-313X.1996.10040601.x
- Mangeon A, Magioli C, Menezes-Salgueiro AD, Cardeal V, de Oliveira C, Galvão VC, Margis R, Engler G, Sachetto-Martins G. AtGRP5, a vacuole-located glycine-rich protein involved in cell elongation. Planta 2009; 230:253-65; PMID:19434422; http://dx.doi.org/10.1007/s00425-009-0940-4
- Clarke MC, Wei W, Lindsey K. High-frequency transformation of A. thaliana by A. tumefaciens. Plant Mol Biol Rep 1992; 10:178-89; http://dx.doi.org/10.1007/BF02668345
- Mangeon A, Magioli C, Tarré E, Cardeal V, Araujo C, Falkenbach E, Rocha CAB, Rangel-Lima C, Sachetto-Martins G. The tissue expression pattern of the AtGRP5 regulatory region is controlled by a combination of positive and negative elements. Plant Cell Rep 2010; 29:461-71; PMID:20195610; http://dx.doi.org/10.1007/s00299-010-0835-7
- Clément M, Ketelaar T, Rodiuc N, Banora MY, Smertenko A, Engler G, Abad P, Hussey PJ, Almeida-Engler J. Actin-depolymerizing factor2-mediated actin dynamics are essential for root-knot nematode infection of Arabidopsis. Plant Cell 2009; 21:2963-79; http://dx.doi.org/10.1105/tpc.109.069104