Abstract
Plant endoparasitic nematodes, root-knot and cyst nematodes (RKNs and CNs) induce within the root vascular cylinder transfer cells used for nourishing, termed giant cells (GCs) and syncytia. Understanding the molecular mechanisms behind this process is essential to develop tools for nematode control. Based on the crucial role in gall development of LBD16, also a key component of the auxin pathway leading to the divisions in the xylem pole pericycle during lateral root (LR) formation, we investigated genes co-regulated with LBD16 in different transcriptomes and analyzed their similarities and differences with those of RKNs and CNs feeding sites (FS). This analysis confirmed LBD16 and its co-regulated genes, integrated in signaling cascades mediated by auxins during LR and callus formation, as a particular feature of RKN-FS distinct to CNs. However, LBD16, and its positively co-regulated genes, were repressed in syncytia, suggesting a selective down- regulation of the LBD16 auxin mediated pathways in CNs-FS. Interestingly, cytokinin-induced genes are enriched in syncytia and we encountered similarities between the transcriptome of shoot regeneration from callus, modulated by cytokinins, and that of syncytia. These findings establish differences in the regulatory networks leading to both FS formation, probably modulated by the auxin/cytokinin balance.
Plant endoparasitic nematodes (PPNs), root-knot and cyst nematodes (RKNs and CNs) induce their feeding cells from vascular cell precursors within the root. The feeding cells from RNKs (Meloidogyne spp.), called giant cells (GCs), are abnormally large cells induced by nematode effectors in the vascular cylinder that undergo repeated mitosis with aborted cytokinesis,Citation1-3 transforming them into transfer-like cells that the nematode uses as sinks of nutrients from the plant.Citation4 Additionally, vascular cells around the GCs divide and increase in number, and cortex cells become hypertrophic forming the typical galls or knots in the roots associated to the infection of these nematodes. In the case of CNs (Heterodera spp and Globodera spp.), a syncytium is formed inside the roots by gradual incorporation of neighboring vascular cells.Citation5 The development of the PPNs inside the root is entirely dependent on the development of the GCs or syncytia. Therefore, knowledge of the molecular mechanisms behind this process is required to develop biotechnological tools against these plagues that cause high economic losses around the world.Citation6
We have previously addressed the role during gall development of LBD16, Citation7 a key component of the auxin pathway leading to the divisions in the xylem pole pericycle (XPP) during lateral root (LR) formation.Citation8 We found that Arabidopsis XPP marker lines (J0121 and J0192; both induced specifically in the xylem pole pericycle of rootsCitation9) were activated in early developing galls/GCs. The activation pattern of pLBD16::GUS resembled that of the G2/M-transition specific ProCycB1;1:CycB1;1(NT)-GUS line and DR5::GUS in early infection stages. LBD16 was regulated by auxins not only in LR, but also in galls, and induced by RKN secretions in protoplasts. Infection tests of several independent LBD16 loss-of-function lines showed a significant reduction in the infection rate of M. javanica and M. arenaria.Citation7 The promoter of LBD16 was not induced within the syncytia formed by H. schachtii in Arabidopsis and, accordingly, the most severe loss of function line did not show an evident effect during the infection with CNs.Citation7 The specific activation of 2 XPP marker lines and the function of LBD16 during RKN infection connect LR initial cells identity with gall and GC formation.
To further investigate the putative role of LBD16 in Arabidopsis galls and GCs, we have here identified the 50 genes with the highest positive, and the 50 genes with the highest negative correlation values (according to their Pearson correlation coefficients) with LBD16 across all the “Perturbations” microarray experiments released at August 2014 from Genevestigator (i.e., those genes with a positive correlation in different experiments were considered positively co-regulated with LBD16, and those with a negative correlation were considered co-regulated negatively with LBD16). We then downloaded their expression values (log2 values, referred always to the controls used in each experiment) in the different transcriptomes available from Arabidopsis infected with RKNs and cyst nematodes, using our previously designed tool NEMATIC.Citation10 LBD16 was up-regulated in the early-medium stages of GC and gall developmentCitation7,11 while it was down-regulated in microaspirated syncytia.Citation12 From the 50 positively co-regulated genes from Genevestigator, 20 were differentially expressed (DE) also in the plant-nematode transcriptomes (Table S1). As expected, due to the opposite regulation pattern of LBD16 in syncytia as compared to galls and GCs, most of the positively co-regulated genes with LBD16 were also upregulated in galls and GCs, but downregulated in syncytia. Among them, we found other LBD genes co-regulated with LBD16, i.e., LBD17, LBD18 or LBD33. Additionally, from the 20 LBD16 co-regulated genes and DE in the RKN and CN transcriptomes, 16 were up-regulated by exogenous auxin treatment,Citation13 (Table S1) reinforcing the importance of LBD16 in the galls auxin- mediated signaling. The fact that LBD16 and auxin inducible co-regulated genes were repressed in syncytia (Table S1) suggested a selective down- regulation of the LBD16 auxin mediated pathways in the CNs feeding sites, in contrast to RKNs. These results are in accordance to the absence of phenotype in LBD16 loss-of-function plants infected with Heterodera sppCitation7 regardless of other auxin-regulated genes induced in syncytia (for a review see refs. 3, 14). Similar results were obtained for the genes co-regulated negatively with LBD16, as they were found mainly down-regulated in galls and GCs while they showed a significant up-regulation in the CNs feeding sites (Table S2). Strikingly, from the 41 negatively co-regulated genes that were upregulated in syncytia, 28 belonged to the category of photosynthesis from Mapman,Citation15 (Table S2) a characteristic of the syncytia transcriptome that is different to that of GCs.Citation12,16 In agreement to this, differentiation of plastids occurs in the syncytium.Citation12 Therefore, the expression patterns and the molecular network of co-regulated genes with LBD16 in other biological processes seems also conserved during the RKNs feeding site development.
To deepen in the putative role of LBD16 and its co-regulated genes in PPN feeding sites, we compared their expression values in GCs, galls and syncytia with the expression values that they showed in the 3287 transcriptomes deposited in the “Perturbations” section of Genevestigator.Citation17 The most similar expression profile for the pool of genes co-regulated with LBD16 in RKN feeding sites was found in the transcriptome of Arabidopsis explants incubated on solid callus inducing media ( and S1), while those co-regulated with syncytia showed similar expression values to the transcriptomes of callus in the process of shoot regeneration ( and S2). In this last study, LBD16 and LBD18 were downregulated as they are in syncytia, suggesting that they should be repressed during the developmental shift from callus to shoot development. Moreover, during shoot regeneration from callus, induction of photosynthetic genes is describedCitation18 similarly to syncytia.Citation12 These results reinforce the molecular link between callus and gall formation suggested in Cabrera et al.Citation7 with LBD16 and other LBD genes as key players during these processes.Citation19 However, the down regulation of LBD16 and co-regulated genes in syncytia suggest that this pathway should be repressed during syncytia development, probably to favor developmental programs related to shoot regeneration from callus. Interestingly, the balance between auxins and cytokinins is crucial for the maintenance or progression to shoot from callus.Citation20 In this respect, the proportion of cytokinin up-regulated genes in syncytia is much higher than those induced by auxins,Citation10 which is indispensable for the shoot regeneration from callus.Citation19 This is also in accordance to the fact that plastids develop in syncytia,Citation12 as plastid development is induced in darkness in the presence of cytokinins,Citation21 a similar situation that might be happening in syncytia. This is also in accordance to the fact that genes encoding chloroplast proteins were among the most strongly upregulated genes in early forming syncytia.Citation12 However, a higher proportion of auxin than cytokinin inducible genes is necessary for callus induction,Citation18 equivalent to that of early developing Arabidopsis GCs.Citation10
Figure 1. A simplified model showing the general results of in silico comparisons of the GCs and galls transcriptomes highlighting similarities to that of hormone-regulated genes and those co-expressed with LBD16. The most similar expression profile for the pool of genes co-regulated with LBD16 in galls and GCs was found in the transcriptome of Arabidopsis explants incubated on solid callus inducing media while those co-regulated in syncytia showed similar expression values to the transcriptomes of callus in the process of shoot regeneration indicated in the diagram. GUS activity of a DR5::GUS line on a 4 dpi gall showing intense staining in GCs. Syncytia show abundant chlorophyll fluorescence under fluorescence microscopy indicative of the presence of chloroplasts. N, nematode; all giant cells are marked with an asterisk. Drawings of callus in white boxes and their connections to the syncytia, GCs and galls microarray data are based on in silico analysis from the microarray experiments in Genevestigator released at August 2014 (Fig S1 and S2).
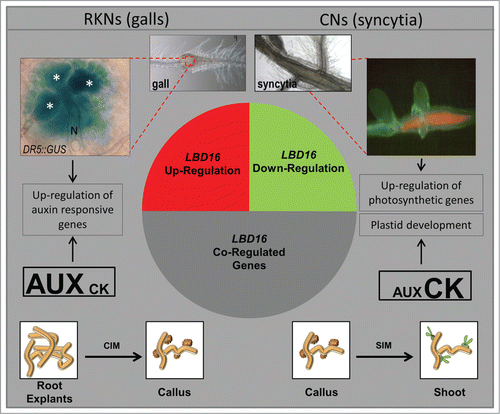
In conclusion, in silico data confirm LBD16 and its co-regulated genes as a hallmark of the RKN feeding sites compared to syncytia. Those genes are integrated in signaling cascades mediated by auxins that are shared also by LR and callus development. In contrast, LBD16 and its positively co-regulated genes were repressed in syncytia. However, cytokinins induced genes are enriched in syncytia transcriptome, showing also clear similarities with the transcriptome of shoot regeneration from callus that is modulated preferentially by cytokinins. Those findings establish differences in the regulatory networks leading to both feeding sites formation probably modulated by the auxin/cytokinin balance.
Disclosure of Potential Conflicts of Interest
No potential conflicts of interest were disclosed.
Table_S2.docx
Download MS Word (19.4 KB)Table_S1.docx
Download MS Word (15.5 KB)Figures_S1_and_S2.docx
Download MS Word (669.1 KB)Supplemental Material
Supplemental data for this article can be accessed on thepublisher's website.
Funding
This work was supported by the Spanish Government (AGL2010-17388 and AGL2013-48787, to C. Escobar, CSD2007-057 to C. Fenoll, PLANT-KBBE PLANT-043, PCIN-2013-053 to C. Fenoll and C. Escobar). Javier Cabrera was supported by a fellowship from the Ministry of Education, Spain. We acknowledge Prof. Dr. Florian M. W. Grundler and Dr. Shahid M. Siddique from Bonn University for kindly provide us with the syncytia fluorescence microscopy picture from .
References
- Huang CS, Maggenti AR. Mitotic aberrations and nuclear changes of developing giant cells in Vicia faba caused by root knot nematode, Meloidogyne javanica. Phytopathology 1969; 59:447-55.
- Caillaud MC, Lecomte P, Jammes F, Quentin M, Pagnotta S, Andrio E, de Almeida Engler J, Marfaing N, Gounon P, Abad P, et al. MAP65–3 microtubule-associated protein is essential for nematode-induced giant cell ontogenesis in Arabidopsis. Plant Cell 2008; 20:423-37; PMID:18263774
- Kyndt T, Vieira P, Gheysen G, De Almeida-Engler J. Nematode feeding sites: unique organs in plant roots. Planta 2013; 238:807-18; PMID:23824525; http://dx.doi.org/10.1007/s00425-013-1923-z
- Cabrera J, Barcala M, Fenoll C, Escobar C. Transcriptomic signatures of transfer cells in early developing nematode feeding cells of Arabidopsis focused on auxin and ethylene signaling. Front Plant Sci 2014; 5:107; PMID:24715895; http://dx.doi.org/10.3389/fpls.2014.00107
- Sobczak M, Golinowski W. Structure of cyst nematode feeding sites. In: Berg RH, Taylor CG, eds. Cell Biology of Plant Nematode Parasitism. Heidelberg, Germany: Springer Verlag, 2009; pp 153-88.
- Nicol JM, Turner SJ, Coyne DL, Nijs L. Current nematode threats to world agriculture. In: Jones J, Gheysen G, Fenoll C, eds. Genomics and Molecular Genetics of Plant–Nematode Interactions. Dordrecht: Springer Verlag, 2011; pp 21-45.
- Cabrera J, Díaz-Manzano F, Sánchez M, Rosso MN, Melillo T, Goh T, Fukaki H, Cabello S, Hofmann J, Fenoll C, et al. A role for LATERAL ORGAN BOUNDARIES-DOMAIN 16 during the interaction Arabidopsis-Meloidogyne spp. provides a molecular link between lateral root and root-knot nematode feeding site development. New Phytol 2014; 203:632-45; PMID:24803293; http://dx.doi.org/10.1111/nph.12826
- Goh T, Joi S, Mimura T, Fukaki H. The establishment of asymmetry in Arabidopsis lateral root founder cells is regulated by LBD16/ASL18 and related LBD/ASL proteins. Development 2012; 139:883-93; PMID:22278921; http://dx.doi.org/10.1242/dev.071928
- Laplaze L, Parizot B, Baker A, Ricaud L, Martinière A, Auguy F, Franche C, Nussaume L, Bogusz D, Haseloff J. GAL4-GFP enhancer trap lines for genetic manipulation of lateral root development in Arabidopsis thaliana. J Exp Bot 2005; 56(419):2433-42; PMID:16043452; http://dx.doi.org/10.1093/jxb/eri236
- Cabrera J, Bustos R, Favery B, Fenoll C, Escobar C. NEMATIC: a simple and versatile tool for the in silico analysis of plant-nematode interactions. Mol. Plant Pathol 2014; 15:627-36; PMID:24330140; http://dx.doi.org/10.1111/mpp.12114
- Jammes F, Lecomte P, de Almeida-Engler J, Bitton F, Martin-Magniette ML, Renou JP, Abad P, Favery B. Genome-wide expression profiling of the host response to root-knot nematode infection in Arabidopsis. Plant J 2005; 44:447-58; PMID:16236154; http://dx.doi.org/10.1111/j.1365-313X.2005.02532.x
- Szakasits D, Heinen P, Wieczorek K, Hofmann J, Wagner F, Kreil DP, Sykacek P, Grundler FM, Bohlmann H. The transcriptome of syncytia induced by the cyst nematode Heterodera schachtii in Arabidopsis roots. Plant J 2009; 57:771-84; PMID:18980640
- Nemhauser JL, Hong F, Chory J. Different plant hormones regulate similar processes through largely non-overlapping transcriptional responses. Cell 2006; 126:467-75; PMID:16901781; http://dx.doi.org/10.1016/j.cell.2006.05.050
- Quentin M, Hewezi T, Damiani I, Abad P, Baum T, Favery B. How pathogens affect root structure. In: Crespi M ed. Root Genomics and Soil Interactions. Blackwell Publishing Ltd., Oxford, UK, 2012.
- Thimm O, Blasing O, Gibon Y, Nagel A, Meyer S, Kruger P, Selbig J, Müller LA, Rhee SY, Stitt, M. MAPMAN: a user-driven tool to display genomics data sets onto diagrams of metabolic pathways and other biological processes. Plant J 2004; 37:914-39; PMID:14996223; http://dx.doi.org/10.1111/j.1365-313X.2004.02016.x
- Barcala M, Garcia A, Cabrera J, Casson S, Lindsey K, Favery B, García-Casado G, Solano R, Fenoll C, Escobar, C. Early transcriptomic events in microdissected Arabidopsis nematode-induced giant cells. Plant J 2010; 61:698-712; PMID:20003167
- Hruz T, Laule O, Szabo G, Wessendorp F, Bleuler S, Oertle L, Widmayer P, Gruissem W, Zimmermann P. Genevestigator v3: a reference expression database for the meta-analysis of transcriptomes. Adv Bioinformatics 2008; 2008:420747; PMID:19956698; http://dx.doi.org/10.1155/2008/420747
- Ping C, Lall S, Nettleton D, Howell SH. Gene expression programs during shoot, root, and callus development in Arabidopsis tissue culture. Plant Physiol 2006; 141:620-37; PMID:16648215; http://dx.doi.org/10.1104/pp.106.081240
- Fan M, Xu C, Xu K, Hu Y. LATERAL ORGAN BOUNDARIES DOMAIN transcription factors direct callus formation in Arabidopsis regeneration. Cell Res 2012; 22:1169-80; PMID:22508267; http://dx.doi.org/10.1038/cr.2012.63
- Skoog F, Miller CO. Chemical regulation of growth and organ formation in plant tissue cultured in vitro. Symp Soc Exp Biol 1957; 11:118-31; PMID:13486467
- Chory J, Reinecke D, Sim S, Washburn T, Brenner M. A role for cytokinins in de-etiolation in Arabidopsis (det mutants have an altered response to cytokinins). Plant Physiol 1994; 104:339-47; PMID:12232085
- Ji H, Gheysen G, Denil S, Lindsey K, Topping JF, Nahar K, Haegeman A, De Vos WH, Trooskens G, Van Criekinge W, De Meyer T, Kyndt T. Transcriptional analysis through RNA sequencing of giant cells induced by Meloidogyne graminicola in rice roots. J Exp Bot. 2013; 64(12):3885-98. doi: 10.1093/jxb/ert219