Abstract
Nitric oxide (NO) and glutathione (GSH) are 2 vital components of the antioxidant system that play diverse roles in plant responses to abiotic stresses. Recently, we have reported that exogenous supply of both these molecules reduced copper (Cu) toxicity in rice seedlings. Individual as well as co-treatment of sodium nitroprusside (SNP: a NO donor) and GSH with Cu significantly mitigated the adverse effects of Cu, evident in the reduced level of oxidative markers such as H2O2, superoxide (O2·−), malondialdehyde (MDA), and proline (Pro). GSH content and most of the antioxidative and glyoxalase enzymes were up-regulated upon Cu stress, indicating their responses were co-related with the level of stress. Our results indicated that direct ROS scavenging, reduced Cu uptake, and the balanced antioxidative and glyoxalase systems, at least in part, successfully executed NO- and GSH-mediated alleviation of Cu toxicity in rice seedlings. In addition, the combined effect of adding SNP and GSH together was more efficient than the effect of adding them individually. Here, we are speculating that 1) GSH and Pro could be used as potential markers for copper stress, and 2) adding SNP and GSH might produce S-nitrosoglutathione (GSNO) which could be a source of bioactive NO and may affect many regulatory processes involved in Cu-stress tolerance. We further note that the combined effect of adding SNP and GSH was pronounced in inhibiting the uptake and translocation of Cu in rice seedlings.
In this era of industrial revolution, rapid urbanization, and modern agricultural practices, heavy metal pollution has become a significant global concern due to its deleterious effects on the health of animals and plants.Citation1 Among the heavy metals, copper (Cu) is considered essential in trace amounts and regulates many physiological and biochemical processes involved in plant growth, development, and protective mechanisms.Citation2 Soil rarely produces excessive levels of Cu on its own. However, anthropogenic activities such as repeated use of Cu-containing fungicides and slurries elevate Cu to toxic levels in soils. Once Cu contamination occurs, it can persist for many years because of its low solubility and mobility.Citation2,3 Plants growing in Cu-enriched soil exhibit phytotoxic symptoms manifested principally as stunted growth, chlorosis, necrosis, and leaf rolling.Citation3 Since Cu acts as a cofactor of numerous proteins and enzymes involved in major cellular processes, including photosynthesis, respiration, nitrogen assimilation, and senescence, a little excess Cu can inhibit all these processes and thereby severely compromise plant growth and development.Citation4 Being a redox active metal, Cu also potentiates oxidative stress through forming deadly free radicals. Free radicals and other reactive oxygen species (ROS) are highly reactive and toxic and can damage thiols, other biological molecules, and lipid membranes.Citation5 In our study, 100 μM Cu exposure resulted in significant accumulation of ROS such as O2•– and H2O2, which closely correlates with the level of malondialdehyde (MDA, a lipid peroxidation product). However, applying SNP and GSH dramatically reduced the level of lipid peroxidation as indicated by a decreased level of ROS and MDA.Citation6 In general, ROS and MDA are used as markers of oxidative stress in plants under heavy metal stress, as confirmed in our study. In addition, we also noted a gradual increase in GSH and Pro in response to an increasing level of Cu stress in the rice seedlings ().
Figure 1. (A) Effect of copper on the levels of GSH and Pro after 72 h of Cu treatment. Bar charts indicate GSH and Pro contents in nmol/g FW whereas line charts indicate ratio of GSH and Pro to protein (nmol/mg of protein). Different letters indicate significant differences between treatments at P < 0.05, according to DMRT. (B) Phenotypical aspects of copper toxicity in hydroponically grown rice seedlings. Fourteen-day-old rice seedlings were treated with 0, 100, 200, 300, 400 μM of CuSO4. Photograph was taken after 72 h of Cu treatment.
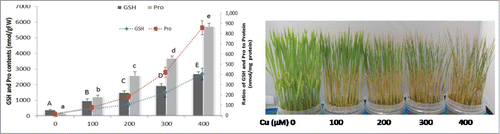
Figure 2. Possible mechanism of exogenous GSNO-mediated Cu tolerance in rice seedlings. Exogenous application of GSH and SNP can produce GSNO in presence of O2. GSNO can inhibit metal uptake by forming complexes with Cu. GSNO can be uptaken by the root and transported to the upper parts through phloem. GSNO can be decomposed to GSSG and NH3 by the GSNOR using NADPH. Alternatively, GSNO can be broken down to NO and GSSG in presence of reductants such as Cu+, AsA and GSH. GSSG can be converted to GSH by GR. GSH can then play important role in stress tolerance through redox regulation, ROS and MG detoxifications, and metal chelation. On the other hand, GSNO can transfer the NO to Cys, Tyr or metals of proteins, a process known as post translational modification, which can modify the function of a broad spectrum of proteins leading to stress tolerance.
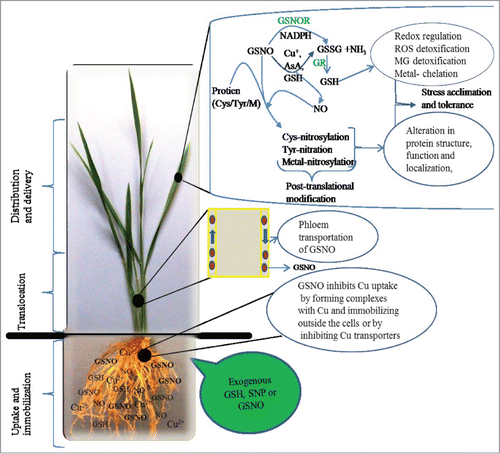
GSH is a low molecular weight, water soluble antioxidant present in all compartments of plant cells and plays vital roles essentially against heavy metal-induced toxicity.Citation7,8 GSH is also involved in sulfur metabolism, xenobiotics detoxification, heavy metal chelation, ROS and methylglyoxal (MG) elimination, and in the regulation of the redox balance.Citation7,8 It is also strongly involved in fine-tuning of redox signaling during environmental stresses and is therefore regarded as a stress marker in plant ecophysiological studies.Citation9 It is well established that not only GSH concentration, but also the GSH/GSSG ratio, is important in maintaining cell redox status.Citation8 Any imbalance in the cellular GSH level, both deficiency and excess, may adversely influence cellular metabolism.Citation7 In addition, the GSH/GSSG redox system may have a regulatory role in modulating signaling cascades during the post-transcriptional and post-translational level due to their interaction with other redox systems such as glutaredoxin, peroxiredoxin, thioredoxin and mitogen activated protein kinases.Citation8 The cellular level of GSH and the GSH/GSSG redox status are differentially regulated in response to a variety of metal stresses.Citation8,10 Many reports state that cellular GSH content increased or decreased or remained constant after metal exposure and is related to the difference in species/genotypes or the experimental conditions.Citation11-15
In our study, reduced and oxidized GSH increased markedly in Cu-stressed seedlings while the GSH/GSSG ratio decreased.Citation6 Cuypers et al.Citation13 also reported similar findings in which the levels of GSH, GSSG, and the GSSG/GSH ratio were always higher in Cu-treated plants than the control. A progressive time-dependent increase in GSH content was reported in Scenedesmus bijugatus exposed to an increasing level of Cu.Citation14 However, the elevation of GSH does not always correlate with enhanced tolerance to heavy metals. Perhaps GSH alone is not sufficient to support the complex mechanism of resistance to heavy metal-induced stress.Citation7 Wójcik and TukiendorfCitation15 also identified that a decrease in GSH content led to a decrease in Cd tolerance but an increase in GSH content did not enhance Cd tolerance, and it even showed a toxic effect in Arabidopsis.
Disturbance of the plant-water relation is a general consequence of heavy metal toxicity. A decrease in the water content of leaves and roots is associated with ion toxicity, stomatal malfunctioning, and decreased CO2 assimilation and photosynthesis which ultimately results in serious metabolic perturbations reducing plant growth and development.Citation1,16,17 To adjust physiological water shortage, plants accumulate an array of organic compounds, known as osmolytes. Pro, besides acting as an osmolyte, participates in metal chelation, ROS detoxification, and signal transduction.Citation18 Many plants have been shown to accumulate Pro in large quantities when exposed to heavy metal stresses.Citation11,18,19
Metabolic acclimation via the accumulation of Pro often correlates with improved tolerance to heavy metal stresses. However, despite its beneficial effects, Pro imparts toxic effects if over-accumulated or applied in excessive concentrations.Citation19 Pro can mimic the toxic effects of Cd in rice seedlings when accumulated in excess.Citation20 Other studies also claim that the level of accumulated Pro reflects the intensity of stressed symptoms when plants are subjected to different types of abiotic stresses.Citation11,21,22 In our study, Pro increased markedly upon Cu stress, which seems to be compensation for water loss generated by Cu in the leaves.Citation6 However, over-accumulated Pro did not correlate with protection against Cu stress but indicated a consequence of metabolic perturbation. Similarly, Pro accumulation in salt-stressed Cathranthus roseus negatively correlates to RWC, biomass, and K accumulation.Citation23
shows that the visual toxicity symptoms increased with an increasing concentration of Cu in the nutrient solution. We also observed a concomitant increase in the levels of GSH and Pro despite the severe conditions of the seedlings due to an increasing concentration of Cu (). Our previous findingCitation6,11 and current data (), therefore, show that the levels of GSH and Pro accumulation reflect stressed symptoms rather than stress resistance. Therefore, here we suggest that both GSH and Pro could be considered as potential biomarkers against Cu stress in rice seedlings. This information will be useful to understand Cu tolerance and to establish biochemical markers for rice plants growing in Cu-contaminated areas.
In contrast, adding SNP, GSH, and SNP+GSH to Cu-treated rice seedlings effectively counteracted the Cu-induced increase in GSH and Pro content, indicating partial relief in these plants from Cu stress.Citation6 Similar to our findings, exogenous NO and GSH were also found to counteract a Cd-induced increase in GSH content in rice cultivars.Citation24,25 Reduced Pro content by SNP has also been reported in canola plants under Ni stress.Citation26 GSH- and SNP+GSH-supplemented seedlings showed greater reduction in Pro content than with SNP alone, indicating these seedlings suffered from less stress. NO- and GSH-mediated relief of Cu stress can partially be supported by 1) the antioxidant nature of both protectants scavenging ROS directly, and 2) forming NO-Cu or GSH-Cu conjugates in the nutrient medium and thus inhibit Cu uptake and accumulation (). The above mechanisms allowed the seedlings to suffer less oxidative and Cu loads, and hence, eventually supports the reason behind less accumulation of GSH and Pro.
Considering the ROS level, induction level of enzyme activities, Cu accumulation, and visual toxicity, the degree of effectiveness in alleviating Cu toxicity can be presented as SNP+GSH>GSH>SNP.Citation6 Adding SNP+GSH was based on the hypothesis that SNP-released NO binds readily with GSH to form S-nitrosoglutathione (GSNO), which would serve as a NO reservoir and a long-distance carrier of NO. Although we did not quantify the formation of GSNO in our study, the better response of the rice seedlings treated with SNP+GSH led us to assume that the combined effect of adding SNP and GSH might provide a distinct mechanism in combating Cu stress in rice seedlings. We speculate that the combined application of GSH and SNP produces GSNO, which might form a strong complex with Cu, and then immobilize or sequestrate it into vacuoles (). Formation of complexes between metal ions and thiol compounds is considered a specific defense strategy against heavy metal toxicity. Koen et al.Citation27 reported that exogenous GSNO significantly affected the concentration of Cu in Arabidopsis.
Numerous research findings have shown that NO plays many crucial roles in plant tolerance to abiotic stresses including heavy metals.Citation28-30 However, NO can have both protective and cytotoxic effects depending on its concentration and location in the plant cells.Citation30 Since NO is short-lived and cytotoxic at high concentrations, GSNO could be a proper candidate for bioavailability of NO under physiological and stress conditions.Citation31 In our study, we observed that SNP+GSH-treated seedlings were more vigorous than the SNP only-treated seedlings, which suggests that adding SNP with GSH minimized the direct effect of NO.Citation6 Mounting evidence indicates that NO is a signaling molecule found to be involved in diverse cellular functions in plants.Citation29,30 NO regulates different processes by inducing gene expression, mobilizing second messengers, and modulating lipid signaling and post-translational modification of numerous proteins.Citation30,32 GSNO plays vital roles in NO-mediated post translational modification of proteins.Citation31 GSNO-mediated S-nitrosylation of thiol, nitration of tyrosine, glutathiolation, and binding to metal centers modulates the functions of various ion channels, G-proteins, respiratory proteins, transcription factors, and multiple enzymes and thereby appears to have a significant physiological and biochemical importance in NO metabolism in plants.Citation32-34 Antioxidant enzymes such as APX, GR, DHAR, CAT, and GAPDH are subjected to S-nitrosylation during environmental stresses and indicate an important role of GSNO in ROS metabolism.Citation32 So far the presence of GSNO and its modulation have been reported in several plant species under biotic and abiotic stress conditions.Citation33-35
However, very few studies are directed toward the effects of exogenous GSNO on its endogenous level and modification of potential proteins under stress conditions. In addition, GSNO reductase (GSNOR), a major enzyme in GSNO metabolism, can control the intercellular level of GSNO and consequently the effects of NO in cells. Recent studies show that GSNOR activity and the levels of NO, GSH, and GSNO are correlated in response to environmental stresses.Citation35,36 Therefore, GSNOR could be an important molecular target as it indirectly regulates S-nitrosylation-dependent signaling as well as the cellular redox state through modulating the level of NO and GSH ().
NO and GSH modulate each other's level and GSNO acts as a biological reservoir of both these molecules. Any modification in the cellular level of GSNO might have a profound effect on cellular metabolism in plants. Both these molecules are involved in the mitigation of abiotic stresses including metal toxicity through diverse mechanisms. Therefore, the interaction of GSH with NO could be an important perspective in plant protective mechanisms since both molecules participate in the redox state and signaling processes during responses to various developmental, and biotic and abiotic stresses.
Disclosure of Potential Conflicts of Interest
No potential conflicts of interest were disclosed.
References
- Nagajyoti PC, Lee KD, Sreekanth TVM. Heavy metals, occurrence and toxicity for plants: a review. Environ Chem Lett 2010; 8:199-216; http://dx.doi.org/10.1007/s10311-010-0297-8
- Yruela I. Copper in plants: acquisition, transport and interactions. Funct Plant Biol 2009; 36:409-30; http://dx.doi.org/10.1071/FP08288
- Burkhead JL, Reynolds KAG, Abdel-Ghany SE, Cohu CM, Pilon M. Copper homeostasis. New Phytol 2009;182:799-816; PMID:19402880; http://dx.doi.org/10.1111/j.1469-8137.2009.02846.x
- Fernandes JC, Henriques FS. Biochemical, physiological and structural effects of excess copper in plants. Bot Rev 1991; 57:247-73; http://dx.doi.org/10.1007/BF02858564
- Mittler R. Oxidative stress, antioxidants and stress tolerance. Trends Plant Sci 2002; 7:405-10; PMID:12234732; http://dx.doi.org/10.1016/S1360-1385(02)02312-9
- Mostofa MG, Seraj ZI, Fujita M. Exogenous sodium nitroprusside and glutathione alleviate copper toxicity by reducing copper uptake and oxidative damage in rice (Oryza sativa L.) seedlings. Protoplasma 2014; http://dx.doi.org/10.1007/s00709-014-0639-7
- Noctor G, Mhamdi A, Chaouch S, Han Y, Neukermans J, Queval G, Foyer CH. Glutathione in plants: an integrated overview. Plant Cell Env 2012; 35:454-8; http://dx.doi.org/10.1111/j.1365-3040.2011.02400.x
- Anjum NA, Ahmad I, Mohmood I, Pacheco M, Duarte AC, Pereira E, Umar S, Ahmad A, Khan NA, Iqbal M, et al. Modulation of glutathione and its related enzymes in plants' responses to toxic metals and metalloids - a review. Environ Exp Bot 2012; 75:307-24.
- Tausz M, Sˇircelj H, Grill D. The GSH system as a stress marker in plant ecophysiology: is a stress-response concept valid?. J Exp Bot 2004; 55:1955-62; PMID:15234995; http://dx.doi.org/10.1093/jxb/erh194
- Gill SS, Tuteja N. Cadmium stress tolerance in crop plants: probing the role of sulfur. Plant Signal Behav 2011; 6:215-22; PMID:21330784; http://dx.doi.org/10.4161/psb.6.2.14880
- Mostofa MG, Fujita M. Salicylic acid alleviates copper toxicity in rice (Oryza sativa L.) seedlings by up-regulating antioxidative and glyoxalase systems. Ecotoxicology 2013; 22:959-73; PMID:23579392; http://dx.doi.org/10.1007/s10646-013-1073-x
- Chen F, Wang F, Wu FB, Mao WH, Zhang GP, Zhou M. Modulation of exogenous GSH on antioxidant defense system against Cd stress in two barley genotypes differing in Cd tolerance. Plant Physiol Biochem 2010; 48:663-72; PMID:20605723; http://dx.doi.org/10.1016/j.plaphy.2010.05.001
- Cuypers A, Vangronsveld J, Clijsters H. Biphasic effect of copper on the ascorbate–glutathione pathway in primary leaves of Phaseolus vulgaris seedlings during the early stages of metal assimilation. Physiol Plant 2000;110:512-7; http://dx.doi.org/10.1111/j.1399-3054.2000.1100413.x
- Nagalakshmi N, Prasad MNV. Responses of GSH cycle enzymes and GSH metabolism to Cu stress in Scenedesmus bijugatus. Plant Sci. 2001; 160:291-9; PMID:11164601; http://dx.doi.org/10.1016/S0168-9452(00)00392-7
- Wójcik M, Tukiendorf A. Glutathione in adaptation of Arabidopsis thaliana to cadmium stress. Biol Plant 2011; 55:125-32; http://dx.doi.org/10.1007/s10535-011-0017-7
- Kastori R, Petrovic M, Petrovic N. Effect of excess lead, cadmium, copper and zinc on water relations in sunflower. J Plant Nut 1992; 15:2427-39; http://dx.doi.org/10.1080/01904169209364485
- Vernay P, Gauthier-Moussard C, Hitmi A. Interaction of bioaccumulation of heavy metal chromium with water relation, mineral nutrition and photosynthesis in developed leaves of Lolium perenne L. Chemosphere 2007; 68:1563-75; PMID:17434568; http://dx.doi.org/10.1016/j.chemosphere.2007.02.052
- Hayat S, Hayat Q, Alyemeni MN, Wani AS, Pichtel J, Ahmad A. Role of proline under changing environments. Plant Signal Behav 2012; 7:1456-66; PMID:22951402; http://dx.doi.org/10.4161/psb.21949
- Backor M, Fahselt D, Wu CT. Free proline content is positively correlated with copper tolerance of the lichen photobiont Trebouxia erici (Chlorophyta). Plant Sci 2004; 167:151-157; http://dx.doi.org/10.1016/j.plantsci.2004.03.012
- Chen SL, Kao CH. Cadmium induced changes in proline level and peroxidase activity in roots of rice seedlings. Plant Growth Regul 1995; 17:67-71.
- Mostofa MG, Yoshida N, Fujita M. Spermidine pretreatment enhances heat tolerance in rice seedlings through modulating antioxidative and glyoxalase systems. Plant Growth Regul 2013; 73:31-44; http://dx.doi.org/10.1007/s10725-013-9865-9
- Metwally A, Finkemeier I, Georgi M, Dietz KJ. Salicylic acid alleviates the cadmium toxicity in barley seedlings. Plant Physiol 2003; 132:272-81; PMID:12746532; http://dx.doi.org/10.1104/pp.102.018457
- Chang B, Yang L, Cong W, Zu Y, Tang Z. The improved resistance to high salinity induced by trehalose is associated with ionic regulation and osmotic adjustment in Catharanthus roseus. Plant Physiol Biochem 2014; 14:140-8; http://dx.doi.org/10.1016/j.plaphy.2014.02.001
- Panda P, Nath S, Chanu TT, Sharma GD, Panda SK. Cadmium stress-induced oxidative stress and role of nitric oxide in rice (Oryza sativa L.). Acta Physiol Plant 2011; 33:1737-47; http://dx.doi.org/10.1007/s11738-011-0710-3
- Cai Y, Cao F, Cheng W, Zhang G, Wu F. Modulation of exogenous glutathione in phytochelatins and photosynthetic performance against Cd stress in the two rice genotypes differing in Cd tolerance. Biol Trace Elem Res 2010; 143:1159-73; PMID:21191821; http://dx.doi.org/10.1007/s12011-010-8929-1
- Kazemi N, Khavari-Nejad RA, Fahimi H, Saadatmand S, Nejad-Sattari T. Effects of exogenous salicylic acid and nitric oxide on lipid peroxidation and antioxidant enzyme activities in leaves of Brassica napus L. under nickel stress. Sci. Hortic 2010; 126:402-7; http://dx.doi.org/10.1016/j.scienta.2010.07.037
- Koen E, Szymańska K, Klinguer A, Dobrowolska G, Besson-Bard A, Wendehenne D. Nitric oxide and glutathione impact the expression of iron uptake- and iron transport-related genes as well as the content of metals in A. thaliana plants grown under iron deficiency. Plant Signal Behav 2012; 7:1246-50; PMID:22902693; http://dx.doi.org/10.4161/psb.21548
- Xu J, Yin H, Li Y, Liu X. Nitric oxide is associated with long-term zinc tolerance in Solanum nigrum. Plant Physiol 2010; 154:1319-34; PMID:20855519; http://dx.doi.org/10.1104/pp.110.162982
- Xiong J, Fu G, Tao L, Zhu C. Roles of nitric oxide in alleviating heavy metal toxicity in plants. Arch Biochem Biophys 2010; 497:13-20; PMID:20193657; http://dx.doi.org/10.1016/j.abb.2010.02.014
- Siddiqui MH, Al‑Whaibi MH, Basalah MO. Role of nitric oxide in tolerance of plants to abiotic stress. Protoplasma 2011; 248:447-55; PMID:20827494; http://dx.doi.org/10.1007/s00709-010-0206-9
- Corpas FJ, Alché JD, Barroso JB. Current overview of S-nitrosoglutathione (GSNO) in higher plants. Front Plant Sci. 2013; 4:1-3; PMID:23346092
- Romero-Puertas MC, Rodríguez-Serrano M, Sandalio LM. Protein S-nitrosylation in plants under abiotic stress: an overview. Front Plant Sci 2013; 4:1-6; PMID:23346092; http://dx.doi.org/10.3389/fpls.2013.00373
- Espunya MC, De Michele R, Gómez-Cadenas A, Martínez MC. S-Nitrosoglutathione is a component of wound- and salicylic acid-induced systemic responses in Arabidopsis thaliana. J Exp Bot 2012; 63:3219-27; PMID:22371078; http://dx.doi.org/10.1093/jxb/ers043
- Barroso JB, Corpas FJ, Carreras A, Rodríguez-Serrano M, Esteban FJ, Fernández-Ocaña A, Chaki M, Romero-Puertas MC, Valderrama R, Sandalio LM, et al. Localization of S-nitrosoglutathione and expression of S-nitrosoglutathione reductase in pea plants under cadmium stress. J Exp Bot 2006; 57:1785-93; PMID:16595575; http://dx.doi.org/10.1093/jxb/erj175
- Chaki M, Valderrama R, Fernández-Ocaña AM, Carreras A, Gómez-Rodríguez MV, Pedrajas JR, Begara-Morales JC, Sánchez-Calvo B, Luque F, Leterrier M, et al. Mechanical wounding induces a nitrosative stress by down-regulation of GSNO reductase and an increase in S-nitrosothiols in sunflower (Helianthus annuus) seedlings. J Exp Bot 2011; 62:1803-13; PMID:21172815; http://dx.doi.org/10.1093/jxb/erq358
- Leterrier M, Chaki M, Airaki M, Valderrama R, Palma JM, Barroso JB, Corpas FJ. Function of S-nitrosoglutathione reductase (GSNOR) in plant development and under biotic/abiotic stress. Plant Signal Behav 2011; 6:789-93; PMID:21543898; http://dx.doi.org/10.4161/psb.6.6.15161