Abstract
Magnesium (Mg) is one of the most important nutrients, involves mainly in plant growth and development. However, the signaling pathways response to magnesium stresses (MgSs) is known little, but several studies lately may improve the research development. Several phytohormones such as abscisic acid (ABA), ethylene, auxins, and their factors were found responding to MgSs, and there may be some signal pathways linking these hormones and downstream reactions together, e.g., carbon fixation and transfer, starch and sugar metabolism, ion uptaking and reactive oxygen species (ROS) increasing. Consequently, Arabidopsis morphogenesis is remodeled. In this review, we mainly discussed recent literatures involving in plant response to MgSs (Mg deficiency (MgD) and Mg toxicity (MgT)), including plant morphogenesis remodeling, magnesium transporters and signaling transductions. Moreover, the future study challenges related to the responding signalings to MgSs in plants are also presented. Regardless, the iceberg of signal transduction of MgSs in plants is appeared.
Magnesium (Mg), a most abundant free divalent cation in a cell, is one of the core macronutrients in organism growth and development, including human.Citation1-3 In particular, Mg plays a central role in plant chlorophyll biosynthesis and carbon fixation as a cofactor of a series of enzymes involved in carbon metabolism.Citation4 However, the physiological and molecular functions of Mg in plants have been neglected for decades due to its abundance in soil (the 8th most abundant element on earth), high solubility in water for plant absorption, and complex functionalities.Citation3 Mg homeostasis in plant cells has been elaborated with the discovery of magnesium transporters (MaTRs).Citation5-7 Surprisingly, microarray studies revealed that the transcriptional levels of these plant MgTRs changed little under MgSs (deficiency or excess/toxicity), whereas, numerous other transporters were activated by MgSs.Citation8-10 However, the signal transduction responding to MgSs is largely unknown, although some reports indicated that expression levels of many genes related to phytohormone biosynthesis and signalings are significantly changed by MgSs.Citation8-11 Our previous studies discovered that at least 2 hormones, namely abscisic acid (ABA) and gibberellins (GA), and their signaling factors, DELLA and ABI1, are responding to magnesium toxicity (MgT).Citation1 These results suggest that the discovery of signal transduction for MgT in plants could be a gateway to explore the regulatory mechanisms of responses to MgSs. The aim of this review is to summarize the current knowledge of signal transduction responding to MgSs in plants involving morphogenesis remodeling, Mg transporters (MgTRs), and signal transduction. A model for plants responding to MgSs was also proposed.
Morphogenesis Remodeling of Plants by Magnesium Stresses
Mg concentrations in soil solutions are considered between 125 μM and 8.5 mM,Citation12 however, plants encounter MgSs commonly in nature. Mg2+ deficiency occurs generally in plants growing in the tropical regions due to their acidic soils, high temperature, high rainfall,Citation13 and some aluminum toxic fields.Citation14 Conditions with dry soil and high levels of competing elements, such as potassium and calcium, also result in Mg deficiency.Citation13 Mg toxicity in plants usually happens in serpentine soils formed by the weathering of ultramafic rocks,Citation15 which widely distribute throughout the globe across all climatic zones.Citation16 Therefore, Mg levels below 125 μM in soil solutions may proved to be deficient (namely Mg deficiency, MgD) for plant growth and development, whereas, its levels ≥8 .5 mM in soil solutions might be considered excess or toxic for plant growth and development. For Arabidopsis, as shown in , 1.5 mM Mg is considered as the optimum concentration for its growth and less or more than this level could obstruct the growth. More recently, we found that the phenotypes of Arabidopsis plants changed significantly above 20 mM Mg in solid medium.Citation1 Thus, excess Mg is defined as Mg toxicity (MgT) and MgSs include both MgT and MgD.Citation1,4,8
Figure 1. Phenotypes of 14 d old Arabidopsis thaliana (Landsberg erecta) seedlings. (A) Iodine staining of starch of leaves. (B) Characteristics of seedlings and root hair.
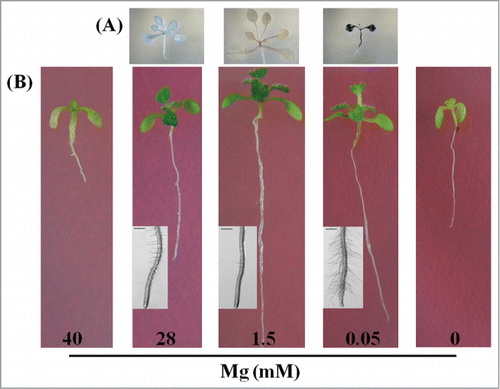
MgSs markedly inhibit plant growth, resulting in shorter roots, smaller shoots, and necrotic spots.Citation1,4,9,10,Citation15,17,Citation18 Hermans et al.Citation4,8 found that MgD reduced the growth of young leaves more than the roots, but our results indicated that both root and leaf were severely inhibited by MgD (). The severity of MgD effects could depend on plants' development stages. For instance, young seedlings (2 weeks ageCitation1) required more nutrients compared with 5 weeksCitation8 plants. Niu et al.Citation19 reported that root hairs of Arabidopsis decreased progressively with increasing Mg supply, we had the same results when Mg level was too low (0.05 mM, ), but MgT (Mg2+: 28 mM) improved root hair development.Citation1,20 It may be due to different culture systems (liquid mediumCitation19 and solid mediumCitation1), developmental phases (olderCitation19 and youngerCitation1) and ecotypes (Col-019 and LerCitation1), 10 mM MgCitation2+ in our experiments had no significant changes in phenotypes.Citation1
In addition to carbon fixation in photosynthesis, magnesium also acts as activator or cofactor of enzymes in carbohydrate metabolism, e.g., fructose-1,6-bisphosphatase (FBPase), glutamate synthetase, UDP-D-, ADP-D-, and GDPD-glucose pyrophosphorylase, and UDP-D-glucuronic acid pyrophosphorylase.Citation4 Therefore, loss and excess of Mg might lead to different phenotypes, for instance, MgD resulted in over accumulation, whereas, MgT induced excessive degradation of starch and sugar in leaves (). The accumulation of starch and sugar in leaves might be due to blockage in carbohydrates loading in phloem under MgD, and consequently impaired sucrose transport from shoot to root, especially in early MgD stage. Therefore, photosynthetic activity is disrupted that could lead to produce reactive oxygen species (ROS) which substantially degraded chloroplast constituents, such as chlorophyll and membrane lipids.Citation4,21 On the other hand, under MgT,Citation1 the transcriptional levels of starch biosynthesis enzymes such as SS1, SS2, GBSS1 and APL1 were severely inhibited, whereas the transcriptional levels of starch degradation enzymes such as AtAMY1 and BAM1, were highly improved. Accordingly, the leaves with MgT treatment turned into translucence with iodine dye () and starch contents were substantially decreased.Citation1
Growth of plants on Mg deficient medium could cause decrease in shoot and root Mg concentrations,Citation8,17,20 but little is known about the uptake of other ions, for instance, iron, calcium, and potassium in MgD medium. However, we found that the contents of calcium and zinc were significantly decreased, whereas, the contents of manganese and iron were slightly increased under MgT in Arabidopsis plants.Citation1 These findings were in lined with the results obtained from studies on serpentine soils.Citation15 MgT could also help plants to absorb excessive Mg,Citation1,17 might be due to up-regulation of transcriptional levels of some Mg transporters.Citation1 Those results indicated that different response mechanisms such as starch biosynthesis and degradation, and ion uptake might be involved in the remodeling of plant morphogenesis, responding to MgD and MgT, respectively. Our former studies suggested that these processes might be regulated by different signal transduction pathways, for instance, the mutant plants (abi1-1 (a ABA- insensitive mutant) and DELLA-Q plants (a constitutive elevated GA response mutant quadruple-DELLA (gai-t6 rga-t2 rgl1–1 rgl2–1)) grew well with MgT treatmentsCitation1 but had similar awful phenotypes as wild plants under MgD conditions (, photos of abi1–1 and DELLA-Q plants are not offered here).
Magnesium Transporters in Plants Responding to Magnesium Stresses
AtMHX, a Mg2+/H+ exchanger, is the first cloned MgTR in plantsCitation5, expressed in the vascular region, particularly in the phloem.Citation11 Interestingly, its cis-regulons are within the leader intronCitation22 and 5′untranslated region. The later one includes an upstream open reading frame (uORF) to the initiation codon of ORF. uORF significantly inhibits AtMHX expression by degradation of AtMHX mRNA,Citation11,23 and these processes may be regulated by ABA and auxins.Citation11 The novel literatures presented that overexpression of AtMHX increased expression and activity of the vacuolar H+-ATPase, moreover, transgenic plants were sensitive to high Mg2+ level, and got severe necrotic lesions,Citation24,25 consistent with our results that the expression of AtMHX was inhibited by MgT.Citation1 These possibly indicate that AtMHX mainly maintains metal and proton homeostasis in cells, the homeostasis is essential for photosynthesis and numerous enzymatic reactions.Citation11 However, AtMHX and its upstream regulators in the signal transduction responding to MgSs are still unclear.
Most of the known Mg transporters (MgTRs) are CorA transporters,Citation26 referring to reviews.Citation27,28 Ten CorA superfamily AtMGT genes were cloned from Arabidopsis,Citation6,29 and were considered as the primary Mg2+ transporters in high plants that could play key roles in Mg2+ uptake, distribution and homeostasis in cells. Moreover, the importance of these genes and transporters could be verified from their diverse subcellular localization, for instance, in plasma membrane, endoplasmic reticulum, vacuole, mitochondria, and chloroplast.Citation27,30 However, only AtMGT6,Citation30 AtMGT7,Citation31 AtMGT1,Citation32 double (AtMRS2–1/10) and triple (AtMRS2–1/5/10) knockout linesCitation33 were discovered in responding to Mg starvation. The dwarf phenotypes of the later 2 mutants under MgD treatments could be ameliorated with lowering Ca2+ concentration,Citation33 indicating the role of these transporters in Ca2+ and Mg2+ homeostasis in cells. It was found that atmgt1 plants had almost similar biomass and leaf chlorophyll content with wild types under high Mg2+ stress (MgT) but the expression levels of AtMGT1, AtMGT7 and AtMHX were up-regulated in the wild type though non-significantly.Citation10 Although little change in the expression levels of these MgTRs were observed in microarray analysis when the plants were under MgSs,Citation8-10 results obtained from real time PCR detection showed significant changes for many MgTRs, for example AtMGT6 responding to Mg starvation.Citation30 We recently found that 8 AtMGTs and AtMHX were responding to MgTCitation1 and the findings were consistent with results obtained from serpentine conditions, where 6 MgTRs were highly expressed in the mesophyll and some could be correlated with higher accumulation of Mg in plants.Citation34 These indicate that most of MgTRs are more sensitive to MgT than to MgD.
AtMGT5 and AtMGT9 are essential for pollen development and male fertility.Citation34,35 Transgenic plants with overexpression of AtMGT1 and OsMGT1 were tolerant to aluminum (Al) and manganese toxicityCitation14,32 might be due to increased Mg accumulation in cytosol.Citation36,37 However, low Mg status in plants enhanced tolerance to cadmium.Citation38 Recently, it was found that MgTR AtCNGC10 is in charge of MgCitation2+ uptake and long-distance transport.Citation39 Other ion transporters could take part in response to MgSs. For example, cax1 (a member of tonoplast calcium-proton antiporter proteins), cas3 and cax1/cax3, showed better tolerance to serpentine soils with low Ca2+ and high MgCitation2+.40-42 These indicate that MgTRs may play more roles in plant development and growth exception of Mg transportation and other ion transporters may also involve in MgSs' responses. On the other hand, Mg may have some interactions with other ions.
However, there are still many questions to be answered: 1) what are the functions of MgTRs in plants, especially their reaction mechanisms to MgSs, since most of MgTRs has not been exactly evaluated? 2) Are there any other undiscovered MgTRs for MgCitation2+ uptake or intracellular distribution exist in plants? 3) Are AtMHX and some of AtMGTs responding to MgSs truly regulated by some uncharted signal transduction pathways, such as ABA and auxin signalings? 4) Do MgTRs also participate in other plant growth and development pathways, for example in pollen development and Al tolerance? In fact, we recently found that ABA and DELLA proteins participate in signalings response to long-term MgT in Arabidopsis thaliana.Citation1 A DELLA-Q mutant, which lacked 4 DELLA proteins, showed lower MgCitation2+ uptake and expression levels of some MgTRs under MgT conditions as compared to WT, implying some uncharted signal pathways from phytohormones to downstream MgTRs.
Signal Transduction of Magnesium Stresses in Plants
MgT significantly increases the transcriptional levels of many genes encoding enzymes in phytohormone biosyntheses, such as ABA (9-cis-epoxycarotenoid dioxygenase), ethylene (1-aminocyclopropane-1-carboxylate synthase) and jasmonic acid (lipoxygenase),Citation10 these are widely considered to mediate biotic or abiotic stress responses in plants. In Arabidopsis, we found that content of ABA increased more than 5 times in plants treated with MgT as compared to the untreated ones.Citation1 The transcriptional expression levels of ABA biosynthesis genes such as ABA1 and ABA2 were also accordingly elevated in different degrees under MgTCitation1. Furthermore, many promoter sequences of the significantly regulated genes under MgT were found containing the ABA motifs,Citation10 and coincidentally, the expression of transporter AtMHX is induced by auxin and ABA in vascular tissues.Citation11 These results suggest that ABA may play a critical role in response to MgT in plants. Our results support this hypothesis. Plants of an ABA-insensitive mutant abi1–1grew well under MgT conditions comparing with wild plants, and both exogenous ABA and MgT treatments accumulated DELLA protein RGA,Citation1 but RGA was not accumulated in abi1–1 plants with ABA treatment.Citation43 DELLA proteins (DELLAs), nuclear-localized, are core transcriptional negative regulators of gibberellin signaling, and 5 members were found in Arabidopsis genome [repressor of GA1–3 (RGA), RGA like1 (RGL1), RGL2, RGL3 and gibberellin-insensitive (GAI)].Citation43 DELLAs involve many adaptive responses including salinity, cold, drought, light and phosphate starvation, and act as core cross-talk nodes for several phytohormone signalings such as gibberellin, auxin, ethylene, ABA, brassinosteroid, salicylic acid and jasmonate.Citation44 Therefore, it could be deduced that MgT may induce ABA biosynthesis that could improve DELLAs accumulation through ABI1. Moreover, high content of DELLAs consequently inhibits plant growth and development (). A constitutive elevated GA response mutant DELLA-Q was used to confirm the inference. It was observed that DELLA-Q plants grew well, similar to phenotypes of abi1–1 plants with MgT treatment.Citation1 The subsequent analysis of DELLA-Q and wild plants demonstrated that loss of 4 DELLAs could decrease Mg and ABA excessive accumulation in plants by decreasing expression levels of some MgTRs and ABA biosynthesis enzymes. Moreover, the mutation of 4 DELLAs could increase starch and sugar content through slight upregulation of expression of starch biosynthesis enzymes and down-regulation of starch degradation enzymesCitation1. Therefore, DELLAs could possibly be responsible for restraining plant growth and development under MgT (). Recently, Niu et al.Citation19 reported that reactive oxygen species (ROS) and cytosolic Ca2+ could be the signal factors responding to MgSs, hence, validated the previous hypothesis that antioxidation might be one of the early responses to MgSs.Citation9,21,45
Figure 2. Schematic hypothetical model of the signaling networks responding to magnesium stresses in plants, referring to references herein. Abbreviations: MgD, magnesium deficiency; MgT, magnesium toxicity; MgTRs, magnesium transporters; ABA, abscisic acid; ROS, reactive oxygen species.
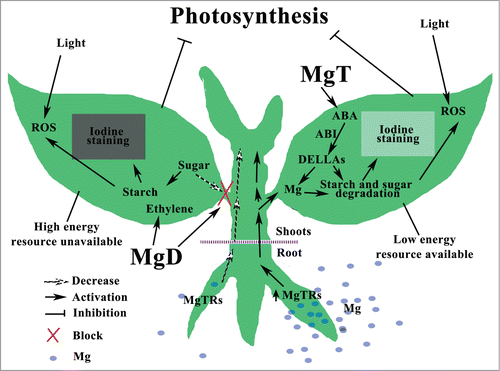
Generally, MgD blocks sugar transport through phloem from leaves to roots, this improves starch accumulation in leaves and feedback inhibits photosynthesis, increases ROS in cell, and consequently restrains plant growth.Citation4,21,28 However, the signal transduction reacting to MgD is known little (). Hermans et alCitation8 reported that no changes of ABA contents were observed in plants treated with MgD, even though half of the upregulated MgD response genes in leaves are ABA responsive. However, as reported recently, ABA levels could be significantly increased in both plant roots and shoots treated with MgTCitation1. Hermans et alCitation9 also found that ethylene played a key role in response to MgD, as the expression levels of several genes (e.g. At5g43450, At1g06620 and At2g25450) encoding enzymes in the C2H4 biosynthetic pathway were enhanced. Consequently the MgD treated plants produced twice as much C2H4 gas as control plants. Those results showed that response and adaptation of plants to MgSs have some unique signaling pathways with possible interactions among them. Although similar phenotypes induced by MgD and MgT in plants, they might employ different signal transduction pathways. However, a whole picture of MgS response signaling in plants is still hidden. Fortunately, the newest researches.Citation1,19,30,33,Citation36 offer us novel aspects on plant physiology responding to MgSs. As shown in , a hypothetical schematic model of the signaling networks responding to MgSs in plants is being proposed. Overly accumulation of starch in response to MgD and degradation of starch in leaves under MgT could either directly inhibit photosynthesis or cause loss of energy during photosynthesis, respectively, that could lead to ROS accumulation in cells. Furthermore, ROS might generate signals that could cause disruption of subcellular organelle responding to MgSs in plants. Recent studies indicate that some phytohormones are involved in the signaling response to MgSs in plants,Citation1,8,9,10 for instance, ethylene to MgD,Citation9 and ABA to MgT.Citation1 For MgT, ABA might depend on DELLAs to inhibit plant growth (ABA-ABI1-DELLA-plant). Significantly, MgT improves Mg uptake, along with high expression levels of some MgTRs in plants,Citation1 whereas, plant Mg decreases quickly with MgD treatments.Citation9
Future Challenges
That ‘the tip of the iceberg' was used to describe the discovery of MgTRs in plants a decade ago,Citation7 however, functions of many MgTRs are unclear and experimental evidence is still lacking that can prove the increased accumulation of Mg in plants in response to MgD or crop growth improvement in MgT areas through genetic modification of those MgTRs. The study of signaling in plants reacting to MgSs is also just a beginning. However, there are some challenges limiting the efforts to unwind the functional mysteries of Mg in plants. Unlike other ions such as potassium, phosphate and iron, few Arabidopsis mutantsCitation42 were found to be responding to MgSs. Moreover, with the existing knowledge about the biological roles of Mg, we cannot distinguish the factors involved in regulating the signaling pathways in response to MgSs.Citation1-4 However, the genomic, transcriptomic and proteomic technologies are developed rapidly in last several years, and this will strongly boost the research process of the signaling pathways of MgSs in plants.
Disclosure of Potential Conflicts of Interest
No potential conflicts of interest were disclosed.
Acknowledgments
We thank Dr. Frantisek Baluska for kindly inviting this review and an anonymous reviewer for comments.
Funding
This work was supported by the Science Foundation of Zhejiang Sci-Tech University [14042008-Y]; the Zhejiang Provincial Natural Science Foundation of China [LY13C130001]; the Zhejiang Provincial Top Key Discipline of Biology [2012A03-C]; the Nature Science Foundation of China [grant Nos. 31270299 and 81373908]; the Program for New Century Excellent Talents in University [NCET-11–0440].
References
- Guo W, Cong Y, Hussain N, Wang Y, Liu Z, Jiang L, Liang Z, Chen K. The remodeling of seedling development in response to long-term magnesium toxicity and regulation by ABA-DELLA signaling in Arabidopsis. Plant Cell Physiol 2014; 55:1713-26; PMID:25074907; http://dx.doi.org/10.1093/pcp/pcu102
- Cowan JA. Structural and catalytic chemistry of magnesium-dependent enzymes. Biometals 2002; 15:225-35; PMID:12206389; http://dx.doi.org/10.1023/A:1016022730880
- Maguire ME, Cowan JA. Magnesium chemistry and biochemistry. Biometals 2002; 15:203-10; PMID:12206387; http://dx.doi.org/10.1023/A:1016058229972
- Hermans C, Verbruggen N. Physiological characterization of Mg deficiency in Arabidopsis thaliana. J Exp Bot 2005; 56:2153-61; PMID:15983014; http://dx.doi.org/10.1093/jxb/eri215
- Shaul O, Hilgemann DW, de-Almeida-Engler J, Van Montagu M, Inze D, Galili G. Cloning and characterization of a novel Mg2+/H+ exchanger. EMBO J 1999; 18:3973; PMID:10406802; http://dx.doi.org/10.1093/emboj/18.14.3973
- Li L, Tutone AF, Drummond RS, Gardner RC, Luan S. A novel family of magnesium transport genes in Arabidopsis. Plant Cell 2001; 13:2761-75; PMID:11752386; http://dx.doi.org/10.1105/tpc.13.12.2761
- Shaul O. Magnesium transport and function in plants: the tip of the iceberg. Biometals 2002; 15:309-23; PMID:12206396; http://dx.doi.org/10.1023/A:1016091118585
- Hermans C, Vuylsteke M, Coppens F, Craciun A, Inze D, Verbruggen N. Early transcriptomic changes induced by magnesium deficiency in Arabidopsis thaliana reveal the alteration of circadian clock gene expression in roots and the triggering of abscisic acid-responsive genes. New Phytol 2010; 187:119-31; PMID:20406411; http://dx.doi.org/10.1111/j.1469-8137.2010.03258.x
- Hermans C, Vuylsteke M, Coppens F, Cristescu SM, Harren FJ, Inze D, Verbruggen N. Systems analysis of the responses to long-term magnesium deficiency and restoration in Arabidopsis thaliana. New Phytol 2010; 187:132-44; PMID:20412444; http://dx.doi.org/10.1111/j.1469-8137.2010.03257.x
- Visscher AM, Paul AL, Kirst M, Guy CL, Schuerger AC, Ferl RJ. Growth performance and root transcriptome remodeling of Arabidopsis in response to Mars-like levels of magnesium sulfate. PLoS ONE 2010; 5:e12348; PMID:20808807; http://dx.doi.org/10.1371/journal.pone.0012348
- David-Assael O, Berezin I, Shoshani-Knaani N, Saul H, Mizrachy-Dagri T, Chen J, et al. AtMHX is an auxin and ABA-regulated transporter whose expression pattern suggests a role in metal homeostasis in tissues with photosynthetic potential. Funct Plant Biol 2006; 33:661-72; http://dx.doi.org/10.1071/FP05295
- Broadley MR, Hammond JP, King GJ, Astley D, Bowen HC, Meacham MC, Mead A, Pink DA, Teakle GR, Hayden RM, et al. Shoot calcium and magnesium concentrations differ between subtaxa, are highly heritable, and associate with potentially pleiotropic loci in Brassica oleracea. Plant Physiol 2008; 146:1707-20; PMID:18281414; http://dx.doi.org/10.1104/pp.107.114645
- Sun X, Kay AD, Kang H, Small GE, Liu G, Zhou X, Yin S, Liu C. Correlated biogeographic variation of magnesium across trophic levels in a terrestrial food chain. PLoS ONE 2013; 8:e78444; PMID:24223807; http://dx.doi.org/10.1371/journal.pone.0078444
- Chen ZC, Yamaji N, Motoyama R, Nagamura Y, Ma JF. Up-regulation of a magnesium transporter gene OsMGT1 is required for conferring aluminum tolerance in rice. Plant Physiol 2012; 159:1624-33; PMID:22732245; http://dx.doi.org/10.1104/pp.112.199778
- Brady KU, Kruckeberg AR, Jr HDB. Evolutionary ecology of plant sdaptation to serpentine soils. Annu Rev Ecol Evol Syst 2005; 36:243-66; http://dx.doi.org/10.1146/annurev.ecolsys.35.021103.105730
- Chiarucci A, Baker A. Advances in the ecology of serpentine soils. Plant Soil 2007; 293:1-2; http://dx.doi.org/10.1007/s11104-007-9268-7
- Niu Y, Chai R, Liu L, Jin G, Liu M, Tang C, Zhang Y. Magnesium availability regulates the development of root hairs in Arabidopsis thaliana (L.) Heynh. Plant Cell Environ 2014; 37(12):2795-813; PMID:24851702; http://dx.doi.org/10.1111/pce.12362
- Venkatesan S, Jayaganesh S. Characterisation of magnesium toxicity, its influence on amino acid synthesis pathway and biochemical parameters of tea. Res J Phytochem 2010; 4:67-77.
- Niu Y, Jin G, Zhang YS. Root development under control of magnesium availability. Plant Signal Behav 2014; 9:e29720; PMID:25029279; http://dx.doi.org/10.4161/psb.29720
- Gruber BD, Giehl RFH, Friedel S, von Wirén N. Plasticity of the Arabidopsis root system under nutrient deficiencies. Plant Physiol 2013; 163:161-79; PMID:23852440; http://dx.doi.org/10.1104/pp.113.218453
- Cakmak I, Kirkby EA. Role of magnesium in carbon partitioning and alleviating photooxidative damage. Physiol Plant 2008; 133:692-704; PMID:18724409; http://dx.doi.org/10.1111/j.1399-3054.2007.01042.x
- Akua T, Berezin I, Shaul O. The leader intron of AtMHX can elicit, in the absence of splicing, low-level intron-mediated enhancement that depends on the internal intron sequence. BMC Plant Biol 2010; 10:1-10; PMID:20047654; http://dx.doi.org/10.1186/1471-2229-10-93
- David-Assael O, Saul H, Saul V, Mizrachy-Dagri T, Berezin I, Brook E, Shaul O. Expression of AtMHX, an Arabidopsis vacuolar metal transporter, is repressed by the 5′ untranslated region of its gene. J Exp Bot 2005; 56:1039-47; PMID:15710632; http://dx.doi.org/10.1093/jxb/eri097
- Gaash R, Elazar M, Mizrahi K, Avramov-Mor M, Berezin I, Shaul O. Phylogeny and a structural model of plant MHX transporters. BMC Plant Biol 2013; 13:75; PMID:23634958; http://dx.doi.org/10.1186/1471-2229-13-75
- Berezin I, Mizrachy-Dagry T, Brook E, Mizrahi K, Elazar M, Zhuo S, Saul-Tcherkas V, Shaul O. Overexpression of AtMHX in tobacco causes increased sensitivity to Mg2+, Zn2+, and Cd2+ ions, induction of V-ATPase expression, and a reduction in plant size. Plant Cell Rep 2008; 27:939-49; PMID:18327593; http://dx.doi.org/10.1007/s00299-007-0502-9
- Smith RL, Banks JL, Snavely MD, Maguire ME. Sequence and topology of the CorA magnesium transport systems of Salmonella typhimurium and Escherichia coli: identification of a new class of transport protein. J Biol Chem 1993; 268:14071; PMID:8314774
- Cong Y, Luo D, Chen K, Jiang L, Guo W. The development of magnesium transport systems in organisms (in Chinese). J Agr Biotechnol 2012; 20:837-48.
- Waters BM. Moving magnesium in plant cells. New Phytol 2011; 190:510-3; PMID:21496026; http://dx.doi.org/10.1111/j.1469-8137.2011.03724.x
- Schock I, Gregan J, Steinhauser S, Schweyen R, Brennicke A, Knoop V. A member of a novel Arabidopsis thaliana gene family of candidate Mg2+ ion transporters complements a yeast mitochondrial group II intron-splicing mutant. Plant J 2000; 24:489; PMID:11115130; http://dx.doi.org/10.1046/j.1365-313x.2000.00895.x
- Mao D, Chen J, Tian L, Liu Z, Yang L, Tang R, Li J, Lu C, Yang Y, Shi J, et al. Arabidopsis transporter MGT6 mediates magnesium uptake and is required for growth under magnesium limitation. Plant Cell 2014; 26(5):2234-2248.
- Gebert M, Meschenmoser K, Svidova S, Weghuber J, Schweyen R, Eifler K, Lenz H, Weyand K, Knoop V. A root-expressed magnesium transporter of the MRS2/MGT gene family in Arabidopsis thaliana allows for growth in low-Mg2+ environments. Plant Cell 2009; 21:4018-30; PMID:19966073; http://dx.doi.org/10.1105/tpc.109.070557
- Deng W, Luo K, Li D, Zheng X, Wei X, Smith W, Thammina C, Lu L, Li Y, Pei Y. Overexpression of an Arabidopsis magnesium transport gene, AtMGT1, in Nicotiana benthamiana confers Al tolerance. J Exp Bot 2006; 57:4235-43; PMID:17101715; http://dx.doi.org/10.1093/jxb/erl201
- Lenz H, Dombinov V, Dreistein J, Reinhard MR, Gebert M, Knoop V. Magnesium deficiency phenotypes upon multiple knockout of Arabidopsis thaliana MRS2 clade B genes can be ameliorated by concomitantly reduced calcium supply. Plant cell physiol 2013; 54:1118-31; PMID:23628997; http://dx.doi.org/10.1093/pcp/pct062
- Li L, Sokolov LN, Yang Y. A mitochondrial magnesium transporter functions in Arabidopsis pollen development. Mol Plant 2008; 1:675; PMID:19825572; http://dx.doi.org/10.1093/mp/ssn031
- Chen J, Li LG, Liu ZH, Yuan YJ, Guo LL, Mao DD, Tian LF, Chen LB, Luan S, Li DP. Magnesium transporter AtMGT9 is essential for pollen development in Arabidopsis. Cell Res 2009; 19:887-98; PMID:19436262; http://dx.doi.org/10.1038/cr.2009.58
- Bose J, Babourina O, Shabala S, Rengel Z. Low-pH and aluminum resistance in Arabidopsis correlates with high cytosolic magnesium content and increased magnesium uptake by plant roots. Plant Cell Physiol 2013; 54:1093-104; PMID:23620479; http://dx.doi.org/10.1093/pcp/pct064
- Goss MJ, Carvalho MJGPR. Manganese toxicity: The significance of magnesium for the sensitivity of wheat plants. Plant Soil 1992; 139:91-8; http://dx.doi.org/10.1007/BF00012846
- Hermans C, Chen J, Coppens F, Inzé D, Verbruggen N. Low magnesium status in plants enhances tolerance to cadmium exposure. New Phytol 2011; 192:428-36; PMID:21762164; http://dx.doi.org/10.1111/j.1469-8137.2011.03814.x
- Guo KM, Babourina O, Christopher DA, Borsic T, Rengel Z. The cyclic nucleotide-gated channel AtCNGC10 transports Ca2+ and Mg2+ in Arabidopsis. Physiol Plant 2010; 139:303-12; PMID:20210874
- Cheng N-H, Pittman JK, Barkla BJ, Shigaki T, Hirschi KD. The Arabidopsis cax1 mutant exhibits impaired ion homeostasis, development, and hormonal responses and reveals interplay among vacuolar transporters. Plant Cell 2003; 15:347-64; PMID:12566577; http://dx.doi.org/10.1105/tpc.007385
- Cheng NH, Pittman JK, Shigaki T, Lachmansingh J, LeClere S, Lahner B, Salt DE, Hirschi KD. Functional association of Arabidopsis CAX1 and CAX3 is required for normal growth and ion homeostasis. Plant Physiol 2005; 138:2048-60; PMID:16055687; http://dx.doi.org/10.1104/pp.105.061218
- Bradshaw HD, Jr. Mutations in CAX1 produce phenotypes characteristic of plants tolerant to serpentine soils. New Phytol 2005; 167:81-8; PMID:15948832; http://dx.doi.org/10.1111/j.1469-8137.2005.01408.x
- Achard P, Cheng H, De Grauwe L, Decat J, Schoutteten H, Moritz T, Van Der Straeten D, Peng J, Harberd NP. Integration of plant responses to environmentally activated phytohormonal signals. Science 2006; 311:91-4; PMID:16400150; http://dx.doi.org/10.1126/science.1118642
- Colebrook EH, Thomas SG, Phillips AL, Hedden P. The role of gibberellin signalling in plant responses to abiotic stress. J Exp Bot 2014; 217:67-75; http://dx.doi.org/10.1242/jeb.089938
- Cakmak I, Hengeler C, Marschner H. Changes in phloem export of sucrose in leaves in response to phosphorus, potassium and magnesium deficiency in bean plants. J Exp Bot 1994; 45:1251-7; http://dx.doi.org/10.1093/jxb/45.9.1251