Abstract
The fibrillogenesis of a peptide corresponding to residues 35–51 of human α-lactalbumin (1GYDTQAIVENNESTEYG17) can be dramatically enhanced by the addition of a tetrapeptide TDYG homologous to its C-terminus (TEYG). Generation of spontaneous hydrolytic products similar to this peptide was demonstrated by mass-spectrometry analysis of GYDTQAIVENNESTEYG peptide solution components during fibrillogenesis. Possible mechanisms and roles of short peptides in protein metabolism are discussed.
Introduction
Amyloid fibril formation is a common phenomenon in human pathology.Citation1 Recent studies have shown that certain diseases such as hereditary glaucomaCitation2,3 or influenza infectionCitation4 include a stage of amyloid-like oligomerization and amyloid fibril formation. A universal strategy for amyloidosis treatment currently has still yet to be developed.Citation5
The mechanisms of amyloid-like fibril and oligomer formation are highly analogous among various proteins. A number of fibrillogenic peptides (Amyloid-β peptide, amyloidogenic peptides from human transthyretin, T4 lyzozyme, and IAPP) have symmetrical palindrome-like structure (characterized by the presence of the point in the sequence, at an equal distance from which toward the N- and C-termini the same or similar charge/hydrophobicity amino acid residues are located).Citation7 Palindromic protein subsequences have been implicated to have a role in functional activity of enzymes as they often situate near protein activity sitesCitation8,9 and in a number of mammalian regulation system communications through specific protein-protein interactions.Citation8 Mirror-symmetry motifs are also thought to define structural properties of some proteins.Citation10 Symmetrical regions were found in the prion protein, e.g. an aromatic palindrome motif conserved among different speciesCitation11 and the AGAAAAGA palindrome in PrP that is required to generate a productive PrPSc-PrPC complex.Citation12 The mechanisms that may be employed by symmetrical sequences in most of those cases, and their role in amyloidogenesis in particular, remain uncertain.
We have demonstrated in the earlier workCitation6,7 that mirror-symmetric peptides WT (1GYDTQAIVENNESTEYG17) and ID (1GYDTQTVVNNNGHTDYG17) can form amyloid-like fibrils capable of stimulating human α-lactalbumin (HAL) oligomerization and fibrillogenesis under physiological conditions in vitro. Peptide WT is comprised of a HAL sequence representing conservative mirror-symmetry motif found in α-lactalbumins. The N- and C- termini of WT were the subject of our particular interest due to possible role of the palindromiC-terminal parts of this peptide in fibrillogenesis.
Results
Fibrillogenesis enhancement
To elucidate the role of terminal residues of the WT peptide during fibrillogenesis, we investigated the influence of a short peptide TDYG homologous to the C-terminus of the WT peptide upon fibrillogenesis of whole peptide. This tetrapeptide R (TDYG) was created through the substitution of the Glu (E) residue with an Asp (D) in the WT C-terminus (14TEYG17). The E to D substitution (that effected in removal of one methyl group) allowed us to easily distinguish the added tetrapeptide from any of WT spontaneous proteolysis product in mass-spectrometry and reverse-phase chromatography measurements. In control experiments (TEM and Thioflavine T assays) it was shown that the R peptide is not able to form fibrils under WT fibrillogenesis conditions (data not shown). When R peptide was added to WT in a double molar excess ratio, mature fibril formation commenced immediately after addition and was nearly completed in the first 0.5 hours of incubation at 55°C, as seen in TEM images () and confirmed by ThT assay (). It seems likely that fibril formation occurs much faster than our 0.5 hours estimate, as the sample becomes visibly turbid immediately after peptide R addition, and dynamic light scattering shows that large (>500 nm) aggregate formation has already occurred within the first 15 minutes of incubation even at room temperature (S.B. Landa, personal communications). In contrast, peptide WT alone did not form any substantial amount of amyloid-like fibrils after 0.5 hours of incubation (), as ThT assay of this sample showed only a small increase in ThT fluorescence (). The level of ThT fluorescence comparable to that seen in the presence of R was achieved in the pure WT sample only after 5 hours of incubation (), when mature amyloid-like fibrils become visible in the TEM image (). These results show that the addition of R peptide to the WT solution under optimal fibrillogenesis conditions leads to a rapid (within 0.5 hours) fibril formation.
Figure 1. Transmissive electron microscopy image of (a) WT peptide after 0.5 hours of incubation. (b) WT with R peptide together after 0.5 hours of incubation post-addition of R peptide. (c) WT peptide after 5 hours of incubation.
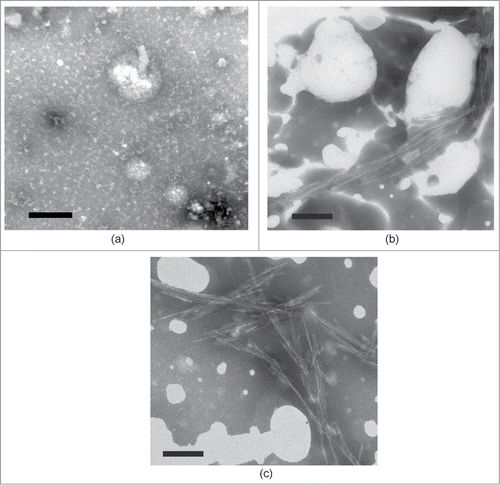
Figure 2. Fluorescence intensity of WT peptide with ThT. (A) WT peptide alone after 0.5 hours of incubation; (B) WT peptide incubated with R peptide for 0.5 hours; (C) WT peptide after 5 hours of incubation.
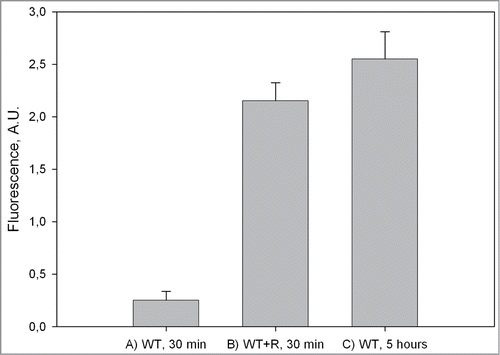
After a further 1 month incubation at +4°C, non-stimulated and stimulated fibrils were centrifuged at 14000 g for 15 minutes and the resultant supernatant was then further subjected to analytical chromatography using reverse-phase HPLC (). The supernatant contained almost exclusively peptide R while virtually all of the WT peptide has formed aggregates. The TEM images of the long-incubated samples are presented at respectively.
WT peptide hydrolysis
The WT peptide solutions were incubated in one of 2 buffers: pH 2, which stimulates fibrillogenesis, or pH 7.4, where fibrillogenesis does not occur. WT peptide dissolved in PBS-buffer, pH 7.4, was used as the negative control and was incubated under the same temperature conditions as WT peptide dissolved in Gly-HCl buffer, pH 2. After overnight incubation, samples were filtered through 50 nm filter and then further subjected to fractionation by column chromatography using preparative reverse-phase HPLC.
For identification of the collected fractions components, mass-spectrometry analysis was performed. WT peptide incubated in pH 7.4 PBS-buffer did not show any presence of fragments smaller than peptide monomer. Components with molecular masses corresponding to the monomer and partially deamidated monomer were detected. The chromatography peak corresponding to the deamidated form was not present in the freshly-made peptide solution and accounted for approximately 20% of the peptide. In the pH 2.0 incubated sample we found an intact WT and a variety of deamidated peptide forms. We also identified a significant fraction containing a fragment with a molecular mass corresponding to residues 14–17 of WT peptide, Citation14TEYGCitation17 (469.1 Da), . Although the other fragment of the peptide,Citation1GYDTQAIVENNES,Citation13 has not been identified, analysis did show numerous peaks corresponding to overlapping fragments of Citation1GYDTQAIVENNES,Citation13 such as Citation9ENNECitation5,12QAIVENCitation3,10DTQAIVE.Citation9
Discussion
We demonstrate that the addition of a small tetrapeptide drastically increases the rate of fibrillogenesis of peptide WT. Since, according to HPLC data, peptide R does not fully incorporate into the fibrils and by itself does not seem to be capable of oligomerization, the mechanism of its fibrilogenic properties most likely includes its interaction with WT species. Computer simulationsCitation7 have indicated that WT peptides can form oligomeric β-like structures via interaction of the aromatic tyrosine residues in the mirror symmetric tails (GYDT and TEYG) of the peptide molecules in antiparallel orientation. Due to more rapid diffusion and lack of steric interference, peptide R could more rapidly interact with the 1GYDT4 part of WT, stabilizing it in conformation predisposed to oligomerization. We could expect the tetrapeptide GYDT (N-terminal part of WT) to have a similar effect on WT fibrillogenesis. However, while our TEM and AFM measurements seemed to confirm the increased fibrillogenesis rate in WT-GYDT mixture (data not shown), the increased tendency of this tetrapeptide to self-oligomerization did not allow drawing any definite conclusions from those experiments.
Fibrillogenesis of peptide WT in the absence of R peptide could follow a similar mechanism, where the enhancer (TEYG) is produced via a spontaneous proteolysis step. We have observed a spontaneous cleavage of the peptide bond between the Ser13 and Thr14 (1GYDTQAIVENNES13*14TEYG17) of WT that occurs under fibrillogenic conditions. The mechanism of this bond cleavage is not clear. It may be related to deamidation of one of the 2 asparagine residues,Citation13 which we have observed in WT after 18 hours of incubation at pH 7.4, and, more prominently, at pH 2. The peptide contains ENN motif, which has been reported be deamidation-prone.Citation14 Deamidation in the mirror-symmetry motif under consideration have also been reported in equine α-lactalbumine.Citation15 However, the mass-spectrometry measurements did not show any presence of ESTEYG fragment that would be produced by deamidation of the second asparagine of the ENN fragment and the subsequent peptide bond disruption via a succinimide intermediate.Citation13 The TEYG fragment clearly identified by mass-spectrometry following WT incubation at pH 2 imply that either deamidation-induced cleavage occurs via a mechanism different from described in,Citation13 or there are additional proteolysis steps that occur rapidly following ESTEYG formation.
It is known that the proteolysis and fibrillogenesis of proteins are interrelated. Proteolysis of the β-amyloid precursor protein is a crucial step for Alzheimer disease pathogenesis.Citation16. Proteolytic digestion of C-terminus of the human L55P transthyretin does not allow this protein to form fibrils at all.Citation17 It was shown that spontaneous disruption of peptide bonds is an important step for chicken egg white lysozyme (39% homology to HAL) fibrillogenesis.Citation18 The addition of proteolysed lysozyme to a solution containing intact lysozyme under conditions required for fibrillogenesis led to avoidance of lag-phase thereby significantly accelerating this process. Furthermore, it was shown that PrP fibrillogenesis can be accelerated by prion protein peptides.Citation19
Peptide WT represents a system where fibrillogenesis of a mirror-symmetric peptide is stimulated by a small specific part of such a peptide, which can in turn be produced by spontaneous proteolysis. While this mechanism is unlikely be realized in the amyloidosis of the entire α-lactalbumin (in fact, peptide R seems to have no effect on fibrillogenesis of HAL), it may conceivably be ulilized in some cases to transfer a conformation transition from one protein molecule to another. The simple model described here could be useful for the elucidation of the role of short peptides in stabilization of protein conformational changes as well as it may aid the development of an universal strategy for amyloidosis treatment.
Materials and Methods
Reagents
All chemicals and reagents were purchased from Sigma, USA. Peptides TDYG (R) and GYDTQAIVENNESTEYG (WT) were synthetized at NPF Verta Ltd. (purity greater than 90%).
WT fibrillogenesis
WT peptide was dissolved in Milli-Q water and then the pH of solution was lowered with 1M Gly-HCl buffer, pH 2.0, to a final concentration of 50 mM for the buffer, 1 mg/ml for WT peptide. The volume of the sample was 500 μl. The solution was incubated at 55°C for 5 hours with continuous agitation in an Eppendorf orbital shaker at 300 rpm. Aliquots taken at 0.5 hours and at 5 hours were analyzed by TEM and ThT assay. For the short peptide experiment, R peptide was dissolved in 50 mM Gly-HCl buffer and added to a solution containing WT peptide for a final concentation of 1 mg/ml of the WT peptide and a double molar excess of R peptide. Aliquots of R peptide combined with WT were analyzed at 0.5 hours and 5 hours by TEM and ThT .assay.
ThT assay
A modified ThT assay method by LeVine IIICitation20 was used. Aliquots (10 μl) of peptide solutions were added to 300 μl of 10μM ThT solution in PBS buffer. Measurements were performed in a HITACHI F-4010 fluorescence spectrophotometer (Hitachi, Japan) with excitation wavelength 440 nm (bandpass 5 nm) and emission wavelength 478 nm (bandpass 5 nm).
HPLC
Reverse-phase HPLC was performed using a BioLogic DuoFlow Pathfinder 20 system (BioRad, USA) and a YMC TriArt C18 column (250*4.6 mm, 5 μm grains) for analysis and YMC C18 (150*10 mm, 10 μm grains) for preparative purposes. The column was equilibrated with 5 ml of H2O:acetonitrile:TFA (97.9:2:0.1) solution at 1 ml/min flow rate. Then sample was injected and eluated with linear gradient of H2O:acetonitrile:TFA (2:97.9:0.1) solution at 1 ml/min (9 ml/min for preparative purposes) flow rate. Volume of the sample was 50 μl for analysis and 1000 μl for preparative purposes. Absorbance was read at 214 nm (corresponding to peptide bond and low-molecular-weight organic compound absorption) and 280 nm (corresponding to the absorption of amino acid residues containing an aromatic group). Chromatograms were analyzed with use of BioRad ChromLab software.
Mass-spectrometry
The WT fractions were desiccated in a SpeedVac. C18 ZipTips were prewashed with 100% acetonitrile followed by a wash with 0.1% trifluoroacetic acid (TFA). Dried fractions were dissolved in 0.1% TFA, loaded into a ZipTip, washed with 0.1% TFA, and finally eluted with a solution containing 70% acetonitrile and 0.1% TFA. About 0.4 mkl of samples were mixed on the target plate with 0.4 μl of 2,5-dihydroxybenzoic acid (DHB)(Aldrich) diluted to 20 mg/ml in 50% Acetonitril and 0.1% TFA and then dried under vacuum. Mass spectrometry was performed with a Bruker UltraFlex MALDI-TOF mass-spectrometer at the Institute of Physico-Chemical Medicine of Ministry of Healthcare of the Russian Federation (Moscow).
Electron microscopy
Electron microscopy was performed on a JEOL JEM 1000 microscope using the standard negative staining technique with phosphotungstic acid salts.
Disclosure of Potential Conflicts of Interest
No potential conflicts of interest were disclosed.
Acknowledgments
The authors wish to thank Brian Hoettels for his help in the manuscript preparation.
Funding
This work was supported by the federal target program “Scientific and Scientific-Pedagogical Personnel of Innovative Russia” for 2009–2013 (activity 1.5, agreement 8482) and partially supported by RFBR, research project No.14-24-01103.
References
- Merlini G, Bellotti V. Molecular mechanisms of Amyloidosis. N Engl J Med 2003; 349:583-596; PMID:12904524
- Orwig S, Perry C, Kim L, Turnage K, Zhang R, Vollrath D, Schmidt-Krey I, Lieberman R. Amyloid fibril formation by the glaucoma-associated olfactomedin domain of myocilin. J Mol Biol 2012; 421(2-3):242-255; PMID:22197377; http://dx.doi.org/10.1016/j.jmb.2011.12.016
- Egorov V, Grudinina N, Lebedev D, Shaldzhyan A, Slita A, Sirotkin A, Vasin A, Shavlovsky M. Amyloidogenic peptide homologous to fragment 129–148 of human myocilin. Prion 2013; 7(3):248-252; PMID:23764838; http://dx.doi.org/10.4161/pri.24239
- Chevalier C, A Al Bazzal J, Vidic V, Février C, Bourdieu E, Bouguyon R, Le Goffic J, Vautherot J, Bernard M, Moudjou S, et al. B1-F2 influenza A virus protein adopts a β-sheet conformation and forms amyloid fibers in membrane environments. J Biol Chem 2010; 285(17):13233-13243; PMID:20172856; http://dx.doi.org/10.1074/jbc.M109.067710
- Merlini G, Seldin D, Gertz M, Amyloidosis: pathogenesis and new therapeutic options. J Clin Oncol 2011; 29(14):1924-1933; PMID:21483018; http://dx.doi.org/10.1200/JCO.2010.32.2271
- Egorov V, Solovyov K, Grudinina N, Lebedev D, Isaev-Ivanov V, Kiselev O, Shawlovsky M, Atomic force microscopy study of peptides homologous to β-domain of α-lactalbumins. Prot Pept Lett 2007; 14(5):411-414; http://dx.doi.org/10.2174/092986607780782858
- Egorov V, Garmaj Y, Solovyov K, Grudinina N, Aleinikova T, Sirotkin A, Kiselev O, Shawlovsky M. Amyloidogenic peptide homologous to β-domain region of α-lactalbumin. Doklady Biochemistry Biophysics 2007; 414:152-154; PMID:17695325; http://dx.doi.org/10.1134/S1607672907030167
- Chipens GI, Ievina NG, Tsilinskis EE, Hidden symmetry of peptide and protein primary structures. Bioorg Khim 1992; 18(12):1445-1453; PMID:1300996
- Shpakov AO. Internal symmetry of the mirror type in the primary structure of proteins: identification and the functional role. J Evol Biochem Physiol 2000; 36 (6):685- 700; http://dx.doi.org/10.1023/A:1017570620437
- Sheari A, Kargar M, Katanforoush A, Arab S, Sadeghi M, Pezeshk H, Eslahchi C, Marashi SA. A tale of two symmetrical tails: structural and functional characteristics of palindromes in proteins. BMC Bioinformaticsn 2008; 9:274 ; http://dx.doi.org/10.1186/1471-2105-9-274
- Sulkowski E, Aromatic palindrome motif in prion proteins, FASEB J 1992; 6(6):2363; PMID:1544547
- Norstrom EM, Mastrianni JA. The AGAAAAGA palindrome in PrP is required to generate a productive PrPSc-PrPC complex that leads to prion propagation, J Biol Chem 2005; 280(29):27236-43; PMID:15917252; http://dx.doi.org/10.1074/jbc.M413441200
- Stephenson R, Clarke S, Succinimide formation from aspartyl and asparaginyl peptides as a model for the spontaneous degradation of proteins. J Biol Chem 1989; 264(11):6164-6170; PMID:2703484
- Chelius D, Rehder DS, Bondarenko PV, Identification and characterization of deamidation sites in the conserved regions of human immunoglobulin gamma antibodies. Anal Chem 2005; 77(18):6004-6011; PMID:16159134; http://dx.doi.org/10.1021/ac050672d
- Girardet J-M, N’negue M-A, Egito AS, Campagna S, Lagrange A, Gaillard J-L. Multiple forms of equine α-lactalbumin: evidence for N-glycosylated and deamidated forms, Int Dairy J 2004; 14(3):207-217; http://dx.doi.org/10.1016/j.idairyj.2003.08.001
- Selkoe D. Alzheimer disease: genes, proteins, and therapy, Physiological Rev 2001; 81(2):741-766; PMID:11274343
- Solovyov K, Gasteva A, Egorov V, Aleinikova T, Sirotkin A, Shvartsman A, Shavlovsky M. Role of the C-terminal fragment of human transthyretin in abnormal fibrillogenesis. Biochemistry(Moscow) 2006; 71:672-680
- Mishra R, Sörgjerd K, Nyström S, Nordigården A, Yu Y-C, Hammarström P. Lysozyme amyloidogenesis is accelerated by specific nicking and fragmentation but decelerated by intact protein binding and conversion. J Mol Biol 2007; 336:1029-1044; PMID:17196616
- Nguyen J, Baldwin M, Cohen F, Prusiner S, Prion protein peptides induce α-helix to β-sheet conformational transitions. Biochemistry 1995; 34(13):4186-4192; PMID:7703230; http://dx.doi.org/10.1021/bi00013a006
- LeVine H, III. Thioflavin T interaction with amyloid β-sheet structures. Amyloid: Int J Exp Clin Invest 1995; 2:1-6