Abstract
The cellular prion protein (PrPC) is a highly conserved protein, which is anchored to the outer surface of the plasma membrane. Even though its physiological function has already been investigated in different cell or mouse models where PrPC expression is either upregulated or depleted, its exact physiological role in a mammalian organism remains elusive. Recent studies indicate that PrPC has multiple functions and is involved in cognition, learning, anxiety, locomotion, depression, offensive aggression and nest building behavior. While young animals (3 months of age) show only marginal abnormalities, most of the deficits become apparent as the animals age, which might indicate its role in neurodegeneration or neuroprotection. However, the exact biochemical mechanism and signal transduction pathways involving PrPC are only gradually becoming clearer. We report the observations made in different studies using different Prnp0/0 mouse models and propose that PrPC plays an important role in the regulation of the cytoskeleton and associated proteins. In particular, we showed a nocodazole treatment influenced colocalization of PrPC and α tubulin 1. In addition, we confirmed the observed deficits in nest building using a different backcrossed Prnp0/0 mouse line.
Abbreviations
EPM | = | elevated plus maze |
FC | = | fear conditioning |
FST | = | forced-swimming test |
NOR | = | novel object recognition |
OF | = | open field |
TST | = | tail suspension test |
Introduction
The opportunity to study the function of PrPC improved dramatically with the creation of Prnp ablated transgenic mice.Citation1-4 These Prnp ablated mice were shown to be resistant to prion diseases.Citation5 Curiously, the Nagasaki, Rcm0, and Zurich II lines displayed a phenotype that was dramatically different from the Zurich I and Edinburgh lines. The Zurich I and Edinburgh lines, produced by simple disruption of an open reading frame of the Prnp gene, developed normally and appeared to have no obvious behavioral defects.Citation1,2 In contrast, the Nagasaki, Rcm0 and Zurich II mouse lines, produced by using more extensive deletion methodology, showed an impaired motor coordination and died of cerebellar ataxia resulting from loss of Purkinje cells.Citation3,4 The latter phenotype was discovered to be the consequence of a Prnp promoter driven expression of an adjacent protein, Doppel, in the brain and not the lack of PrPC.Citation3,4,6 Introducing the Prnp gene into these Doppel expressing mice rescues them from the effects of demyelination and Purkinje cell degradation.Citation7
Behavior Abnormalities of Prnp0/0 Mice Increased As The Mice Aged
The function of PrPC has been investigated by using different transgenic mouse models, a variety of behavioral tasks, and mice of different ages. These studies and their outcomes were summarized in . The initial studies of PrPC knockout mice (Prnp0/0) did not reveal any notable behavioral deficits or abnormalities.Citation1,2 Upon closer examination, interestingly, Prnp ablated mice showed subtle physiological defects. Mice lacking PrPC were observed to have sleep and circadian rhythm alterations that could be restored upon expression of the Prnp gene.Citation8,9 Recovery from sleep deprivation was noticeably slower in Prnp0/0 mice than in wild-type (WT) mice. Ablated mice showed hypercorticism during the night and following stress, and their adrenocorticotropic hormone remained high.Citation10
Table 1. Observed behavioral abnormalities in Prnp0/0 mouse lines with different genetic backgrounds
The behavior of Prnp0/0 mice and WT mice has been studied using a variety of well-established behavioral tests. PrP ablated mice performed as well as WT mice in the Morris Water maze, the Y maze discrimination test and the 2-way avoidance test.Citation2,11 More detailed studies revealed that Prnp0/0 (11 months) mice showed a decrease in locomotor activity in the open field test compared to their WT counterparts.Citation13 Furthermore, their nest building abilities were significantly poorer than WT controls.Citation12 These findings were initially observed in a Prnp0/0 mouse line with a mixed 129B6 backgroundCitation12 and later confirmed in backcrossed Prnp0/0 mice (C57BL/6NRJ) ). The Prnp0/0 mice also showed a decrease in memory performance, associative learning, and basal anxiety as they aged (starting at 9 months) compared to WT controls.Citation12
Figure 1. Deficits in nest building behavior of Prnp0/0 mice. (A) The nest building behavior of WT (1) and Prnp0/0 mice (2) was assayed by assessing the nest quality after overnight exposure to a sheet of tissue paper. (B) Comparing the nest quality, statistically significant differences were obtained between WT and Prnp0/0 mice independent of their age. Values are depicted as mean ± SEM (n = 6). The quality of the nests was evaluated using a modified 5-point scale according to method of Deacon et al.Citation39 Tissue not noticeably touched (>90 % intact, 1 point); tissue partially shredded (50–90% remained intact, 2 points); mostly shredded but not identifiable as a nest (>50 % of the tissue is shredded, 3 points), an almost intact nest (>90 % of the tissue is shredded, 4 points); an intact nest (100% of the tissue is shredded, 5 points).
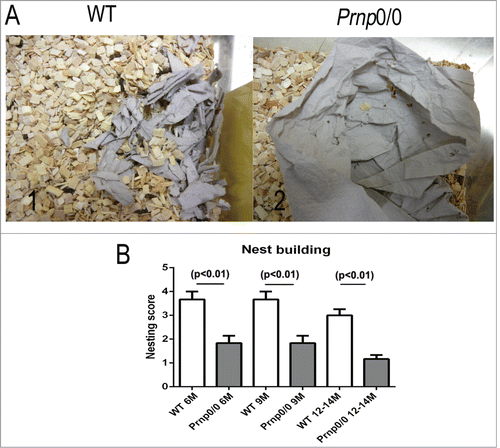
These observations were supported by other studies using the Zurich I Prnp0/0 mouse line which demonstrated that Prnp0/0 mice have cognitive deficits in short-term social recognition or short- and long-term memory retention ().Citation14-16 They had decreased anxiety after acute stress conditionsCitation17 but not under normal conditions.Citation14,18,19 Olfactory deficits in cookie finding, increased grooming and an increased latency to initiate exploration of a new environment () were also observed. An increased latency to initiate exploration and deficits in spatial learning were seen in Prnp0/0 mice on the 129/Ola background.Citation15 These age-dependent results have been interpreted to suggest that PrPC plays a role in protecting animals from the effects of aging, such as oxidative stress.Citation12,13
Prpn ablated and WT mice were used to study the role of PrPC in animals subjected to increased physiological demands, such as those with brain seizures or ischemia.Citation20 These studies demonstrated that PrPC expression in WT-mice was upregulated in response to focal cerebral ischemia. Prnp0/0 mice displayed significantly greater infarct volumes when compared to WT controls following both permanent and transient ischemia.Citation21 Even though it has been suggested that PrPC exerts neuroprotective effects via phosphatidylinositol 3-kinase (PI3K)/Akt20 and mitogen-activated protein kinase/extracellular signal-regulated kinase (MAPK/ERK) pathways,Citation22 the molecular mechanisms underlying PrPC mediated neuroprotection following ischemic brain injury appears to be different and will require further characterization.
Prnp0/0 mice were shown to be more sensitive to pain,Citation23 displayed depressive behavior,Citation24 and have altered aggressive behavior.Citation25 These results demonstrated that the engineered mice display different age-dependent and age-independent behaviors compared to WT controls.
When the behavioral studies of Prnp ablated mice were summarized and compared (), it was clear that they were not always consistent. These inconsistencies may have been due to the genetic background of the transgenic mice or the strain of mice (Nagasaki, Zurich I, Zurich II and Edinburgh strains), where the deletion of genes other than Prnp could not be excluded. Additional varying factors were the number of animals, the age of the mice (usually between 3 and 9 months) and inconsistences in applying behavioral tests which may result in contradictory findings. Moreover, it might be possible that PrP ablated mice have a strain-dependent compensatory mechanism that could have a specific strain-dependent impact.
Prnp0/0 Mice Exhibit Alterations in The Cytoskeleton
The cytoskeleton executes many important physiological functions, e.g. cell structural stability, maintenance of neurons, intracellular transport, metabolism, and cell division. Three principal kinds of filaments make up the cytoskeleton in eukaryotic cells, microfilaments, intermediate filaments, and microtubules. Disruption of the physiological metabolism of the cytoskeleton is associated with several neurodegenerative diseases, including Alzheimer's Disease (AD), Parkinson's Disease (PD), and Amyotrophic Lateral Sclerosis (ALS). In these diseases, the disruption of cytoskeletal metabolism is the result of a cascade of events including the toxic accumulation of affected proteins (tau), mitochondrial dysfunction, and oxidative stress.Citation26
These observations suggested an associative role for PrPC in maintaining the cytoskeleton, since many (>40%) of the PrPC binding partners, including actin, β-actin-like protein, vimentin, tubulin, cofilin-1 and profilin-1 were found to be involved with cytoskeletal processes.Citation27,28 We observed that several of the proteins comprising the intermediary filaments of the cytoskeleton, neurofilament heavy chain (NF-H), neurofilament light chain (NF-L), spectrin, α-internexin, or vimentin, were differentially abundant in the insoluble fractions of older (18 months) Prnp0/0 mice as compared to age matched controls.Citation12 Furthermore, the phosphorylation of NF-H protein was decreased in a Prnp-knock-down cell model as well as in old (18–20 months) Prnp0/0 mice as compared to age-matched controls.Citation12 This was found to be important, since elevated levels of NF phosphorylation during agingCitation29 are associated with an increase in the stability and organization of NFs within the cytoskeleton.Citation30 Interestingly, this decreased NF phosphorylation was found to be associated with a downregulation of the kinase Fyn. The regulation of Fyn kinase was found to occur via the formation of a protein complex between PrPC and caveolin-1.Citation31 These results indicate that PrPC is associated with cytoskeletal metabolism at the protein level.
At the physiological level, Prnp0/0 mice exhibit a reduced number of β tubulin III-positive neurons and pyramidal cells in the dentate gyrus, which indicates that PrPC has a role in the microtubule system.Citation12
The microtubular cellular structures in the cytoskeleton were found to play a central role in intracellular transport, metabolism, and cell division. Microtubule networks are used as tracks for intracellular protein trafficking. In other studies, tubulin was found to interact with PrPC.Citation27,32 The binding of PrPC to tubulin may result in the ordered oligomerization of tubulin and the inhibition of microtubule formation.Citation33 We investigated the potential role of PrPC in altering the metabolism of the tubulin cytoskeleton by incubating Prnp transfected murine hippocampus (HpL3–4) neuronal cells with a drug that interferes with microtubule polymerization, nocodazole (). When these cells were treated for 3 h and 24 h with nocodazole, an altered PrPC localization pattern was observed, where PrPC was situated nearer the cytosolic region of the cell (). We used the distribution of fluorescent intensities to quantify the colocalization rate of α tubulin 1 and PrPC and compared untreated and nocodazole treated cells.
Figure 2. Effect of nocodazole on α tubulin 1 and PrPC colocalization. HpL3–4 cells were transfected with Prnp (PrP+/+) and then treated with nocodazole (4 μM) for different time intervals. α tubulin 1 (green) and PrPC (red) were stained in (A) untreated cells and in nocodazole treated cells (3 and 24 hours) (B-C). Nuclei were stained with TO-PRO-3 fluorescent dye (Life-technologies). Distribution of PrPC and α tubulin 1 were analyzed using anti-PrPC (3F4) and anti-α tubulin 1 antibodies (Leica TCS SPE microscope). The scatter plots show the quantitative localization of α tubulin 1 and PrPC. After 3 h of treatment with nocodazole there was a significant loss of colocalization between α tubulin 1 and PrPC as compared to untreated cells. After 24 h of treatment colocalization of both proteins increased. At least 25 cells were observed per condition per experiment with an equal exposure time (Scale bar: 10 μm). The scatter plots of the individual pixels from paired images were generated by Image (WCIF plugin) software.
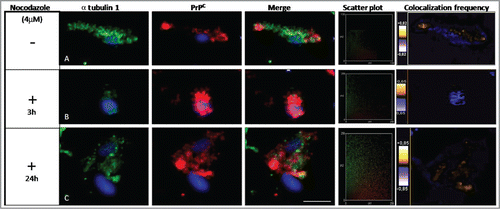
The distribution of fluorescence intensities in the scatter plots was used to quantify the localization of α tubulin 1 and PrPC after treatment with nocodazole. These results demonstrated a significant loss of colocalization between α tubulin 1 and PrPC after 3 h of treatment, as compared to untreated cells. However, after 24 hours of exposure to nocodazole, PrPC and α tubulin 1 showed more colocalization ().
We interpreted the reduction of the colocalization coefficient after 3 h of nocodazole treatment to be a consequence of degradation of the α tubulin 1 transport machinery. This would result in a lower amount of α tubulin 1 being expressed, which may result in the accumulation of PrPC in the cytosolic or nuclear region. As a potential consequence the colocalization rate may decrease. We presume the transport machinery can recover after 24 h which may result in the observed increase in the colocalization rate.
Table 2. α tubulin 1 partially colocalizes with PrPC. Pearson's correlation coefficient rP (−1 ≤ r p ≤ 1) demonstrated partial colocalization (p = 0.049, n = 5) between α tubulin 1 and PrPC in untreated HpL3–4 cells transfected with PrPC. Treatment of the cells with nocodazole for 3 h or 24 h showed less colocalization as compared to untreated cells. Colocalization coefficients, M1 and M2 ranged between 0 and 1, showing partially colocalized pixels of interest within each channel. The cell lysates from HpL3–4 treated cells were then used to verify PrPC and α tubulin 1 expression.
Moreover, we quantified the colocalization rates using Pearson's correlation coefficients (). This analysis confirmed that α tubulin 1 and PrPC showed altered colocalization after 3 h treatment with nocodazole. If the cells were incubated with nocodazole for 24 hours, PrPC and α tubulin 1 were observed to share the same compartments (), which showed that the effects of nocodazole on the organization of microtubules were reversible.Citation34
Conclusion
These results and those summarized provide strong evidence of the import role that PrPC plays in brain function. PrPC has a role in memory, associative learning, general anxiety, nesting (confirmed on a backcrossed Prnp0/0 mouse line), aggression, and depression. Deficits in cognition, learning and fear increased with age, which suggests that PrPC has a neuroprotective function with respect to brain trauma and stress.
Furthermore, PrPC is associated with all 3 parts of the cytoskeleton (microtubules, intermediary filaments and microfilaments) either as a regulator or as an interaction partner. Moreover, it colocalizes with α tubulin 1, an important component of microtubules, suggesting a molecular mechanism for the age-associated structural changes we observed in the cytoskeleton.
Disclosure of Potential Conflicts of Interest
No potential conflicts of interest were disclosed.
Acknowledgments
The authors wish to acknowledge Melissa L. Erickson-Beltran for her help in preparing this manuscript.
Funding
The work was supported by a grant from the European Commission: Protecting the food chain from prions: shaping European priorities through basic and applied research (priority, no. 222887) project number: FP7-KBBE-2007–2A and Neurodegenerative Disease Research (JPND – DEMTEST: Biomarker based diagnosis of rapidly progressive dementias-optimization of diagnostic protocols, 01ED1201A) as well as from the Alzheimer-Forschungs-Initiative e.V. (AFI 12851).
References
- Manson JC, Clarke AR, Hooper ML, Aitchison L, McConnell I, Hope J. 129/Ola mice carrying a null mutation in PrP that abolishes mRNA production are developmentally normal. Mol Neurobiol 1994; 8:121-7; PMID:7999308; http://dx.doi.org/10.1007/BF02780662
- Bueler H, Fischer M, Lang Y, Bluethmann H, Lipp HP, DeArmond SJ, Prusiner SB, Aguet M, Weissmann C. Normal development and behaviour of mice lacking the neuronal cell-surface PrP protein. Nature 1992; 356:577-82; PMID:1373228; http://dx.doi.org/10.1038/356577a0
- Moore RC, Lee IY, Silverman GL, Harrison PM, Strome R, Heinrich C, Karunaratne A, Pasternak SH, Chishti MA, Liang Y, et al. Ataxia in prion protein (PrP)-deficient mice is associated with upregulation of the novel PrP-like protein doppel. J Mol Biol 1999; 292:797-817; PMID:PMID:10525406; http://dx.doi.org/10.1006/jmbi.1999.3108
- Rossi D, Cozzio A, Flechsig E, Klein MA, Rulicke T, Aguzzi A, Weissmann C. Onset of ataxia and Purkinje cell loss in PrP null mice inversely correlated with Dpl level in brain. EMBO J 2001; 20:694-702; PMID:11179214; http://dx.doi.org/10.1093/emboj/20.4.694
- Bueler H, Aguzzi A, Sailer A, Greiner RA, Autenried P, Aguet M, Weissmann C. Mice devoid of PrP are resistant to scrapie. Cell 1993; 73:1339-47; PMID:8100741; http://dx.doi.org/10.1016/0092-8674(93)90360-3
- Li A, Sakaguchi S, Shigematsu K, Atarashi R, Roy BC, Nakaoke R, Arima K, Okimura N, Kopacek J, Katamine S. Physiological expression of the gene for PrP-like protein, PrPLP/Dpl, by brain endothelial cells and its ectopic expression in neurons of PrP-deficient mice ataxic due to Purkinje cell degeneration. Am J Pathol 2000; 157:1447-52; PMID:11073804; http://dx.doi.org/10.1016/S0002-9440(10)64782-7
- Nishida N, Tremblay P, Sugimoto T, Shigematsu K, Shirabe S, Petromilli C, Erpel SP, Nakaoke R, Atarashi R, Houtani T, et al. A mouse prion protein transgene rescues mice deficient for the prion protein gene from purkinje cell degeneration and demyelination. Laboratory Invest 1999; 79:689-97; PMID:10378511
- Tobler I, Gaus SE, Deboer T, Achermann P, Fischer M, Rulicke T, Moser M, Oesch B, McBride PA, Manson JC. Altered circadian activity rhythms and sleep in mice devoid of prion protein. Nature 1996; 380:639-42; PMID:8602267; http://dx.doi.org/10.1038/380639a0
- Tobler I, Deboer T, Fischer M. Sleep and sleep regulation in normal and prion protein-deficient mice. J Neurosci 1997; 17:1869-79; PMID:9030645
- Sanchez-Alavez M, Criado JR, Klein I, Moroncini G, Conti B. Hypothalamic-pituitary-adrenal axis disregulation in PrPC-null mice. Neuroreport 2008; 19:1473-7; PMID:18797300; http://dx.doi.org/10.1097/WNR.0b013e32830f1e90
- Lipp HP, Stagliar-Bozicevic M, Fischer M, Wolfer DP. A 2-year longitudinal study of swimming navigation in mice devoid of the prion protein: no evidence for neurological anomalies or spatial learning impairments. Behav Brain Res 1998; 95:47-54; PMID:9754876; http://dx.doi.org/10.1016/S0166-4328(97)00209-X
- Schmitz M, Greis C, Ottis P, Silva CJ, Schulz-Schaeffer WJ, Wrede A, Koppe K, Onisko B, Requena JR, Govindarajan N, et al. Loss of Prion Protein Leads to Age-Dependent Behavioral Abnormalities and Changes in Cytoskeletal Protein Expression. Mol Neurobiol 2014; 50:923-36; PMID:24604355.
- Rial D, Duarte FS, Xikota JC, Schmitz AE, Dafre AL, Figueiredo CP, Walz R, Prediger RD. Cellular prion protein modulates age-related behavioral and neurochemical alterations in mice. Neuroscience 2009; 164:896-907; PMID:19747526; http://dx.doi.org/10.1016/j.neuroscience.2009.09.005
- Coitinho AS, Roesler R, Martins VR, Brentani RR, Izquierdo I. Cellular prion protein ablation impairs behavior as a function of age. Neuroreport 2003; 14:1375-9; PMID:12876477
- Criado JR, Sanchez-Alavez M, Conti B, Giacchino JL, Wills DN, Henriksen SJ, Race R, Manson JC, Chesebro B, Oldstone MB. Mice devoid of prion protein have cognitive deficits that are rescued by reconstitution of PrP in neurons. Neurobiol Dis 2005; 19:255-65; PMID:15837581; http://dx.doi.org/10.1016/j.nbd.2005.01.001
- Nishida N, Katamine S, Shigematsu K, Nakatani A, Sakamoto N, Hasegawa S, Nakaoke R, Atarashi R, Kataoka Y, Miyamoto T. Prion protein is necessary for latent learning and long-term memory retention. Cell Mol Neurobiol 1997; 17:537-45; PMID:9353594; http://dx.doi.org/10.1023/A:1026315006619
- Nico PB, de-Paris F, Vinade ER, Amaral OB, Rockenbach I, Soares BL, Guarnieri R, Wichert-Ana L, Calvo F, Walz R, et al. Altered behavioural response to acute stress in mice lacking cellular prion protein. Behav Brain Res 2005; 162:173-81; PMID:15970215; http://dx.doi.org/10.1016/j.bbr.2005.02.003
- Lobão-Soares B, Walz R, Prediger RD, Freitas RL, Calvo F, Bianchin MM, Leite JP, Landemberger MC, Coimbra NC. Cellular prion protein modulates defensive attention and innate fear-induced behaviour evoked in transgenic mice submitted to an agonistic encounter with the tropical coral snake Oxyrhopus guibei. Behav Brain Res 2008; 194:129-37; PMID:18590772; http://dx.doi.org/10.1016/j.bbr.2008.06.006
- Roesler R, Walz R, Quevedo J, de-Paris F, Zanata SM, Graner E, Izquierdo I, Martins VR, Brentani RR. Normal inhibitory avoidance learning and anxiety, but increased locomotor activity in mice devoid of PrP(C). Brain Res Mol Brain Res 1999; 71:349-53; PMID:10521590
- Weise J, Sandau R, Schwarting S, Crome O, Wrede A, Schulz-Schaeffer W, Zerr I, Bahr M. Deletion of cellular prion protein results in reduced Akt activation, enhanced postischemic caspase-3 activation, and exacerbation of ischemic brain injury. Stroke; a journal of cerebral circulation 2006; 37:1296-300; PMID:16574930; http://dx.doi.org/10.1161/01.STR.0000217262.03192.d4
- McLennan NF, Brennan PM, McNeill A, Davies I, Fotheringham A, Rennison KA, Ritchie D, Brannan F, Head MW, Ironside JW, et al. Prion protein accumulation and neuroprotection in hypoxic brain damage. Am J Pathol 2004; 165:227-35; PMID:15215178; http://dx.doi.org/10.1016/S0002-9440(10)63291-9
- Spudich A, Frigg R, Kilic E, Kilic U, Oesch B, Raeber A, Bassetti CL, Hermann DM. Aggravation of ischemic brain injury by prion protein deficiency: role of ERK-1/-2 and STAT-1. Neurobiol Dis 2005; 20:442-9; PMID:15893468; http://dx.doi.org/10.1016/j.nbd.2005.04.002
- Gadotti VM, Zamponi GW. Cellular prion protein protects from inflammatory and neuropathic pain. Mol Pain 2011; 7:59; PMID:21843375; http://dx.doi.org/10.1186/1744-8069-7-59
- Gadotti VM, Bonfield SP, Zamponi GW. Depressive-like behaviour of mice lacking cellular prion protein. Behav Brain Res 2012; 227:319-23; PMID:21439331; http://dx.doi.org/10.1016/j.bbr.2011.03.012
- Tomaz B, Aljaz M, Ales J, Gregor M. Deletion of the prion gene Prnp affects offensive aggression in mice. Behav Brain Res 2014; 266:216-21; PMID:24631389; http://dx.doi.org/10.1016/j.bbr.2014.03.006
- McMurray CT. Neurodegeneration: diseases of the cytoskeleton? Cell Death Differ 2000; 7:861-5; PMID:11279530; http://dx.doi.org/10.1038/sj.cdd.4400764
- Zafar S, von Ahsen N, Oellerich M, Zerr I, Schulz-Schaeffer WJ, Armstrong VW, Asif AR. Proteomics approach to identify the interacting partners of cellular prion protein and characterization of Rab7a interaction in neuronal cells. J Proteome Res 2011; 10:3123-35; PMID:21604690; http://dx.doi.org/10.1021/pr2001989
- Zafar S, Asif AR, Ramljak S, Tahir W, Schmitz M, Zerr I. Anchorless 23-230 PrPC interactomics for elucidation of PrPC protective role. Mol Neurobiol 2014; 49:1385-99; PMID:24390569; http://dx.doi.org/10.1007/s12035-013-8616-2
- Veeranna, Yang DS, Lee JH, Vinod KY, Stavrides P, Amin ND, Pant HC, Nixon RA. Declining phosphatases underlie aging-related hyperphosphorylation of neurofilaments. Neurobiol Aging 2011; 32:2016-29; PMID:20031277; http://dx.doi.org/10.1016/j.neurobiolaging.2009.12.001
- Gotow T. Neurofilaments in health and disease. Med Electron Microsc 2000; 33:173-99; PMID:11810476; http://dx.doi.org/10.1007/s007950000019
- Pantera B, Bini C, Cirri P, Paoli P, Camici G, Manao G, Caselli A. PrPc activation induces neurite outgrowth and differentiation in PC12 cells: role for caveolin-1 in the signal transduction pathway. J Neurochem 2009; 110:194-207; PMID:19457127; http://dx.doi.org/10.1111/j.1471-4159.2009.06123.x
- Nieznanski K, Nieznanska H, Skowronek KJ, Osiecka KM, Stepkowski D. Direct interaction between prion protein and tubulin. Biochem Biophys Res Commun 2005; 334:403-11; PMID:16004966; http://dx.doi.org/10.1016/j.bbrc.2005.06.092
- Nieznanski K, Podlubnaya ZA, Nieznanska H. Prion protein inhibits microtubule assembly by inducing tubulin oligomerization. Biochem Biophys Res Commun 2006; 349:391-9; PMID:16934224; http://dx.doi.org/10.1016/j.bbrc.2006.08.051
- Polioudaki H, Kastrinaki MC, Papadaki HA, Theodoropoulos PA. Microtubule-interacting drugs induce moderate and reversible damage to human bone marrow mesenchymal stem cells. Cell proliferation 2009; 42:434-47; PMID:19486015; http://dx.doi.org/10.1111/j.1365-2184.2009.00607.x
- Nico PB, Lobão-Soares B, Landemberger MC, Marques W, Jr., Tasca CI, de Mello CF, Walz R, Carlotti CG, Jr., Brentani RR, Sakamoto AC, et al. Impaired exercise capacity, but unaltered mitochondrial respiration in skeletal or cardiac muscle of mice lacking cellular prion protein. Neurosci Lett 2005; 388:21-6; PMID:16039050; http://dx.doi.org/10.1016/j.neulet.2005.06.033
- Lobão-Soares B, Walz R, Carlotti CG, Jr., Sakamoto AC, Calvo F, Terzian AL, da Silva JA, Wichert-Ana L, Coimbra NC, Bianchin MM. Cellular prion protein regulates the motor behaviour performance and anxiety-induced responses in genetically modified mice. Behav Brain Res 2007; 183:87-94; PMID:17618696; http://dx.doi.org/10.1016/j.bbr.2007.05.027
- Meotti FC, Carqueja CL, Gadotti Vde M, Tasca CI, Walz R, Santos AR. Involvement of cellular prion protein in the nociceptive response in mice. Brain Res 2007; 1151:84-90; PMID:17433806; http://dx.doi.org/10.1016/j.brainres.2007.03.024
- Le Pichon CE, Valley MT, Polymenidou M, Chesler AT, Sagdullaev BT, Aguzzi A, Firestein S. Olfactory behavior and physiology are disrupted in prion protein knockout mice. Nat Neurosci 2009; 12:60-9; PMID:19098904; http://dx.doi.org/10.1038/nn.2238
- Deacon RMJ. Assessing nest building in mice. Nat Protocols 2006; 1:1117-1119: PMID:17406392; http://dx.doi.org/10.1038/nprot.2006.170