Abstract
Maintenance of haematopoietic stem cells and differentiation of committed progenitors occurs in highly specialized niches. The interactions of haematopoietic stem and progenitor cells (HSPCs) with cells, growth factors and extracellular matrix (ECM) components of the bone marrow (BM) microenvironment control homeostasis of HSPCs. We only start to understand the complexity of the haematopoietic niche(s) that comprises endosteal, arterial, sinusoidal, mesenchymal and neuronal components. These distinct niches produce a broad range of soluble factors and adhesion molecules that modulate HSPC fate during normal hematopoiesis and BM regeneration. Adhesive interactions between HSPCs and the microenvironment will influence their localization and differentiation potential. In this review we highlight the current understanding of the functional role of ECM- and adhesion (regulating) molecules in the haematopoietic niche during homeostatic and regenerative hematopoiesis. This knowledge may lead to the improvement of current cellular therapies and more efficient development of future cellular products.
Haematopoietic Stem Cells: Introduction
Billions of mature blood cells are produced daily from a limited number of haematopoietic stem cells (HSCs) in the bone marrow (BM) via a hierarchical differentiation process called hematopoiesis.Citation1-4 The number of HSCs is tightly regulated by intrinsic and extrinsic signals to balance the maintenance of the HSC population and the differentiation of HSCs into haematopoietic progenitors that supply the life-long daily need for mature blood cells.Citation5 Regulation of this balance also controls haematopoietic regeneration after, for instance, radiation or chemotherapy. The majority of HSCs is present in a quiescent state. In mice, HSCs divide on average once per 32 days, but a subpopulation of HSCs divides only 5 times during the lifespan of a mouse, although these cells are reversibly activated upon massive haematopoietic cell loss.Citation6,7 Depending on intrinsic and microenvironmental cues, HSC proliferation results in self-renewal or differentiation. HSC differentiation yields HSCs with limited self-renewal capacities or multipotent progenitors (MPPs) that lack self-renewal capacities and subsequently produce lineage-restricted progenitors.Citation5,8
The ability of environmental cues to affect stem cell fate is a concept from embryology that extends to the adult organism. Haematopoietic stem and progenitor cells (HSPCs) interact with neighboring cells, matrix components and supportive factors in the BM, which define a niche that is critical for the homeostatic maintenance of the HSC population.Citation9 Adhesive interactions between HSPCs and their microenvironment influence their self-renewal, proliferation and differentiation fate. However, it is not completely elucidated which adhesive molecules are involved in these processes, and how they exert these effects.
This review provides an overview of the features of haematopoietic stem cells and the BM niche, with emphasis on extracellular matrix (ECM), cellular adhesion molecules and other environmental cues that affect stem cell fate. First we focus on the ECM- and adhesion molecules being involved in the homeostatic situation. The second part focuses on adhesion molecules that are differentially regulated during BM regeneration. Comparative array studies of HSPCs revealed many adhesion molecules that were differentially regulated upon bone marrow injuryCitation10 and/or between HSPC differentiation stages.Citation11 In particular we focus on the ECM protein BIGH3 (transforming-growth-factor-β inducible gene h3), the adhesion molecules CD164 (Endolyn), CD166 (activated leukocyte cell adhesion molecule; ALCAM), Embigin and Biglycan and their role in the homeostatic and regenerative BM environment.
Haematopoietic stem cells: HSPC phenotypes
Great progress has been made in the functional and phenotypic characterization of adult HSPCs. Long-term human HSCs (LT-HSC) are defined by their ability to repopulate BM upon transplantation in sub-lethally irradiated non-obese diabetic severe combined immune deficiency (NOD-SCID) mice, and provide a life-long (>16 weeks) multi-lineage haematopoietic reconstitution, reviewed in Schroeder et al.Citation12 Strict definitions of LT-HSCs even require multi-lineage repopulation of secondary transplanted mice. Transplantation of short-term HSCs (ST-HSC) in sub-lethally irradiated NOD-SCID mice results in short-term reconstitution of the mice by all mature haematopoietic lineages, but only for a limited time course due to their limited self-renewal capacity. In vitro assays like cobble stone area forming cell (CAFC) assays are developed and calibrated to compare the in vitro stem cell capacity of cell populations with the in vivo repopulation capacities.Citation13
These functional features are correlated with the expression of cell surface markers that enables prospective isolation of specific populations of HS(P)Cs. HSC functional activity is detected in human CD34+CD38− BM cells,Citation14 and is more enriched in the population of protein tyrosine phosphatase CD45RA negative and thymus-antigen (Thy1; CD90) positive CD34+CD38− cells (CD34+CD38−CD45RA−CD90+).Citation15 Additional selection for Laminin-binding Integrin-α6 (ITGA6/CD49f) positive cells allowed isolation of a very pure human HSC population, as long-term multi-lineage repopulation was obtained in 28% of the injected mice, by single cell intrafemoral injection into female NOD-SCID- interleukin 2 receptor gamma chain (IL2Rg) null mice. The ITGA6− cells within the HSPC population showed only short-term multi-lineage reconstitution of the BM,Citation16,17 suggesting a major role for ITGA6 in the regulation of LT-HSCs. The most primitive, but extremely rare human HSCs, are CD34− and lineage negative, tyrosine protein kinase Kit (c-Kit; CD117) negative and Fms-like Tyrosine Kinase-3 negative (Lin−c-Kit−Flt3−) and the reconstitution capacities upon intrafemoral single cell injections are comparable to those of ITGA6+ HSCs.Citation18,19
Murine HSCs are isolated as Flt3−, CD34−, c-Kit-ligand positive, stem-cell-antigen-1 positive (Ly6A; Sca1) and lineage negative (Flt3−CD34−KitL+Sca1+Lin−)Citation20 or,alternatively, as signaling-lymphocytic-activation-molecule (SLAMF1; CD150) positive, SLAMF2 (CD48) negative, Integrin-α2B (CD41) negative and KitL+Sca1+Lin− (CD150+CD48−CD41−KitL+Sca+Lin−) cells.Citation21 Single cell transplantations of these populations showed in 20–33% of the injected mice a multi-lineage repopulation, reviewed in Challen & Goodell.Citation22 Heterogeneity still exists within these purified populations, indicated by intrinsically distinct lineage-bias or self-renewal capacities within these populations dependent on e.g. Integrin-α2 (ITGA2) and CD150 expression.Citation12,21-24 In addition, environmental cues are necessary for the maintenance of self-renewal, support of survival, proliferation and lineage instruction.Citation25,26
Haematopoietic stem cells: in vitro cell cultures of HSPCs
In vitro HSPC expansion has long relied on co-culture of HSCs with stromal or endothelial cells, which provide signals that suppress HSPC differentiation.Citation27 Nowadays, it is possible to expand HSPCs using cytokine cocktails in the absence of stromal cells. Expansion of murine HSPCs in feeder-free cell cultures was achieved by use of the Lodish and Zhang cocktail,Citation28 resulting in a 30-fold net HSPC expansion. Cytokine cocktails containing stem cell factor (SCF), Flt3-ligand (Flt3L) and thrombopoietin (TPO) occasionally supplemented with Interleukin-6 (IL-6), IL-11, IL-3 or granulocyte-macrophage-cell-stimulating-factor (GM-CSF) have been extensively studied in HSC expansion in co-cultures and feeder-free-cultures, reviewed in Sauvageau et al.Citation29 Haematopoietic regulators that specify HSPCs during ontogeny are also able to increase in vitro HSPC expansion. Addition of wingless-type-MMTV-integration-site-3A (Wnt3A) or Wnt5ACitation30-33 or an immobilized form of NotchCitation34,35 can induce HSPC expansion in vitro. Other promising developmental regulators that improve in vitro HSPC expansion include soluble Sonic Hedgehog, the endothelium produced insulin-like-growth factor 2 (IGF2), IGF binding protein (IGFBP2), angiopoietin-like proteins (Angplt) and the novel microenvironmental factor Pleiothrophin, that is involved in the coagulation cascade.Citation36 A group of small chemical molecules also includes potent inducers of HSPC expansion: the copper chelator tertraethylenepentamine (TEPA) attenuates HSPC differentiation, resulting in expansion of early progenitors.Citation37 Other promising chemical compounds are the histone deacetylase inhibitor Valproid Acid, the Wnt-agonist BIO, prostaglandin E2 (PGE2) that may inhibit apoptosis of HSPCs, and the TPO-agonist NR-101, reviewed in Walasek et al.Citation38 Despite all progress in HSPC expansion protocols, it is still impossible to maintain LT-HSCs in culture. HS(P)C fate determination is tightly regulated by extrinsic signals, including soluble molecules and complex outside-in-signaling networks.
Haematopoietic stem cells: normal life cycle of HSPCs
BM microenvironmental signals are needed to keep HSPCs localized in the BM, whereas other signals mobilize HSPCs into the circulation and vice versa mobilized HSPCs home back to the BM upon specific cues. These processes depend on directed HSPC migration, which involves signaling in response to chemotactic stimuli, polarization of signaling complexes, cytoskeletal remodeling and polarization of adhesive structures.Citation7,39 Chemokines bind G-coupled receptors on HSPCs, which triggers signaling cascades leading to directed motility. The most important chemokine for HSPCs is CXCL12 (also named stromal-derived-factor-1; SDF1). It is produced by BM-cells and bound by the chemokine receptors CXCR4 and CXCR7 on HSPCs.Citation40,41 In CXCL12 or CXCR4 deficient embryonic mice, haematopoietic precursors fail to shift from the fetal liver into the fetal BM.Citation42,43 In addition, CXCL12 is important for HSPC homeostasis, since conditional deletion of CXCR4 in adulthood reduced HSC numbers in the BM.Citation44 Both, CXCL12 and CXCR4 are essential for HSPC homing to and egress from the BM, and relocalization into different BM-microenvironments,Citation7,37,45 emphasizing that CXCL12 is required for normal BM-niche function.
Therapeutic mobilization of human HSPCs into the circulation is routineously obtained by administration of Granulocytic-colony-stimulating-factor (G-CSF) or G-CSF combined with cyclosphosphamide.Citation46 or other variants of chemotherapy. This inhibits osteoprogenitor proliferation, thereby reducing the CXCL12 concentrations in the BM and allowing HSPC egress.Citation47-49 In addition, G-CSF stimulates neutrophils to release proteases to further cleave CXCR4,Citation45,50 although non-proteolytic mechanisms seem to be dominant, as protease deficient mice show normal G-CSF induced HSPC mobilization.Citation51
Similarly, injection of GRObeta, the ligand of the chemokine-receptor CXCR2, mobilizes HSPCs from the BM into the circulation in mice, indicating an essential role of CXCR2 in maintaining the HSPC population localized in the BM-niche.Citation52 In addition to chemotactic proteins, gradients of the bioactive lipids sphingosine-1-phosphate and ceramide-1-phosphate induce HSPC migration, thereby cross-talking with chemokine-induced migration.Citation53-55 Furthermore, complement proteins (C1q, C3a, C5a), hyaluronic acid and pharmacological agents such as valproic acid can be used to induce HSPC mobilization, reviewed in Marquez-Curtis et al.Citation56 Interestingly, the soluble SLIT proteins are expressed by bone marrow (BM) stromal cells and function as chemorepellents. Their cognate ROBO receptors are expressed on HSPCs and previous work in our department demonstrated that pretreatment of human HSPCs with SLIT3 increased homing to the bone marrow of NOD-SCID mice.Citation57 Together, this emphasizes the complex regulation of HSPC maintenance in the BM-niche.
Bone Marrow Niches
Bone marrow niches: introduction
The BM microenvironment forms a specialized niche for HSPC maintenance. A specific HSPC niche was first proposed in 1978 by Schofield et al.Citation38 The adult BM consists of endosteal osteoblasts, lining the calcified bone matrix, endothelial cells, forming the arterial and venal vasculature, reticular cells, smooth muscle cells, adipocytes, macrophages, stromal cells and sparse sympathic nerves. These cells together shape into a structural network embedded in viscous ECM, thus forming a scaffold with which HSPCs can interact.
It has been proposed that HSCs are localized mainly in 2 different types of bone marrow niches, namely the endosteal niche, which is close to the bone and lined by osteoblasts,Citation58,59 and the vascular niche, which includes sinusoids and their endothelial cells.Citation21 Yet, this separation of 2 distinct niches might not be as strict as originally suggested, since vasculature is also found close to the endosteum. It has recently become clear that endothelial cells play a crucial role in the maintenance of self-renewal HSCs, among others through the production of stem cell factorCitation60 and E-selectin.Citation61 The HSC supporting function of the vascular bone marrow niche is further enhanced by a population of perivascular, Nestin-expressing stromal cells.Citation62 Moreover, a distinction between arteriolar and sinusoidal vasculature became evident, revealing that small arterioles support long-term repopulating HSCs, while sinusoids harbour less potent repopulating HSPCs.Citation63
The contribution of distinct cellular compartments to BM homeostasis was revealed by studies that use targeted deletion of growth factors or receptors. In addition, genetic mutagenesis and biochemical studies have established the role of distinct ECM proteins in BM homeostasis. A closer view at recent insights regarding the actual composition of the BM niche and the contribution of cellular and ECM components to BM homeostasis is provided here.
Bone marrow niches: endosteal niche
HSPCs reside preferentially in the spongeous bone in the metaphyse of the long bones, rather then in the diaphyse.Citation64 Within the spongeous bone HSPCs preferentially localize to the endosteal surfaces of osteoids, where bone matrix, osteoblasts, osteoclasts, and marrow meet.Citation65,66 Continuous remodeling of trabecular bone by osteoclasts liberates cytokines that are immobilized by proteoglycans and support HSC maintenance.Citation67 This scenario is supported by studies, showing that HSCs express a calcium-sensing receptor (CaR) and preferentially home to and are retained at calcium-enriched regions where trabecular bone is being remodeled.Citation68 Osteoblasts can expand long-term culture-initiating cells in vitro, and are shown to regulate the number of HSCs in vivo.Citation58,69-71 Osteoblast-specific activation of the parathyroid hormone (PTH) pathway induces expansion of osteoblasts and results in increased HSPC numbers, through enhanced Notch-ligand expression by the osteoblasts.Citation58 In addition, PTH treatment improved the engraftment and survival after BM transplantation.Citation58 However, activation of the PTH pathway in osteocytes did expand osteoblasts and trabecular bone, but not HSPCs,Citation58 indicating that osteoblast expansion itself is not sufficient for HSPC expansion. Similarly, multiplication of osteoblasts by the agent strontium did not increase HSPC numbers, suggesting that only certain subtypes of osteoblasts regulate HSPC numbers,Citation48,72 which may include Nestin+ peritrabecular cells.Citation58
Osteoblasts support HSPC maintenance by secretion of factors like CXCL12, osteopontin, angiopoietin-1, TPO and SCF.Citation48,73-75 In addition, osteoblasts function in HSPC retention in the BM. G-CSF reduces osteoblast numbers and lowers CXCL12 expression that results in mobilization of HPSCs into the blood.Citation76,77,45 Similarly, reduction of collagen-embedded osteocytes inhibited the osteoblast activity and impaired the G-CSF-induced HSPC mobilization.Citation48 This again illustrates that osteoblasts (indirectly) mediate the HSPC-BM-niche, by direct contact and secreted factors.Citation51
Bone marrow niches: vascular niche
Endothelial cells function as bi-directional gate keepers of the blood-BM-barrier and endothelial permeability is tightly regulated.Citation78 Treatment of mice with fibroblast-growth-factor-2 (FGF-2) decreased the BM endothelial permeability and inhibited the HSPC homing and egress, via inhibition of the Nitric Oxygen (NO) content, matrix-metallo-proteinase-9 (MMP9) activity and vascular-endothelial-cadherin (VE-Cadherin) shedding.Citation78
Endothelial cells contribute to the vascular niche, and promote HSPC proliferation, as opposed to the endosteal niche that promotes quiescence.Citation79,80 However, controversy was raised, because the marrow is highly vascularized and endothelial cells are abundantly present in close proximity of the endosteum.Citation61,81 Moreover, endothelial cells are enriched in the endosteal BM region compared to central BM.Citation61 The model is now further refined by the recent proposal of 2 functional different vascular niches: the cycling sinusoidal niche and the quiescent arteriolar niche.Citation63 Arteriolar endothelium is surrounded by Nestin-GFP-bright, chondrotoin sulfate proteoglycan 4 (CSPG4/NG2)-positive, α-smooth-muscle-actin-positive, leptin receptor (LepR)-negative pericytes and is innervated by symphatic nerves, whereas sinusoids were negative for these markers and positive for the perivascular marker LepR. Arteriolar pericytes are more quiescent then sinusoidal pericytes, moreover, quiescent HSCs were overrepresented in the proximity of arterioles, and HSCs in these regions were protected from cytostatics. Sinusoidal endothelium harbored cycling HSC. Upon G-CSF treatment HS(P)Cs are relocated away from the arteriolar niche.Citation63
This illustrates that the arteriolar vascular niche, residing close to the endosteum, contributes to the quiescence of HSCs, whereas a dynamic balance exists between arteriolar and sinusoidal HSCs.
Bone marrow niches: stromal subpopulations function as niche
Mesenchymal stromal cells can differentiate into osteoblasts, adipocytes and chondrocytes. It was demonstrated that murine Nestin+ stromal cells constitute an essential HSC niche component. Nestin+ stromal cells contain all the bone-marrow colony-forming-unit fibroblastic (stromal stem cell) activity from the flushed femur and were propagated as non-adherent ‘mesenspheres’ that can self-renew and expand in serial transplantations.Citation62 Moreover, a major subpopulation (75%) of human stromal cells that co-express platelet-derived-growth-factor-receptor (PDGFRα) and Integrin-αV (ITGAV/CD51) are Nestin+ cells, that form functional ‘mesenspheres’ and provide HSPC support.Citation82
Based on expression of the low-affinity-nerve-growth-factor-receptor (CD271) and the melanoma-cell-adhesion-molecule (CD146), our group recently identified 2 new human BM stromal subpopulations, that show enrichment for stromal stem cells, the colony-forming-unit fibroblast (CFU-F).Citation83 The main subset in adult BM was CD271+CD146−, whereas the CD271+CD146+ population was dominant in pediatric and fetal BM.Citation83 The CD271+CD146− fraction localized to the endosteal niches.Citation84 The endosteal niche harbors quiescent HSCs and the increase of the CD271+CD146− stromal cells with age corresponds to the increase in long-term HSCs in aged murine BM.Citation85,86 Interestingly, our preliminary data suggest that there is a difference in haematopoietic support between stromal subsets, and that the CD271+CD146− stromal fraction may give better long term HSC support (Maijenburg et al, manuscript in preparation).
BM niche cells maintain HSPCs by direct cell-cell contacts and synthesis of soluble factors such as CXCL12 and SCF, as mentioned above. The contribution to haematopoietic support of the CXCL12 and SCF synthesis by stromal stem cells and differentiated stromal cell populations is revealed by cell-type-specific conditional deletion of these factors.Citation60,87-89 CXCL12 synthesis in osteoblasts (Collagen 2.3+) was dispensable for HSC maintenance, however, it reduced the number of B-lymphoid progenitors.Citation87,88 These data suggest that the endosteal niche is accumulating the early lymphoid progenitors. The function of the osteogenic lineage was further revealed by conditional deletion of CXCL12 in osteoprogenitors (Osterix+) that show induced mobilization of HSPCs into blood and spleen.Citation87 Conditional deletion of CXCL12 in sinusoidal pericytes (LepR+) did not influence HSPC numbers in the BM, but also induced HSPC mobilization into blood and spleen.Citation87 Remarkably, the synthesis of SCF by these perivascular (LepR+) cells was essential for HSC maintenance.Citation60 Modest defects in HSC numbers and function were revealed upon endothelial specific (endothelial tyrosine kinase/Tie2+ cells) CXCL12 deletion.Citation87,88 Conditional deletion of CXCL12 in a broad range of (Peroxiredoxin1; PRX1+) stromal cells, including all Nestin+ cells and a fraction of the osteoprogenitors, showed a dramatic reduction in BM HSPCs and displayed recruitment of HSPCs into the spleen.Citation88 Remarkably, CXCL12 expression in Nestin+ pericytes, which include mesenchymal stem cells, is dispensable for HSC maintenance and retention.Citation87 Deletion of Nestin+ cells, however, induced HSPC mobilization into the spleen.Citation62 These data indicate that perivascular stromal (stem) cells, osteoprogenitors and endothelial cells contribute to HS(P)C maintenance and retention.
Bone marrow niches: neuronal regulation of the BM niche
There is accumulating evidence that sympathic nerve signaling is involved in HSPC maintenance in the BM, as reviewed by others.Citation90 Initial experiments were performed in mice with disrupted sympathic nerve conduction (ceramide galactosyltransferase-deficient mice). The egress of HSPCs upon G-CSF injection was completely prevented in these mice.Citation47 Norepinephrine seems the responsible factor, as administration of a β2-adrenergic agonist enhanced the mobilization of HSPCs upon G-CSF treatment in control mice. In addition, dopamine deficient mice retained normal CXCL12 levels and osteoblast numbers upon G-CSF treatment, while HSPC mobilization was impaired.Citation47 Together, these data suggest that G-CSF-induced HSPC mobilization depends on the activation of sympathic neuronal signals. G-CSF treatment activates sympathic signals, which reduce osteoblast numbers and mobilize HSPCs.Citation47
Remarkably, also monocytes and/or macrophages are involved in the BM niche network.Citation91-93 Deletion of BM macrophages reduced CXCL12 expression in Nestin+ pericytes and mobilized HSPCs into the blood.Citation91 Therefore, macrophages retain HSPCs in the BM. This physiological mobilization of HSPCs upon depletion of BM macrophages was reduced, but still present, in mice that were deficient in the sympathic β3-receptors.Citation91 This indicates that BM macrophages and sympathic signaling act via distinct pathways, and fulfill antagonistic functions. In addition, depletion of BM macrophages also enhanced G-CSF-induced HSPC mobilization.Citation91 Together, BM macrophages and sympathic signals balance both the physiologically and the G-CSF-induced HSPC egress.
Bone marrow niches: perfusion
Low oxygen levels are proposed to contribute to HSC maintenance in the endosteal niche. However, the vasculature penetrates the complete BM up to a distance corresponding to a few cell-diameters. This suggests that perfusion rates are not expected to be highly discriminative between BM anatomical sides.Citation81 However, perfusion of the BM vasculature with the small fluorescent molecule Hoechst, showed that cells that were less exposed to the perfused Hoechst harbour the most HSC capacity, indicating that long-term HSCs reside in less perfused niches.Citation94 Moreover, the anatomical locations of the less perfused niches are not necessarily at the endosteum. Recently, direct high-resolution measurement of oxygen tensions in the BM revealed that Nestin+ vessels, locating closer to the endosteum, contain higher oxygen tensions, compared to Nestin− vessels.Citation95 Similarly, both vascular and extravascular oxygen tensions are lower at sites near the central sinusoid (>40 um away from the bone surface) compared to sites within 20 um of the bone surface.Citation95 This suggests that vascular oxygen levels drop when the vessels penetrate deeper into the BM due to the high BM-cellularity and oxygen demand.
In addition, analyses of the hypoxic signaling molecules in HSPCs reveal their metabolic state. Upon knock-out of hypoxia-inducible factor-1alpha (HIF1A) in mice, HSPC cell cycle quiescence was completely lost.Citation96 At homeostatic conditions, HIF1A knockout mice show normal phenotypic HSPC numbers and haematopoietic compartments, but upon BM stress the HSPCs get exhausted.Citation96 In line with this, the HIF1A target Cripto is specifically expressed in immature HSPCs. Cripto is essential for HSPCs localization in the endosteal niche, and for maintenance of repopulating capacities of HSPCs in culture.Citation97,98 It remains unclear why HSPCs are found preferentially in close proximity of microvessels in the endosteal zone by imaging cytometry, while a hypoxic profile, including HIF1A expression, was confirmed.Citation81 Therefore, the hypoxic state of HSPCs might also be regulated by stem cell-specific mechanisms.Citation81,99,100 Low oxygen levels are necessary but not sufficient for HSPC quiescence.Citation101 In conclusion, HSPCs are characterized by a hypoxic state, but the mutual contributions of the microenvironment and the stem cell-specific mechanisms are to be elucidated.
Bone marrow niches: soluble factors
Soluble factors involved in HSPC mobilization and homing are discussed above. Various other niche-derived soluble factors regulate HSC maintenance. Angiopoietin and its receptor Tie2, highly produced by endothelial cells, are required for the maintenance of quiescent HSCs.Citation102 The glycoprotein osteopontin, highly expressed in the endosteal niche, was required to control HSC numbers.Citation103,104 Free calcium ions, released by osteoclastic activity in the endosteal niche, are required as well, since depletion of the calcium-sensing receptor reduced the number of HSCs in the BM.Citation105 HSPC maintenance is also regulated by SCF and TPO that are proposed to function in the vascular and the osteoblastic niche, respectively.Citation75,106,107 Furthermore, stromal derived transforming-growth-factor-β (TGF-β) is a strong inhibitor of HSPC proliferation. Active TGF-β specifically inhibits the proliferation of early multipotent HSPCs, whereas it enhances the proliferation of later progenitors.Citation108-112 TGF-β also skews progenitor lineage choice, as myeloid differentiation is stimulated by TGF-β, while lymphoid differentiation is inhibited.Citation22 In conclusion, a wide range of niche-derived chemokines and other soluble factors mediate the interaction between HSPCs and the BM niches.
Extracellular Matrix Molecules in the Bone Marrow
Extracellular matrix molecules: introduction
The BM extracellular matrix is produced by niche cells and interacts with matrix-associated molecules, including immobilized secreted proteins and membrane-proteins with large extracellular domains.Citation113,114 The most abundantly present ECM proteins in the BM are fibronectin, the collagens I-XI, laminin, tenascin, thrombospondin and elastin.Citation115-119 Important for ECM integrity are also the proteoglycans with their large glycosaminoglycan (GAG) sidechains, including hyaluronic acid, chondroitin sulfate, dermatan sulfate, heparan sulfate, keratan sulfate and heparin.Citation120 Other ECM (associated) molecules are the soluble or membrane bound glycoproteins of the sialomucin family, including CD34, glycosylation dependent cell adhesion molecule1 (GlyCAM1), mucosal vascular addressin CAM1 (MadCAM1), platelet-selectin glycoprotein ligand (PSGL1/CD162), sialophorin (SPN/CD43), CD45RA and CD164. Essential mediators of the cell-cell and cell-matrix interactions in the BM are integrins and selectins,Citation121-123 whereas membrane bound receptors of the immunoglobin superfamily, including intercellular adhesion molecule-1 (ICAM1; CD54), vascular cell adhesion molecule-1 (VCAM1; CD105) and CD166, also shape the bone marrow niche.Citation124
Matrix biophysical properties
Recently, the importance of mechanic features of the BM matrix in HSC fate decision has been highlighted.Citation125,126 Murine BM cultured on tropo-elastin-coated plates is enriched for HSCs, but this was not observed when the density of the tropoelastin-coating was increased by crosslinking.Citation127 This indicates that substrate biophysical properties regulate HSC fate, although it does not clarify whether this is a direct effect.
The direct impact of matrix density on HSPCs was analyzed by culturing HSCs on collagen-coated polyacrylamide (PA) substrates with variable stiffness. The viability, spreading and adhesion of HSCs were increased with increasing collagen concentrations on PA substrates, although colony forming capacities and in vivo repopulating capacities were not modulated.Citation125 Similarly, presentation of ECM molecules in nanostructure patterns with variable interligand spacing modulated HSPC adhesion, although colony forming capacities were not affected.Citation128
These studies illustrate that matrix density and the presentation of specific adhesive ligands contribute to the HSPC niche in the BM. The evaluation of semisolid hydrogels and other artificial BM niches with or without the addition of cellular and molecular factors will reveal insight in HSPC niche requirements.Citation129-135
Proteoglycans, collagens and fibronectin
Proteoglycans are essential for maintenance of long-term culture-initiating cells in in vitro cultures, via interaction with IL3 and other growth factors.Citation136 An abundant proteoglycan in the BM is perlecan (heparan sulfate proteoglycan; HSPG).Citation137 Perlecan interacts with other ECM molecules, thereby stabilizing for example Collagen-X that is formed by hypertrophic chondrocytes at the chondro-osseus junction of the spongeous bone. Mutations in perlecan or collagen-X indirectly attenuate hematopoiesis via disruption of the endochondral ossification processes, resulting in marrow aplasia and lymphopenia.Citation67,138 In addition, loss of function mutations in the proteoglycan glypican-3 perturbed hematopoiesis.Citation139 Heparan sulfate modification of proteoglycans is also an essential element in the BM, critical for the establishment of the HSPC niche.Citation67,140,141 In addition, hyaluronic acid, the non-sulfated proteoglycan, is required for in vitro hematopoiesis and enhances hematopoiesis in the bone marrow after 5-fluorouracil (5-FU) treatment.Citation142 Together, this illustrates that proteoglycans and collagens are essential for HSPC maintenance.
In addition, specific inhibition of collagen secretion in the BM (by non-toxic levels of Cis-4-hydroxyproline) reduces hematopoiesis and the number of haematopoietic progenitors.Citation143 Direct adhesion of HSPCs to fibronectin attenuates HSPC proliferation and differentiation, which is mediated by integrins.Citation144 In addition, the abundant ECM protein tenascin-C supports the regeneration of haematopoietic cells after myeloablation.Citation145 Therefore, adhesion of HSPCs to fibronectin and other ECM molecules in the BM, contributes to the BM niche and regulates HSPC maintenance.
Extracellular matrix and integrins
HSPCs can interact with other cells and matrix molecules via the transmembrane glycoprotein family of integrins. Integrins are heterodimeric proteins, composed of an α- and a β-subunit, that are selected from 16 α-subunits and 8 β-subunits. HSPCs express Integrin-α4β1 (VLA4; ITGA4 and ITGB1), -α5β1 (VLA5; ITGA5 and ITGB1), -αLβ2 (LFA1; ITGAL and ITGB2) and -αMβ2 (MAC-1; ITGAM and ITGB2) and 10% of the BM HSPCs expresses Integrin-β3 (ITGB3).Citation146-148 Recently, human LT-HSCs are specifically identified by expression of ITGA6Citation16,17 (see paragraph 1.4).
Integrin-ligand binding induces “outside-in” signaling, that regulate cell differentiation.Citation149 On the other hand, cellular processes regulate integrin-dependent adhesion through “inside-out” signaling, including clustering of integrins and conformation changes of integrins, which increases the affinity for their ligands (integrin activation).Citation150
Blocking of β2-integrins inhibits adhesion of HSPCs to bone-marrow stroma in vitro, without inducing mobilization of stem cells into the blood in vivo.Citation151 Antibodies against VLA4, however, do induce mobilization.Citation152,153 Treatment of human HSPCs with antibodies against ITGB1 also prevented engraftment of HSPCs in mice and sheep.Citation154,155 ITGB1 associates with the potassium ion channel upon HSPC activation, which is essential for engraftment in the BM nicheCitation156-158 and also is suggested to sustain leukemic cells in the BM-niche.Citation159
In conclusion, integrin signaling is essential for a broad range of HSPC functions, including the reconstitution of the BM-niche and HSPC differentiation.
Extracellular matrix and selectins
Another protein family that plays a crucial role in cell-cell and cell-matrix interactions in the specific BM microenvironments is the selectin family. Selectins are transmembrane proteins and the family members endothelial (E-), leucocyte (L-) and platelet (P-) selectin share closely related extracellular domains, whereas their transmembrane- and intracellular domains are not conserved. Selectins bind sialylated, fucosylated or sulfated carbohydrate moieties on glycoproteins and proteoglycans. E-selectin ligands expressed in HSPCs include the CD34 antigen, PSGL-1, the HSPC-specific modified CD44 antigen HCELL (haematopoietic cell E-/L-selectin ligand), E-selectin ligand-1 (ESL-1; GLG1) and glycosphingolipids.Citation61,160 Other E-selectin ligands include CD43, endoglycan (a CD34-family member) and Basigin (CD147).Citation161
E-selectin is exclusively expressed on endothelial cells, with high expression in small sinusoids of the BM.Citation162,163 E-selectin mRNA expression was enriched in the endosteal region of the BM compared to central BM. Accordingly, E-selectin-expressing endothelial cells were enriched in the endosteal BM region compared to central BM. It is specifically co-expressed with CXCL12 in BM sinusoids and form targets of HSPC homing after transplantations.Citation162
E-selectin mediates adhesion of HSPCs to BM endothelial cellsCitation164 and promotes HSC proliferation and maturation.Citation61 Knock-down of E-selectin or treatment of mice with an E-selectin antagonists enhanced HSC quiescence and self-renewal, which was partially mediated by interaction of PSGL-1 and HCELL on HSPCs with endothelial E-selectin.Citation61 This indicates that the vasculature of the endosteal BM region actively induce HSC proliferation and maturation.Citation61 Thus, in addition to the endosteal arteriolar niche that supports HSPC quiescence,Citation60 an sinusoidal niche exists that activates HSPCs and is characterized by E-selectin expression.
P-selectin, however, is diffusely expressed throughout the BM vasculature and also expressed in endothelial cells of large blood vessels.Citation162 L-selectin is expressed on leucocytes and mediates adhesion and migration of HSPCs on lymphatic endothelial cells.Citation165 Both P- and L-Selectin bind ECM molecules, including the chondroitin-sulfated versicanCitation166 and the heparin-sulfated collagen-18 and collagen-15.Citation167 Thus, selectins mediate cell-cell and cell-matrix interactions, and E-selectin specifies microdomains in the sinusoidal BM niche that contributes to activation and proliferation of HSPCs.
Extracellular matrix and homeostatic factors
HSPC homeostasis is also regulated by structural blood plasma proteins that are responsible for fibrinolysis during the dissolution of thrombotic clots. Fibrin clots are dissolved by plasmin, an enzyme that is activated when its zymogen, plasminogen (PLG), is cleaved by urokinase PLG activator (uPA) or tissue PLG activator (tPA). The uPA protein is the main ligand for the uPA receptor (uPAR) that is GPI-anchored at the surface of HSPCs, thereby converting cell surface PLG into active Plasmin. PLG and uPAR are both required for G-CSF-induced HSPC mobilization, because the G-CSF-induced mobilization was impaired in PLG- or uPAR knock-out mice.Citation168,169 Conversely, genetic loss of plasmin inhibitor increased HSPC mobilization.Citation170 Plasmin stimulates the HSPC mobilization by its proteolytic activity toward collagen, fibrin, laminin and fibronectin. In addition, it activates other proteases, as matrix metalloproteases (MMP3/9/12/13), elastase and cathepsin B.Citation171,172 In conclusion, coagulation factors are essential regulators of HSPC mobilization and retention through processing of extracellular matrix proteins.
Adhesion Molecules in Regenerative Bone Marrow
Molecular signatures of regenerative bone marrow: introduction
Comparative array studies of HSCs revealed many proteins that are involved in cell-cell and cell-matrix interactions and are differentially regulated during BM regeneration compared to steady state conditions.Citation10,11,173 In addition, many adhesion proteins were differentially regulated in HSCs during development.Citation174 For this review we focus on cell adhesion molecules that are differentially expressed during regeneration, in particular Biglycan,Citation10,11 Embigin,Citation11CD164,Citation10,11 CD166Citation10 and BIGH3.Citation10,175 Some targets upregulated in murine regenerative BM were also overrepresented among targets upregulated during murine HSC differentiation (short-term HSCs versus long-term HSCs) (). In addition, targets downregulated during regenerative BM conditions are overrepresented among targets downregulated during murine HSC development (Fetal-liver-HSCs vs. BM-HSCs). These trends imply a strong correlation between regulation of adhesion molecules in HSPCs during regeneration, differentiation and development. We compared the mRNA expression of CD48, CD164, CD166, BIGH3, Embigin and Biglycan in HSPCs derived from human BM, cord blood and mobilized peripheral blood (MPB) (; , right column). These proteins are differentially expressed during regeneration, and some of them are also differentially expressed during developmentCitation10,174 () and/or in HSPCs derived from adult BM compared to MPB-derived HSPCs. However, the direction of this correlation is not consistent. This implies that, during the process of BM regeneration and HSPC mobilization, distinct gene expression profiles are found in HS(P)Cs that persistently remain in the BM and HSPCs that are mobilized into the blood. These variations may represent HSPC populations originating from distinct BM niches.
Table 1. Differential expression of adhesion molecules in HSPCs during BM regeneration10,175, HSPC differentiation11 and development10,174 The analyzed mRNA targets are in the first column. The second column depicts the change in mRNA in murine BM HSPCs after 5-fluorouracil (5FU) treatment, G-CSF treatment or combined G-CSF and cyclophosphamide (Cy) treatment.Citation5,10,17 The second column depicts how the mRNA expression in short-term HSPCs relates to that in long-term HSPCs in the murine BM.Citation11 The third column represents the difference in mRNA expression between HSPCs isolated from the fetal-liver or the fetal-BM.Citation4,10,17 The last column indicates the differential mRNA expression of adhesion molecules as determined on different sources of human CD34+ cells as depicted in . Arrows in green boxes mean increased mRNA expression, arrows in red boxes mean decreased mRNA expression. NS = not significant
Figure 1. Relative mRNA expression of adhesion molecules in HSPCs from BM, cord blood (CB) and mobilized peripheral blood (MPB). CD34+ cells were isolated using a MACS cell separation kit from Miltenyi Biotec. Cells were lysed and mRNA was isolated using a Qiagen mRNA isolation kit. c-DNA was prepared and the mRNA was quantified using a StepOnePlus Taqman machine. The mRNA expression was calculated relative to the GUS mRNA expression in each sample, by use of the 2−(ΔCt) value. Subsequently, the expression of each gene was depicted relative to its expression in BM HSPCs. Statistics were performed using a Student T-test. n ≥ 5 for MPB; n ≥ 4 for BM; n ≥ 5 for CB; bars represent mean ± SEM. * means P > 0.05.
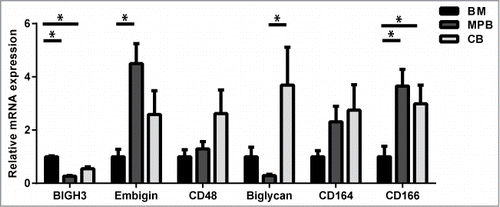
BIGH3 and its paralogue periostin in regenerative bone marrow
Analysis of murine BM-derived HSPCs in homeostatic and regenerative conditions identified the extracellular matrix protein (TGF-Beta Induced Gene-H3) BIGH3, also named TGF-β induced gene (TGFBI), being one of the top most upregulated proteins one day after G-CSF, cyclophosphamide or combined G-CSF and cyclophosphamide treatment.Citation175 Similarly, BIGH3 is upregulated in murine BM HSPCs after 5FU treatment.Citation10 BIGH3 expression is also enhanced during fetal development, showing increased expression in murine fetal BM HSPCs compared to fetal liver HSPCs.Citation174 This suggests a role for BIGH3 in HSPC-niche interactions during homeostasis and regeneration of the BM HSPC population.
In addition, BIGH3 is the top-one most differentially expressed gene comparing BM-derived mesenchymal stromal cells to HSPCs,Citation176 indicating the abundant production of (soluble) BIGH3 in the stromal niche. Similarly, BIGH3 is higher expressed in CB-derived stromal cells compared to CB-derived total mononuclear cells.Citation177 BIGH3 is involved in osteogenic differentiation, being upregulated in the first 3 d of stromal to osteogenic differentiation,Citation178 while being downregulated in osteoblast terminal differentiation.Citation178-180
BIGH3 is a secreted protein detected in various human tissues.Citation181,182 The 68-kDa protein consists of 4 fasciclin 1 domains and a C-terminal arginyl-glycyl-aspartic acid (RGD) sequence, both of which can mediate the interaction of BIGH3 with integrins.Citation183 Whereas the role of BIGH3 in cell-ECM interactions is extensively studied,Citation183 less is known about its role in the haematopoietic BM microenvironment.
HSPCs increase the BIGH3 expression upon adherance to mesenchymal stromal cells compared to non-adhered or fibronectin-adhered HSPCs.Citation184 We recently showed that CD34+ HSPCs adhere to BIGH3 in an integrin-dependent manner.Citation185 Expression of BIGH3 in HSPCs inhibited their adhesion and CXCL12-induced migration capacities. The balance between the small GTPases ras-related-C3-botulinum-toxin-substrate-1 (RAC1) and Ras-homolog-A (RHOA) was affected upon BIGH3 expression, as indicated by inhibition of the phorbol-myristate-acetate (PMA)-induced activation of RAC1.Citation185 Therefore, we propose that BIGH3 secretion may prepare the HSPCs for BM egress, as BIGH3 shifts the RAC1/RHOA balance and modulates HSPC adhesion to its niche, via blockage of integrins and other adhesion molecules.
BIGH3 was originally identified as a TGF-β responsive gene in lung adenocarcinoma cells.Citation181 TGF-β itself is a potent inhibitor of the growth of primitive haematopoietic stem cellsCitation186-188 and skews HSCs toward a myelocytic HSC subtype.Citation22 This raises the question whether BIGH3 has similar effects on hematopoiesis. We showed that BIGH3 is strongly upregulated in HSPCs in response to TGF-β (Klamer et al, manuscript submitted for publication). Reduced BIGH3 levels in HSPCs enhanced the ability of mesenchymal stromal cells to maintain HSCs, which may be explained by reduced cell cycle progression and proliferation upon reduced BIGH3 levels. Therefore, regulation of the expression of BIGH3 in HSPCs modulates their fate (Klamer et al, manuscript submitted for publication).
Considering the role of coagulation factors in HSPC mobilization and niche-interactions, it is of interest that BIGH3 is cleaved by plasminCitation189 and contains a vitamin-K-dependent gamma-carboxylation site, only prevalent in proteins that are involved in coagulation pathways.Citation190 Moreover, BIGH3 stimulates thrombus formation by activation of platelets, suggesting that it contributes to stabilization of blood clots, whereas its proteolysis by plasmin may contribute to the resolution of clots and to HSPC homeostasis.Citation191
Remarkably, transgenic mice that abundantly express mutant BIGH3 (containing the R555W mutation) under control of an ubiquitous promoter, show lymphoid follicular hyperplasia of the spleen and increased spleen size,Citation192 which may indicate deregulated hematopoiesis and/or lymphopoiesis by aberrant BIGH3 expression.
The ECM protein Periostin is the only paralogue of BIGH3 within the human genome and their encoding sequences are 48% homolog. Periostin is expressed in tissue that stands biomechanical forces and it enhances collagen cross-linking.Citation193 However, its expression in HSPCs is not described to change upon BM regeneration.Citation10,173 Nonetheless, Periostin modifies the BM microenvironment, by enhanced bone formation at the periosteum.Citation194,195 In addition, Periostin is highly expressed in the BM fibroblast cell line OP9, that supports hematopoiesis in the presence of TPO, whereas it is not expressed in fibroblasts that fail to support hematopoiesis, suggesting a role for Periostin in HSPC maintenance and expansion.Citation196 On the other hand, BM expression levels of Periostin correlate with the grade of BM fibrosis in myelofibrotic pathologies and moderate levels of Periostin were proposed to support the clonal expansion of haematopoietic cells in myelofibrosis by interaction with integrins and remodeling of the BM ECM.Citation197 Interestingly, recent studies reveal that Periostin enhances axon outgrowth of embryonic derived glial cells after spinal tract injury.Citation198 Therefore, Periostin may be important in HSPC-niche interactions by remodeling of the periosteum and the BM ECM and possible by nerve development, shaping both the neuronal and osteoblastic BM niche.
In conclusion, BIGH3 is a relatively novel ECM factor in the BM niche and its expression in HSPCs may contribute to BM homeostasis by altering HSPC-niche interactions and the differentiation status of HSPCs (as depicted in ).
Figure 2. Model of the endosteal, arteriolar and vascular bone marrow niches. The arteriolar niche localizes close to the endosteum and harbours mostly quiescent HSCs, whereas the sinusoidal niches are distributed throughout the BM and harbour mostly HSPCs that are in cell cycle. During haematopoietic regeneration after myeloablation, HSPCs proliferate and are mostly localized in sinusoidal niches. A range of ECM and matrix-associated molecules are differentially expressed upon BM regeneration. BIGH3 (purple dots) expression in HSPCs is upregulated in regenerative BM, suggesting its expression is dominant in sinusoidal HSPCs. BIGH3 expression is higher in BM-derived HSPCs compared to MPB-derived HSPCs, indicating that its expression is down-regulated upon transmigration into the blood. Adapted by permission from Macmillan Publishers, Ltd, http://www.nature.com/nri/posters, 2007 volume 7 no. 6 (T. Graf and A. Trumpp)
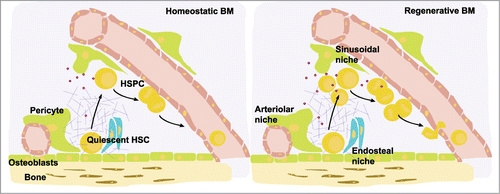
Embigin, CD164, CD166 and biglycan in regenerative bone marrow
One of the other genes upregulated in HSPCs during BM regeneration encodes Embigin or gp70.Citation10 Embigin was originally isolated from teratocarcinoma cells and is a transmembrane glycoprotein member of the immunoglobulin superfamily.Citation199 Embigin enhances integrin-dependent cell-substrate adhesion.Citation199,200 Embigin is required for proper plasma membrane expression of monocarboxylate transporters (MT1-4) that exchange lactate for protons during anaerobic metabolic activity.Citation201 Basigin fulfills similar functions, but shows higher affinity for MT1 and MT4, while Embigin is associated with MT2.Citation202 These complexes provide neurons with lactate as energy source and prevent acidification of the intracellular milieu.Citation203 Embigin mRNA was highly upregulated in denerved muscle cells compared to innervated musclesCitation204 and is involved in neuromuscular synapse formation. In haematopoietic cells, Embigin is highly expressed in HPSCs, upregulated in activated T-cells, and specifically repressed upon B-cells maturation.Citation205 It is upregulated in short-term HSPCs compared to long-term HSCs,Citation11 and we found it higher expressed in MPB- compared to BM-derived HSPCs (). The upregulation of Embigin in activated HSPCs and T-cells and its neuronal function hint toward a role of Embigin in the neuronal regulation of hematopoiesis.
CD164, CD166 and Biglycan are down-regulated upon BM regeneration. CD164, also named Endolyn, is a member of the sialomucin family. It is expressed on human CD34+ haematopoietic progenitors and BM stromal and endothelial cells.Citation206 Early HSPCs also express CD164, indicated by co-expression of early haematopoietic markers: prominin-1+ (CD133+), CD38−, CD117+, CD90+ and (FLT3; CD135+).Citation207,208 CD164 mediates HSPC adhesion to stromal and endothelial cells via homotypic interactions and inhibits HSPC proliferation.Citation206 CD164 is absent in most mature blood cells, although the sialyl-independent epitopes are present in monocytes and weakly present in granulocytes.Citation207 CD164 is required for CXCR4-mediated HSPC migration and takes part in the membrane migration complex together with CXCR4 and integrins.Citation209 Moreover, in various tumors upregulation of CD164 is associated with bone metastasis.Citation210,211 Remarkably, the expression of CD164 is increased in HSPCs from patients with idiopathic myelofibrosis, a condition that is characterized by BM fibrosis and mobilization of HSPCs into the peripheral blood.Citation212 Thus, CD164 is an adhesion molecule on early and more differentiated HSPCs as well as BM niche cells, and modulates HSPC adhesion, migration and differentiation.
Activated leukocyte cell adhesion molecule (ALCAM), also named CD166, is a transmembrane glycoprotein of the immunoglobulin superfamily and was originally isolated from CD34+ BM HSPCs.Citation213 CD166 mediates homotypic cell-cell adhesion and binds the T-cell antigen CD6.Citation214,215 Immature osteoblasts express CD166 and CD166-negative osteoblasts fail to support HSPCs.Citation216 CD166 knockout mice show normal hematopoiesis upon birth, but their HSCs show reduced long-term function.Citation217 CD166-deficient adult mice show decreased blood leukocyte numbers and increased phenotypic long-term HSCs. Upon transplantation, however, these HSCs display myeloid bias and express age-associated genes, indicating enhanced aging of the haematopoietic system in the absence of CD166.Citation217 CD166 expression is required for effective (bone) metastasis of various tumors, and its expression is enhanced upon active TGF-β release.Citation218,219 In various tumors, cancer stem cells can be identified by high membrane expression of CD166.Citation220,221 These studies show that CD166 regulates self-renewal in HSCs and tumor cells, and its expression in the endosteal niche and HSPCs is essential for proper hematopoiesis.
Biglycan is downregulated upon BM injury and is higher expressed in LT-HSCs compared to ST-HSCs. Biglycan is a small proteoglycan and binds other ECM molecules, such as collagen, fibronectin, Decorin, fibromodulin and BIGH3.Citation222 Furthermore, Biglycan binds TGF-β, thereby blocking its activity.Citation223 Murine knock-down of Biglycan resulted in decreased bone massCitation224 and a reduced production of BM stromal cells and osteoprogenitors.Citation224 Biglycan induces nitric oxide synthases, thereby protecting cells from hypoxia and reoxygination injury.Citation225 Biglycan expression correlates with the malignancy of colorectal and gastric cancer.Citation226,227 Biglycan is high expressed in tumor endothelial cells and is required for endothelial cell migration.Citation228,229 Biglycan, like Embigin, is also involved in the formation of the neuromuscular synapse.Citation230 Thus, Biglycan is a major regulator of stromal, osteogenic and possibly neuronal BM niche cells, and thereby facilitates hematopoiesis, reflected by its higher expression in LT-HSCs.Citation11
In conclusion, differential expression of various ECM- and adhesion molecules in HSPCs upon BM regeneration is an integral process within the complex regulatory network that controls hematopoiesis by HSPC-niche interactions.
Conclusions
The decision of HSPCs to sustain the HS(P)C population in the BM-niche or to differentiate and proliferate, depends on a complex network of HSPC-niche interactions and cellular signaling pathways. The HSPC niche consist of an endosteal, arterial, sinusoidal, mesenchymal and neuronal component and a broad range of soluble factors, ECM- and adhesion molecules modulate the HSPC fate during homeostasis and BM regeneration. A range of ECM and adhesion molecules is differentially regulated upon BM regeneration and includes novel factors, like BIGH3, that modulate HSPC-niche interactions.
Further analysis of the described ECM and adhesion molecules would reveal their exact role in the bone marrow niche. Importantly, manipulation of their expression in HSPCs, the ex vivo microenvironment or the recipient microenvironment would improve the generation of cellular products. Procedures to either induce HSC self-renewal (relevant for cord blood transplantation) or enhanced HSC differentiation/proliferation (generation of mature haematopoietic cells) might benefit. Thus, better understanding of the functions of extracellular matrix and adhesion might lead to improvement of current cellular therapies and efficient development of future cellular products.
Disclosure of Potential Conflicts of Interest
No potential conflicts of interest were disclosed.
Acknowledgments
We faithfully thank CE van der Schoot and M von Lindern for critical reading of the manuscript.
Funding
SK is supported by PPO-C (grant 06-005). CV is supported by the Landsteiner Foundation for Blood Transfusion Research (LSBR Fellowship, #1101F).
References
- Weissman IL, Anderson DJ, Gage F. Stem and progenitor cells: origins, phenotypes, lineage commitments, and transdifferentiations. Annu Rev Cell Dev Biol 2001; 17:387-403; PMID:11687494; http://dx.doi.org/10.1146/annurev.cellbio.17.1.387
- Brown G, Hughes PJ, Michell RH, Rolink AG, Ceredig R. The sequential determination model of hematopoiesis. Trends Immunol 2007; 28:442-8; PMID:17825625; http://dx.doi.org/10.1016/j.it.2007.07.007
- Ceredig R, Rolink AG, Brown G. Models of haematopoiesis: seeing the wood for the trees. Nat Rev Immunol 2009; 9:293-300; http://dx.doi.org/10.1038/nri2525
- Kawamoto H, Katsura Y. A new paradigm for hematopoietic cell lineages: revision of the classical concept of the myeloid-lymphoid dichotomy. Trends Immunol 2009; 30:193-200; PMID:19356980; http://dx.doi.org/10.1016/j.it.2009.03.001
- Pazianos G, Uqoezwa M, Reya T. The elements of stem cell self-renewal: a genetic perspective. Biotechniques 2003; 35:1240-7; PMID:14682059
- van der Wath RC, Wilson A, Laurenti E, Trumpp A, Lio P. Estimating dormant and active hematopoietic stem cell kinetics through extensive modeling of bromodeoxyuridine label-retaining cell dynamics. PLoS One 2009; 4:e6972; PMID:19771180; http://dx.doi.org/10.1371/journal.pone.0006972
- Wilson A, Laurenti E, Oser G, van der Wath RC, Blanco-Bose W, Jaworski M, Offner S, Dunant CF, Eshkind L, Bockamp E, et al. Hematopoietic stem cells reversibly switch from dormancy to self-renewal during homeostasis and repair. Cell 2008; 135:1118-29; PMID:19062086; http://dx.doi.org/10.1016/j.cell.2008.10.048
- Seita J, Weissman IL. Hematopoietic stem cell: self-renewal versus differentiation. Wiley Interdiscip Rev Syst Biol Med 2010; 2:640-53; PMID:20890962; http://dx.doi.org/10.1002/wsbm.86
- Schofield R. The relationship between the spleen colony-forming cell and the haemopoietic stem cell. Blood Cells 1978; 4:7-25; PMID:747780
- Venezia TA, Merchant AA, Ramos CA, Whitehouse NL, Young AS, Shaw CA, Goodell MA. Molecular signatures of proliferation and quiescence in hematopoietic stem cells. PLoS Biol 2004; 2:e301; PMID:15459755; http://dx.doi.org/10.1371/journal.pbio.0020301
- Forsberg EC, Prohaska SS, Katzman S, Heffner GC, Stuart JM, Weissman IL. Differential expression of novel potential regulators in hematopoietic stem cells. PLoS Genet 2005; 1:e28; PMID:16151515; http://dx.doi.org/10.1371/journal.pgen.0010028
- Schroeder T. Hematopoietic stem cell heterogeneity: subtypes, not unpredictable behavior. Cell Stem Cell 2010; 6:203-7; PMID:20207223; http://dx.doi.org/10.1016/j.stem.2010.02.006
- van Hennik PB, de Koning AE, Ploemacher RE. Seeding efficiency of primitive human hematopoietic cells in nonobese diabeticsevere combined immune deficiency mice: implications for stem cell frequency assessment. Blood 1999; 94:3055-61; PMID:10556189
- Kondo M, Wagers AJ, Manz MG, Prohaska SS, Scherer DC, Beilhack GF, Shizuru JA, Weissman IL. Biology of hematopoietic stem cells and progenitors: implications for clinical application. Annu Rev Immunol 2003; 21:759-806; PMID:12615892; http://dx.doi.org/10.1146/annurev.immunol.21.120601.141007
- Majeti R, Park CY, Weissman IL. Identification of a hierarchy of multipotent hematopoietic progenitors in human cord blood. Cell Stem Cell 2007; 1:635-45; PMID:18371405; http://dx.doi.org/10.1016/j.stem.2007.10.001
- Notta F, Doulatov S, Laurenti E, Poeppl A, Jurisica I, Dick JE. Isolation of single human hematopoietic stem cells capable of long-term multilineage engraftment. Science 2011; 333:218-21; PMID:21737740; http://dx.doi.org/10.1126/science.1201219
- Challen GA, Goodell MA. Bridge over troubled stem cells. Mol Ther 2011; 19:1756-8; PMID:21964307; http://dx.doi.org/10.1038/mt.2011.184
- Kimura T, Wang J, Matsui K, Imai S, Yokoyama S, Nishikawa M, Ikehara S, Sonoda Y. Proliferative and migratory potentials of human cord blood-derived. Int J Hematol 2004; 79:328-33; PMID:15218959; http://dx.doi.org/10.1532/IJH97.03158
- Kimura T, Asada R, Wang J, Kimura T, Morioka M, Matsui K, Kobayashi K, Henmi K, Imai S, Kita M, et al. Identification of long-term repopulating potential of human cord blood-derived CD34-flt3- severe combined immunodeficiency-repopulating cells by intra-bone marrow injection. Stem Cells 2007; 25:1348-55; PMID:17303816; http://dx.doi.org/10.1634/stemcells.2006-0727
- Christensen JL, Weissman IL. Flk-2 is a marker in hematopoietic stem cell differentiation: a simple method to isolate long-term stem cells. Proc Natl Acad Sci U S A 2001; 98:14541-6; PMID:11724967; http://dx.doi.org/10.1073/pnas.261562798
- Kiel MJ, Yilmaz OH, Iwashita T, Yilmaz OH, Terhorst C, Morrison SJ. SLAM family receptors distinguish hematopoietic stem and progenitor cells and reveal endothelial niches for stem cells. Cell 2005; 121:1109-21; PMID:15989959; http://dx.doi.org/10.1016/j.cell.2005.05.026
- Challen GA, Boles NC, Chambers SM, Goodell MA. Distinct hematopoietic stem cell subtypes are differentially regulated by TGF-beta1. Cell Stem Cell 2010; 6:265-78; PMID:20207229; http://dx.doi.org/10.1016/j.stem.2010.02.002
- Benveniste P, Frelin C, Janmohamed S, Barbara M, Herrington R, Hyam D, Iscove NN. Intermediate-term hematopoietic stem cells with extended but time-limited reconstitution potential. Cell Stem Cell 2010; 6:48-58; PMID:20074534; http://dx.doi.org/10.1016/j.stem.2009.11.014
- Kent DG, Copley MR, Benz C, Wöhrer S, Dykstra BJ, Ma E, Cheyne J, Zhao Y, Bowie MB, Zhao Y, et al. Prospective isolation and molecular characterization of hematopoietic stem cells with durable self-renewal potential. Blood 2009; 113:6342-50; PMID:19377048; http://dx.doi.org/10.1182/blood-2008-12-192054
- Rieger MA, Hoppe PS, Smejkal BM, Eitelhuber AC, Schroeder T. Hematopoietic cytokines can instruct lineage choice. Science 2009; 325:217-8; PMID:19590005; http://dx.doi.org/10.1126/science.1171461
- Muller-Sieburg CE, Cho RH, Karlsson L, Huang JF, Sieburg HB. Myeloid-biased hematopoietic stem cells have extensive self-renewal capacity but generate diminished lymphoid progeny with impaired IL-7 responsiveness. Blood 2004; 103:4111-8; PMID:14976059; http://dx.doi.org/10.1182/blood-2003-10-3448
- Breems DA, Blokland EA, Siebel KE, Mayen AE, Engels LJ, Ploemacher RE. Stroma-contact prevents loss of hematopoietic stem cell quality during ex vivo expansion of CD34+ mobilized peripheral blood stem cells. Blood 1998; 91:111-7; PMID:9414274
- Zhang CC, Lodish HF. Insulin-like growth factor 2 expressed in a novel fetal liver cell population is a growth factor for hematopoietic stem cells. Blood 2004; 103:2513-21; PMID:14592820; http://dx.doi.org/10.1182/blood-2003-08-2955
- Sauvageau G, Iscove NN, Humphries RK. In vitro and in vivo expansion of hematopoietic stem cells. Oncogene 2004; 23:7223-32; PMID:15378082; http://dx.doi.org/10.1038/sj.onc.1207942
- Austin TW, Solar GP, Ziegler FC, Liem L, Matthews W. A role for the Wnt gene family in hematopoiesis: expansion of multilineage progenitor cells. Blood 1997; 89:3624-35; PMID:9160667
- Willert K, Brown JD, Danenberg E, Duncan AW, Weissman IL, Reya T, Yates JR 3rd, Nusse R. Wnt proteins are lipid-modified and can act as stem cell growth factors. Nature 2003; 423:448-52; PMID:12717451; http://dx.doi.org/10.1038/nature01611
- Murdoch B, Chadwick K, Martin M, Shojaei F, Shah KV, Gallacher L, Moon RT, Bhatia M. Wnt-5A augments repopulating capacity and primitive hematopoietic development of human blood stem cells in vivo. Proc Natl Acad Sci U S A 2003; 100:3422-7; PMID:12626754; http://dx.doi.org/10.1073/pnas.0130233100
- Van Den Berg DJ, Sharma AK, Bruno E, Hoffman R. Role of members of the Wnt gene family in human hematopoiesis. Blood 1998; 92:3189-202; PMID:9787155
- Varnum-Finney B, Brashem-Stein C, Bernstein ID. Combined effects of Notch signaling and cytokines induce a multiple log increase in precursors with lymphoid and myeloid reconstituting ability. Blood 2003; 101:1784-9; PMID:12411302; http://dx.doi.org/10.1182/blood-2002-06-1862
- Delaney C, Varnum-Finney B, Aoyama K, Brashem-Stein C, Bernstein ID. Dose-dependent effects of the Notch ligand Delta1 on ex vivo differentiation and in vivo marrow repopulating ability of cord blood cells. Blood 2005; 106:2693-9; PMID:15976178; http://dx.doi.org/10.1182/blood-2005-03-1131
- Himburg HA, Muramoto GG, Daher P, Meadows SK, Russell JL, Doan P, Chi JT, Salter AB, Lento WE, Reya T, et al. Pleiotrophin regulates the expansion and regeneration of hematopoietic stem cells. Nat Med 2010; 16:475-82; PMID:20305662; http://dx.doi.org/10.1038/nm.2119
- Peled A, Petit I, Kollet O, Magid M, Ponomaryov T, Byk T, Nagler A, Ben-Hur H, Many A, Shultz L, et al. Dependence of human stem cell engraftment and repopulation of NODSCID mice on CXCR4. Science 1999; 283:845-8; PMID:9933168; http://dx.doi.org/10.1126/science.283.5403.845
- Walasek MA, van OR, de HG. Hematopoietic stem cell expansion: challenges and opportunities. Ann N Y Acad Sci 2012; 1266:138-50; PMID:22901265; http://dx.doi.org/10.1111/j.1749-6632.2012.06549.x
- Kaplan RN, Psaila B, Lyden D. Niche-to-niche migration of bone-marrow-derived cells. Trends Mol Med 2007; 13:72-81; PMID:17197241; http://dx.doi.org/10.1016/j.molmed.2006.12.003
- Balabanian K, Lagane B, Pablos JL, Laurent L, Planchenault T, Verola O, Lebbe C, Kerob D, Dupuy A, Hermine O, et al. WHIM syndromes with different genetic anomalies are accounted for by impaired CXCR4 desensitization to CXCL12. Blood 2005; 105:2449-57; PMID:15536153; http://dx.doi.org/10.1182/blood-2004-06-2289
- Bleul CC, Fuhlbrigge RC, Casasnovas JM, Aiuti A, Springer TA. A highly efficacious lymphocyte chemoattractant, stromal cell-derived factor 1 (SDF-1). J Exp Med 1996; 184:1101-9; PMID:9064327; http://dx.doi.org/10.1084/jem.184.3.1101
- Zou YR, Kottmann AH, Kuroda M, Taniuchi I, Littman DR. Function of the chemokine receptor CXCR4 in haematopoiesis and in cerebellar development. Nature 1998; 393:595-9; PMID:9634238; http://dx.doi.org/10.1038/31269
- Ma Q, Jones D, Borghesani PR, Segal RA, Nagasawa T, Kishimoto T, Bronson RT, Springer TA. Impaired B-lymphopoiesis, myelopoiesis, and derailed cerebellar neuron migration in C. Proc Natl Acad Sci U S A 1998; 95:9448-53; PMID:9689100; http://dx.doi.org/10.1073/pnas.95.16.9448
- Ara T, Itoi M, Kawabata K, Egawa T, Tokoyoda K, Sugiyama T, Fujii N, Amagai T, Nagasawa T. A role of CXC chemokine ligand 12stromal cell-derived factor-1pre-B cell growth stimulating factor and its receptor CXCR4 in fetal and adult T cell development in vivo. J Immunol 2003; 170:4649-55; PMID:12707343; http://dx.doi.org/10.4049/jimmunol.170.9.4649
- Petit I, Szyper-Kravitz M, Nagler A, Lahav M, Peled A, Habler L, Ponomaryov T, Taichman RS, Arenzana-Seisdedos F, Fujii N, et al. G-CSF induces stem cell mobilization by decreasing bone marrow SDF-1 and up-regulating CXCR4. Nat Immunol 2002; 3:687-94; PMID:12068293; http://dx.doi.org/10.1038/ni813
- Lapidot T, Petit I. Current understanding of stem cell mobilization: the roles of chemokines, proteolytic enzymes, adhesion molecules, cytokines, and stromal cells. Exp Hematol 2002; 30:973-81; PMID:12225788; http://dx.doi.org/10.1016/S0301-472X(02)00883-4
- Katayama Y, Battista M, Kao WM, Hidalgo A, Peired AJ, Thomas SA, Frenette PS. Signals from the sympathetic nervous system regulate hematopoietic stem cell egress from bone marrow. Cell 2006; 124:407-21; PMID:16439213; http://dx.doi.org/10.1016/j.cell.2005.10.041
- Asada N, Katayama Y, Sato M, Minagawa K, Wakahashi K, Kawano H, Kawano Y, Sada A, Ikeda K, Matsui T, et al. Matrix-embedded osteocytes regulate mobilization of hematopoietic stemprogenitor cells. Cell Stem Cell 2013; 12:737-47; PMID:23746979; http://dx.doi.org/10.1016/j.stem.2013.05.001
- Kawamori Y, Katayama Y, Asada N, Minagawa K, Sato M, Okamura A, Shimoyama M, Nakagawa K, Okano T, Tanimoto M, et al. Role for vitamin D receptor in the neuronal control of the hematopoietic stem cell niche. Blood 2010; 116:5528-35; PMID:20813899; http://dx.doi.org/10.1182/blood-2010-04-279216
- Levesque JP, Hendy J, Takamatsu Y, Williams B, Winkler IG, Simmons PJ. Mobilization by either cyclophosphamide or granulocyte colony-stimulating factor transforms the bone marrow into a highly proteolytic environment. Exp Hematol 2002; 30:440-49; PMID:12031650; http://dx.doi.org/10.1016/S0301-472X(02)00788-9
- Li S, Zhai Q, Zou D, Meng H, Xie Z, Li C, Wang Y, Qi J, Cheng T, Qiu L. A pivotal role of bone remodeling in granulocyte colony stimulating factor induced hematopoietic stemprogenitor cells mobilization. J Cell Physiol 2013; 228:1002-9; PMID:23042582; http://dx.doi.org/10.1002/jcp.24246
- Pelus LM, Fukuda S. Peripheral blood stem cell mobilization: the CXCR2 ligand GRObeta rapidly mobilizes hematopoietic stem cells with enhanced engraftment properties. Exp Hematol 2006; 34:1010-20; PMID:16863907; http://dx.doi.org/10.1016/j.exphem.2006.04.004
- Ratajczak MZ, Kim C, Wu W, Shin DM, Bryndza E, Kucia M, Ratajczak J. The role of innate immunity in trafficking of hematopoietic stem cells-an emerging link between activation of complement cascade and chemotactic gradients of bioactive sphingolipids. Adv Exp Med Biol 2012; 946:37-54; PMID:21948361; http://dx.doi.org/10.1007/978-1-4614-0106-3_3
- Ratajczak MZ, Lee H, Wysoczynski M, Wan W, Marlicz W, Laughlin MJ, Kucia M, Janowska-Wieczorek A, Ratajczak J. Novel insight into stem cell mobilization-plasma sphingosine-1-phosphate is a major chemoattractant that directs the egress of hematopoietic stem progenitor cells from the bone marrow and its level in peripheral blood increases during mobilization due to activation of complement cascademembrane attack complex. Leukemia 2010; 24:976-85; PMID:20357827; http://dx.doi.org/10.1038/leu.2010.53
- Golan K, Kollet O, Lapidot T. Dynamic Cross Talk between S1P and CXCL12 Regulates Hematopoietic Stem Cells Migration, Development and Bone Remodeling. Pharmaceuticals (Basel) 2013; 6:1145-69; PMID:24276423; http://dx.doi.org/10.3390/ph6091145
- Marquez-Curtis LA, Turner AR, Sridharan S, Ratajczak MZ, Janowska-Wieczorek A. The ins and outs of hematopoietic stem cells: studies to improve transplantation outcomes. Stem Cell Rev 2011; 7:590-607; PMID:21140298; http://dx.doi.org/10.1007/s12015-010-9212-8
- Geutskens SB, Andrews WD, van Stalborch AM, Brussen K, Holtrop-de Haan SE, Parnavelas JG, Hordijk PL, van Hennik PB. Control of human hematopoietic stemprogenitor cell migration by the extracellular matrix protein Slit3. Lab Invest 2012; 92:1129-39; PMID:22614124; http://dx.doi.org/10.1038/labinvest.2012.81
- Calvi LM, Adams GB, Weibrecht KW, Weber JM, Olson DP, Knight MC, Martin RP, Schipani E, Divieti P, Bringhurst FR, et al. Osteoblastic cells regulate the haematopoietic stem cell niche. Nature 2003; 425:841-6; PMID:14574413; http://dx.doi.org/10.1038/nature02040
- Zhang J, Niu C, Ye L, Huang H, He X, Tong WG, Ross J, Haug J, Johnson T, Feng JQ, et al. Identification of the haematopoietic stem cell niche and control of the niche size. Nature 2003; 425:836-41; PMID:14574412; http://dx.doi.org/10.1038/nature02041
- Ding L, Saunders TL, Enikolopov G, Morrison SJ. Endothelial and perivascular cells maintain haematopoietic stem cells. Nature 2012; 481:457-62; PMID:22281595; http://dx.doi.org/10.1038/nature10783
- Winkler IG, Barbier V, Nowlan B, Jacobsen RN, Forristal CE, Patton JT, Magnani JL, Lévesque JP. Vascular niche E-selectin regulates hematopoietic stem cell dormancy, self renewal and chemoresistance. Nat Med 2012; 18:1651-7; PMID:23086476; http://dx.doi.org/10.1038/nm.2969
- Mendez-Ferrer S, Michurina TV, Ferraro F, Mazloom AR, Macarthur BD, Lira SA, Scadden DT, Ma'ayan A, Enikolopov GN, Frenette PS. Mesenchymal and haematopoietic stem cells form a unique bone marrow niche. Nature 2010; 466:829-34; PMID:20703299; http://dx.doi.org/10.1038/nature09262
- Kunisaki Y, Bruns I, Scheiermann C, Ahmed J, Pinho S, Zhang D, Mizoguchi T, Wei Q, Lucas D, Ito K, et al. Arteriolar niches maintain haematopoietic stem cell quiescence. Nature 2013; 502:637-43; PMID:24107994; http://dx.doi.org/10.1038/nature12612
- Wilson A, Oser GM, Jaworski M, Blanco-Bose WE, Laurenti E, Adolphe C, Essers MA, Macdonald HR, Trumpp A. Dormant and self-renewing hematopoietic stem cells and their niches. Ann NY Acad Sci 2007; 1106:64-75; PMID:17442778; http://dx.doi.org/10.1196/annals.1392.021
- Nilsson SK, Dooner MS, Tiarks CY, Weier HU, Quesenberry PJ. Potential and distribution of transplanted hematopoietic stem cells in a nonablated mouse model. Blood 1997; 89:4013-20; PMID:9166840
- Nilsson SK, Johnston HM, Coverdale JA. Spatial localization of transplanted hemopoietic stem cells: inferences for the localization of stem cell niches. Blood 2001; 97:2293-9; PMID:11290590; http://dx.doi.org/10.1182/blood.V97.8.2293
- Rodgers KD, San Antonio JD, Jacenko O. Heparan sulfate proteoglycans: a GAGgle of skeletal-hematopoietic regulators. Dev Dyn 2008; 237:2622-42; PMID:18629873; http://dx.doi.org/10.1002/dvdy.21593
- Adams GB, Scadden DT. The hematopoietic stem cell in its place. Nat Immunol 2006; 7:333-7; PMID:16550195; http://dx.doi.org/10.1038/ni1331
- Taichman RS, Reilly MJ, Emerson SG. Human osteoblasts support human hematopoietic progenitor cells in vitro bone marrow cultures. Blood 1996; 87:518-24; PMID:8555473
- Adams GB. Deconstructing the hematopoietic stem cell niche: revealing the therapeutic potential. Regen Med 2008; 3:523-30; PMID:18588474; http://dx.doi.org/10.2217/17460751.3.4.523
- Visnjic D, Kalajzic Z, Rowe DW, Katavic V, Lorenzo J, Aguila HL. Hematopoiesis is severely altered in mice with an induced osteoblast deficiency. Blood 2004; 103:3258-64; PMID:14726388; http://dx.doi.org/10.1182/blood-2003-11-4011
- Lymperi S, Horwood N, Marley S, Gordon MY, Cope AP, Dazzi F. Strontium can increase some osteoblasts without increasing hematopoietic stem cells. Blood 2008; 111:1173-81; PMID:17971481; http://dx.doi.org/10.1182/blood-2007-03-082800
- de Barros AP, Takiya CM, Garzoni LR, Leal-Ferreira ML, Dutra HS, Chiarini LB, Meirelles MN, Borojevic R, Rossi MI. Osteoblasts and bone marrow mesenchymal stromal cells control hematopoietic stem cell migration and proliferation in 3D in vitro model. PLoS One 2010; 5:e9093; PMID:20161704; http://dx.doi.org/10.1371/journal.pone.0009093
- Kiel MJ, Radice GL, Morrison SJ. Lack of evidence that hematopoietic stem cells depend on N-cadherin-mediated adhesion to osteoblasts for their maintenance. Cell Stem Cell 2007; 1:204-17; PMID:18371351; http://dx.doi.org/10.1016/j.stem.2007.06.001
- Yoshihara H, Arai F, Hosokawa K, Hagiwara T, Takubo K, Nakamura Y, Gomei Y, Iwasaki H, Matsuoka S, Miyamoto K, et al. ThrombopoietinMPL signaling regulates hematopoietic stem cell quiescence and interaction with the osteoblastic niche. Cell Stem Cell 2007; 1:685-97; PMID:18371409; http://dx.doi.org/10.1016/j.stem.2007.10.020
- Christopher MJ, Link DC. Granulocyte colony-stimulating factor induces osteoblast apoptosis and inhibits osteoblast differentiation. J Bone Miner Res 2008; 23:1765-74; PMID:18597629; http://dx.doi.org/10.1359/jbmr.080612
- Semerad CL, Christopher MJ, Liu F, Short B, Simmons PJ, Winkler I, Levesque JP, Chappel J, Ross FP, Link DC. G-CSF potently inhibits osteoblast activity and CXCL12 mRNA expression in the bone marrow. Blood 2005; 106:3020-7; PMID:16037394; http://dx.doi.org/10.1182/blood-2004-01-0272
- Itkin T, Ludin A, Gradus B, Gur-Cohen S, Kalinkovich A, Schajnovitz A, Ovadya Y, Kollet O, Canaani J, Shezen E, et al. FGF-2 expands murine hematopoietic stem and progenitor cells via proliferation of stromal cells, c-Kit activation, and CXCL12 down-regulation. Blood 2012; 120:1843-55; PMID:22645180; http://dx.doi.org/10.1182/blood-2011-11-394692
- Ema H, Suda T. Two anatomically distinct niches regulate stem cell activity. Blood 2012; 120:2174-81; PMID:22786878; http://dx.doi.org/10.1182/blood-2012-04-424507
- Nakamura-Ishizu A, Suda T. Hematopoietic stem cell niche: an interplay among a repertoire of multiple functional niches. Biochim Biophys Acta 2013; 1830:2404-9; PMID:22967757; http://dx.doi.org/10.1016/j.bbagen.2012.08.023
- Nombela-Arrieta C, Pivarnik G, Winkel B, Canty KJ, Harley B, Mahoney JE, Park SY, Lu J, Protopopov A, Silberstein LE. Quantitative imaging of haematopoietic stem and progenitor cell localization and hypoxic status in the bone marrow microenvironment. Nat Cell Biol 2013; 15:533-43; PMID:23624405; http://dx.doi.org/10.1038/ncb2730
- Pinho S, Lacombe J, Hanoun M, Mizoguchi T, Bruns I, Kunisaki Y, Frenette PS. PDGFRalpha and CD51 mark human nestin +sphere-forming mesenchymal stem cells capable of hematopoietic progenitor cell expansion. J Exp Med 2013; 210:1351-67; PMID:23776077; http://dx.doi.org/10.1084/jem.20122252
- Maijenburg MW, Kleijer M, Vermeul K, Mul EP, van Alphen FP, van der Schoot CE, Voermans C. The composition of the mesenchymal stromal cell compartment in human bone marrow changes during development and aging. Haematologica 2012; 97:179-83; PMID:21993672; http://dx.doi.org/10.3324/haematol.2011.047753
- Tormin A, Li O, Brune JC, Walsh S, Schütz B, Ehinger M, Ditzel N, Kassem M, Scheding S. CD146 expression on primary nonhematopoietic bone marrow stem cells is correlated with in situ localization. Blood 2011; 117:5067-77; PMID:21415267; http://dx.doi.org/10.1182/blood-2010-08-304287
- Morrison SJ, Wandycz AM, Akashi K, Globerson A, Weissman IL. The aging of hematopoietic stem cells. Nat Med 1996; 2:1011-6; PMID:8782459; http://dx.doi.org/10.1038/nm0996-1011
- Rossi DJ, Bryder D, Seita J, Nussenzweig A, Hoeijmakers J, Weissman IL. Deficiencies in DNA damage repair limit the function of haematopoietic stem cells with age. Nature 2007; 447:725-9; PMID:17554309; http://dx.doi.org/10.1038/nature05862
- Ding L, Morrison SJ. Haematopoietic stem cells and early lymphoid progenitors occupy distinct bone marrow niches. Nature 2013; 495:231-5; PMID:23434755; http://dx.doi.org/10.1038/nature11885
- Greenbaum A, Hsu YM, Day RB, Schuettpelz LG, Christopher MJ, Borgerding JN, Nagasawa T, Link DC. CXCL12 in early mesenchymal progenitors is required for haematopoietic stem-cell maintenance. Nature 2013; 495:227-30; PMID:23434756; http://dx.doi.org/10.1038/nature11926
- Hanoun M, Frenette PS. This niche is a maze; an amazing niche. Cell Stem Cell 2013; 12:391-2; PMID:23561440; http://dx.doi.org/10.1016/j.stem.2013.03.012
- del Toro R, Mendez-Ferrer S. Autonomic regulation of hematopoiesis and cancer. Haematologica 2013; 98:1663-6; PMID:24186311; http://dx.doi.org/10.3324/haematol.2013.084764
- Chow A, Lucas D, Hidalgo A, Méndez-Ferrer S, Hashimoto D, Scheiermann C, Battista M, Leboeuf M, Prophete C, van Rooijen N, et al. Bone marrow CD169+ macrophages promote the retention of hematopoietic stem and progenitor cells in the mesenchymal stem cell niche. J Exp Med 2011; 208:261-71; PMID:21282381; http://dx.doi.org/10.1084/jem.20101688
- Christopher MJ, Rao M, Liu F, Woloszynek JR, Link DC. Expression of the G-CSF receptor in monocytic cells is sufficient to mediate hematopoietic progenitor mobilization by G-CSF in mice. J Exp Med 2011; 208:251-60; PMID:21282380; http://dx.doi.org/10.1084/jem.20101700
- Winkler IG, Sims NA, Pettit AR, Barbier V, Nowlan B, Helwani F, Poulton IJ, van Rooijen N, Alexander KA, Raggatt LJ, et al. Bone marrow macrophages maintain hematopoietic stem cell (HSC) niches and their depletion mobilizes HSCs. Blood 2010; 116:4815-28; PMID:20713966; http://dx.doi.org/10.1182/blood-2009-11-253534
- Winkler IG, Barbier V, Wadley R, Zannettino AC, Williams S, Lévesque JP. Positioning of bone marrow hematopoietic and stromal cells relative to blood flow in vivo: serially reconstituting hematopoietic stem cells reside in distinct nonperfused niches. Blood 2010; 116:375-85; PMID:20393133; http://dx.doi.org/10.1182/blood-2009-07-233437
- Spencer JA, Ferraro F, Roussakis E, Klein A, Wu J, Runnels JM, Zaher W, Mortensen LJ, Alt C, Turcotte R, et al. Direct measurement of local oxygen concentration in the bone marrow of live animals. Nature 2014; 508:269-73; PMID:24590072; http://dx.doi.org/10.1038/nature13034
- Takubo K, Goda N, Yamada W et al. Regulation of the HIF-1alpha level is essential for hematopoietic stem cells. Cell Stem Cell 2010; 7:391-402; PMID:20804974; http://dx.doi.org/10.1016/j.stem.2010.06.020
- Miharada K, Karlsson G, Rehn M, Rörby E, Siva K, Cammenga J, Karlsson S. Hematopoietic stem cells are regulated by Cripto, as an intermediary of HIF-1alpha in the hypoxic bone marrow niche. Ann N Y Acad Sci 2012; 1266:55-62; PMID:22901256; http://dx.doi.org/10.1111/j.1749-6632.2012.06564.x
- Miharada K, Karlsson G, Rehn M, Rörby E, Siva K, Cammenga J, Karlsson S. Cripto regulates hematopoietic stem cells as a hypoxic-niche-related factor through cell surface receptor GRP78. Cell Stem Cell 2011; 9:330-44; PMID:21982233; http://dx.doi.org/10.1016/j.stem.2011.07.016
- Simsek T, Kocabas F, Zheng J, Deberardinis RJ, Mahmoud AI, Olson EN, Schneider JW, Zhang CC, Sadek HA. The distinct metabolic profile of hematopoietic stem cells reflects their location in a hypoxic niche. Cell Stem Cell 2010; 7:380-90; PMID:20804973; http://dx.doi.org/10.1016/j.stem.2010.07.011
- Suda T, Takubo K, Semenza GL. Metabolic regulation of hematopoietic stem cells in the hypoxic niche. Cell Stem Cell 2011; 9:298-310; PMID:21982230; http://dx.doi.org/10.1016/j.stem.2011.09.010
- Guitart AV, Hammoud M, Dello SP, Ivanovic Z, Praloran V. Slow-cyclingquiescence balance of hematopoietic stem cells is related to physiological gradient of oxygen. Exp Hematol 2010; 38:847-51; PMID:20547202; http://dx.doi.org/10.1016/j.exphem.2010.06.002
- Arai F, Hirao A, Ohmura M, Sato H, Matsuoka S, Takubo K, Ito K, Koh GY, Suda T. Tie2angiopoietin-1 signaling regulates hematopoietic stem cell quiescence in the bone marrow niche. Cell 2004; 118:149-61; PMID:15260986; http://dx.doi.org/10.1016/j.cell.2004.07.004
- Stier S, Ko Y, Forkert R, Neuhaus T, Grünewald E, Cheng T, Dombkowski D, Calvi LM, Rittling SR, Scadden DT. Osteopontin is a hematopoietic stem cell niche component that negatively regulates stem cell pool size. J Exp Med 2005; 201:1781-91; PMID:15928197; http://dx.doi.org/10.1084/jem.20041992
- Nilsson SK, Johnston HM, Whitty GA, Williams B, Webb RJ, Denhardt DT, Bertoncello I, Bendall LJ, Simmons PJ, Haylock DN. Osteopontin, a key component of the hematopoietic stem cell niche and regulator of primitive hematopoietic progenitor cells. Blood 2005; 106:1232-9; PMID:15845900; http://dx.doi.org/10.1182/blood-2004-11-4422
- Adams GB, Chabner KT, Alley IR, Olson DP, Szczepiorkowski ZM, Poznansky MC, Kos CH, Pollak MR, Brown EM, Scadden DT. Stem cell engraftment at the endosteal niche is specified by the calcium-sensing receptor. Nature 2006; 439:599-603; PMID:16382241; http://dx.doi.org/10.1038/nature04247
- Barker JE. SlSld hematopoietic progenitors are deficient in situ. Exp Hematol 1994; 22:174-7; PMID:7507859
- Qian H, Buza-Vidas N, Hyland CD, Jensen CT, Antonchuk J, Månsson R, Thoren LA, Ekblom M, Alexander WS, Jacobsen SE. Critical role of thrombopoietin in maintaining adult quiescent hematopoietic stem cells. Cell Stem Cell 2007; 1:671-84; PMID:18371408; http://dx.doi.org/10.1016/j.stem.2007.10.008
- Ottmann OG, Pelus LM. Differential proliferative effects of transforming growth factor-beta on human hematopoietic progenitor cells. J Immunol 1988; 140:2661-5; PMID:3258618
- Van Ranst PC, Snoeck HW, Lardon F, Lenjou M, Nijs G, Weekx SF, Rodrigus I, Berneman ZN, Van Bockstaele DR. TGF-beta and MIP-1 alpha exert their main inhibitory activity on very primitive CD34+2. Exp.Hematol. 1996; 24:1509-15; PMID:8950234
- Fortunel NO, Hatzfeld A, Hatzfeld JA. Transforming growth factor-beta: pleiotropic role in the regulation of hematopoiesis. Blood 2000; 96:2022-36; PMID:10979943
- Veiby OP, Jacobsen FW, Cui L, Lyman SD, Jacobsen SE. The flt3 ligand promotes the survival of primitive hemopoietic progenitor cells with myeloid as well as B lymphoid potential. Suppression of apoptosis and counteraction by TNF-alpha and TGF-beta. J Immunol 1996; 157:2953-60; PMID:8816402
- Batard P, Monier MN, Fortunel N, Ducos K, Sansilvestri-Morel P, Phan T, Hatzfeld A, Hatzfeld JA. TGF-(beta)1 maintains hematopoietic immaturity by a reversible negative control of cell cycle and induces CD34 antigen up-modulation. J Cell Sci 2000; 113 (Pt 3):383-90; PMID:10639326
- Cromar GL, Xiong X, Chautard E, Ricard-Blum S, Parkinson J. Toward a systems level view of the ECM and related proteins: a framework for the systematic definition and analysis of biological systems. Proteins 2012; 80:1522-44; PMID:22275077; http://dx.doi.org/10.1002/prot.24036
- Hynes RO, Naba A. Overview of the matrisome–an inventory of extracellular matrix constituents and functions. Cold Spring Harb Perspect Biol 2012; 4:a004903; PMID:21937732; http://dx.doi.org/10.1101/cshperspect.a004903
- Klein G. The extracellular matrix of the hematopoietic microenvironment. Experientia 1995; 51:914-26; PMID:7556572; http://dx.doi.org/10.1007/BF01921741
- Carter DH, Sloan P, Aaron JE. Immunolocalization of collagen types I and III, tenascin, and fibronectin in intramembranous bone. J Histochem Cytochem 1991; 39:599-606; PMID:1707904; http://dx.doi.org/10.1177/39.5.1707904
- Hamilton R, Campbell FR. Immunochemical localization of extracellular materials in bone marrow of rats. Anat Rec 1991; 231:218-24; PMID:1746722; http://dx.doi.org/10.1002/ar.1092310210
- Nilsson SK, Debatis ME, Dooner MS, Madri JA, Quesenberry PJ, Becker PS. Immunofluorescence characterization of key extracellular matrix proteins in murine bone marrow in situ. J Histochem Cytochem 1998; 46:371-7; PMID:9487119; http://dx.doi.org/10.1177/002215549804600311
- Savagner P, Karavanova I, Perantoni A, Thiery JP, Yamada KM. Slug mRNA is expressed by specific mesodermal derivatives during rodent organogenesis. Dev Dyn 1998; 213:182-7; PMID:9786418; http://dx.doi.org/10.1002/(SICI)1097-0177(199810)213 :2%3c182::AID-AJA3%3e3.0.CO;2-C
- Rodgers KD, San Antonio JD, Jacenko O. Heparan sulfate proteoglycans: a GAGgle of skeletal-hematopoietic regulators. Dev Dyn 2008; 237:2622-42; PMID:18629873; http://dx.doi.org/10.1002/dvdy.21593
- Prosper F, Verfaillie CM. Regulation of hematopoiesis through adhesion receptors. J Leukoc Biol 2001; 69:307-16; PMID:11261776
- Sahin AO, Buitenhuis M. Molecular mechanisms underlying adhesion and migration of hematopoietic stem cells. Cell Adh Migr 2012; 6:39-48; PMID:22647939; http://dx.doi.org/10.4161/cam.18975
- Pillozzi S, Becchetti A. Ion channels in hematopoietic and mesenchymal stem cells. Stem Cells Int 2012; 2012:217910; PMID:22919401; http://dx.doi.org/10.1155/2012/217910
- Bevilacqua MP. Endothelial-leukocyte adhesion molecules. Annu Rev Immunol 1993; 11:767-804; PMID:8476577; http://dx.doi.org/10.1146/annurev.iy.11.040193.004003
- Choi JS, Harley BA. The combined influence of substrate elasticity and ligand density on the viability and biophysical properties of hematopoietic stem and progenitor cells. Biomaterials 2012; 33:4460-8; PMID:22444641; http://dx.doi.org/10.1016/j.biomaterials.2012.03.010
- Discher DE, Mooney DJ, Zandstra PW. Growth factors, matrices, and forces combine and control stem cells. Science 2009; 324:1673-7; PMID:19556500; http://dx.doi.org/10.1126/science.1171643
- Holst J, Watson S, Lord MS, Eamegdool SS, Bax DV, Nivison-Smith LB, Kondyurin A, Ma L, Oberhauser AF, Weiss AS, et al. Substrate elasticity provides mechanical signals for the expansion of hemopoietic stem and progenitor cells. Nat Biotechnol 2010; 28:1123-8; PMID:20890282; http://dx.doi.org/10.1038/nbt.1687
- Muth CA, Steinl C, Klein G, Lee-Thedieck C. Regulation of hematopoietic stem cell behavior by the nanostructured presentation of extracellular matrix components. PLoS One 2013; 8:e54778; PMID:23405094; http://dx.doi.org/10.1371/journal.pone.0054778
- Lutolf MP, Doyonnas R, Havenstrite K, Koleckar K, Blau HM. Perturbation of single hematopoietic stem cell fates in artificial niches. Integr Biol (Camb) 2009; 1:59-69; PMID:20023792; http://dx.doi.org/10.1039/b815718a
- Sharma MB, Limaye LS, Kale VP. Mimicking the functional hematopoietic stem cell niche in vitro: recapitulation of marrow physiology by hydrogel-based three-dimensional cultures of mesenchymal stromal cells. Haematologica 2012; 97:651-60; PMID:22058199; http://dx.doi.org/10.3324/haematol.2011.050500
- Raic A, Rodling L, Kalbacher H, Lee-Thedieck C. Biomimetic macroporous PEG hydrogels as 3D scaffolds for the multiplication of human hematopoietic stem and progenitor cells. Biomaterials 2014; 35:929-40; PMID:24176196; http://dx.doi.org/10.1016/j.biomaterials.2013.10.038
- Mahadik BP, Wheeler TD, Skertich LJ, Kenis PJ, Harley BA. Microfluidic generation of gradient hydrogels to modulate hematopoietic stem cell culture environment. Adv Healthc Mate. 2013; 3:449-58; PMID:23997020; http://dx.doi.org/10.1002/adhm.201300263
- Vu TT, Lim C, Lim M. Characterization of leukemic cell behaviors in a soft marrow mimetic alginate hydrogel. J Biomed Mater Res B Appl Biomater 2012; 100:1980-8; PMID:22888018; http://dx.doi.org/10.1002/jbm.b.32765
- Cuchiara ML, Horter KL, Banda OA, West JL. Covalent immobilization of stem cell factor and stromal derived factor 1alpha for in vitro culture of hematopoietic progenitor cells. Acta Biomater 2013; 9:9258-69; PMID:23958779; http://dx.doi.org/10.1016/j.actbio.2013.08.012
- Ferreira MV, Labude N, Piroth D, Jahnen-Dechent W, Knüchel R, Hieronymus T, Zenke M, Neuss S. Compatibility of different polymers for cord blood-derived hematopoietic progenitor cells. J Mater Sci Mater Med 2012; 23:109-16; PMID:22071984; http://dx.doi.org/10.1007/s10856-011-4483-4
- Gupta P, Oegema TR, Jr., Brazil JJ, Dudek AZ, Slungaard A, Verfaillie CM. Structurally specific heparan sulfates support primitive human hematopoiesis by formation of a multimolecular stem cell niche. Blood 1998; 92:4641-51; PMID:9845530
- Schofield KP, Gallagher JT, David G. Expression of proteoglycan core proteins in human bone marrow stroma. Biochem J 1999; 343 Pt 3:663-68; http://dx.doi.org/10.1042/0264-6021:3430663
- Sweeney E, Roberts D, Jacenko O. Altered matrix at the chondro-osseous junction leads to defects in lymphopoiesis. Ann N Y Acad Sci 2011; 1237:79-87; PMID:22082369; http://dx.doi.org/10.1111/j.1749-6632.2011.06227.x
- Viviano BL, Silverstein L, Pflederer C, Paine-Saunders S, Mills K, Saunders S. Altered hematopoiesis in glypican-3-deficient mice results in decreased osteoclast differentiation and a delay in endochondral ossification. Dev Biol 2005; 282:152-62; PMID:15936336; http://dx.doi.org/10.1016/j.ydbio.2005.03.003
- Di GF, Lewandowski D, Cabannes E, Nancy-Portebois V, Petitou M, Fichelson S, Romeo PH. Heparan sulfate mimetics can efficiently mobilize long-term hematopoietic stem cells. Haematologica 2012; 97:491-99; PMID:22180429; http://dx.doi.org/10.3324/haematol.2011.047662
- Mazzon C, Anselmo A, Cibella J, Soldani C, Destro A, Kim N, Roncalli M, Burden SJ, Dustin ML, Sarukhan A, et al. The critical role of agrin in the hematopoietic stem cell niche. Blood 2011; 118:2733-42; PMID:21653324; http://dx.doi.org/10.1182/blood-2011-01-331272
- Matrosova VY, Orlovskaya IA, Serobyan N, Khaldoyanidi SK. Hyaluronic acid facilitates the recovery of hematopoiesis following 5-fluorouracil administration. Stem Cells 2004; 22:544-55; PMID:15277700; http://dx.doi.org/10.1634/stemcells.22-4-544
- Zuckerman KS, Rhodes RK, Goodrum DD, Patel VR, Sparks B, Wells J, Wicha MS, Mayo LA. Inhibition of collagen deposition in the extracellular matrix prevents the establishment of a stroma supportive of hematopoiesis in long-term murine bone marrow cultures. J Clin Invest 1985; 75:970-5; PMID:3980732; http://dx.doi.org/10.1172/JCI111798
- Hurley RW, McCarthy JB, Verfaillie CM. Direct adhesion to bone marrow stroma via fibronectin receptors inhibits hematopoietic progenitor proliferation. J Clin Invest 1995; 96:511-9; PMID:7542285; http://dx.doi.org/10.1172/JCI118063
- Nakamura-Ishizu A, Okuno Y, Omatsu Y, Okabe K, Morimoto J, Uede T, Nagasawa T, Suda T, Kubota Y. Extracellular matrix protein tenascin-C is required in the bone marrow microenvironment primed for hematopoietic regeneration. Blood 2012; 119:5429-37; PMID:22553313; http://dx.doi.org/10.1182/blood-2011-11-393645
- Knapp W, Strobl H, Scheinecker C, Bello-Fernandez C, Majdic O. Molecular characterization of CD34+ human hematopoietic progenitor cells. Ann Hematol 1995; 70:281-96; PMID:7543291; http://dx.doi.org/10.1007/BF01696614
- Kerst JM, Sanders JB, Slaper-Cortenbach IC, Doorakkers MC, Hooibrink B, van Oers RH, von dem Borne AE, van der Schoot CE. Alpha 4 beta 1 and alpha 5 beta 1 are differentially expressed during myelopoiesis and mediate the adherence of human CD34+ cells to fibronectin in an activation-dependent way. Blood 1993; 81:344-51; PMID:7678511
- Coulombel L, Auffray I, Gaugler MH, Rosemblatt M. Expression and function of integrins on hematopoietic progenitor cells. Acta Haematol 1997; 97:13-21; PMID:8980606; http://dx.doi.org/10.1159/000203655
- Hemler ME, Kassner PD, Chan BM. Functional roles for integrin alpha subunit cytoplasmic domains. Cold Spring Harb Symp Quant Biol 1992; 57:213-20; PMID:1339660; http://dx.doi.org/10.1101/SQB.1992.057.01.026
- Diamond MS, Springer TA. The dynamic regulation of integrin adhesiveness. Curr Biol 1994; 4:506-17; PMID:7922371; http://dx.doi.org/10.1016/S0960-9822(00)00111-1
- Teixido J, Hemler ME, Greenberger JS, Anklesaria P. Role of beta 1 and beta 2 integrins in the adhesion of human CD34hi stem cells to bone marrow stroma. J Clin Invest 1992; 90:358-67; PMID:1379610; http://dx.doi.org/10.1172/JCI115870
- Papayannopoulou T, Nakamoto B. Peripheralization of hemopoietic progenitors in primates treated with anti-VLA4 integrin. Proc Natl Acad Sci U S A 1993; 90:9374-8; PMID:7692447; http://dx.doi.org/10.1073/pnas.90.20.9374
- Rettig MP, Ansstas G, DiPersio JF. Mobilization of hematopoietic stem and progenitor cells using inhibitors of CXCR4 and VLA-4. Leukemia 2012; 26:34-53; PMID:21886173; http://dx.doi.org/10.1038/leu.2011.197
- Mohle R, Murea S, Kirsch M, Haas R. Differential expression of L-selectin, VLA-4, and LFA-1 on CD34+ progenitor cells from bone marrow and peripheral blood during G-CSF-enhanced recovery. Exp Hematol 1995; 23:1535-42; PMID:8542944
- Zanjani ED, Flake AW, Almeida-Porada G, Tran N, Papayannopoulou T. Homing of human cells in the fetal sheep model: modulation by antibodies activating or inhibiting very late activation antigen-4-dependent function. Blood 1999; 94:2515-22; PMID:10498625
- Pillozzi S, Brizzi MF, Balzi M, Crociani O, Cherubini A, Guasti L, Bartolozzi B, Becchetti A, Wanke E, Bernabei PA, et al. HERG potassium channels are constitutively expressed in primary human acute myeloid leukemias and regulate cell proliferation of normal and leukemic hemopoietic progenitors. Leukemia 2002; 16:1791-8; PMID:12200695; http://dx.doi.org/10.1038/sj.leu.2402572
- Pillozzi S, Brizzi MF, Bernabei PA, Bartolozzi B, Caporale R, Basile V, Boddi V, Pegoraro L, Becchetti A, Arcangeli A. VEGFR-1 (FLT-1), beta1 integrin, and hERG K +channel for a macromolecular signaling complex in acute myeloid leukemia: role in cell migration and clinical outcome. Blood 2007; 110:1238-50; PMID:17420287; http://dx.doi.org/10.1182/blood-2006-02-003772
- Pillozzi S, Becchetti A. Ion channels in hematopoietic and mesenchymal stem cells. Stem Cells Int 2012; 2012:217910; PMID:22919401; http://dx.doi.org/10.1155/2012/217910
- Li H, Liu L, Guo L, Zhang J, Du W, Li X, Liu W, Chen X, Huang S. HERG K+ channel expression in CD34+CD38-CD123(high) cells and primary leukemia cells and analysis of its regulation in leukemia cells. Int J Hematol 2008; 87:387-92; PMID:18415658; http://dx.doi.org/10.1007/s12185-008-0056-9
- Dimitroff CJ, Lee JY, Schor KS, Sandmaier BM, Sackstein R. differential L-selectin binding activities of human hematopoietic cell L-selectin ligands, HCELL and PSGL-1. J Biol Chem 2001; 276:47623-31; http://dx.doi.org/10.1074/jbc.M105997200
- Zarbock A, Ley K, McEver RP, Hidalgo A. Leukocyte ligands for endothelial selectins: specialized glycoconjugates that mediate rolling and signaling under flow. Blood 2011; 118:6743-51; PMID:22021370; http://dx.doi.org/10.1182/blood-2011-07-343566
- Sipkins DA, Wei X, Wu JW, Runnels JM, Côté D, Means TK, Luster AD, Scadden DT, Lin CP. In vivo imaging of specialized bone marrow endothelial microdomains for tumour engraftment. Nature 2005; 435:969-73; PMID:15959517; http://dx.doi.org/10.1038/nature03703
- Schweitzer KM, Drager AM, van d VP, Thijsen SF, Zevenbergen A, Theijsmeijer AP, van der Schoot CE, Langenhuijsen MM. Constitutive expression of E-selectin and vascular cell adhesion molecule-1 on endothelial cells of hematopoietic tissues. Am J Pathol 1996; 148:165-75; PMID:8546203
- Rood PM, Gerritsen WR, Kramer D, Ranzijn C, von dem Borne AE, van der Schoot CE. Adhesion of hematopoietic progenitor cells to human bone marrow or umbilical vein derived endothelial cell lines: a comparison. Exp Hematol 1999; 27:1306-1314; PMID:10428507; http://dx.doi.org/10.1016/S0301-472X(99)00068-5
- Arbones ML, Ord DC, Ley K, Ratech H, Maynard-Curry C, Otten G, Capon DJ, Tedder TF. Lymphocyte homing and leukocyte rolling and migration are impaired in L-selectin-deficient mice. Immunity 1994; 1:247-60; PMID:7534203; http://dx.doi.org/10.1016/1074-7613(94)90076-0
- Kawashima H, Hirose M, Hirose J, Nagakubo D, Plaas AH, Miyasaka M. Binding of a large chondroitin sulfatedermatan sulfate proteoglycan, versican, to L-selectin, P-selectin, and CD44. J Biol Chem 2000; 275:35448-56; PMID:10950950; http://dx.doi.org/10.1074/jbc.M003387200
- Kawashima H, Atarashi K, Hirose M, Hirose J, Yamada S, Sugahara K, Miyasaka M. Oversulfated chondroitindermatan sulfates containing GlcAbeta1IdoAalpha1-3GalNAc(4,6-O-disulfate) interact with L- and P-selectin and chemokines. J Biol Chem 2002; 277:12921-30; PMID:11821431; http://dx.doi.org/10.1074/jbc.M200396200
- Tjwa M, Sidenius N, Moura R, Jansen S, Theunissen K, Andolfo A, De Mol M, Dewerchin M, Moons L, Blasi F, et al. Membrane-anchored uPAR regulates the proliferation, marrow pool size, engraftment, and mobilization of mouse hematopoietic stemprogenitor cells. J Clin Invest 2009; 119:1008-18; PMID:19273908
- Gong Y, Fan Y, Hoover-Plow J. Plasminogen regulates stromal cell-derived factor-1CXCR4-mediated hematopoietic stem cell mobilization by activation of matrix metalloproteinase-9. Arterioscler Thromb Vasc Biol 2011; 31:2035-43; PMID:21719761; http://dx.doi.org/10.1161/ATVBAHA.111.229583
- Tjwa M, Janssens S, Carmeliet P. Plasmin therapy enhances mobilization of HPCs after G-CSF. Blood 2008; 112:4048-50; PMID:18723692; http://dx.doi.org/10.1182/blood-2008-07-166587
- Gong Y, Hoover-Plow J. The plasminogen system in regulating stem cell mobilization. J Biomed Biotechnol 2012; 2012:437920; PMID:23118508; http://dx.doi.org/10.1155/2012/437920
- Plow EF, Herren T, Redlitz A, Miles LA, Hoover-Plow JL. The cell biology of the plasminogen system. FASEB J 1995; 9:939-45; PMID:7615163
- Bruno L, Hoffmann R, McBlane F, Brown J, Gupta R, Joshi C, Pearson S, Seidl T, Heyworth C, Enver T. Molecular signatures of self-renewal, differentiation, and lineage choice in multipotential hemopoietic progenitor cells in vitro. Mol Cell Biol 2004; 24:741-56; PMID:14701746; http://dx.doi.org/10.1128/MCB.24.2.741-756.2004
- Ciriza J, Garcia-Ojeda ME. Expression of migration-related genes is progressively upregulated in murine Lineage-Sca-1+c-Kit+ population from the fetal to adult stages of development. Stem Cell Res Ther 2010; 1:14; PMID:20637061; http://dx.doi.org/10.1186/scrt14
- Voermans C, Lento WE, Uqoezwa M, DiMascio LN, Chhotani A, Rattis FM, Reya T. Identification of novel regulators of hematopoietic stem cell mobilization. Blood (ASH Annu Meeting Abstr) 2005; 106:Abstract 1724
- Ren J, Jin P, Sabatino M, Balakumaran A, Feng J, Kuznetsov SA, Klein HG, Robey PG, Stroncek DF. Global transcriptome analysis of human bone marrow stromal cells (BMSC) reveals proliferative, mobile and interactive cells that produce abundant extracellular matrix proteins, some of which may affect BMSC potency. Cytotherapy 2011; 13:661-74; PMID:21250865; http://dx.doi.org/10.3109/14653249.2010.548379
- Jeong JA, Hong SH, Gang EJ, Ahn C, Hwang SH, Yang IH, Han H, Kim H. Differential gene expression profiling of human umbilical cord blood-derived mesenchymal stem cells by DNA microarray. Stem Cells 2005; 23:584-93; PMID:15790779; http://dx.doi.org/10.1634/stemcells.2004-0304
- Chiellini C, Cochet O, Negroni L, Samson M, Poggi M, Ailhaud G, Alessi MC, Dani C, Amri EZ. Characterization of human mesenchymal stem cell secretome at early steps of adipocyte and osteoblast differentiation. BMC Mol Biol 2008; 9:26; PMID:18302751
- Kim JE, Kim EH, Han EH, Park RW, Park IH, Jun SH, Kim JC, Young MF, Kim IS. A TGF-beta-inducible cell adhesion molecule, betaig-h3, is downregulated in melorheostosis and involved in osteogenesis. J Cell Biochem 2000; 77:169-78; PMID:10723084; http://dx.doi.org/10.1002/(SICI)1097-4644(20000501)77 :2%3c169::AID-JCB1%3e3.0.CO;2-L
- Thapa N, Kang KB, Kim IS. Beta ig-h3 mediates osteoblast adhesion and inhibits differentiation. Bone 2005; 36:232-42; PMID:15780949; http://dx.doi.org/10.1016/j.bone.2004.08.007
- Skonier J, Neubauer M, Madisen L, Bennett K, Plowman GD, Purchio AF. cDNA cloning and sequence analysis of beta ig-h3, a novel gene induced in a human adenocarcinoma cell line after treatment with transforming growth factor-beta. DNA Cell Biol 1992; 11:511-22; PMID:1388724; http://dx.doi.org/10.1089/dna.1992.11.511
- Zhao YL, Piao CQ, Hei TK. Downregulation of Betaig-h3 gene is causally linked to tumorigenic phenotype in asbestos treated immortalized human bronchial epithelial cells. Oncogene 2002; 21:7471-7; PMID:12386809; http://dx.doi.org/10.1038/sj.onc.1205891
- Billings PC, Whitbeck JC, Adams CS, Abrams WR, Cohen AJ, Engelsberg BN, Howard PS, Rosenbloom J. The transforming growth factor-beta-inducible matrix protein (beta)ig-h3 interacts with fibronectin. J Biol Chem 2002; 277:28003-9; PMID:12034705; http://dx.doi.org/10.1074/jbc.M106837200
- Wagner W, Wein F, Roderburg C, Saffrich R, Faber A, Krause U, Schubert M, Benes V, Eckstein V, Maul H, et al. Adhesion of hematopoietic progenitor cells to human mesenchymal stem cells as a model for cell-cell interaction. Exp Hematol 2007; 35:314-25; PMID:17258080; http://dx.doi.org/10.1016/j.exphem.2006.10.003
- Klamer SE, Kuijk CG, Hordijk PL, van der Schoot CE, von Lindern M, van Hennik PB, Voermans C. BIGH3 modulates adhesion and migration of hematopoietic stem and progenitor cells. Cell Adh Migr 2013; 7:434-49; PMID:24152593; http://dx.doi.org/10.4161/cam.26596
- Yamazaki S, Ema H, Karlsson G, Yamaguchi T, Miyoshi H, Shioda S, Taketo MM, Karlsson S, Iwama A, Nakauchi H. Nonmyelinating Schwann cells maintain hematopoietic stem cell hibernation in the bone marrow niche. Cell 2011; 147:1146-58; PMID:22118468; http://dx.doi.org/10.1016/j.cell.2011.09.053
- Ruscetti FW, Bartelmez SH. Transforming growth factor beta, pleiotropic regulator of hematopoietic stem cells: potential physiological and clinical relevance. Int J Hematol 2001; 74:18-25; PMID:11530800; http://dx.doi.org/10.1007/BF02982545
- Scandura JM, Boccuni P, Massague J, Nimer SD. Transforming growth factor beta-induced cell cycle arrest of human hematopoietic cells requires p57KIP2 up-regulation. Proc Natl Acad Sci U S A 2004; 101:15231-6; PMID:15477587; http://dx.doi.org/10.1073/pnas.0406771101
- Ween MP, Lokman NA, Hoffmann P, Rodgers RJ, Ricciardelli C, Oehler MK. Transforming growth factor-beta-induced protein secreted by peritoneal cells increases the metastatic potential of ovarian cancer cells. Int J Cancer 2011; 128:1570-84; PMID:20521251; http://dx.doi.org/10.1002/ijc.25494
- McCann JC, Ames BN. Vitamin K, an example of triage theory: is micronutrient inadequacy linked to diseases of aging? Am J Clin Nutr 2009; 90:889-907; PMID:19692494; http://dx.doi.org/10.3945/ajcn.2009.27930
- Kim HJ, Kim PK, Bae SM, Son HN, Thoudam DS, Kim JE, Lee BH, Park RW, Kim IS. Transforming growth factor-beta-induced protein (TGFBIpbeta ig-h3) activates platelets and promotes thrombogenesis. Blood 2009; 114:5206-15; PMID:19738031; http://dx.doi.org/10.1182/blood-2009-03-212415
- Bustamante M, Tasinato A, Maurer F, Elkochairi I, Lepore MG, Arsenijevic Y, Pedrazzini T, Munier FL, Schorderet DF. Overexpression of a mutant form of TGFBIBIGH3 induces retinal degeneration in transgenic mice. Mol Vis 2008; 14:1129-37; PMID:18568131
- Kudo A. Periostin in fibrillogenesis for tissue regeneration: periostin actions inside and outside the cell. Cell Mol Life Sci 2011; 68:3201-7; PMID:21833583; http://dx.doi.org/10.1007/s00018-011-0784-5
- Bonnet N, Standley KN, Bianchi EN, Stadelmann V, Foti M, Conway SJ, Ferrari SL. The matricellular protein periostin is required for sost inhibition and the anabolic response to mechanical loading and physical activity. J Biol Chem 2009; 284:35939-50; PMID:19837663; http://dx.doi.org/10.1074/jbc.M109.060335
- Rios H, Koushik SV, Wang H, Wang J, Zhou HM, Lindsley A, Rogers R, Chen Z, Maeda M, Kruzynska-Frejtag A, et al. periostin null mice exhibit dwarfism, incisor enamel defects, and an early-onset periodontal disease-like phenotype. Mol Cell Biol. 2005; 25:11131-44; PMID:16314533; http://dx.doi.org/10.1128/MCB.25.24.11131-11144.2005
- Lagergren A, Mansson R, Zetterblad J, Smith E, Basta B, Bryder D, Akerblad P, Sigvardsson M. The Cxcl12, periostin, and Ccl9 genes are direct targets for early B-cell factor in OP-9 stroma cells. J Biol Chem 2007; 282:14454-62; PMID:17374609; http://dx.doi.org/10.1074/jbc.M610263200
- Oku E, Kanaji T, Takata Y, Oshima K, Seki R, Morishige S, Imamura R, Ohtsubo K, Hashiguchi M, Osaki K, et al. Periostin and bone marrow fibrosis. Int J Hematol 2008; 88:57-63; PMID:18465194; http://dx.doi.org/10.1007/s12185-008-0095-2
- Shih CH, Lacagnina M, Leuer-Bisciotti K, Proschel C. Astroglial-derived periostin promotes axonal regeneration after spinal cord injury. J Neurosci 2014; 34:2438-43; PMID:24523534; http://dx.doi.org/10.1523/JNEUROSCI.2947-13.2014
- Ozawa M, Huang RP, Furukawa T, Muramatsu T. A teratocarcinoma glycoprotein carrying a developmentally regulated carbohydrate marker is a member of the immunoglobulin gene superfamily. J Biol Chem 1988; 263:3059-62; PMID:2963822
- Huang RP, Ozawa M, Kadomatsu K, Muramatsu T. Embigin, a member of the immunoglobulin superfamily expressed in embryonic cells, enhances cell-substratum adhesion. Dev Biol 1993; 155:307-14; PMID:8432389; http://dx.doi.org/10.1006/dbio.1993.1030
- Halestrap AP. Monocarboxylic Acid transport. Compr Physiol 2013; 3:1611-43; PMID:24265240; http://dx.doi.org/10.1002/cphy.c130008
- Wilson MC, Meredith D, Fox JE, Manoharan C, Davies AJ, Halestrap AP. Basigin (CD147) is the target for organomercurial inhibition of monocarboxylate transporter isoforms 1 and 4: the ancillary protein for the insensitive MCT2 is EMBIGIN (gp70). J Biol Chem 2005; 280:27213-21; PMID:15917240; http://dx.doi.org/10.1074/jbc.M411950200
- Wilson MC, Kraus M, Marzban H, Sarna JR, Wang Y, Hawkes R, Halestrap AP, Beesley PW. The neuroplastin adhesion molecules are accessory proteins that chaperone the monocarboxylate transporter MCT2 to the neuronal cell surface. PLoS One 2013; 8:e78654; PMID:24260123; http://dx.doi.org/10.1371/journal.pone.0078654
- Lain E, Carnejac S, Escher P, Wilson MC, Lømo T, Gajendran N, Brenner HR. A novel role for embigin to promote sprouting of motor nerve terminals at the neuromuscular junction. J Biol Chem 2009; 284:8930-39; PMID:19164284; http://dx.doi.org/10.1074/jbc.M809491200
- Pridans C, Holmes ML, Polli M, Wettenhall JM, Dakic A, Corcoran LM, Smyth GK, Nutt SL. Identification of Pax5 target genes in early B cell differentiation. J Immunol 2008; 180:1719-28; PMID:18209069; http://dx.doi.org/10.4049/jimmunol.180.3.1719
- Zannettino AC, Buhring HJ, Niutta S, Watt SM, Benton MA, Simmons PJ. The sialomucin CD164 (MGC-24v) is an adhesive glycoprotein expressed by human hematopoietic progenitors and bone marrow stromal cells that serves as a potent negative regulator of hematopoiesis. Blood 1998; 92:2613-28; PMID:9763543
- Watt SM, Buhring HJ, Rappold I, Chan JY, Lee-Prudhoe J, Jones T, Zannettino AC, Simmons PJ, Doyonnas R, Sheer D, et al. CD164, a novel sialomucin on CD34(+) and erythroid subsets, is located on human chromosome 6q21. Blood 1998; 92:849-66; PMID:9680353
- Buhring HJ, Seiffert M, Bock TA, Scheding S, Thiel A, Scheffold A, Kanz L, Brugger W. Expression of novel surface antigens on early hematopoietic cells. Ann N Y Acad Sci 1999; 872:25-38; PMID:10372108; http://dx.doi.org/10.1111/j.1749-6632.1999.tb08450.x
- Forde S, Tye BJ, Newey SE, Roubelakis M, Smythe J, McGuckin CP, Pettengell R, Watt SM. Endolyn (CD164) modulates the CXCL12-mediated migration of umbilical cord blood CD133+ cells. Blood 2007; 109:1825-33; PMID:17077324; http://dx.doi.org/10.1182/blood-2006-05-023028
- Tang J, Zhang L, She X, Zhou G, Yu F, Xiang J, Li G. Inhibiting CD164 expression in colon cancer cell line HCT116 leads to reduced cancer cell proliferation, mobility, and metastasis in vitro and in vivo. Cancer Invest 2012; 30:380-9; PMID:22409183; http://dx.doi.org/10.3109/07357907.2012.666692
- Havens AM, Jung Y, Sun YX, Wang J, Shah RB, Bühring HJ, Pienta KJ, Taichman RS. The role of sialomucin CD164 (MGC-24v or endolyn) in prostate cancer metastasis. BMC Cancer 2006; 6:195; PMID:16859559; http://dx.doi.org/10.1186/1471-2407-6-195
- Guglielmelli P, Zini R, Bogani C, Salati S, Pancrazzi A, Bianchi E, Mannelli F, Ferrari S, Le Bousse-Kerdilès MC, Bosi A, et al. Molecular profiling of CD34+ cells in idiopathic myelofibrosis identifies a set of disease-associated genes and reveals the clinical significance of Wilms’ tumor gene 1 (WT1). Stem Cells 2007; 25:165-73; PMID:16990584; http://dx.doi.org/10.1634/stemcells.2006-0351
- Uchida N, Yang Z, Combs J, Pourquié O, Nguyen M, Ramanathan R, Fu J, Welply A, Chen S, Weddell G, et al. The characterization, molecular cloning, and expression of a novel hematopoietic cell antigen from CD34+ human bone marrow cells. Blood 1997; 89:2706-16; PMID:9108388
- Degen WG, van Kempen LC, Gijzen EG, van Groningen JJ, van Kooyk Y, Bloemers HP, Swart GW. MEMD, a new cell adhesion molecule in metastasizing human melanoma cell lines, is identical to ALCAM (activated leukocyte cell adhesion molecule). Am J Pathol 1998; 152:805-13; PMID:9502422
- Hassan NJ, Barclay AN, Brown MH. Frontline: Optimal T cell activation requires the engagement of CD6 and CD166. Eur J Immunol 2004; 34:930-40; PMID:15048703; http://dx.doi.org/10.1002/eji.200424856
- Chitteti BR, Cheng YH, Kacena MA, Srour EF. Hierarchical organization of osteoblasts reveals the significant role of CD166 in hematopoietic stem cell maintenance and function. Bone 2013; 54:58-67; PMID:23369988; http://dx.doi.org/10.1016/j.bone.2013.01.038
- Jeannet R, Cai Q, Liu H, Vu H, Kuo YH. Alcam regulates long-term hematopoietic stem cell engraftment and self-renewal. Stem Cells 2013; 31:560-71; PMID:23280653; http://dx.doi.org/10.1002/stem.1309
- Hansen AG, Arnold SA, Jiang M et al. ALCAMCD166 is a TGFbeta responsive marker and functional regulator of prostate cancer metastasis to bone. Cancer Res 2014; 74:1404-15; PMID:24385212; http://dx.doi.org/10.1158/0008-5472.CAN-13-1296
- Piao D, Jiang T, Liu G, Wang B, Xu J, Zhu A. Clinical implications of activated leukocyte cell adhesion molecule expression in breast cancer. Mol Biol Rep 2012; 39:661-8; PMID:21670959; http://dx.doi.org/10.1007/s11033-011-0783-5
- Zhang WC, Shyh-Chang N, Yang H, Rai A, Umashankar S, Ma S, Soh BS, Sun LL, Tai BC, Nga ME, et al. Glycine decarboxylase activity drives non-small cell lung cancer tumor-initiating cells and tumorigenesis. Cell 2012; 148:259-72; PMID:22225612; http://dx.doi.org/10.1016/j.cell.2011.11.050
- Jiao J, Hindoyan A, Wang S, Tran LM, Goldstein AS, Lawson D, Chen D, Li Y, Guo C, Zhang B, et al. Identification of CD166 as a surface marker for enriching prostate stemprogenitor and cancer initiating cells. PLoS One 2012; 7:e42564; PMID:22880034; http://dx.doi.org/10.1371/journal.pone.0042564
- Reinboth B, Thomas J, Hanssen E, Gibson MA. Beta ig-h3 interacts directly with biglycan and decorin, promotes collagen VI aggregation, and participates in ternary complexing with these macromolecules. J Biol Chem 2006; 281:7816-24; PMID:16434404; http://dx.doi.org/10.1074/jbc.M511316200
- Hildebrand A, Romaris M, Rasmussen LM, Heinegård D, Twardzik DR, Border WA, Ruoslahti E. Interaction of the small interstitial proteoglycans biglycan, decorin and fibromodulin with transforming growth factor beta. Biochem J 1994; 302 (Pt 2):527-34; PMID:8093006
- Xu T, Bianco P, Fisher LW, Longenecker G, Smith E, Goldstein S, Bonadio J, Boskey A, Heegaard AM, Sommer B, et al. Targeted disruption of the biglycan gene leads to an osteoporosis-like phenotype in mice. Nat Genet 1998; 20:78-82; PMID:9731537; http://dx.doi.org/10.1038/2477
- Csont T, Gorbe A, Bereczki E, Szunyog A, Aypar E, Tóth ME, Varga ZV, Csonka C, Fülöp F, Sántha M, et al. Biglycan protects cardiomyocytes against hypoxiareoxygenation injury: role of nitric oxide. J Mol Cell Cardiol 2010; 48:649-52; PMID:20096286; http://dx.doi.org/10.1016/j.yjmcc.2010.01.013
- Gu X, Ma Y, Xiao J, Zheng H, Song C, Gong Y, Xing X. Up-regulated biglycan expression correlates with the malignancy in human colorectal cancers. Clin Exp Med 2012; 12:195-9; PMID:21879307; http://dx.doi.org/10.1007/s10238-011-0155-4
- Wang B, Li GX, Zhang SG, Wang Q, Wen YG, Tang HM, Zhou CZ, Xing AY, Fan JW, Yan DW, et al. Biglycan expression correlates with aggressiveness and poor prognosis of gastric cancer. Exp Biol Med (Maywood) 2011; 236:1247-53; PMID:21998129; http://dx.doi.org/10.1258/ebm.2011.011124
- Yamamoto K, Ohga N, Hida Y, Maishi N, Kawamoto T, Kitayama K, Akiyama K, Osawa T, Kondoh M, Matsuda K, et al. Biglycan is a specific marker and an autocrine angiogenic factor of tumour endothelial cells. Br J Cancer 2012; 106:1214-23; PMID:22374465; http://dx.doi.org/10.1038/bjc.2012.59
- Recktenwald CV, Leisz S, Steven A, Mimura K, Müller A, Wulfänger J, Kiessling R, Seliger B. HER-2neu-mediated down-regulation of biglycan associated with altered growth properties. J Biol Chem 2012; 287:24320-9; PMID:22582394; http://dx.doi.org/10.1074/jbc.M111.334425
- Amenta AR, Creely HE, Mercado ML, Hagiwara H, McKechnie BA, Lechner BE, Rossi SG, Wang Q, Owens RT, Marrero E, et al. Biglycan is an extracellular MuSK binding protein important for synapse stability. J Neurosci 2012; 32:2324-34; PMID:22396407; http://dx.doi.org/10.1523/JNEUROSCI.4610-11.2012