Abstract
Optimising response to tyrosine kinase inhibitors in cancer remains an extensive field of research. Intravital imaging is an emerging tool, which can be used in drug discovery to facilitate and fine-tune maximum drug response in live tumors. A greater understanding of intratumoural delivery and pharmacodynamics of a drug can be obtained by imaging drug target-specific fluorescence resonance energy transfer (FRET) biosensors in real time. Here, we outline our recent work using a Src-FRET biosensor as a readout of Src activity to gauge optimal tyrosine kinase inhibition in response to dasatinib treatment regimens in vivo. By simultaneously monitoring both the inhibition of Src using FRET imaging, and the modulation of the surrounding extracellular matrix using second harmonic generation (SHG) imaging, we were able to show enhanced drug penetrance and delivery to live pancreatic tumors. We discuss the implications of this dual intravital imaging approach in the context of altered tumor-stromal interactions, while summarising how this approach could be applied to assess other combination strategies or tyrosine kinase inhibitors in a preclinical setting.
Abbreviations
ECM | = | extracellular matrix |
ECFP | = | enhanced cyan fluorescent protein |
FLIM | = | fluorescent lifetime imaging microscopy |
FRET | = | fluorescence resonance energy transfer |
PDAC | = | pancreatic ductal adenocarcinoma |
SH2 | = | Src-homology-2 |
SHG | = | second harmonic generation |
RTK | = | receptor tyrosine kinase |
Introduction
Optimal drug delivery into solid tumors can be impaired by several factorsCitation1,2 Deregulation of the extracellular matrix (ECM) composition of the tumor microenvironment is a major determinant controlling drug penetrance. The increased deposition of ECM and subsequent fibrosis in pancreatic ductal adenocarinoma (PDAC) can limit drug perfusion into the tumor mass.Citation3 This process in pancreatic cancer is governed by tumor-associated stellate cells, which produce excessive amounts of collagen type I and other ECM components, such as fibronectins, leading to restricted drug delivery within the tissue.Citation4,5 Due to its fibrillar structure collagen type I can be visualized using multi-photon intravital microscopy. Its compact molecular structure generates a second harmonic generation (SHG) signal when excited with high energy pulsed laser.Citation6 This signal can then be further quantifiedCitation7 and the manipulation and efficacy of anti-ECM treatment regimens can be evaluated by live intravital imaging.Citation8
Recently, we demonstrated a correlation between Src expression levels and reduced survival in human pancreatic cancer, and showed that the activity of Src is an indicator of invasion and poor prognosis in human pancreatic cancer patients.Citation9 Importantly, we also established that the phase II, small molecule Src inhibitor, dasatinib, which is currently being clinically evaluated in combination with chemotherapy in locally advanced PDAC,Citation10 inhibited invasion of primary PDAC cells generated from the KrasG12D/+, Trp53R172H/+, Pdx1-Cre (KPC) model of pancreatic cancer, and reduced the development of metastases by approximately 50%.Citation11-13 In order to visualize Src activity upon treatment and inhibition with dasatinib within single cancer cells, we employed a FRET-based Src reporter in cells generated from this model to map the pharmacodynamics of the Src inhibitor dasatinib in vivo ().Citation8,9,12,14
Figure 1. FRET-based Src biosensor in vivo. (A) Functional schematic of the Src biosensor in both a FRET (bottom) and non-FRET confirmation (top). The probe is phosphorylated by active Src and dephosphorylated by phosphatases within the cell. The activity of Src is represented by the FLIM-bar, denoting Src-active (red to yellow) and -inactive colors (green to blue). (B and C) Src activity distribution in single cells at subcellular resolution in relation to local vasculature in vivo, with vasculature in red (quantum dots, Qtracker655), collagen in magenta (detected by SHG imaging) and cells in the FLIM colors; Scale bars in (B and C), 50 μm.
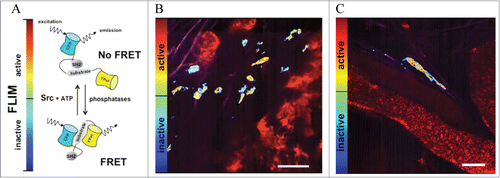
The Src reporter consists of an SH2 domain, a flexible linker and a peptide sequence derived from the c-Src substrate p130cas, flanked by 2 spectrally overlapping fluorophores ECFP and YPet.Citation14 When the substrate of Src kinase is unphosphorylated, the fluorophores remain in close proximity, leading to high FRET efficiency. Upon Src-induced phosphorylation, the substrate peptide binds to the SH2 domain and separates YPet from ECFP, thereby decreasing FRET efficiency (). This can be quantified by measuring the fluorescent lifetime (FLIM) of ECFP, which decreases when the reporter assumes a FRET-conformation. FLIM-FRET signals are then represented in heat-maps, where red to yellow colors are attributed to Src activity and blue to green colors represent inactive Src ().
This application of live functional imaging of Src activity with the use of FLIM-FRET allowed us to gauge Src activity and drug treatment response with high spatiotemporal resolution in a complex setting. We assessed the temporal inactivation of Src in response to dasatinib in live tumor tissue and were able to observe and quantify a drug clearance rate post oral administration (). Src was inactive at 2 hours post-dasatinib treatment and a gradual reactivation was observed after 4–6 hours, peaking and overcompensating at 16 hours before returning to control levels after 24 hours. We then assessed spatial regulation by examining Src activity in distinct locations within a complex microenvironment. Three-dimensional organotypic assays showed increased Src activity in proportion to PDAC invasive status (,Citation8,15). Similarly, this gradient also exists within live tumors, where Src activity is enhanced at invasive borders, relative to the tumor center, correlating with metastasis in this model.Citation8,9 Importantly, the organization and deposition of ECM components correlated with Src activity in vivo, where a highly organized, dense ECM was associated with high Src activity (quantified by SHG imaging and Gray Level Co-occurrence Matrix (GLCM) analysis, as previously describedCitation7,8,16). Further analysis revealed that Src regulation in response to dasatinib was also governed by the proximity of cells to the host vasculature (shown using quantum dots ()). Cells distal to the vasculature (>100 μm) were less responsive to dasatinib treatment than those close to blood vessels (, <25 μm). This detailed spatiotemporal mapping of drug performance in the context of vascularisation in vivo, combined with the capacity to simultaneously track ECM integrity, allowed us to utilize this technology to monitor improvements in dasatinib delivery at a subcellular resolution in response to ECM-targeted therapy.Citation8
Figure 2. Monitoring targeted response to dasatinib in vivo and in vitro using FLIM-FRET. (A) Drug treatment regimen and imaging schedule (top) allowing for monitoring of dasatinib treatment efficacy in vivo over time, with collagen in magenta (detected by SHG imaging) and cells in green (middle) with corresponding lifetime maps of Src activity (bottom). (B) Src activity distribution in single cells in relation to penetration depth through collagen matrix in vitro, assessed by immunohistochemistry for phosphorylated Src (left, pSrc positive cells stain brown) or by FLIM-FRET with corresponding quantification at 20 μm resolution steps (right). Columns, mean; bars, standard errors; *, p = 0.027; **, p = 0.021; ***, p = 0.006; ****, p < 0.001 by unpaired Student t test. Figure, partially reproduced from original highlighted paper (Citation8 courtesy of Cancer Research).
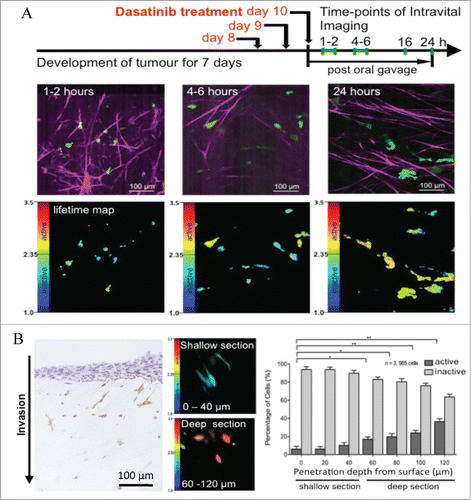
Figure 3. Shifting of spatial distribution of Src activity distant from local vasculature after drug treatment, as revealed by intravital imaging. (A) Innate gradient of Src activity distant from tumor vasculature, visualized by FLIM-FRET in conjunction with the quantum dot signal. (B) Quantification of shift in distribution of Src activity in single cells in relation to vessel proximity after dasatinib treatment (C) or after dasatinib treatment in combination with an ECM inhibitor (cyclopamine). Columns, mean; bars, standard errors. Figure, rearranged and reproduced from original highlighted paper (Citation8 courtesy of Cancer Research).
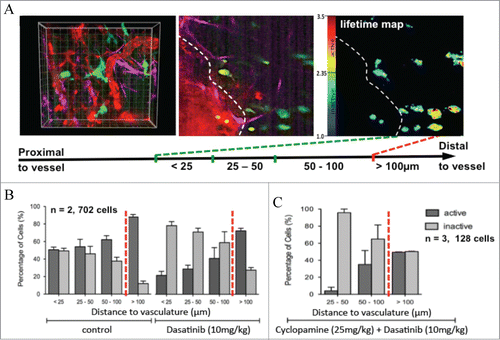
Targeting the ECM architecture to enhance drug penetration has recently been employed to improve chemotherapeutic drug delivery in solid tumor types such as pancreatic cancer. In particular, the role that stromal tissue plays in the perfusion deficit found in pancreatic cancer has been a rapidly evolving area of research.Citation17-19 In the KPC pancreatic mouse model described here, treatment with the hedgehog signaling inhibitor, IP-926, to deplete the tumor-associated ECM, increased tumor perfusion and the therapeutic index of standard-of-care chemotherapeutic agents such as gemcitabine resulting in increased overall survival.Citation19 Similarly, the enzymatic depletion of ECM glycosaminoglycans has recently been shown to improve the intratumoural delivery and efficacy of gemcitabine in vivo. Citation17,18 These studies therefore suggested that the current failure of drugs in the treatment of solid tumors may arise, partly, from a potentially reversible impairment in intratumoural drug delivery. We therefore determined whether a similar strategy could be adopted for dasatinib treatment in vivo.Citation9,10
In order to make the inherently dense tumor mass more accessible to dasatinib treatment, tumors were pre-treated with the hedgehog signaling inhibitor, cyclopamine, to reduce ECM content.Citation19,20 Manipulation of the surrounding ECM levels with cyclopamine and maintenance of tissue integrity was monitored by SHG imaging in conjunction with simultaneous FLIM-FRET imaging of the Src reporter.Citation8 A significant improvement in Src inhibition was achieved throughout the tumor mass using this approach, both at the tumor border versus the center, and at sites distant from the vasculature. Interestingly, at >100 μm from the vasculature, a limit was reached whereby no further inactivation of Src could be achieved. This could potentially be due to the limit of perfusion within the tissue or could be due to hypoxic and metabolic changes in tumor cells distant from the vasculature preventing dasatinib efficacy in this subpopulation. Further studies to assess whether this is the case are currently being carried out.
The concept that the ECM acts as a barrier to dasatinib diffusion within the tumor is unlikely to be the only explanation for the improved dasatinib response we observe in combination with ECM-targeted therapy. In , we propose that in cases of high fibrosis there is a bi-directional positive feedback loop between tumor cells and the surrounding ECM inducing Src signaling via integrin engagement, increasing the robustness of Src activity.Citation21,22 Upon ECM depletion or relaxation, this feedback may be reduced, thereby indirectly priming Src activity within the tumor to be more receptive to dasatinib treatment in combination therapy. In accordance with this, direct treatment of PDAC cells in vitro, with cyclopamine alone, had no effect on Src activity, while in the presence of signaling from the surrounding matrix in vivo, cyclopamine priming enhanced Src inactivation in response to dasatinib. Current studies to determine the level to which indirect ECM signaling or malperfusion plays a role in this dual targeting approach are underway.Citation23,24
Figure 4. Improving drug distribution away from the vasculature by targeting the ECM and decreasing its deposition, leading to a reduction in ECM exerted force and decreased integrin engagement and thus potentially a decrease / a longer suppression of Src activity.
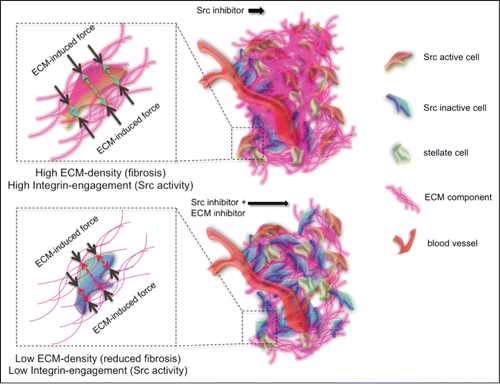
In this work we used a single time point to observe improved spatial inactivation of Src with combination therapy. It would be interesting for future studies to determine whether, upon ECM priming, the timing of Src inactivation occurs more rapidly or whether dasatinib accumulates and resides in the tumor for different time periods due to reduced matrix levels. Monitoring the relationships between spatial coverage and timing of drug delivery and drug retention is difficult, and this must be taken into consideration when streamlining improved drug targeting in future studies.Citation25
Targeting Other Aspects of the Tumor Microenvironment
In addition to the abundance of ECM components, tumors are heterogeneous tissues containing other elements such as blood vessels, lymphatic tissue and tumor-associated cell types such as fibroblasts, pericytes and immune cells.Citation26-31 The tumor microenvironment can therefore supply cancer cells with important cues during tumor progression and as is the case for the ECM, other aspects of the tumor environment could be important drug targets.
Blood vessels as transporters of oxygen, nutrients and therapeutic drugs are commonly deregulated in tumors. Their architecture is often poorly organized, leading to irregular blood flow, and due to the highly proliferative nature of tumor cells, blood and lymphatic vessels can become compressed within many solid tumors. As a result, this disordered composition of the vascular system often hinders drug penetrance in solid tumor types such as pancreatic cancer.Citation1,17,18 In line with this, recent work has demonstrated that ECM depletion reverts this compression and improves vascularity indirectly.Citation17,18 Another approach to enhance treatment efficacy could therefore be the indirect remodelling of the tumor vasculature. This has recently been shown with angiotensin inhibition and the associated downstream decrease in expression of profibrotic signals such as transforming growth factor (TGF)-β1, connective tissue growth factor (CTGF) and endothelin (ET)-1.Citation32 Here, the authors show that stress exerted upon vessels by excessive deposition of collagen and ECM components in breast and pancreatic tumors was decreased by angiotensin inhibition using losartan, and thus vascular perfusion and chemotherapeutic drug delivery was increased.Citation32 Repurposing of angiotensin inhibitors or similar drugs in this way could therefore be used in future combination treatments to improve drug penetrance. Similarly, actively increasing vascular patency directly could also help anti-cancer agents enter the tumor tissue more readily (). To achieve higher penetration of anti-cancer agents, drugs such as fasudilCitation33,34 or ascorbateCitation35 could be used, which have been shown previously to directly increase the patency of vessels. Monitoring and maximizing these direct or indirect improvements of drug targeting combinations by FLIM-FRET analysis may be used in future preclinical settings to fine-tune such drug targeting combinations.Citation8,36,37
Conversely, many tumor types are often associated with poor vasculature and vascular leakage, which impairs the efficiency of intratumoural blood pressure to drive effective drug delivery to the entire tumor. Targeting the vasculature using anti-angiogenics could help to improve drug delivery and retention in this scenario by normalization of the vessels, leading to a reduced number of leaky vessels and improving the intratumoural blood pressure of the existing vasculature (). Recently, the VEGF receptor tyrosine kinase inhibitor axitinib allowed for a longer retention time of an anti-cancer pro-drug within tumors to improve cyclophosphamide cytotoxic activity in gliosarcoma xenografts.Citation38 This blood vessel stabilization approach has also previously been observed for the treatment of tumors with the anti-angiogenic antibodies DC101 or bevacizumab as monotherapies.Citation39,40 These effects, however, were only transiently obtained and thus a careful scheduling of pre-treatment of tumors with anti-angiogenics in combination with other anti-cancer agents is key for their potential future clinical success. In order to optimally induce the normalization of the vasculature at the right time of treatment and thus maximise the retention of the drug in question, we propose that live intravital imaging of target inactivation could be employed in conjunction with monitoring blood vessel changes and permeability using quantum dots or fluorescent dextrans, as recently achieved.Citation8,37,41-43
Figure 5. Targeting the tumor vasculature to increase drug treatment efficacies. (A) Application of a potential anti-angiogenic leading to vessel normalization prior to treatment with the Src inhibitor could lead to an increase of drug retention time within the tumor mass. (B) An increase in vascular patency could lead to an increase in the potential influx of the Src inhibitor.
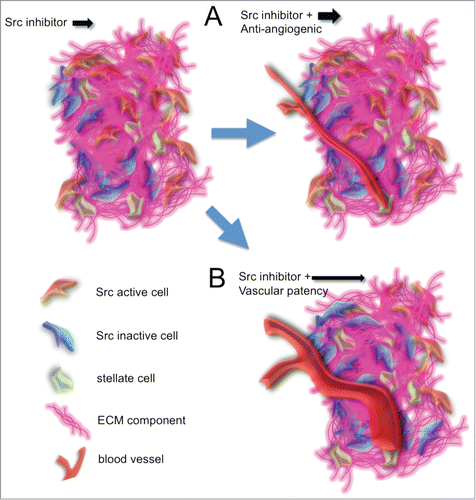
Figure 6. Schematic of potential applications of intravital FRET imaging. Depicting the current highlighted study, dasatinib matched with Src reporter, drug A with reporter and target A, drug (B) with indirect reporter and target B, drug (C) with downstream reporters and targets (C or D). Drug (D) in other tumor-associated cell types (e.g. fibroblasts, immune cells) providing indirect paracrine read out of the tumor cells of target D or read out of drug E by autocrine signaling inhibition of target (D).
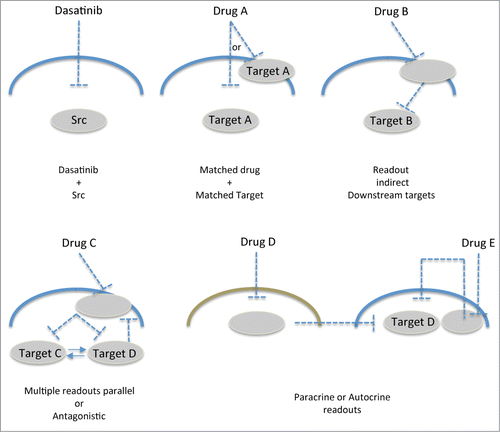
Our application of matching the drug of choice with available target reporters opens up new possibilities for precise monitoring of spatiotemporal signaling in drug discovery. Here, we matched dasatinib treatment with a Src-FRET reporter in a model of invasive pancreatic cancer in which Src inhibition has previously shown promise in both animal models and current phase II clinical trials.Citation9,10 However, FRET reporters of different targets in other models of disease or drug treatments could also be applied in a comparable fashion ( matched with target A). Similarly, indirect readout of downstream targets could also be achieved using this setup (). For example, Src activity downstream of EGFR targeting via gefitinib could be assessed in the current setup and may provide additional detail to the signal transduction cascade in receptor tyrosine kinase (RTK)-driven disease states in response to drug treatment.Citation44,45 Expression of multiple FRET biosensors within the same cell, which do not spectrally overlap, are also becoming available and could provide distinct parallel or antagonistic information in response to single agent treatments (,Citation46-49). This spatiotemporal approach could also be used to monitor signaling feedback loops to dissect redundant or resistance mechanisms in response to drug treatment (,Citation50). Moreover, targeting of stromal cell types, such as fibroblasts or immune cell populations within tumors, could also be assessed for paracrine interactions with cancer cells and indirect targets could be monitored with fine temporal and spatial resolution using this approach ().
Furthermore, this application in subcutaneous xenograft tumors lacks the inherent host environment unique to the tissue type in question. Our latest genetic engineering of a FRET reporter mouse,Citation51 crossed to pancreatic, mammary and intestinal tumor models has recently allowed us to apply this approach to native tissue and could provide significant insight into how molecular targets commonly hijacked in cancer behave in more physiological and functional settings in the future.Citation51 Finally, the use of cutaneous or abdominal optical windowsCitation52–54 permits longitudinal monitoring of protein activity in vivo in the same animal over repeated imaging periods and may further advance the screening of drug targeting efficacy in this setting.
In conclusion, the capacity to monitor changes in drug targeting at the molecular level in the context of the tumor landscape, including the proximity of cells to the host vasculature, their location with regards to tumor invasive borders and the integrity of the surrounding ECM or blood supply, could provide unprecedented insight into how best to streamline drug targeting within live tumor environments.
Disclosure of Potential Conflicts of Interest
No potential conflicts of interest were disclosed.
Acknowledgments
The authors wish to thank Dr Haley Bennett, Dr David Croucher and Dr Ellen Van Dam for critical reading of the manuscript.
Funding
This work was funded by NHMRC, ARC, CINSW, Cancer Council NSW and CRUK core grant.
References
- Au JLS, Jang SH, Zheng J, Chen CT, Song S, Hu L, Wientjes MG. Determinants of drug delivery and transport to solid tumors. J Control Release 2001; 74(1-3):31-46; PMID:11489481; http://dx.doi.org/10.1016/S0168-3659(01)00308-X
- Minchinton AI, Tannock IF. Drug penetration in solid tumours. Nat Rev Cancer 2006; 6(8):583-92; PMID:16862189; http://dx.doi.org/10.1038/nrc1893
- Erkan M, Hausmann S, Michalski CW, Fingerle AA, Dobritz M, Kleeff J, Friess H. The role of stroma in pancreatic cancer: diagnostic and therapeutic implications. Nat Rev Gastroenterol Hepatol 2012; 9(8):454-67; PMID:22710569; http://dx.doi.org/10.1038/nrgastro.2012.115
- Bachem MG, Schunemann M, Ramadani M, Siech M, Beger H, Buck A, Zhou S, Schmid-Kotsas A, Adler G. Pancreatic carcinoma cells induce fibrosis by stimulating proliferation and matrix synthesis of stellate cells. Gastroenterology 2005; 128(4):907-21; PMID:15825074; http://dx.doi.org/10.1053/j.gastro.2004.12.036
- Vonlaufen A, Joshi S, Qu CF, Phillips PA, Xu ZH, Parker NR, Toi CS, Pirola RC, Wilson JS, Goldstein D, Apte MV. Pancreatic stellate cells: Partners in crime with pancreatic cancer cells. Cancer Res 2008; 68(7):2085-93; PMID:18381413; http://dx.doi.org/10.1158/0008-5472.CAN-07-2477
- Roth S, Freund I. Second harmonic generation in collagen. J Chem Phys 1979; 70(4):1637-43; http://dx.doi.org/10.1063/1.437677
- Cicchi R, Kapsokalyvas D, De Giorgi V, Maio V, Van Wiechen A, Massi D, Lotti T, Pavone FS. Scoring of collagen organization in healthy and diseased human dermis by multiphoton microscopy. J Biophotonics. 2010; 3(1-2):34-43; PMID:19771581
- Nobis M, McGhee EJ, Morton JP, Schwarz JP, Karim SA, Quinn J, Edward M, Campbell AD, McGarry LC, Evans TR, et al. Intravital FLIM-FRET Imaging Reveals Dasatinib-Induced Spatial Control of Src in Pancreatic Cancer. Cancer Res. 2013; 73(15):4674-86; PMID:23749641; http://dx.doi.org/10.1158/0008-5472.CAN-12-4545
- Morton JP, Karim SA, Graham K, Timpson P, Jamieson N, Athineos D, Doyle B, McKay C, Heung MY, Oien KA, et al. Dasatinib Inhibits the Development of Metastases in a Mouse Model of Pancreatic Ductal Adenocarcinoma. Gastroenterology. 2010; 139(1):292-303; PMID:20303350; http://dx.doi.org/10.1053/j.gastro.2010.03.034
- Evans TRJ, Van Cutsem E, Moore MJ, Purvis JD, Strauss LC, Rock EP, et al. Dasatinib combined with gemcitabine (Gem) in patients (pts) with locally advanced pancreatic adenocarcinoma (PaCa): Design of CA180-375, a placebo-controlled, randomized, double-blind phase II trial. J Clin Oncol. 2012; 30(15); .
- Hingorani SR, Wang LF, Multani AS, Combs C, Deramaudt TB, Hruban RH, Rustgi AK, Chang S, Tuveson DA. Trp53(R172H) and KraS(G12D) cooperate to promote chromosomal instability and widely metastatic pancreatic ductal adenocarcinoma in mice. Cancer Cell. 2005; 7(5):469-83; PMID:15894267; http://dx.doi.org/10.1016/j.ccr.2005.04.023
- Morton JP, Timpson P, Karim SA, Ridgway RA, Athineos D, Doyle B, Jamieson NB, Oien KA, Lowy AM, Brunton VG, et al. Mutant p53 drives metastasis and overcomes growth arrest/senescence in pancreatic cancer. Proc Natl Acad Sci U S A. 2010; 107(1):246-51; PMID:20018721; http://dx.doi.org/10.1073/pnas.0908428107
- Hruban RH, Adsay NV, Albores-Saavedra J, Anver MR, Biankin AV, Boivin GP, Furth EE, Furukawa T, Klein A, Klimstra DS, et al. Pathology of genetically engineered mouse models of pancreatic exocrine cancer: Consensus report and recommendations. Cancer Res. 2006; 66(1):95-106; PMID:16397221; http://dx.doi.org/10.1158/0008-5472.CAN-05-2168
- Wang YX, Botvinick EL, Zhao YH, Berns MW, Usami S, Tsien RY, Chien S. Visualizing the mechanical activation of Src. Nature. 2005; 434(7036):1040-5; PMID:15846350; http://dx.doi.org/10.1038/nature03469
- Timpson P, McGhee EJ, Erami Z, Nobis M, Quinn JA, Edward M, Anderson KI. Organotypic collagen I assay: a malleable platform to assess cell behaviour in a 3-dimensional context. J Vis Exp 2011; (56):e3089; PMID:22025017
- Samuel MS, Lopez JI, McGhee EJ, Croft DR, Strachan D, Timpson P, Munro J, Schröder E, Zhou J, Brunton VG, et al. Actomyosin-Mediated Cellular Tension Drives Increased Tissue Stiffness and β-Catenin Activation to Induce Epidermal Hyperplasia and Tumor Growth. Cancer Cell. 2011; 19(6):776-91; PMID:21665151; http://dx.doi.org/10.1016/j.ccr.2011.05.008
- Jacobetz MA, Chan DS, Neesse A, Bapiro TE, Cook N, Frese KK, Feig C, Nakagawa T, Caldwell ME, Zecchini HI, et al. Hyaluronan impairs vascular function and drug delivery in a mouse model of pancreatic cancer. Gut. 2013; 62(1):112-U53; PMID:22466618; http://dx.doi.org/10.1136/gutjnl-2012-302529
- Provenzano PP, Cuevas C, Chang AE, Goel VK, Von Hoff DD, Hingorani SR. Enzymatic Targeting of the Stroma Ablates Physical Barriers to Treatment of Pancreatic Ductal Adenocarcinoma. Cancer Cell. 2012; 21(3):418-29; PMID:22439937; http://dx.doi.org/10.1016/j.ccr.2012.01.007
- Olive KP, Jacobetz MA, Davidson CJ, Gopinathan A, McIntyre D, Honess D, Madhu B, Goldgraben MA, Caldwell ME, Allard D, et al. Inhibition of Hedgehog Signaling Enhances Delivery of Chemotherapy in a Mouse Model of Pancreatic Cancer. Science. 2009; 324(5933):1457-61; PMID:19460966; http://dx.doi.org/10.1126/science.1171362
- Thayer SP, di Magliano MP, Heiser PW, Nielsen CM, Roberts DJ, Lauwers GY, Qi YP, Gysin S, Fernández-del Castillo C, Yajnik V, et al. Hedgehog is an early and late mediator of pancreatic cancer tumorigenesis. Nature. 2003; 425(6960):851-6; PMID:14520413; http://dx.doi.org/10.1038/nature02009
- Timpson P, Jones GE, Frame MC, Brunton VG. Coordination of cell polarization and migration by the Rho family GTPases requires Src tyrosine kinase activity. Curr Biol. 2001; 11(23):1836-46; PMID:11728306; http://dx.doi.org/10.1016/S0960-9822(01)00583-8
- Ginsberg MH, Partridge A, Shattil SJ. Integrin regulation. Curr Opin Cell Biol. 2005; 17(5):509-16; PMID:16099636; http://dx.doi.org/10.1016/j.ceb.2005.08.010
- Cabodi S, Di Stefano P, Leal MDC, Tinnirello A, Bisaro B, Morello V, Damiano L, Aramu S, Repetto D, Tornillo G, et al. Integrins and Signal Transduction. In: Becchetti A, Arcangeli A, editors. Integrins and Ion Channels: Molecular Complexes and Signaling. Berlin:Springer-Verlag Berlin; 2010; 43-54.; .
- Mitra SK, Schlaepfer DD. Integrin-regulated FAK-Src signaling in normal and cancer cells. Curr Opin Cell Biol. 2006; 18(5):516-23; PMID:16919435; http://dx.doi.org/10.1016/j.ceb.2006.08.011
- Nobis M, Carragher NO, McGhee EJ, Morton JP, Sansom OJ, Anderson KI, Timpson P. Advanced intravital subcellular imaging reveals vital three-dimensional signalling events driving cancer cell behaviour and drug responses in live tissue. Febs J. 2013; 280(21):5177-97; PMID:23678945; http://dx.doi.org/10.1111/febs.12348
- Borovski T, Melo FDE, Vermeulen L, Medema JP. Cancer Stem Cell Niche: The Place to Be. Cancer Res. 2011; 71(3):634-9; PMID:21266356; http://dx.doi.org/10.1158/0008-5472.CAN-10-3220
- Lu PF, Weaver VM, Werb Z. The extracellular matrix: A dynamic niche in cancer progression. J Cell Biol. 2012; 196(4):395-406; PMID:22351925; http://dx.doi.org/10.1083/jcb.201102147
- Friedl P, Alexander S. Cancer Invasion and the Microenvironment: Plasticity and Reciprocity. Cell. 2011; 147(5):992-1009; PMID:22118458; http://dx.doi.org/10.1016/j.cell.2011.11.016
- Edward M, Gillan C, Micha D, Tammi RH. Tumour regulation of fibroblast hyaluronan expression: a mechanism to facilitate tumour growth and invasion. Carcinogenesis. 2005; 26(7):1215-23; PMID:15746159; http://dx.doi.org/10.1093/carcin/bgi064
- Imamura T, Iguchi H, Manabe T, Ohshio G, Yoshimura T, Wang ZH, Suwa H, Ishigami S, Imamura M. Quantitative analysis of collagen and collagen subtypes I, III, and V in human pancreatic cancer, tumor-associated chronic pancreatitis, and alcoholic chronic pancreatitis. Pancreas. 1995; 11(4):357-64; PMID:8532652; http://dx.doi.org/10.1097/00006676-199511000-00007
- Devy J, Duca L, Cantarelli B, Joseph-Pietras D, Scandolera A, Rusciani A, Parent L, Thevenard J, Pasco SB, Tarpin M, et al. Elastin-derived peptides enhance melanoma growth in vivo by upregulating the activation of Mcol-A (MMP-1) collagenase. Br J Cancer. 2010; 103(10):1562-70; PMID:20959825; http://dx.doi.org/10.1038/sj.bjc.6605926
- Chauhan VP, Martin JD, Liu H, Lacorre DA, Jain SR, Kozin SV, Stylianopoulos T, Mousa AS, Han X, Adstamongkonkul P, et al. Angiotensin inhibition enhances drug delivery and potentiates chemotherapy by decompressing tumour blood vessels. Nat Commun. 2013; 4:2516; PMID:24084631; http://dx.doi.org/10.1038/ncomms3516
- Fukumoto Y, Matoba T, Ito A, Tanaka H, Kishi T, Hayashidani S, Abe K, Takeshita A, Shimokawa H. Acute vasodilator effects of a Rho-kinase inhibitor, fasudil, in patients with severe pulmonary hypertension. Heart. 2005; 91(3):391-2; PMID:15710736; http://dx.doi.org/10.1136/hrt.2003.029470
- Fujita H, Fukumoto Y, Saji K, Sugimura K, Demachi J, Nawata J, Shimokawa H. Acute vasodilator effects of inhaled fasudil, a specific Rho-kinase inhibitor, in patients with pulmonary arterial hypertension. Heart Vessels. 2010; 25(2):144-9; PMID:20339976; http://dx.doi.org/10.1007/s00380-009-1176-8
- Ekman T, Risberg B, Bagge U, Braide M. Blocking of endothelil-leukocyte interaction (rolling) does not improve reflow in the rat gastric-mucosa after hemorrhagic-shock and retransfusion. Shock. 1994; 2(4):257-61; PMID:7757517; http://dx.doi.org/10.1097/00024382-199410000-00004
- Timpson P, McGhee EJ, Morton JP, von Kriegsheim A, Schwarz JP, Karim SA, Doyle B, Quinn JA, Carragher NO, Edward M, et al. Spatial Regulation of RhoA Activity during Pancreatic Cancer Cell Invasion Driven by Mutant p53. Cancer Res. 2011; 71(3):747-57; PMID:21266354; http://dx.doi.org/10.1158/0008-5472.CAN-10-2267
- McGhee EJ, Morton JP, Von Kriegsheim A, Schwarz JP, Karim SA, Carragher NO, Sansom OJ, Anderson KI, Timpson P. FLIM-FRET imaging in vivo reveals 3D-environment spatially regulates RhoGTPase activity during cancer cell invasion. Small GTPases. 2011; 2(Article):239-44; PMID:22145098; http://dx.doi.org/10.4161/sgtp.2.4.17275
- Ma J, Chen C-S, Blute T, Waxman DJ. Antiangiogenesis enhances intratumoral drug retention. Cancer Res. 2011; 71(7):2675-85. http://dx.doi.org/10.1158/0008-5472.CAN-10-3242
- Ma J, Waxman DJ. Combination of antiangiogenesis with chemotherapy for more effective cancer treatment. Molecular Cancer Therapeutics. 2008; 7(12):3670-84; PMID:19074844; http://dx.doi.org/10.1158/1535-7163.MCT-08-0715
- Jain RK. Normalization of tumor vasculature: An emerging concept in antiangiogenic therapy. Science. 2005; 307(5706):58-62; PMID:15637262; http://dx.doi.org/10.1126/science.1104819
- Manning CS, Jenkins R, Hooper S, Gerhardt H, Marais R, Adams S, et al. Intravital imaging reveals conversion between distinct tumor vascular morphologies and localized vascular response to Sunitinib. IntraVital. 2013; 2(1):e24790; http://dx.doi.org/10.4161/intv.24790
- Pink DBS, Schulte W, Parseghian MH, Zijlstra A, Lewis JD. Real-Time Visualization and Quantitation of Vascular Permeability In Vivo: Implications for Drug Delivery. PLoS One. 2012; 7(3):e33760; PMID:22479438; http://dx.doi.org/10.1371/journal.pone.0033760
- Egawa G, Nakamizo S, Natsuaki Y, Doi H, Miyachi Y, Kabashima K. Intravital analysis of vascular permeability in mice using two-photon microscopy. Sci Rep. 2013; 3:1932; PMID:23732999; http://dx.doi.org/10.1038/srep01932
- Timpson P, Lynch DK, Schramek D, Walker F, Daly RJ. Cortaictin overexpression inhibits ligand-induced down-regulation of the epidermal growth factor receptor. Cancer Res. 2005; 65(8):3273-80; PMID:15833860
- Timpson P, Wilson AS, Lehrbach GM, Sutherland RL, Musgrove EA, Daly RJ. Aberrant expression of cortactin in head and neck squamous cell carcinoma cells is associated with enhanced cell proliferation and resistance to the epidermal growth factor receptor inhibitor gefitinib. Cancer Res. 2007; 67(19):9304-14; PMID:17909038; http://dx.doi.org/10.1158/0008-5472.CAN-07-0798
- Ouyang MX, Huang H, Shaner NC, Remacle AG, Shiryaev SA, Strongin AY, Tsien RY, Wang Y. Simultaneous Visualization of Protumorigenic Src and MT1-MMP Activities with Fluorescence Resonance Energy Transfer. Cancer Res. 2010; 70(6):2204-12; PMID:20197470; http://dx.doi.org/10.1158/0008-5472.CAN-09-3698
- Grant DM, Zhang W, McGhee EJ, Bunney TD, Talbot CB, Kumar S, Munro I, Dunsby C, Neil MA, Katan M, et al. Multiplexed FRET to Image Multiple Signaling Events in Live Cells. Biophys J. 2008; 95(10):L69-L71; PMID:18757561; http://dx.doi.org/10.1529/biophysj.108.139204
- Peyker A, Rocks O, Bastiaens PIH. Imaging activation of two Ras isoforms simultaneously in a single cell. Chembiochem. 2005; 6(1):78-85; PMID:15637661; http://dx.doi.org/10.1002/cbic.200400280
- Shcherbakova DM, Hink MA, Joosen L, Gadella TWJ, Verkhusha VV. An orange fluorescent protein with a large stokes shift for single-excitation multicolor FCCS and FRET imaging. J Am Chem Soc. 2012; 134(18):7913-23; PMID:22486524; http://dx.doi.org/10.1021/ja3018972
- Grecco HE, Roda-Navarro P, Girod A, Hou J, Frahm T, Truxius DC, Pepperkok R, Squire A, Bastiaens PI. In situ analysis of tyrosine phosphorylation networks by FLIM on cell arrays. Nat Methods 2010; 7(6):467-U80; PMID:20453867; http://dx.doi.org/10.1038/nmeth.1458
- Johnsson A-KE, Dai Y, Nobis M, Baker MJ, McGhee EJ, Walker S, Schwarz JP, Kadir S, Morton JP, Myant KB, et al. The Rac-FRET Mouse Reveals Tight Spatiotemporal Control of Rac Activity in Primary Cells and Tissues. Cell Rep 2014; 6(6):1153-64; PMID:24630994; http://dx.doi.org/10.1016/j.celrep.2014.02.024
- Kedrin D, Gligorijevic B, Wyckoff J, Verkhusha VV, Condeelis J, Segall JE, van Rheenen J. Intravital imaging of metastatic behavior through a mammary imaging window. Nat Methods. 2008; 5(12):1019-21; PMID:18997781; http://dx.doi.org/10.1038/nmeth.1269
- Ritsma L, Steller EJA, Ellenbroek SIJ, Kranenburg O, Rinkes I, van Rheenen J. Surgical implantation of an abdominal imaging window for intravital microscopy. Nature Protocols. 2013; 8(3):583-94; PMID:23429719; http://dx.doi.org/10.1038/nprot.2013.026
- Ritsma L, Ellenbroek SIJ, Zomer A, Snippert HJ, de Sauvage FJ, Simons BD, Clevers H, van Rheenen J. Intestinal crypt homeostasis revealed at single-stem-cell level by in vivo live imaging. Nature. 2014; 507:362-366; PMID:24531760; http://dx.doi.org/10.1038/nature12972