Abstract
Platelet-rich plasma (PRP) is a platelet concentrate in a small volume of plasma. It is highly enriched in growth factors able to stimulate the migration and growth of bone-forming cells. PRP is often used in clinical applications, as dental surgery and fracture healing. Platelet derived growth factor (PDGF), is highly concentrated in PRP and it was shown in our previous studies to provide the chemotactic stimulus to SaOS-2 osteoblasts to move in a microchemotaxis assay. Aim of the present studies is to analyze the effects of a PRP pretreatment (short time course: 30–150 min) of SaOS-2 cells with PRP on the organization of actin cytoskeleton, the main effector of cell mobility. The results indicate that a pretreatment with PRP increases chemokinesis and chemotaxis and concomitantly induces the organization of actin microfilaments, visualized by immunocytochemistry, in a directionally elongated phenotype, which is characteristic of the cells able to move. PRP also produces a transient increase in the expression of PGDF α receptor. This reorganization is blocked by the immunoneutralization of PDGF demonstrating the responsibility of this growth factor in triggering the mechanisms responsible for cellular movements.
Introduction
Bone is a dynamic tissue the integrity of which is maintained by a well organized balance of bone resorption by osteoclasts and bone formation by osteoblasts.Citation1 Osteoblasts are bone-forming cells derived from multipotent mesenchymal stem cells, which also give rise to chondrocytes, muscle cells, and adipocytes, whereas osteoclasts are multinucleated giant cells differentiated for bone resorption and derived from the monocyte/macrophage lineage precursor cells.Citation2
Fracture healing is a complex physiological process initiated in response to injury. It involves the coordinated participation of haematopoietic and immune cells within the bone marrow in conjunction with vascular and skeletal cell precursors, including mesenchymal stem cells (MSCs), recruited from the surrounding tissues and the circulation.Citation3 Platelets play an important role both in the initial hemostatic process and in the further phases of wound healing, releasing multiple growth factors and cytokines including: IL-2, IL-8, TNF-α, stromal-derived factor 12, vascular endothelial growth factor (VEGF), platelet derived growth factor (PDGF), transforming growth factor β (TGF-β), insulin like growth factor-1 (IGF1), bone morphogenetic proteins (BMP) Citation3-5 and basic fibroblast growth factor (bFGF) bFGF.Citation3-6
PRP is an autologous concentration of human platelets in a small volume of plasma; this preparation has a very high concentration of growth factors derived from the α granules released upon platelet activation and able to stimulate the migration and growth of bone-forming cells. In recent years, PRP has been often used in a variety of clinical applications, including spine and dental surgery, wound and fracture healing, as well as cartilage repair.Citation3,7-12 For recent review on bone reconstruction therapy and tissue regeneration see.Citation13,14
In previous studies Citation15,16 of this laboratory we have evaluated the effect of PRP and of the 2 more abundant growth factors present in this preparation, PDGF and TGF−β, on the migration and proliferation of the human osteoblastic cell line SaOS-2. The overall results obtained indicate that PRP dose-dependently stimulates both chemotaxis and cell proliferation. PDGF and TGF-β appear to exert distinct effects on the 2 parameters, the former appears to be involved in stimulating cell migration while the latter in inhibiting cell proliferation.Citation15 Among the different PDGF isoforms contained in PRP the-AB isoform appears the major responsible of the PRP chemotaxis.Citation16
Since in the above mentioned studies PRP has been used as chemoattractant in a Boyden Chamber microchemotaxis assay, aim of the studies here presented is to evaluate if a pretreatment with PRP on SaOS-2 cells might produce a modulation of the cytoskeletal organization of actin microfilaments which in turn affects the cell motility.
Results
To test if a PRP pretreatment could affect mobility of SaOS-2 cells, we performed a migration test in the Boyden Chamber. In it is possible to observe the effect of 1 hour pre-incubation of the osteoblasts with PRP diluted 1:100 and 1:1000 on the ability of the cells to cross the Boyden Chamber membrane in 16 hours without the drive of a chemotactic stimulus (chemokynesis). Even though there is an increasing trend in cell movement across the membrane in response to PRP, PRP significantly stimulates the cellular movement across the membrane; the effect of the two PRP concentrations is not statistically different.
Figure 1. Effect of 1 hour PRP pre-treatment at 1/100 and 1/1000 dilution on SaOS-2 chemokinesis. The migration assay was performed in Boyden Chamber in 16 hours. Results are expressed as means of percentage of cells migrated versus control.
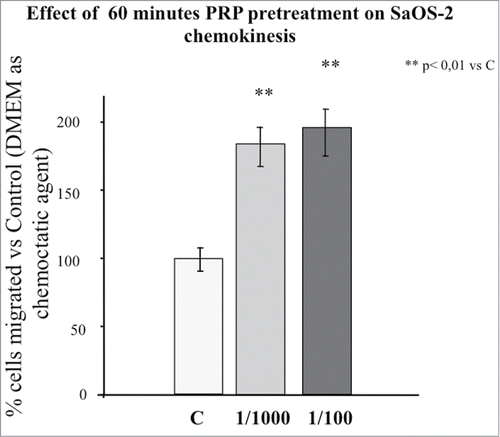
When a known chemoattractant (FBS 1%) was utilized in the Boyden Chamber assay, the number of cells that crossed the membrane increased so much that the migration assay had to be shortened to 4 hours to obtain a reliable cell count. shows the effect of 1 hour incubation with the same PRP dilutions used in chemokinesis experiments on the chemotaxis induced by 1% FBS. The SaOS-2 migration is strongly stimulated by the pre-incubation with PRP but, as in the case of chemokinesis, a significant dose-dependent effect of PRP is not present indicating that the cellular mechanisms triggered by PRP are saturated or almost saturated at 1:1000 dilution. Therefore, all further experiments were done utilizing the higher dilution. A single migration test utilizing a PRP pre-incubation with the dilution 1:2000 did not induce a significant cell migration (data not shown).
Figure 2. Effect of 1 hour PRP pre-treatment at 1/100 and 1/1000 dilution on SaOS-2 migration in presence of FBS 1% as chemotactic agent. The migration assay was performed in Boyden Chamber in 4 hours. Results are expressed as means of percentage of cells migrated versus control.
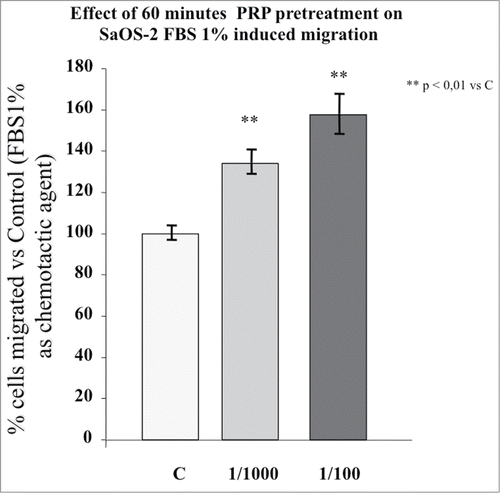
Since we have previously shown that among the growth factors present in PRP, PDGF is responsible of inducing chemotaxis of SaOS-2 cells,Citation16 it was felt of interest to evaluate the effect of the PRP pretreatment on the expression of the α and β PDGF receptors. The data indicate (, panel A) that the expression of the α receptor is almost equally stimulated by both the doses of PRP after 30 minutes of incubation. The effect is rapidly lost and the stimulation is not present in the cells incubated with PRP for one hour. Moreover, the α receptor expression appears inhibited (significantly with the lower dose) in the longest incubation time (150 min).
Figure 3. (A) Effect of a PRP treatment on the PDGF receptor α expression SaOS-2 osteoblasts. Cells treated with culture media, with PRP 1/100 or with PRP 1/1000 for 30, 60 or 150 minutes. (B) Effect of a PRP treatment on the PDGF receptor β expression SaOS-2 osteoblasts. Cells treated with culture media, with PRP 1/100 or with PRP 1/1000 for 30, 60 or 150 minutes.
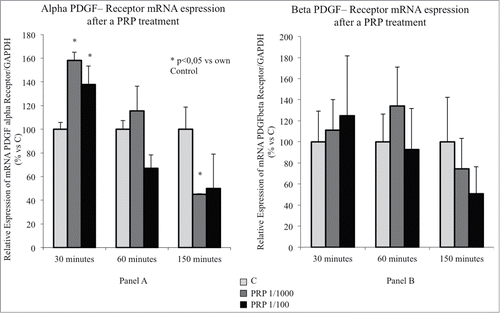
The β receptor, even if shows a very low expression,Citation16 has a similar expression profile (, panel B) but due to the high standard deviation of these measurements the differences never reach the statistical significance.
To test if the cytoskeleton can represent a target of PRP pretreatment, we evaluated by immunofluorescence the rearrangement of actin microfilaments by FITC-phalloidin labeling. In the it is possible to observe the different phases of actin rearrangement after a pretreatment with PRP 1:1000 lasting 30, 60 and 150 minutes. Panel A shows un-stimulated cells possessing a round shape and a diffuse actin network of thin actin filaments. In the following panels (B-D) we can observe a progressive change of osteoblast cytoskeleton morphology, produced by the reorganization of stress fibers along a longitudinal axis, with the formation of F-actin bundles and the appearance of a structure as lamellipodium, rich in actin microspikes. This structure is even more apparent by confocal microscopy (). The images show the front of a spreading or moving cell, with a dense network of actin filaments. Fine, finger-like protrusions extend beyond the leading edge and contain parallel bundles of actin filaments.
Figure 4. Effect of a PRP treatment on rearrangement of acin microfilaments. Images of phalloidin stained SaOS cells treated with culture media (A), with PRP 1/1000 for 30 minutes (B), with PRP 1/1000 for 60 minutes (C), with PRP 1/1000 for 150 minutes (D). Images in fluorescence microscopy at 63× magnification.
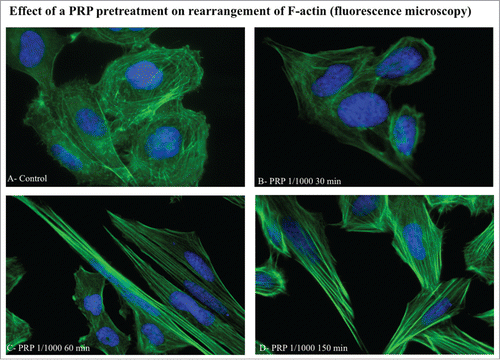
Figure 5. Effect of a PRP treatment on rearrangement of actin microfilaments. Fluorescence images of phalloidin stained SaOS-2 cells treated with culture media (A), PRP 1/1000 for 60 minutes (B) and PRP 1/1000 for 150 minutes (C). In the details (B and C) a lamellipodium rich in actin microspikes. Images in confocal microscopy at 63× magnification, zoom 2×.
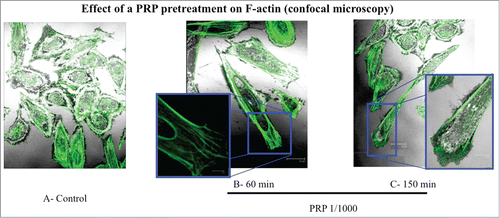
shows the distribution of SaOS-2 cells among 3 different phenotypes described in the literature,Citation17 before and after 60 minutes pre-incubation with PRP 1:1000. The Type I phenotype presents a circular organization of thin actin filaments, the Type II osteoblasts are large with well-formed radial and cortical actin filaments ending in focal contact points; Type III are well-spread with a well-organized array of longitudinal stress fibers aligned with the long axis of the cell.
Figure 6. Phenotype composition of SaOS-2 cells after a 1 hour PRP treatment. Fluorescence images of the 3 types of SaOS-2 cells (Type I, Type II, and Type III) counted in control and PRP treated (for 1 hour) coltures. The number of cells is the mean of 6 fields for each treatment. Images in fluorescence microscopy at 10× magnification.
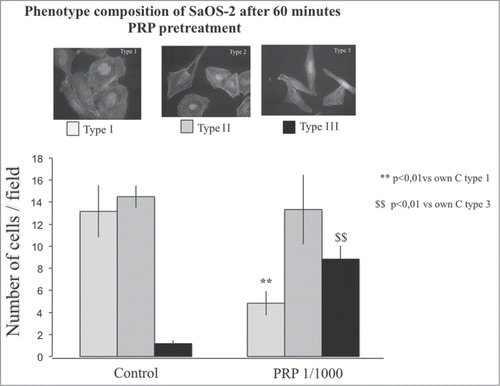
The distribution of the osteoblast types after a PRP pretreatment shows a consistent and significant decrease of type 1 osteoblasts with a shift toward the type III phenotype, indicative of the achievement of the spreading and cellular movements ().
Among all growth factors present in PRP, PDGF, in particular the AB isoform, has been shown to have an important chemoactractive effect in osteoblast migration which can be blocked neutralizing the effect of the growth factor by a suitable PDGF antibody.Citation15 We tested in these studies the ability of an anti-PDGF antibody to neutralize the effect of the PRP pretreatment on the chemokinetic and chemotactic activities of the SaOS-2 cells. It appears from that a pretreatment with PRP plus the PDGF antibody abolishes completely, even at the lower dose tested, the promigratory effect of PRP both in terms of chemokinesis and chemotaxis ().
Figure 7. Effect of different doses of anti-PDGF-PRP pre-treatment on SaOS-2 migration in presence or in absence (DMEM) of FBS 1% as chemotactic agent. Before migration, cells were exposed for 1 hour to PRP at 1/1000 dilution. The migration assay was performed in Boyden Chamber in 4 hours. Results are expressed as means of percentage of cells migrated versus control.
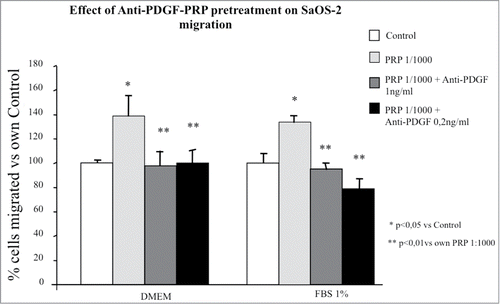
Moreover, the antibody neutralization of PDGF is also able to block the rearrangement of actin cytoskeleton characteristic of the actively moving cells. The morphology of the antibody treated osteoblasts is similar to that present in control cells (), which do not show the typical stress fibers organization acquired after PRP treatment.
Figure 8. Effect of an Anti PDGF-PRP treatment on the rearrangement of actin microfilaments. Fluorescence images of phalloidin stained SaOS-2 cells treated with culture media (A), PRP 1/1000 for 60 minutes (B), PRP 1/1000 plus Ab PDGF 0.2 ng/ml (C) and PRP 1/1000 plus Ab PDGF 1 ng/ml and (D). Images in fluorescence microscopy at 63× magnification.
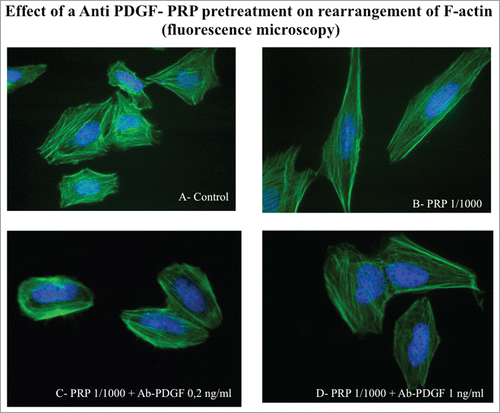
Discussion
The cytoskeletal organization of a cell is extremely important in modulating cell morphology, mobility and adhesion. In this study we have shown that a short incubation of the human osteoblast cell line (SaOS-2) with PRP is able to induce the organization of actin microfilaments in a phenotype indicative of extracellular protein adhesion and cell motility and of improved capabilities to respond to chemotactic stimuli. To the authors’ knowledge this is the first time that cytoskeleton changes induced by PRP have been described in osteoblasts. The antibody neutralization studies have shown that PDGF, among the many growth factors present in PRP, is responsible of this cytoskeletal reorganization. It is possible that PRP acts through a transiently increased expression of the α receptor of PDGF since it is known that some cytokines and growth factors contained in PRP, among which β-FGF, IL-2 and TNF-α, are able to modulate the genetic expression of PDGF-R.Citation18 A significant correlation between the expression of PDGF-AA and PDGF-α receptor has also been described in osteosarcoma cells indicating the existence of a possible positive feed-back mechanism.Citation19
The involvement of actin in cell motility is well documented.Citation20 Cells initiate movement by extending a protrusion of the membrane driven by actin polymerization. This movement is followed by the establishment of adhesion plaques and leads to a cell that is directionally elongated. Actin organization in the cytoplasm of osteoblasts produces a cytoarchitecture which characterizes the cells as having either Type I, Type II, or Type III phenotype.Citation17 In general, Type I osteoblasts are round cells and represent a preliminary stage of actin assembly. Type II are larger with formation of actin stress fibers and increased cellular diameter. Type III cells are elongated osteoblast with organized actin fiber network, demonstrating spreading and cellular movement.Citation21 A short incubation with PRP is sufficient to produce a dose dependent increase of the osteoblast chemokinesis (increased migration in the Boyden chamber in a overnight test in absence of a specific chemotatic attractant) and of FBS 1% induced chemotaxis (4 hour migration test in presence of FBS as a known chemo-attractant). Globally the enhancing effect of PRP on the osteoblast chemotaxis appears to be time (from 30 to 150 min) dependent. These functional data are in close correlation with the morphological aspect of the cell cytoskeleton architecture of the actin microfilaments in the cell. The images show a reorganization of actin microfilaments toward a phenotype (Type III) characteristic of cells able to move: namely actin polymerizes forming lamellipodia, adhesion plaques are established and cells appear directionally elongated. Similar transient (lasting less than 40 min) changes in cytoskeletal morphology have been shown in AG1518 fibroblasts after a short time incubation with PGDF-BB.Citation22 At variance with the cytoskeletal changes observed in fibroblasts the effect of PRP on SaOS-2 osteoblasts appears more progressive and long-lasting (up to 150 min). This is probably due to different signaling pathways differentially activated in specific cell types.
It has been shown that among the growth factors present in PRP, PDGF is responsible of inducing chemotaxis of SaOS-2 cellsCitation15; the immunoneutralization of this growth factor completely inhibits the PRP induced cell migration. Analogous results have been obtained in this study in which the anti-PDGF antibody is able to antagonize the reorganization of actin cytoskeleton in a phenotype indicative of the achievement of the spreading and cellular movements. The specificity of PDGF contained in PRP in inducing chemotactic responsiveness in the osteoblasts is strengthened by the observation that the PRP induced proliferation is not immunoneutralized by PDGF antibody and that doses of synthetic PDGF much higher than that contained in PRP are necessary to induce proliferation.Citation15,16
In previous studies from this lab we demonstrated that PDGF-AB is the most abundant isoform in PRP and that it is the most active in inducing chemotaxis at the concentrations present in PRP.Citation16
Two distinct PDGF receptors, α and β, mediate the effects of the PDGFs on target cells. The PDGF-A and -C chains selectively bind the α receptor, whereas PDGF-D preferentially binds the β receptor and PDGF-B displays a similar affinity for both receptors. SaOS-2 cell line, was shown by reverse transcription-polymerase chain reaction to express both PDGF-α and -β receptors.Citation15
Several other types of cells, beside osteoblasts, have been shown to acquire migration capacity and in some cases to undergo cytoskeletal changes when primed with PDGF: among them hepatic stellate cells,Citation23 marrow-derived mesenchymal stem cells,Citation24 malignant mesothelioma cells,Citation25 subchondral progenitors,Citation26 gingival fibroblasts.Citation27
The clinical use of PRP has recently experienced a surge in different indications and in particular in the procedures involving healing and tissue regeneration, as in dental and oral surgery,Citation9 in bone deficiency healing,Citation3,7,10 in the treatment for tendon and ligament injuries,Citation8,11 in the pathologic processes of articular cartilage.Citation12
In all these indications the recruitment of cells in the site of the lesion might represent the initial and crucial step in the process of healing. As shown in our studies, this process is stimulated by a suitable cytoskeletal reorganization produced by the application of PRP by virtue of the growth factors contained in this preparation and in particular of PDGF. A further clarification of the cellular and molecular mechanism induced by the growth factors contained in PRP might help in improving the clinical application of this easily available natural product.
Materials and methods
Cell cultures
SaOS-2 is a mature osteoblastic cell line (derived from a human osteosarcoma), grown in DMEM supplemented with 10% FBS (fetal bovine serum), glutamine (2 mM), penicillin (100 IU/mL), streptomycin (100 microg/mL) and sodium-pyruvate (1 mM).
PRP preparation
PRP was obtained from blood of pool of normal healthy volunteers and prepared as previously described.Citation15 Briefly, the platelet pellet obtained after centrifugation (platelet enrichment of about 4–5 times, 1.0–1.2 × 106 platelets/microL on average) was activated with calcium gluconate/batroxobine (Pentapharm). The platelet gel was centrifuged (1400 g, 10 min, RT) and the liquid phase, enriched in growth factors (PRP in text and figures), was frozen at −80°C in aliquots. Under these storage conditions, the preparation was equally effective for many months. Aliquots from the same preparation were used for all of the experiments described here.
Pretreatments
Before migration, SaOS-2 cells were exposed to:
The pretreatment with PRP 1/100 or 1/1000 diluted in DMEM serum free was applied for different time exposure (30, 60 or 150 minutes). The migration in Boyden chamber was carried out for 16 hours (chemokinesis studies) or 4 hours (chemotaxis studies).
PRP diluted 1/1000 in serum free DMEM plus 0.2 ng/ml or 1 ng/ml of the Anti- human PDGF antibody (goat polyclonal antibody, Sigma-Aldrich). It recognizes all human PDGF homodimeric and heterodimeric forms and shows no cross-reactivity with other human growth factors tested. Cells maintained in serum free DMEM were used as controls.
For the immunofluorescence studies, the same treatment protocol (PRP 1/100, PRP 1/1000 or Anti-human PDGF) was applied for different time exposure (30, 60 or 150 minutes).
For the gene expression studies the same treatment protocol (PRP 1/100, PRP 1/1000) was applied for different time exposure (30, 60 or 150 minutes).
Microchemokinesis and microchemotaxis assay
The analysis of cell migration was performed using a 48-well Boyden's chamber according to manufacture's instructions (Neuroprobe). Briefly, cells were plated 48 hours before at a density of 600000 cells in 10 mm plate, to avoid confluence. The cells were pretreated for the time and for PRP dilution chosen (see above) and tripsinized. For migration experiments, 28 ul of control media (DMEM) or DMEM+FBS 1% were placed in the lower compartment of the chamber, while the open-bottom wells of the upper compartment were filled with cells (50 ul of cell suspension) collected by trypsin and resuspended in DMEM +0.1% BSA (105 cells/well). Each pair of wells are separated by a polyvinylpyrolidone-free polycarbonate porous membrane (8 micron pores) precoated with gelatine (0.2 mg/mL in PBS) the day before and maintained at 4°C until use. After migration (overnight 37°C or 4 hours 37°C), cells, adherent to the underside of the membrane, were fixed by methanol and stained according to the Diff-Quik kit (Biomap). For quantitative analysis, cells were observed and counted using a 20 × or 40 × objective on an optical microscope. Three random objective fields of stained cells were counted for each well and the mean number of migrating cells was calculated. Each experiment was repeated 3 times. Data are expressed as mean ± SE.
Morphological studies of the cytoskeleton rearrangements
SaOS-2 cells were plated at a density of 4 × 104 cells per well on 14-mm glass coverslips in 24 well plates. After 48 hours cells were exposed to PRP as described above in the ‘treatment’ paragraph. Cells were fixed with paraformaldehyde 3% and sucrose 2% for 10 minutes at 37°. After the fixation, cells were washed twice with PBS with calcium and magnesium and permeabilized with Triton X-100 0.2% in PBS for one minute at room temperature. The fixed cells were washed with PBS tablets for 5 minutes and incubated with phalloidin-FITC 1/1000 (Sigma-Aldrich) for 20 minutes at 37°C. Images were obtained at 40× and 63× magnification for cytosckeleton rearrangement and at 10× magnification for phenotype composition of osteoblasts with a Zeiss Axiovert microscope equipped for fluorescence analysis with the corresponding sets of filters to detect FITC and DAPI emission. Confocal microscopy images were obtained with a laser confocal microscope (Leica TCSNT) at CIMA facility (University of Milan).
SaOS-2 phenotype composition was assessed counting each kind of osteoblast present in each field after phalloidin-FITC staining. Data are expressed as mean ± SE.
Each experiment was repeated 3 times.
Gene expression by quantitative PCR
Total RNA for the evaluation of the expression of α and β PDGF receptors was isolated by a guanidinium isothiocyanate/phenol-chloroform extraction; 2 ug of total RNA were amplified with Real-Time PCR, using the SYBR Green method. Briefly, RNA was retrotranscribed using the High-Capacity cDNA Archive Kit (Invitrogen Life Science); Real-Time PCR was performed using the SYBR Green PCR Master Mix (Invitrogen Life Science). Primers specific for selected genes were designed by primer Express software; the efficiency of each set of primers was near 100% for both target and reference gene (GADPH). The relative quantification was evaluated by comparative CT method (see Citation16).
Statistical analysis
Data were analyzed by ANOVA and expressed as mean ± SE (SPSS 20, PRISM 6).
Acknowledgments
We thank Mrs. Ornella Mornati for the valuable technical assistance.
Funding
This study was funded by the grant “Programma Dell’Università Per la Ricerca” (PUR, 2008).
References
- Harada S, Rodan GA. Control of osteoblast function and regulation of bone mass. Nature 2003; 423:349-55; PMID:12748654; http://dx.doi.org/10.1038/nature01660
- Boyle WJ, Simonet WS, Lacey DL. Osteoclast differentiation and activation. Nature 2003; 423:337-42; PMID:12748652; http://dx.doi.org/10.1038/nature01658
- Dimitriou R, Tsiridis E, Giannoudis PV. Current concepts of molecular aspects of bone healing. Injury 2005; 36:1392-404; PMID:16102764; http://dx.doi.org/10.1016/j.injury.2005.07.019
- Lieberman JR, Daluiski A, Einhorn TA. The role of growth factors in the repair of bone. Biology and clinical applications. J Bone Joint Surg Am Volume 2002; 84-A:1032-44; PMID:12063342
- Werner S, Grose R. Regulation of wound healing by growth factors and cytokines. Physiol Rev 2003; 83:835-70; PMID:12843410
- Perut F, Filardo G, Mariani E, Cenacchi A, Pratelli L, Devescovi V, Kon E, Marcacci M, Facchini A, Baldini N, et al. Preparation method and growth factor content of platelet concentrate influence the osteogenic differentiation of bone marrow stromal cells. Cytotherapy 2013; 15:830-9; PMID:23731763; http://dx.doi.org/10.1016/j.jcyt.2013.01.220
- Latalski M, Elbatrawy YA, Thabet AM, Gregosiewicz A, Raganowicz T, Fatyga M. Enhancing bone healing during distraction osteogenesis with platelet-rich plasma. Injury 2011; 42:821-4; PMID:21513935; http://dx.doi.org/10.1016/j.injury.2011.03.010
- Foster TE, Puskas BL, Mandelbaum BR, Gerhardt MB, Rodeo SA. Platelet-rich plasma: from basic science to clinical applications. Am J Sports Med 2009; 37:2259-72; PMID:19875361; http://dx.doi.org/10.1177/0363546509349921
- Albanese A, Licata ME, Polizzi B, Campisi G. Platelet-rich plasma (PRP) in dental and oral surgery: from the wound healing to bone regeneration. Immun Ageing: I & A 2013; 10:23; PMID:23763951; http://dx.doi.org/10.1186/1742-4933-10-23
- Zhang N, Wu Y-P, Qian S-J, Teng C, Chen S, Li H. Research progress in the mechanism of effect of PRP in bone deficiency healing. Sci World J 2013; 2013:7; PMID:23710132; http://dx.doi.org/10.1155/2013/134582
- Taylor DW, Petrera M, Hendry M, Theodoropoulos JS. A systematic review of the use of platelet-rich plasma in sports medicine as a new treatment for tendon and ligament injuries. Clin J Sport Med 2011; 21:344-52; PMID:21562414; http://dx.doi.org/10.1097/JSM.0b013e31821d0f65
- Smyth NA, Murawski CD, Fortier LA, Cole BJ, Kennedy JG. Platelet-rich plasma in the pathologic processes of cartilage: review of basic science evidence. Arthrosc: J Arthrosc Related Surg: Off Pub Arthrosc Assoc North America Int Arthrosc Assoc 2013; 29:1399-409; PMID:23669235; http://dx.doi.org/10.1016/j.arthro.2013.03.004
- Intini G. The use of platelet-rich plasma in bone reconstruction therapy. Biomaterials 2009; 30:4956-66; PMID:19573909; http://dx.doi.org/10.1016/j.biomaterials.2009.05.055
- Alsousou J, Ali A, Willett K, Harrison P. The role of platelet-rich plasma in tissue regeneration. Platelets 2013; 24:173-82; PMID:22647081; http://dx.doi.org/10.3109/09537104.2012.684730
- Celotti F, Colciago A, Negri-Cesi P, Pravettoni A, Zaninetti R, Sacchi MC. Effect of platelet-rich plasma on migration and proliferation of SaOS-2 osteoblasts: role of platelet-derived growth factor and transforming growth factor-beta. Wound Repair Regen: Off Pub Wound Healing Soc Eur Tissue Repair Soc 2006; 14:195-202; PMID:16630109; http://dx.doi.org/10.1111/j.1743-6109.2006.00110.x
- Colciago A, Celotti F, Casati L, Giancola R, Castano SM, Antonini G, Sacchi MC, Negri-Cesi P. In vitro effects of PDGF isoforms (AA, BB, AB and CC) on migration and proliferation of SaOS-2 osteoblasts and on migration of human osteoblasts. Int J Biomed Sci 2009; 5:380-9; PMID:23675162
- El-Amin SF, Lu HH, Khan Y, Burems J, Mitchell J, Tuan RS, Laurencin CT. Extracellular matrix production by human osteoblasts cultured on biodegradable polymers applicable for tissue engineering. Biomaterials 2003; 24:1213-21; PMID:12527262; http://dx.doi.org/10.1016/S0142-9612(02)00451-9
- Heldin CH, Westermark B. Mechanism of action and in vivo role of platelet-derived growth factor. Physiol Rev 1999; 79:1283-316; PMID:10508235
- Sulzbacher I, Traxler M, Mosberger I, Lang S, Chott A. Platelet-derived growth factor-AA and -alpha receptor expression suggests an autocrine and/or paracrine loop in osteosarcoma. Modern Pathol: An Off J U S Canadian Acad Pathol, Inc 2000; 13:632-7; PMID:10874667; http://dx.doi.org/10.1038/modpathol.3880109
- Ridley AJ, Schwartz MA, Burridge K, Firtel RA, Ginsberg MH, Borisy G, Parsons JT, Horwitz AR. Cell migration: integrating signals from front to back. Science 2003; 302:1704-9; PMID:14657486; http://dx.doi.org/10.1126/science.1092053
- Shah AK, Lazatin J, Sinha RK, Lennox T, Hickok NJ, Tuan RS. Mechanism of BMP-2 stimulated adhesion of osteoblastic cells to titanium alloy. Biol Cell Under Auspices Eur Cell Biol Organ 1999; 91:131-42; PMID:10399828; http://dx.doi.org/10.1016/S0248-4900(99)80037-9
- van Wieringen T, Kimani SG, Hultgard-Ekwall AK, Forsberg J, Reyhani V, Engstrom A, Rubin K. Opposite effects of PDGF-BB and prostaglandin E1 on cell-motility related processes are paralleled by modifications of distinct actin-binding proteins. Exp Cell Res 2009; 315:1745-58; PMID:19233168; http://dx.doi.org/10.1016/j.yexcr.2009.02.005
- Li L, Li J, Wang JY, Yang CQ, Jia ML, Jiang W. Role of RhoA in platelet-derived growth factor-BB-induced migration of rat hepatic stellate cells. Chinese Med J 2010; 123:2502-9; PMID:21034618
- Yoshida S, Iwasaki R, Kawana H, Miyauchi Y, Hoshi H, Miyamoto H, Mori T, Kanagawa H, Katsuyama E, Fujie A, et al. PDGFBB promotes PDGFRα-positive cell migration into artificial bone in vivo. Biochem Biophys Res Commun 2012; 421:785-9; PMID:22554514; http://dx.doi.org/10.1016/j.bbrc.2012.04.084
- Okada A, Yaguchi T, Kanno T, Gotoh A, Nakano T, Nishizaki T. PDGF-D/PDGF-betabeta receptor-regulated chemotaxis of malignant mesothelioma cells. Cell Physiol Biochem: Int J Exp Cell Physiol, Biochem, Pharmacol 2012; 29:241-50; PMID:22415093; http://dx.doi.org/10.1159/000337605
- Kruger JP, Hondke S, Endres M, Pruss A, Siclari A, Kaps C. Human platelet-rich plasma stimulates migration and chondrogenic differentiation of human subchondral progenitor cells. J Orthop Res: Off Pub Orthop Res Soc 2012; 30:845-52; PMID:22058056; http://dx.doi.org/10.1002/jor.22005
- Caceres M, Hidalgo R, Sanz A, Martinez J, Riera P, Smith PC. Effect of platelet-rich plasma on cell adhesion, cell migration, and myofibroblastic differentiation in human gingival fibroblasts. J Periodontol 2008; 79:714-20; PMID:18380566; http://dx.doi.org/10.1902/jop.2008.070395