Abstract
Extracellular matrix (ECM) provides both structural support and contextual information to cells within tissues and organs. The combination of biochemical and biomechanical signals from the ECM modulates responses to extracellular signals toward differentiation, proliferation, or apoptosis; alterations in the ECM are necessary for development and remodeling processes, but aberrations in the composition and organization of ECM are associated with disease pathology and can predispose to development of cancer. The primary cell surface sensors of the ECM are the integrins, which provide the physical connection between the ECM and the cytoskeleton and also convey biochemical information about the composition of the ECM. Transforming growth factor-β (TGF-β) is an extracellular signaling molecule that is a powerful controller of a variety of cellular functions, and that has been found to induce very different outcomes according to cell type and cellular context. It is becoming clear that ECM-mediated signaling through integrins is reciprocally influenced by TGF-β: integrin expression, activation, and responses are affected by cellular exposure to TGF-β, and TGF-β activation and cellular responses are in turn controlled by signaling from the ECM through integrins. Epithelial-mesenchymal transition (EMT), a physiological process that is activated by TGF-β in normal development and in cancer, is also affected by the composition and structure of the ECM. Here, we will outline how signaling from the ECM controls the contextual response to TGF-β, and how this response is selectively modulated during disease, with an emphasis on recent findings, current challenges, and future opportunities.
Basics of ECM and Integrin Signaling
ECM is a dynamic and complex combination of collagens, glycoproteins and proteoglycans.Citation1,2 It provides structural support in bone, cartilage, and the basement membrane; specific association of cells with the ECM also provides contextual information that controls cellular phenotype, including differentiation, proliferation, or apoptosis.Citation3,4 ECM also regulates availability and activity of many signaling molecules, including TGF-β, through controlled sequestration, presentation, and release.Citation5,6 The primary cell surface receptors for the ECM are the integrins, a family of 24 heterodimeric proteins composed of one of 18 α-subunits and 8 β-subunits.Citation7,8 Integrins bind to motifs present in the ECM though an interaction domain located between the α- and β-subunit; while many integrin-binding motifs have been identified, the best studied is the arginine-glycine-aspartate (RGD) sequence that is present in fibronectin and many other extracellular molecules. Integrins become activated in a process that is regulated both by availability of ECM substrate (outside-in activation) and signals from within the cell (inside-out activation).Citation9 Activated integrins can bind to the actin cytoskeleton and recruit a variety of cytosolic components into adhesion complexes. Through those interactions integrins can transduce biochemical signaling dependent on ECM composition, as well as directly link physical forces acting on the ECM to the cellular cytoskeleton ().Citation10,11
Basics of TGF-β Signaling
Three TGF-β isoforms are present in mammals, TGF-β1, -2, and -3, each encoded by a separate gene, and each playing distinct physiological roles during development.Citation12 Exposure of cells to an active TGF-β isoform leads to assembly of a TGF-β-ligated tetrameric receptor complex, composed of 2 type I and 2 type II TGF-β receptor subunits (TGFBRI and TGFBRII). TGFBRII then phosphorylates TGFBRI, enabling it to activate downstream signaling responses, which are regulated through canonical and noncanonical signaling pathways ().
Figure 2. TGF-β signaling. TGF-β signaling can be initiated by binding of TGF-β to TGF-β receptor type III (TGFBRIII), which can then assemble with TGF-β receptors types II and I (TGFBRII, TGFBRI) to form an active signaling complex. In the canonical signaling pathway, the active signaling complex phosphorylates the receptor SMADs (SMAD2 and SMAD3), which then associate with SMAD4, translocate to the nucleus and affect gene transcription. In the noncanonical pathway, phosphorylation of the TGF-β receptor complex leads to activation of cytosolic signaling pathways, including MAPK, PI3K/Akt, and Rho GTPases.
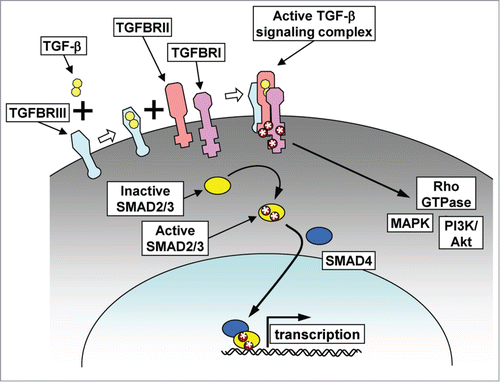
In the canonical signaling pathway, TGFBRI phosphorylates the receptor SMAD proteins, SMAD2 or SMAD3. Phosphorylated receptor SMADs associate with SMAD4 and the resultant oligomeric complex becomes translocated to the nucleus, where it can bind to a variety of other transcription factors and cofactors. Transcriptional alterations induced by the canonical pathway vary according to the strength and sustained maintenance of the TGF-β receptor signaling, the composition and availability of existing transcriptional cofactors, and the epigenetic landscape of the chromatin at the time of TGF-β pathway activation.Citation13 The gene expression effects are highly cell type and context dependent; for example, the canonical signaling pathway inhibits expression of the inhibitor of differentiation (ID1) gene in normal mammary epithelial cells, but activates its expression in breast cancer cells.Citation13-15
In the noncanonical signaling pathway, the activated TGF-β receptor complex directly regulates non-SMAD-dependent pathways to activate, sustain, or modulate cellular responses.Citation16,17 Non-canonical pathways include activation of ERK/MAPK signaling through tyrosine phosphorylation of TGFBR1 and recruitment of Grb/Shc, and subsequent activation of Ras,Citation18,19 which may contribute to TGF-β-dependent induction of senescence and prevention of transformation in normal human mammary epithelial cells.Citation20 TGF-β can also induce JNK/p38 through a SMAD-independent pathway,Citation21,22 which can then reinforce SMAD-dependent transcriptional alterations through a reactive oxygen species (ROS)-mediated mechanism.Citation23 Rho family GTPases can be regulated through SMAD-independent TGF-β signaling: RhoA, Rac1, and Cdc42 can be activated in epithelial cells,Citation24-26 or RhoA can be targeted for degradation via a pathway initiated by direct phosphorylation of the polarity protein PAR6 by TGFBRII.Citation27,28 TGF-β can also activate Akt through SMAD-independent induction of PI3K, which can in turn act as a regulator of the canonical pathway through phosphorylation of ERK and consequent activation of SMADs.Citation29 The principal determinant and mediator of whether TGF-β will signal through the canonical or noncanonical pathway in normal cells is SMAD7, which is able to inhibit phosphorylation of receptor SMADs through multiple mechanisms.Citation30 SMAD7 is a transcriptional target of the canonical pathway, which constitutes a mechanism for channeling signaling from canonical to noncanonical pathways.``
TGF-β expression has been studied in many tumor types, where it has been found to function both as a tumor suppressor and a tumor promoter. In normal cells and in early stage tumors, TGF-β acts to block cell proliferation through the canonical pathways, including via SMAD-dependent inhibition of MYC, as well as activation of cyclin-dependent kinase inhibitors. Additionally, in premalignant cells which have acquired oncogenic mutations, TGF-β can induce apoptosis. In more advanced tumors, the TGF-β-dependent cytostatic effects are suppressed, and EMT-associated cell invasion and metastasis become dominant.Citation31,32 The transition from tumor-inhibitory to tumor-promoting behaviors has been described as the TGF-β paradox, and while multiple components of this transition have been discovered, many aspects remain unknown.Citation33 Additionally, TGF-β can induce a variety of different anti- and pro-tumorigenic effects indirectly by acting on stromal cells in the cancer microenvironment.Citation34
TGF-β Regulation of ECM Secretion and Integrin Function
One of the most highly investigated roles of TGF-β in pathology is in the context of development of tissue fibrosis.Citation35 Under normal circumstances, tissue damage triggers a wound healing response characterized by deposition of transitional ECM, followed by activation and invasion of fibroblasts that remodel and contract the wound ECM; once tissue homeostasis is restored, the fibroblasts undergo apoptosis. Under fibrotic conditions, however, a feedback loop is activated in which excessive ECM deposition leads to increased proliferation and activation of ECM-producing fibroblasts. Maintained for extended periods of time, fibrosis can become a significant problem in its own right, and can also stimulate malignant transformation and promote tumor progression.Citation36,37 TGF-β has been implicated as a critical player in chronic fibrosis of many organs, including lung, kidney, liver, and skin. TGF-β directly stimulates expression of ECM proteins, including collagen, fibronectin, and proteoglycans.Citation38-40 TGF-β induces conversion of fibroblasts into myofibroblasts which can further contract and distort the ECM. TGF-β can also directly stimulate myofibroblast formation from epithelial and endothelial cells through EMT-related processes, and this function is essential for development of fibrosis in several organs.Citation41-44 The relative amount of epithelial- vs fibroblast-derived myofibroblasts is a current point of debate and is likely to be highly tissue specific.
In addition to inducing integrin signaling through increased production of ECM, TGF-β can also regulate integrin function directly. TGF-β can control expression of αv-, β3-, and β1-integrin subunits through both canonical and noncanonical pathways, according to cell type.Citation45-48 TGF-β signaling can also directly phosphorylate and activate β1-integrin, stimulating cell invasion and facilitating tissue regeneration.Citation49,50 TGF-β can also induce cross-talk between integrins and growth factor receptors, including via activation of focal adhesion kinase (FAK)-dependent clustering of ErbB2 (HER2) and integrins α6, β1, and β4 through a pathway initiated by EGFR-dependent phosphorylation and activation of SRC; the overall effect of this pathway is increased cell migration and survival.Citation51
Integrin Regulation of TGF-β Activation and Signaling
Integrins are directly involved in the activation of TGF-β (). Citation52,53 TGF-β isoforms are translated as preproproteins that contain a 25–30 kDa latency associated peptide (LAP) and the 13 kDa TGF-β molecule. LAP-TGF-β homodimers linked by disulfide bonds are formed in the endoplasmic reticulum (ER), followed by protoelytic cleavage of LAP from TGF-β in the Golgi; the resultant homodimers of TGF-β and LAP remain noncovalently associated following secretion as an inactive protein complex called the small latency complex (SLC). Many cell types also produce latent TGF-β-binding protein (LTBP), which can covalently bind to the SLC, producing the large latency complex (LLC), which can become associated with fibrillar ECM molecules.Citation52,53 Outside of the cell, TGF-β can be activated following release from the SLC, which can occur through selective proteolytic digestion, exposure to ROS, or through direct interaction with ECM molecules.Citation54-56 Recent studies have identified a process by which TGF-β1 and TGF-β3 can be released from their LAP-TGF-β complex through a force-dependent conformational shift induced by association of RGD motifs in their respective LAP proteins with integrins; all αv-containing integrins (αvβ1, αvβ3, αvβ5, αvβ6, and αvβ8) as well as α8β1 integrin have been shown to bind LAP, although whether αvβ1 and α8β1 can activate latent TGF-β has not been determined.Citation57-59 The relevance of αv integrin-mediated activation of latent TGF-β was demonstrated by studies showing that transgenic mice with mutations in the RGD motif of the TGF-β1-associated LAP protein recapitulate the phenotype of the TGF-β1 knockout mouse; similar developmental alterations are seen in mice lacking a functional αv-integrin gene.Citation60-62 Of αv-containing integrins that can activate latent TGF-β, αvβ6- and αvβ8-integrins have been shown to play a critical role in TGF-β activation during development and in immune homeostasis, while αvβ3 and αvβ5 may play a more important role in TGF-β activation during fibrosis.Citation6,63,64
Figure 3. Interaction of integrins and TGF-β signaling pathways. (a) Integrin binding to latent TGF-β complexes can lead to release of active TGF-β. (b) Signaling from integrins or TGF-β receptors can stimulate expression of receptors or effectors of the other signaling pathway. (c) Activation of integrins or TGF-β receptors can lead to activation or inhibition of the other signaling receptor. (d) Cooperative signaling from integrins and TGF-β receptor may be necessary to stimulate phenotypic outcomes, including EMT.
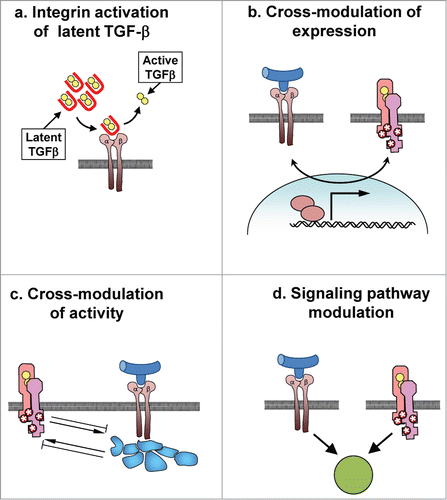
In addition to controlling TGF-β activation, integrins can also affect signaling downstream of the TGF-β receptor.Citation6 This can occur through integrin-mediated activation of the TGF-β receptor, through stimulation of canonical and noncanonical signaling pathways, and through increased transcription of genes encoding TGF-β and TGF-β receptor isoforms.Citation6,65 Recent studies have shown that the pathways regulating these effects vary in different cell and tissue types.Citation66 Activity of β1-integrins and the integrin signaling mediator integrin-linked kinase (ILK) are required for TGF-β-induced EMT in mammary epithelial cells.Citation67,68 Integrin α3β1 is necessary for TGF-β-induced EMT of alveolar epithelial cells and development of pulmonary fibrosis,Citation69 and integrin α1β1 is required for TGF-β-induced kidney fibrosis.Citation70 Integrin αvβ5 and ILK activity are a prerequisite for TGF-β-dependent activation of dermal fibroblasts.Citation71-73 Furthermore, the response of the TGFβ receptor can be potentiated through binding of specific integrins to TGFBRII, leading to phosphorylation of the receptor in a FAK- and SRC- dependent manner.Citation73-78 These effects can be quite cell-type specific: in mammary epithelial cells, the activation of TGFBRII by interaction with integrin β3 is inhibited by signaling from integrin β1, while in chondrocytes, integrin β1 preferentially associates with and activates TGFBRII.Citation76,77
As an additional layer of control, the strength of the signal from the TGF-β receptor complex depends on which TGF-β ligand is bound, as the ligands vary in their ability to bind to TGFBRI and to assemble the active tetrameric receptor complex with TGFBRII; the co-receptor, TGFBRIII, can further modulate the ligand-dependent assembly and activation of the complex.Citation6,34 While a role for integrin-mediated regulation of TGF-β and receptor isoform expression is relatively unexplored, it is known that activation of integrin α5β1 stimulates TGFBRII expression, while expression of both TGFBRI and TGFBRII can be repressed by integrin αvβ3.Citation79,80
Approaches to Therapeutic Intervention
As TGF-β has been found to play a central role in promotion of tumor cell invasion and metastasis, stimulation of pathological EMT, and induction of cancer-promoting microenvironmental changes, it is unsurprising that there has been considerable effort to develop inhibitors targeting the TGF-β pathway as potential cancer therapeutics. To date, multiple inhibitors of the TGF-β signaling have been developed and tested in animal models and clinical trials.Citation34,81,82 These include monoclonal antibody-based inhibitors of TGF-β signaling, for example fresolimumab (GC1008), a fully human monoclonal antibody against TGF-β-1, -2 and -3, currently tested in clinical trials for combinatorial treatment with radiation therapy in metastatic breast cancer (ClinicalTrials.gov identifier: NCT01401062) and in glioma (ClinicalTrials.gov identifier: NCT01472731). Another avenue for TGF-β pathway inhibition is through synthetic antisense oligonucleotides, such as trabedersen (AP 12009), targeting TGF-β2, currently being tested for treatment of glioma,Citation83 pancreatic cancer, melanoma and colorectal cancer.Citation84 Other types of TGF-β pathway inhibitors include small molecule TGF-β receptor kinase inhibitors which bind to and directly block receptor signaling, as well as peptide aptamers which bind to and inhibit downstream mediators of the TGF-β pathway, such as SMADs.Citation34,81,85
An alternative way to interfere with TGF-β signaling would be to target αv integrin subunit integrins required for activation of latent TGF-β. Multiple anti-integrin αv small molecule synthetic inhibitors and monoclonal anitbodies have been developed and are being tested in preclinical and clinical trials.Citation8,86,87 Among these, the monoclonal antibody intentumumabCitation88,89 is currently being tested in patients with melanoma (ClinicalTrials.gov identifier: NCT00246012) and prostate cancer (ClinicalTrials.gov identifier: NCT00537381). It should be noted that, given the pleiotropic effects of TGF-β and potential development of resistance, the current paradigms for targeting TGF-β signaling in cancer treatment have focused on short term dosing in combination with other therapies.
Conclusions
TGF-β is a multi-functional cytokine that regulates virtually all cellular processes. It can act as a tumor suppressor, blocking proliferation and inhibiting stromal mitogens, as well as a tumor promoter and an inducer of cancer-associated EMT, allowing evasion of immune surveillance and stimulating invasion and metastatic spread. Those opposing consequences of TGF-β signaling are highly cell type dependent and regulated by the cellular context. ECM is a key regulator of both initiation of TGF-β signaling and a determinant of its outcomes. Integrins, acting as bidirectional signal transducers between the cellular microenvironment and the cell itself, play crucial roles in this process. Often, the presence of certain integrins is required for activation of latent TGF-β and thus induction of downstream signaling pathways; integrins can also lead to ligand-independent signaling via activation of TGF-β receptor. The complexity and dual nature of TGF-β signaling effects reinforce the need to specifically study the context and determinants of those different responses. Moreover, while it is the paradigmatic view that in early stages of tumor development TGF-β acts to inhibit proliferation, whereas in later stages that aspect of signaling is lost in favor of promotion of EMT and invasiveness, we should be mindful that this phenotypic transition from cytostasis to motility is unlikely to occur in every cell within a tumor, and that competition between pathways responsible for these different phenotypes likely leads to complex and sometimes unexpected outcomes. Most importantly, if TGF-β pathway is to be targeted in cancer therapy, the challenge remains to predict the types and stages of tumors in which TGF-β signaling inhibition would prevent metastasis without facilitating growth of the primary tumor.
Funding
This work was supported by grants from NIH (CA116201), Susan B. Komen Foundation (KG110542), and Jimmy V. Foundation.
References
- Hynes RO, Naba A. Overview of the matrisome–an inventory of extracellular matrix constituents and functions. Cold Spring Harb Perspect Biol 2012; 4:a004903; PMID:21937732; http://dx.doi.org/10.1101/cshperspect.a004903
- Kim SH, Turnbull J, Guimond S. Extracellular matrix and cell signalling: the dynamic cooperation of integrin, proteoglycan and growth factor receptor. J Endocrinol 2011; 209:139-51; PMID: 21307119; http://dx.doi.org/10.1530/JOE-10-0377
- Radisky D, Muschler J, Bissell MJ. Order and disorder: the role of extracellular matrix in epithelial cancer. Cancer Invest 2002; 20:139-53; PMID:11852996; http://dx.doi.org/10.1081/CNV-120000374
- Lu P, Takai K, Weaver VM, Werb Z. Extracellular matrix degradation and remodeling in development and disease. Cold Spring Harb Perspect Biol 2011; 3:pii: a005058; PMID: 21917992
- Hanahan D, Coussens LM. Accessories to the crime: functions of cells recruited to the tumor microenvironment. Cancer Cell 2012; 21:309-22; PMID:22439926; http://dx.doi.org/10.1016/j.ccr.2012.02.022
- Margadant C, Sonnenberg A. Integrin-TGF-beta crosstalk in fibrosis, cancer and wound healing. EMBO Rep 2010; 11:97-105; PMID:20075988; http://dx.doi.org/10.1038/embor.2009.276
- Barczyk M, Carracedo S, Gullberg D. Integrins. Cell Tissue Res 2010; 339:269-80; PMID:19693543; http://dx.doi.org/10.1007/s00441-009-0834-6
- Desgrosellier JS, Cheresh DA. Integrins in cancer: biological implications and therapeutic opportunities. Nat Rev Cancer 2010; 10:9-22; PMID:20029421; http://dx.doi.org/10.1038/nrc2748
- Giancotti FG, Ruoslahti E. Integrin signaling. Science 1999; 285:1028-32; PMID:10446041; http://dx.doi.org/10.1126/science.285.5430.1028
- Glukhova MA, Streuli CH. How integrins control breast biology. Curr Opin Cell Biol 2013; 25:633-41; PMID:23886475; http://dx.doi.org/10.1016/j.ceb.2013.06.010
- Ross TD, Coon BG, Yun S, Baeyens N, Tanaka K, Ouyang M, Schwartz MA. Integrins in mechanotransduction. Curr Opin Cell Biol 2013; 25:613-8; PMID:23797029; http://dx.doi.org/10.1016/j.ceb.2013.05.006
- Shi Y, Massague J. Mechanisms of TGF-beta signaling from cell membrane to the nucleus. Cell 2003; 113:685-700; PMID:12809600; http://dx.doi.org/10.1016/S0092-8674(03)00432-X
- Massague J. TGFbeta signalling in context. Nat Rev Mol Cell Biol 2012; 13:616-30; PMID:22992590; http://dx.doi.org/10.1038/nrm3434
- Kang Y, Chen CR, Massague J. A self-enabling TGFbeta response coupled to stress signaling: Smad engages stress response factor ATF3 for Id1 repression in epithelial cells. Mol Cell 2003; 11:915-26; PMID:12718878; http://dx.doi.org/10.1016/S1097-2765(03)00109-6
- Padua D, Zhang XH, Wang Q, Nadal C, Gerald WL, Gomis RR, Massagué J. TGFbeta primes breast tumors for lung metastasis seeding through angiopoietin-like 4. Cell 2008; 133:66-77; PMID:18394990; http://dx.doi.org/10.1016/j.cell.2008.01.046
- Mu Y, Gudey SK, Landstrom M. Non-Smad signaling pathways. Cell Tissue Res 2012; 347:11-20; PMID:21701805; http://dx.doi.org/10.1007/s00441-011-1201-y
- Zhang YE. Non-Smad pathways in TGF-beta signaling. Cell Res 2009; 19:128-39; PMID:19114990; http://dx.doi.org/10.1038/cr.2008.328
- Galliher AJ, Schiemann WP. Src phosphorylates Tyr284 in TGF-beta type II receptor and regulates TGF-beta stimulation of p38 MAPK during breast cancer cell proliferation and invasion. Cancer Res 2007; 67:3752-8; PMID:17440088; http://dx.doi.org/10.1158/0008-5472.CAN-06-3851
- Lee MK, Pardoux C, Hall MC, Lee PS, Warburton D, Qing J, Smith SM, Derynck R. TGF-beta activates Erk MAP kinase signalling through direct phosphorylation of ShcA. EMBO J 2007; 26:3957-67; PMID:17673906; http://dx.doi.org/10.1038/sj.emboj.7601818
- Cipriano R, Kan CE, Graham J, Danielpour D, Stampfer M, Jackson MW. TGF-beta signaling engages an ATM-CHK2-p53-independent RAS-induced senescence and prevents malignant transformation in human mammary epithelial cells. Proc Natl Acad Sci U S A 2011; 108:8668-73; PMID:21555587; http://dx.doi.org/10.1073/pnas.1015022108
- Itoh S, Thorikay M, Kowanetz M, Moustakas A, Itoh F, Heldin CH, ten Dijke P. Elucidation of Smad requirement in transforming growth factor-beta type I receptor-induced responses. J Biol Chem 2003; 278:3751-61; PMID:12446693; http://dx.doi.org/10.1074/jbc.M208258200
- Yu L, Hebert MC, Zhang YE. TGF-beta receptor-activated p38 MAP kinase mediates Smad-independent TGF-beta responses. EMBO J 2002; 21:3749-59; PMID:12110587; http://dx.doi.org/10.1093/emboj/cdf366
- Martin-Garrido A, Brown DI, Lyle AN, Dikalova A, Seidel-Rogol B, Lassegue B, San Martín A, Griendling KK. NADPH oxidase 4 mediates TGF-beta-induced smooth muscle alpha-actin via p38MAPK and serum response factor. Free Radic Biol Med 2011; 50:354-62; PMID:21074607; http://dx.doi.org/10.1016/j.freeradbiomed.2010.11.007
- Bhowmick NA, Ghiassi M, Bakin A, Aakre M, Lundquist CA, Engel ME, Arteaga CL, Moses HL. Transforming growth factor-beta1 mediates epithelial to mesenchymal transdifferentiation through a RhoA-dependent mechanism. Mol Biol Cell 2001; 12:27-36; PMID:11160820; http://dx.doi.org/10.1091/mbc.12.1.27
- Edlund S, Landstrom M, Heldin CH, Aspenstrom P. Transforming growth factor-beta-induced mobilization of actin cytoskeleton requires signaling by small GTPases Cdc42 and RhoA. Mol Biol Cell 2002; 13:902-14; PMID:11907271; http://dx.doi.org/10.1091/mbc.01-08-0398
- Wilkes MC, Murphy SJ, Garamszegi N, Leof EB. Cell-type-specific activation of PAK2 by transforming growth factor beta independent of Smad2 and Smad3. Mol Cell Biol 2003; 23:8878-89; PMID:14612425; http://dx.doi.org/10.1128/MCB.23.23.8878-8889.2003
- Ozdamar B, Bose R, Barrios-Rodiles M, Wang HR, Zhang Y, Wrana JL. Regulation of the polarity protein Par6 by TGFbeta receptors controls epithelial cell plasticity. Science 2005; 307:1603-9; PMID:15761148; http://dx.doi.org/10.1126/science.1105718
- Viloria-Petit AM, David L, Jia JY, Erdemir T, Bane AL, Pinnaduwage D, Roncari L, Narimatsu M, Bose R, Moffat J, et al. A role for the TGFbeta-Par6 polarity pathway in breast cancer progression. Proc Natl Acad Sci U S A 2009; 106:14028-33; PMID:19667198; http://dx.doi.org/10.1073/pnas.0906796106
- Hough C, Radu M, Dore JJ. Tgf-beta induced Erk phosphorylation of smad linker region regulates smad signaling. PLoS One 2012; 7:e42513; PMID:22880011; http://dx.doi.org/10.1371/journal.pone.0042513
- Yan X, Chen YG. Smad7: not only a regulator, but also a cross-talk mediator of TGF-beta signalling. Biochem J 2011; 434:1-10; PMID:21269274; http://dx.doi.org/10.1042/BJ20101827
- Lamouille S, Xu J, Derynck R. Molecular mechanisms of epithelial-mesenchymal transition. Nat Rev Mol Cell Biol 2014; 15:178-96; PMID:24556840; http://dx.doi.org/10.1038/nrm3758
- Principe DR, Doll JA, Bauer J, Jung B, Munshi HG, Bartholin L, Pasche B, Lee C, Grippo PJ. TGF-beta: duality of function between tumor prevention and carcinogenesis. J Natl Cancer Inst 2014; 106:djt369; PMID:24511106
- Wendt MK, Tian M, Schiemann WP. Deconstructing the mechanisms and consequences of TGF-beta-induced EMT during cancer progression. Cell Tissue Res 2012; 347:85-101; PMID:21691718; http://dx.doi.org/10.1007/s00441-011-1199-1
- Pickup M, Novitskiy S, Moses HL. The roles of TGFbeta in the tumour microenvironment. Nat Rev Cancer 2013; 13:788-99; PMID:24132110; http://dx.doi.org/10.1038/nrc3603
- Verrecchia F, Mauviel A. Transforming growth factor-beta and fibrosis. World J Gastroenterol 2007; 13:3056-62; PMID:17589920
- Mehner C, Radisky DC. Triggering the landslide: The tumor-promotional effects of myofibroblasts. Exp Cell Res 2013; PMID:23528452
- Radisky DC, Bissell MJ. Matrix metalloproteinase-induced genomic instability. Curr Opin Genet Dev 2006; 16:45-50; PMID:16377172; http://dx.doi.org/10.1016/j.gde.2005.12.011
- Ungefroren H, Lenschow W, Chen WB, Faendrich F, Kalthoff H. Regulation of biglycan gene expression by transforming growth factor-beta requires MKK6-p38 mitogen-activated protein Kinase signaling downstream of Smad signaling. J Biol Chem 2003; 278:11041-9; PMID:12538652; http://dx.doi.org/10.1074/jbc.M300035200
- Verrecchia F, Mauviel A. Transforming growth factor-beta signaling through the Smad pathway: role in extracellular matrix gene expression and regulation. J Invest Dermatol 2002; 118:211-5; PMID:11841535; http://dx.doi.org/10.1046/j.1523-1747.2002.01641.x
- Wang N, Wang X, Sun B, Zeng M, Xing C, Zhao X, Yang J. Role of TGF-beta1 in production of fibronectin in vascular smooth muscle cells cultured under high-phosphate conditions. J Nephrol 2013; 26:213-8; PMID:22641576; http://dx.doi.org/10.5301/jn.5000127
- Stallings-Mann ML, Waldmann J, Zhang Y, Miller E, Gauthier ML, Visscher DW, Downey GP, Radisky ES, Fields AP, Radisky DC. Matrix metalloproteinase induction of Rac1b, a key effector of lung cancer progression. Sci Transl Med 2012; 4:142ra95; PMID:22786680; http://dx.doi.org/10.1126/scitranslmed.3004062
- Zeisberg EM, Potenta S, Xie L, Zeisberg M, Kalluri R. Discovery of endothelial to mesenchymal transition as a source for carcinoma-associated fibroblasts. Cancer Res 2007; 67:10123-8; PMID:17974953; http://dx.doi.org/10.1158/0008-5472.CAN-07-3127
- Radisky DC, Kenny PA, Bissell MJ. Fibrosis and cancer: do myofibroblasts come also from epithelial cells via EMT? J Cell Biochem 2007; 101:830-9; PMID:17211838; http://dx.doi.org/10.1002/jcb.21186
- Li M, Krishnaveni MS, Li C, Zhou B, Xing Y, Banfalvi A, Lombardi V, Akbari O, Borok Z, Minoo P. Epithelium-specific deletion of TGF-beta receptor type II protects mice from bleomycin-induced pulmonary fibrosis. J Clin Invest 2011; 121:277-87; PMID:21135509; http://dx.doi.org/10.1172/JCI42090
- Liu H, Radisky DC, Yang D, Xu R, Radisky ES, Bissell MJ, Bishop JM. MYC suppresses cancer metastasis by direct transcriptional silencing of alphav and beta3 integrin subunits. Nat Cell Biol 2012; 14:567-74; PMID:22581054; http://dx.doi.org/10.1038/ncb2491
- Pechkovsky DV, Scaffidi AK, Hackett TL, Ballard J, Shaheen F, Thompson PJ, Thannickal VJ, Knight DA. Transforming growth factor beta1 induces alphavbeta3 integrin expression in human lung fibroblasts via a beta3 integrin-, c-Src-, and p38 MAPK-dependent pathway. J Biol Chem 2008; 283:12898-908; PMID:18353785; http://dx.doi.org/10.1074/jbc.M708226200
- Yeh YC, Wei WC, Wang YK, Lin SC, Sung JM, Tang MJ. Transforming growth factor-{beta}1 induces Smad3-dependent {beta}1 integrin gene expression in epithelial-to-mesenchymal transition during chronic tubulointerstitial fibrosis. Am J Pathol 2010; 177:1743-54; PMID:20709799; http://dx.doi.org/10.2353/ajpath.2010.091183
- Yeh YY, Chiao CC, Kuo WY, Hsiao YC, Chen YJ, Wei YY, Lai TH, Fong YC, Tang CH. TGF-beta1 increases motility and alphavbeta3 integrin up-regulation via PI3K, Akt and NF-kappaB-dependent pathway in human chondrosarcoma cells. Biochem Pharmacol 2008; 75:1292-301; PMID:18191107; http://dx.doi.org/10.1016/j.bcp.2007.11.017
- Fransvea E, Mazzocca A, Antonaci S, Giannelli G. Targeting transforming growth factor (TGF)-betaRI inhibits activation of beta1 integrin and blocks vascular invasion in hepatocellular carcinoma. Hepatology 2009; 49:839-50; PMID:19115199; http://dx.doi.org/10.1002/hep.22731
- Zhang YJ, Tian ZL, Yu XY, Zhao XX, Yao L. Activation of integrin beta1-focal adhesion kinase-RasGTP pathway plays a critical role in TGF beta1-induced podocyte injury. Cell Signal 2013; 25:2769-79; PMID:24036212; http://dx.doi.org/10.1016/j.cellsig.2013.08.044
- Wang SE, Xiang B, Zent R, Quaranta V, Pozzi A, Arteaga CL. Transforming growth factor beta induces clustering of HER2 and integrins by activating Src-focal adhesion kinase and receptor association to the cytoskeleton. Cancer Res 2009; 69:475-82; PMID:19147560; http://dx.doi.org/10.1158/0008-5472.CAN-08-2649
- Munger JS, Sheppard D. Cross talk among TGF-beta signaling pathways, integrins, and the extracellular matrix. Cold Spring Harb Perspect Biol 2011; 3:a005017; PMID:21900405
- Worthington JJ, Klementowicz JE, Travis MA. TGFbeta: a sleeping giant awoken by integrins. Trends Biochem Sci 2011; 36:47-54; PMID:20870411; http://dx.doi.org/10.1016/j.tibs.2010.08.002
- Alcaraz LB, Exposito JY, Chuvin N, Pommier RM, Cluzel C, Martel S, Lai TH, Fong YC, Tang CH. Tenascin-X promotes epithelial-to-mesenchymal transition by activating latent TGF-beta. J Cell Biol 2014; 205:409-28; PMID:24821840; http://dx.doi.org/10.1083/jcb.201308031
- Mu D, Cambier S, Fjellbirkeland L, Baron JL, Munger JS, Kawakatsu H, Sheppard D, Broaddus VC, Nishimura SL. The integrin alpha(v)beta8 mediates epithelial homeostasis through MT1-MMP-dependent activation of TGF-beta1. J Cell Biol 2002; 157:493-507; PMID:11970960; http://dx.doi.org/10.1083/jcb.200109100
- Barcellos-Hoff MH, Dix TA. Redox-mediated activation of latent transforming growth factor-beta 1. Mol Endocrinol 1996; 10:1077-83; PMID:8885242
- Buscemi L, Ramonet D, Klingberg F, Formey A, Smith-Clerc J, Meister JJ, Hinz B. The single-molecule mechanics of the latent TGF-beta1 complex. Curr Biol 2011; 21:2046-54; PMID:22169532; http://dx.doi.org/10.1016/j.cub.2011.11.037
- Shi M, Zhu J, Wang R, Chen X, Mi L, Walz T, Springer TA. Latent TGF-beta structure and activation. Nature 2011; 474:343-9; PMID:21677751; http://dx.doi.org/10.1038/nature10152
- Giacomini MM, Travis MA, Kudo M, Sheppard D. Epithelial cells utilize cortical actin/myosin to activate latent TGF-beta through integrin alpha(v)beta(6)-dependent physical force. Exp Cell Res 2012; 318:716-22; PMID:22309779; http://dx.doi.org/10.1016/j.yexcr.2012.01.020
- Bader BL, Rayburn H, Crowley D, Hynes RO. Extensive vasculogenesis, angiogenesis, and organogenesis precede lethality in mice lacking all alpha v integrins. Cell 1998; 95:507-19; PMID:9827803; http://dx.doi.org/10.1016/S0092-8674(00)81618-9
- Dickson MC, Martin JS, Cousins FM, Kulkarni AB, Karlsson S, Akhurst RJ. Defective haematopoiesis and vasculogenesis in transforming growth factor-beta 1 knock out mice. Development 1995; 121:1845-54; PMID:7600998
- Yang Z, Mu Z, Dabovic B, Jurukovski V, Yu D, Sung J, Xiong X, Munger JS. Absence of integrin-mediated TGFbeta1 activation in vivo recapitulates the phenotype of TGFbeta1-null mice. J Cell Biol 2007; 176:787-93; PMID:17353357; http://dx.doi.org/10.1083/jcb.200611044
- Henderson NC, Arnold TD, Katamura Y, Giacomini MM, Rodriguez JD, McCarty JH, Pellicoro A, Raschperger E, Betsholtz C, Ruminski PG, et al. Targeting of alphav integrin identifies a core molecular pathway that regulates fibrosis in several organs. Nat Med 2013; 19:1617-24; PMID:24216753; http://dx.doi.org/10.1038/nm.3282
- Hinz B. It has to be the alphav: myofibroblast integrins activate latent TGF-beta1. Nat Med 2013; 19:1567-8; PMID:24309651; http://dx.doi.org/10.1038/nm.3421
- Mamuya FA, Duncan MK. aV integrins and TGF-beta-induced EMT: a circle of regulation. J Cell Mol Med 2012; 16:445-55; PMID:21883891; http://dx.doi.org/10.1111/j.1582-4934.2011.01419.x
- Henderson NC, Sheppard D. Integrin-mediated regulation of TGFbeta in fibrosis. Biochim Biophys Acta 2013; 1832:891-6; PMID:23046811; http://dx.doi.org/10.1016/j.bbadis.2012.10.005
- Serrano I, McDonald PC, Lock FE, Dedhar S. Role of the integrin-linked kinase (ILK)/Rictor complex in TGFbeta-1-induced epithelial-mesenchymal transition (EMT). Oncogene 2013; 32:50-60; PMID:22310280; http://dx.doi.org/10.1038/onc.2012.30
- Truong HH, Xiong J, Ghotra VP, Nirmala E, Haazen L, Le Devedec SE, Balcioğlu HE, He S, Snaar-Jagalska BE, Vreugdenhil E, et al. beta1 integrin inhibition elicits a prometastatic switch through the TGFbeta-miR-200-ZEB network in E-cadherin-positive triple-negative breast cancer. Sci Signal 2014; 7:ra15; PMID:24518294
- Kim KK, Wei Y, Szekeres C, Kugler MC, Wolters PJ, Hill ML, Frank JA, Brumwell AN, Wheeler SE, Kreidberg JA, et al. Epithelial cell alpha3beta1 integrin links beta-catenin and Smad signaling to promote myofibroblast formation and pulmonary fibrosis. J Clin Invest 2009; 119:213-24; PMID:19104148
- Chen X, Wang H, Liao HJ, Hu W, Gewin L, Mernaugh G, Zhang S, Zhang ZY, Vega-Montoto L, Vanacore RM, et al. Integrin-mediated type II TGF-beta receptor tyrosine dephosphorylation controls SMAD-dependent profibrotic signaling. J Clin Invest 2014; 124:3295-310; PMID:24983314
- Vi L, de Lasa C, DiGuglielmo GM, Dagnino L. Integrin-linked kinase is required for TGF-beta1 induction of dermal myofibroblast differentiation. J Invest Dermatol 2011; 131:586-93; PMID:21150927; http://dx.doi.org/10.1038/jid.2010.362
- Asano Y, Ihn H, Jinnin M, Mimura Y, Tamaki K. Involvement of alphavbeta5 integrin in the establishment of autocrine TGF-beta signaling in dermal fibroblasts derived from localized scleroderma. J Invest Dermatol 2006; 126:1761-9; PMID:16675963; http://dx.doi.org/10.1038/sj.jid.5700331
- Asano Y, Ihn H, Yamane K, Jinnin M, Tamaki K. Increased expression of integrin alphavbeta5 induces the myofibroblastic differentiation of dermal fibroblasts. Am J Pathol 2006; 168:499-510; PMID:16436664; http://dx.doi.org/10.2353/ajpath.2006.041306
- Scaffidi AK, Petrovic N, Moodley YP, Fogel-Petrovic M, Kroeger KM, Seeber RM, Eidne KA, Thompson PJ, Knight DA. alpha(v)beta(3) Integrin interacts with the transforming growth factor beta (TGFbeta) type II receptor to potentiate the proliferative effects of TGFbeta1 in living human lung fibroblasts. J Biol Chem 2004; 279:37726-33; PMID:15187087; http://dx.doi.org/10.1074/jbc.M403010200
- Galliher AJ, Schiemann WP. Beta3 integrin and Src facilitate transforming growth factor-beta mediated induction of epithelial-mesenchymal transition in mammary epithelial cells. Breast Cancer Res 2006; 8:R42; PMID:16859511; http://dx.doi.org/10.1186/bcr1524
- Parvani JG, Galliher-Beckley AJ, Schiemann BJ, Schiemann WP. Targeted inactivation of beta1 integrin induces beta3 integrin switching, which drives breast cancer metastasis by TGF-beta. Mol Biol Cell 2013; 24:3449-59; PMID:24006485; http://dx.doi.org/10.1091/mbc.E12-10-0776
- Garamszegi N, Garamszegi SP, Samavarchi-Tehrani P, Walford E, Schneiderbauer MM, Wrana JL, Scully SP. Extracellular matrix-induced transforming growth factor-beta receptor signaling dynamics. Oncogene 2010; 29:2368-80; PMID:20101206; http://dx.doi.org/10.1038/onc.2009.514
- Wendt MK, Schiemann WP. Therapeutic targeting of the focal adhesion complex prevents oncogenic TGF-beta signaling and metastasis. Breast Cancer Res 2009; 11:R68; PMID:19740433; http://dx.doi.org/10.1186/bcr2360
- Reynolds LE, Conti FJ, Lucas M, Grose R, Robinson S, Stone M, Saunders G, Dickson C, Hynes RO, Lacy-Hulbert A, et al. Accelerated re-epithelialization in beta3-integrin-deficient- mice is associated with enhanced TGF-beta1 signaling. Nat Med 2005; 11:167-74; PMID:15654327; http://dx.doi.org/10.1038/nm1165
- Wang D, Sun L, Zborowska E, Willson JK, Gong J, Verraraghavan J, Brattain MG. Control of type II transforming growth factor-beta receptor expression by integrin ligation. J Biol Chem 1999; 274:12840-7; PMID:10212271; http://dx.doi.org/10.1074/jbc.274.18.12840
- Akhurst RJ, Hata A. Targeting the TGFbeta signalling pathway in disease. Nat Rev Drug Discov 2012; 11:790-811; PMID:23000686; http://dx.doi.org/10.1038/nrd3810
- Yingling JM, Blanchard KL, Sawyer JS. Development of TGF-beta signalling inhibitors for cancer therapy. Nat Rev Drug Discov 2004; 3:1011-22; PMID:15573100; http://dx.doi.org/10.1038/nrd1580
- Bogdahn U, Hau P, Stockhammer G, Venkataramana NK, Mahapatra AK, Suri A, Balasubramaniam A, Nair S, Oliushine V, Parfenov V, et al. Targeted therapy for high-grade glioma with the TGF-beta2 inhibitor trabedersen: results of a randomized and controlled phase IIb study. Neuro Oncol 2011; 13:132-42; PMID:20980335; http://dx.doi.org/10.1093/neuonc/noq142
- Oettle H, Seufferlein T. Phase I/II study with trabedersen (AP 12009) monotherapy for the treatment of patients with advanced pancreatic cancer, malignant melanoma, and colorectal carcinoma. J Clin Oncol. 2011; 29:abstract 2513
- Connolly EC, Freimuth J, Akhurst RJ. Complexities of TGF-beta targeted cancer therapy. Int J Biol Sci 2012; 8:964-78; PMID:22811618; http://dx.doi.org/10.7150/ijbs.4564
- Cox D, Brennan M, Moran N. Integrins as therapeutic targets: lessons and opportunities. Nat Rev Drug Discov 2010; 9:804-20; PMID:20885411; http://dx.doi.org/10.1038/nrd3266
- Goodman SL, Picard M. Integrins as therapeutic targets. Trends Pharmacol Sci 2012; 33:405-12; PMID:22633092; http://dx.doi.org/10.1016/j.tips.2012.04.002
- Ning S, Tian J, Marshall DJ, Knox SJ. Anti-alphav integrin monoclonal antibody intetumumab enhances the efficacy of radiation therapy and reduces metastasis of human cancer xenografts in nude rats. Cancer Res 2010; 70:7591-9; PMID:20841470; http://dx.doi.org/10.1158/0008-5472.CAN-10-1639
- O'Day S, Pavlick A, Loquai C, Lawson D, Gutzmer R, Richards J, Schadendorf D, Thompson JA, Gonzalez R, Trefzer U, et al. A randomised, phase II study of intetumumab, an anti-alphav-integrin mAb, alone and with dacarbazine in stage IV melanoma. Br J Cancer 2011; 105:346-52; PMID:21750555; http://dx.doi.org/10.1038/bjc.2011.183