Abstract
Two scenarios of neuronal evolution (monophyly and polyphyly) are discussed in the historical timeline starting from the 19th century. The recent genomic studies on Ctenophores re-initiated a broad interest in the hypotheses of independent origins of neurons. However, even earlier work on ctenophores suggested that their nervous systems are unique in many aspects of their organization and a possibility of the independent origin of neurons and synapses was introduced well before modern advances in genomic biology.
In light of recent publicationsCitation1-8 and a growing interest to discover the origins of nervous system it is important to remember that the problem has been repeatedly addressed through the entire history of neuroscience.Citation9-17 Unfortunately, some of the earliest papers on the topic are less accessible today and, sometimes, they are forgotten. Here, I would like to briefly summarize several previous publications proposing the independent origins of nervous systems and synapses.
There are 2 classical scenarios related to the evolution of nervous systems and neurons: Monophyly and Polygenesis. The monophyly hypothesis states that neurons arose from a single ancestral cell lineage and in a common ancestor of all animals with neurons. The genealogy of the brain can be traced back to ancestral central nervous systems (CNS) or a centralized “nerve cord” present in the common ancestor of bilaterian animals (Urbilateria). The main arguments supporting this hypothesis are summarized elsewhere.Citation18-20 Traditionally, the idea of monophyly is derived from the classical Darwinian concept of the “last common ancestor”. At the level of species and organisms this concept constitutes the foundation of biology and phylogeny. However, at the level of specific cells, cell populations, or tissues finding a common predecessor or single ancestral cellular lineage for neurons, or muscles across phyla is problematic. As a result, the framework of evolution of cell lineages was introducedCitation21 but it was not systematically developed in the context of the monophyletic nervous system origin.
The polygenesis hypothesis refers to the polyphyletic origin (Polyphyly), or independent origins of neurons and complex brains among species in different clades.Citation2,3,22 Thus, similar neuronal phenotypes or CNSs are results of the convergent or parallel evolution (see discussion about the terminology inCitation23-25). The combination of both taxon-specific novelties and multiple recruitments of evolutionarily conserved regulatory modules might have contributed to the origin of neurons and formation of neural circuits from genealogically unrelated, or very distantly related, cell lineages. Historically, polyphyly has been the less popular scenario of neuronal evolution but emerging comparative and genomic dataCitation4,5 provide a conceptual challenge to the single-origin hypothesis. Nevertheless, various lines of evidence supporting alternative evolutionary scenarios have been considered for more than a century.
Early Hypotheses of Neuronal Origins
One of the first recorded theories on the origin of neurons was presented by Nicolaus Kleinenberg, a German biologist who had obtained a doctorate degree under Ernst Haeckel. In his anatomical-evolutionary investigation of Hydra,Citation26 Kleinenberg had discovered polarized cells with 2 processes: one “sensory” process toward the environment, and the second with a possible motor function. Kleinenberg designated these cells as “neuromuscular” and suggested they might represent an ancestral ‘hybrid stage’ that gave rise to both pure neuronal and pure muscular cell types. He hypothesized that these polarized cells might have all the required components of a reflex-type circuit (‘the nervous arc’) – the receptor, conductor and effector. These cells were later identified as epithelio-muscular cells. In Kleinenberg's time, the reflex was the dominant paradigm used to describe cellular bases of behavior. We might consider Kleinenberg's hypothesis as the ultimate extension of Monophyly, suggesting a common cell lineage ancestor (i.e. myo-epithelial conductive cells) for all neurons, sensory cells and muscles.
At about the same time, a second hypothesis of neuronal origin was also introduced, by 2 other students of Haeckel. This alternative view can be interpreted in general terms of Polygenesis. Their starting point was a reflex-type arc with 3 components that acted as a functional neural circuit. Brothers Oscar and Richard Hertwig, working on the nervous systems of medusasCitation27, suggested that the receptor, conductor and effector of a simplified circuit arose as separate types of cells from distinct epithelial cell lineages. The Hertwig brothers' report focused on the separate origins of functionally different but physiologically interacting cell types such as specialized sensory cells, conducting neurons or muscle cells. Similar ideas were introduced by ClausCitation28 and Chun,Citation29 working on medusas and ctenophores, respectively. Claus and Chun also proposed that following their independent origins, nerve and muscle cells became associated only secondarily.
The most widely acknowledged systematic analysis on the origin of nervous systems was presented by George Howard Parker in his book “The Elementary Nervous Systems.”Citation11 A nerve reflex arc and incremental grades of animal organization were conceptual points in his reconstructions. Parker distinguished 3 separate stages in the origin of major components of neuronal organization such as (i) the origin of independent effectors (e.g. muscle and gland cells); (ii) origin of sensory organs (or receptors forming receptor-effector system); and (iii) the raise of adjustors (or central nervous organs). He recognized the first stage in extant sponges that possessed only effectors but not receptors or adjustors. The second stage could be potentially observed in Coelenterates that can form receptor-effector systems. The next stage is the development of an intermediate (adjuster/or central neural organ) between receptor and effector; typically characteristic of more complex systems in invertebrates and vertebrates. In these earlier works adjusters can be related to what we now call neurons and nervous systems.
Parker's hypothesis incorporated incremental steps in the origin of complex system, with circuits arising from sensory neurons/receptors, motoneurons/effectors and interneurons within the nervous system itself. Receptors arose by the modification of epithelial cells in close proximity to already differentiated muscle cells and were able to make direct connections to muscle cells. Muscles were considered as the major effectors. This hypothetical, receptor-muscle, stage has not been clearly defined in extant cnidarians or other metazoans whereas advance stages with neuronal elements (or adjusters) are widely represented across all animals with nervous systems. According to Parker the nervous systems in coelenterates (cnidarians + ctenophores) form diffuse nerve nets that are not centralized; signal transmission is diffusely spread through the animal's body. The appearance of polarized transmission in circuits mediated by synapses was considered the essential leap toward the origin of a true central nervous system.Citation13
In these hypotheses and in many that succeed them,Citation30-35 electrical/circuit integration and electrical coupling were viewed as key driving forces of neuronal evolution. The chemical messengers or transmitters, or more accurately — the diversity of transmitters — were not recognized as inherent components of the circuits. This is understandable since the enormous diversity of classical low molecular weight transmitters and neuropeptides was discovered only later in the 20th century (1950–1980s). Nothing was known at that time about the molecular or modular organization of neurons and synapses. It could be stated that the motto of all approaches and mechanisms associated with neural evolution was: “Excitability and electrical conductance.” Neurons were perceived as simple and similar conducting units. The enormous molecular heterogeneity of neurons, transmitters and synapses, as is currently recognized, was virtually unknown till the end of the 20th century.
In the 1950s–1960s Parker's hypothesis was modified by PantinCitation30 and PassanoCitation31 who introduced integrative and pacemaker components into early neural circuits and stressed the importance of endogenous activity in neurons beyond the framework of a simplified reflex arc. In fact, the ‘reflex arc’ was presented as a simplificationCitation30 or optimizationCitation35 of parts of an initially more complex system.
It was established that coelenterate circuits, as in all other nervous systems, are polarized with defined chemical synapses. Some of coelenterate synapses were also found to be symmetrical, supporting the former description of diffuse nerve net architecture in cnidarian and ctenophores.Citation36,37 Subsequently, it was discovered that at least some cnidarian nervous systems consist of several relatively independent nerve nets allowing well-coordinated and sometimes complex behaviors.Citation33 Recent reviews have even introduced the concept of cnidarian central nervous system.Citation38
To reiterate, electrical coordination and coupling was at the core of these earlier theories of neuronal evolution. Pantin proposed that a nervous system originated as complex circuits innervating various motor units to produce a coordinated behavior in an animal.Citation30 Passano had suggested that neurons arose from pacemaker-type cells that might derive from pro-myocytes and function to generate contractions in pro-myocytes, and later in true muscle cells.Citation31 Mackie further developed this idea suggesting that a nervous system might be derived from electrically coupled primordial myoepithelial-like cells that were capable of reception, transmission and contractions.Citation32 Pro-myocytes eventually segregated from these primordial cells, and protoneurons were evolved from the same epithelial cells (that lost their contractile ability) in order to excite myocytes, and possibly other cell types. Neurosecretory cells evolved to transmit polarized signals from sensory cells/neurons to myocytes using chemical synapses. Westfall proposed that receptive, electrogenic and neurosecretory functions co-evolved in earlier protoneurons.Citation39,40 In summary, the relationships between cell types were not clearly defined; cnidarians and their myoepithelial cells were considered as the stage of neuronal evolution close to the ancestral conditions.
Ctenophores were originally grouped with cnidarians into the clade Coelenterata, and so they are less studied. G. A. Horridge was one of the most persistent investigators who seriously considered ctenophores as a distinct and very important reference group in studies of neuronal evolution. Not surprisingly, his suggestions were provocative, even at the very beginning of his research. As summarized by these early extensive studies on ctenophoresCitation41: “There are no 2-neuron arcs in any single reflex. Sensory cells apparently activate a region of the nerve net, from which there are numerous branches to many muscle fibers. This suggests that neurons did not evolve singly but in aggregation of cells that act in unison.”Citation42
At this time, the information about chemical (and even functional) heterogeneity of neuronal populations in cnidarians/ctenophores was very limited and the term “neuron” was quite generalized, primarily assigned to cells with electrical propertiesCitation42 such as conductor or pacemaker. The placozoans (Trichoplax),Citation43,44 a distinct lineage of pre-bilaterian animals lacking nervous and muscular systems, were not considered in any of these earlier reconstructions.
Ancestral Secretory Cells as Evolutionary Precursors of Neurons
With the discovery of chemical messengers as synaptic transmitters, growing evidence suggested that even the “primitive” nervous systems of cnidarians might use similar molecules as intercellular messengers. Haldane proposed that (neuro)transmitters and hormones originated in unicellular organisms.Citation45 Sequentially, a diversity of transmitter-like substances were discovered in plants and prokaryotes.Citation17,46
Grundfest introduced an imperative hypothesis that neurons arose from ancestral secretory cells.Citation47,48 The ancestral cell could be a sensory cell that developed specialized receptive surfaces and separate secretory poles. Over time, “the receptive and secretory portions of the cells gradually were displaced but remained connected by a region with conductile properties. The development of long processes terminating near blood vessels or on other cell types led to differentiation of neurosecretory cells. Neurons were formed when the secretory activity became confined to the termination of the processes.Citation13” Similar ideas were developed by Clark, again stressing that neurosecretory cells originated from secretory epithelial cells.Citation49,50 Collectively, these authors introduced the idea that “secretion is a primitive feature of the nervous system.”
In the 1970s Dmitry A. Sakharov was studying the diversity of transmitter phenotypes and crystallized the idea of polygenesis of neurons. In his reconstructions, the diversity of transmitters is a consequence of the independent origins and parallel evolution of different neuronal lineages that preserved their ancestral type of transmitter specificity.Citation14,51-54 Sakharov also viewed transmitter specificity (or equivalently, secretory specificity) as one of the most evolutionarily conserved characteristics of neurons. He was the first person to utilize the apparent conservation of transmitter phenotypes and modified Remane's criteria (‘Positional’, ‘Structural’, Transitional’Citation55,56) to identify individual homologous neurons in several gastropod lineages; including characterizing homologous dopaminergic, serotonergic and peptidergic neurons in Lymnaea, Helix, Aplysia, Clione and Tritonia.Citation14 To put simply, neurons with different transmitter/secretory specificity are different because they had different origins and genealogies. In what is perhaps the most straightforward corollary of Sakharov's neuronal polygenesis, is the scenario that, for example, the serotonergic and dopaminergic neurons might have evolved from different pre-neuronal/ancestral cell lineages. Different molecules might be independently recruited to support intercellular signaling functions. Even terminology can be adjusted because there are many cases when classical (neuro)transmitters are present in non-neuronal cells. For example, the majority of serotonin in our body is located in mast cells rather in neurons.Citation57-59 Thus, a more correct term would be a transmitter (as a chemical mediator of signaling between cells) rather than neurotransmitter (the term which refers to a more specialized situation where the chemical signaling occurs between neurons only).
Do all Animals have the same Transmitters?
From the dawn of the discovery of transmitters in neuroscience, it was repeatedly confirmed that a diverse repertoire of classical transmitters was present in both vertebrates and invertebrates. Indeed, transmitters initially discovered in invertebrates (such as GABA and serotonin) have proved to mediate chemical signaling between vertebrate neurons as well as other cell types. Moreover, the genes encoding relevant enzymes in synthesis pathways for most transmitters are highly conserved between bilaterians and cnidarians. Thus, in my first comparative studies on ctenophores, I anticipated that they would share of the majority of their neurotransmitter organization with other Eumetazoa (i.e., animals with neurons), along with the majority of cnidarian-bilaterian neurogenic and synapse-related genes.
However, when our group performed extensive genomic, transcriptomic, metabolomic and functional analyses on several ctenophore species, we were very surprised to find that representatives of this lineage of basal metazoans are “missing” the majority of the essential components of canonical neuronal signaling.Citation4,5 In contrast to all other animals with nervous systems, it appears that ctenophores do not use the majority of known canonical low molecular weight transmitters (e.g., acetylcholine, serotonin, dopamine, noradrenaline, adrenaline, octopamine, histamine or glycine) as intercellular messengers. These findings are consistent with the hypothesis that ctenophore neural systems evolved independently () from those in the cnidarian-bilaterian clade and that they evolved a different subset of inter-neuronal signaling molecules (e.g. small secretory peptides). In addition, many bilaterian genes controlling neuronal fate and patterning are absent in the sequenced genomes and transcriptomes of ctenophores.Citation5 Orthologs of many eumetazoan (i.e. organisms with nervous systems) pre-and postsynaptic genes critical for synaptic functions are also absent or have a reduced representation in ctenophores. In summary, ctenophores might use a remarkably different chemical language in their interneuronal communications.Citation4
Figure 1. Two alternative scenarios of neuronal evolution (Ctenophora-basal hypothesis). The polyphyly or multiple origins of neurons as the example of convergent evolution (left). Monophyly or the single-origin hypotheses implies multiple loss of neural systems in sponges and placozoans as well as massive loss of many molecular components involved in neurogenesis and synaptic functions (right). The monophyly hypothesis still implies independent recruitment of other molecular components involved in neural and synaptic functions—the situation which still suggests the extensive parallel evolution of neural organization in ctenophores. Here, ctenophores are considered as sister to other animals (modified fromCitation5). However, even the classical view of the animal phylogeny (sponges are sisters to other animals, see ) still implies the parallel evolution of neurons and neural signaling in the animal kingdom.
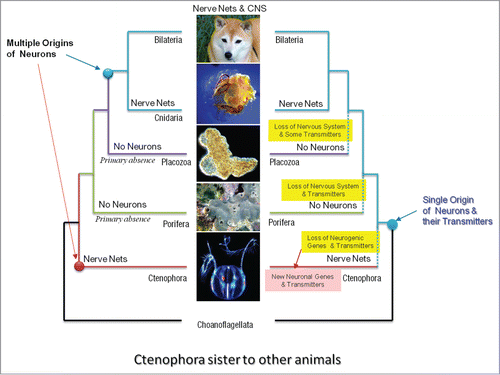
There are 2 scenarios that might explain this situation. Both scenarios are compatible with 2 popular hypotheses of the early animal phylogeny: Ctenophora is the sister group to all Metazoa () vs Porifera is the sister to all other animals ().
Figure 2. Two alternative scenarios of neuronal evolution (Porifera-basal hypothesis). The polyphyly or multiple origins of neurons as the example of convergent evolution (left). There is a primary absence of neurons in sponges; independent origins of neurons in both ctenophora and cnidaria-bilateria clade. Monophyly or the single-origin hypotheses implies (as in ) loss of neural systems in ctenophores and placozoans as well as massive loss of many molecular components involved in neurogenesis and synaptic functions (right). The monophyly hypothesis also implies the independent recruitment of novel molecular components involved in neural and synaptic functions in Ctenophora - the situation which still suggests the extensive parallel evolution of neural organization in this animal lineage. Here, ctenophores are considered as the second branching animal clade; whereas sponges are viewed as sister to other animals (see for the alternative phylogeny).
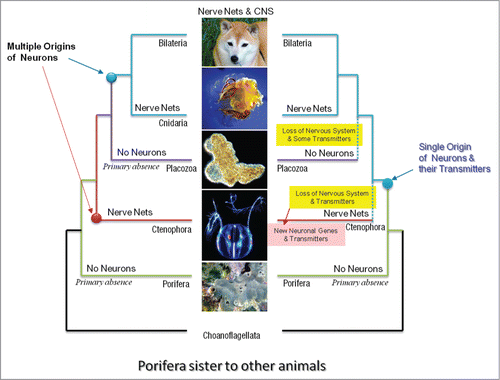
The first scenario is consistent with the most commonly accepted view of a single origin of neurons (monophyly): the common ancestor of ctenophores shared the same transmitters and neurogenic genes as the extant members of the bilaterian-cnidarian clade. In the course of evolution, for unknown reasons, ctenophores subsequently lost most of these genes and replaced most of low molecular weight transmitters by new types of signaling molecules. However, ctenophores are active marine predators with complex behaviors. It is unclear what past events or factors of natural selection would favor the loss of such complex transmitter signaling and neurogenic machinery in free-living (not parasitic!) ctenophores, especially since such cellular machinery is highly conserved in other eumetazoans lineages?
The second scenario assumes the polygenesis of neurons ( and ). Neurons, as secretory cells, evolved independently in ctenophoresCitation4,5 and recruited a largely different subset of secretory molecules for interneuronal signaling. Thus, the dramatically different chemical language in ctenophore nervous systems is a reflection of their extensive parallel or convergent evolution. A corollary of the independent origin of neurons from ancestral secretory cells is the convergent evolution of synapses and synaptic signaling. Indeed, the eukaryotic exocytosis machinery (formation and the release of secretory vesicles) can independently recruit various uptake systems (transporters) and receptor proteins. Subsequently, this process would lead to development of a number of cell lineages with various classes of secretory specificity – ancestors of extant synaptic and neuronal classes.
It might be of interest to note that Horridge discussed similar ideas long ago as a result of his ultra-structural studies of ctenophore synapses which caused him to consider the physical constraints of synaptic transmission in general. “Therefore, the anatomy of a synapse follows from the physical properties of its components, so that anatomical synapses of similar appearance in different groups of animals could be the result of convergent evolution.” Citation41
For any modern evolutionary reconstruction, it would be important to revisit our present and historical definition of neuronsCitation2,12,35,42,60-62 and integrate it with the broad diversity of comparative data. Neurons may be most accurately viewed as polarized secretory cells specialized for the directional propagation of electrical signals which lead to the release of extracellular messengers at terminal processes – features that enable them to transmit signals, primarily chemical in nature, beyond their immediate neighbors without affecting all intervening cells en route. Here, the diversity of chemical/transmitter-mediated signaling is the major attribute of neural circuits. It is the major trait of any nervous system – the characteristic that extend the earlier “electrical” definition of a neuron by Horridge.Citation42 Importantly, a generalized term “neuron” appears to be the functional rather than a genetic category. The enormous molecular/secretory heterogeneity of neurons might have roots in their deep and complex genealogy. Various transmitters could be originally ‘used’ to mediate a polarized secretion as a part of the adaptive response to a localized injury.Citation2 Following injury, every cell releases a diversity of molecules (polar amino acids, ATP, nitric oxide, protons, short peptides, hormones, growth factors, etc.). Some of them may act as early signals for repair and regeneration (and still preserve these ancestral functions in extant animals). Such events might occur multiple times, and in many cell populations. Occasionally, these molecules (‘future” (neuro)transmitters) and more specialized secretory machinery could be independently recruited by different proto-neuronal cell lineages to mediate both fast and slow coordinated behavioral/developmental/metamorphosis responses to environmental stress factors. The Cambrian explosion can be linked to large-scale perturbations of global ecosystems during Ediacaran; and a relatively short period of the Cambrian explosionCitation63 could provide the environmental framework for both rapid speciation events and associated injury-induced neurogenesis in pre-bilaterian animals.
We can find the broad spectrum of very different neurons and synapses in more than 30 currently recognized clades of animals, and use them to reconstruct ancestral cell lineages and relevant molecular toolkits. The central nervous systems of insects and vertebrates have the extreme examples of neuronal specification and highly localized synapses. In contrast, there are a number of small neuronal-like cells with short processes in marine invertebrate larvae – the cells which are morphologically very different from canonical vertebrate neurons. In mollusks and many other invertebrates, certain classes of (neuro)secretory cells have no defined synaptic cleft and postsynaptic membrane; and these cells secrete peptides directly into their open circulatory systems. Additionally, a broad spectrum of proneural classes, representing various ancestral cell lineages, might have persisted for over 600 million years of biological evolution.
In conclusion, I would like to finish this historical overview with an extract of a discussion between 2 masters of comparative neurobiology. This discussion occurred more than 50 years ago discerning the definition of neurons in ctenophores.
“G. O. Mackie: I am interested in the 2 types of cells in the ciliated groove. Both appear to conduct but you call one of them nerves and you say that the other conducts in a ‘neuroid’ fashion. Where do you draw the line between nerve cells and ‘neuroid’ conducting cells?
G. A. Horridge: The epithelial cells have grown elongated and parallel. They conduct over long distances and resemble nerve cells but happen to be ciliated. I would call this an independent origin of a nerve cell, but the whole definition of nerves is in question. As soon as you trace the origin of any category down to its simplest limits you find that your definitions become arbitrary. If you remove stones from a heap until you have 4 left, is that still a heap? If you remove another and you have 3, is that a heap? When you have only 2 left, that is probably not a heap. Similarly, when you discover progressively more elementary nervous system or follow any structure in the animal kingdom down to its simplest limits you find that your definitions are no longer simple.” - cited from.Citation64
Disclosure of Potential Conflicts of Interest
No potential conflicts of interest were disclosed.
Acknowledgments
The author thanks Caleb Bostwick and Yelena Moroz Alpert for their editing and comments to the manuscript. The title of the manuscript is inspired by the book “Genealogy of Neurons”, D. A. Sakharov,1974.
Funding
This work was supported by NSF-0744649 and NSF CNS-0821622; NIH 1R01GM097502, R01MH097062, R21RR025699 and 5R21DA030118; NASA NNX13AJ31G grants; McKnight Brain Research Foundation, University of Florida Opportunity Funds and Florida Biodiversity Institute collection funds.
References
- Miller, G. Origins. On the origin of the nervous system. Science 2009; 325:24-26; PMID:19574364; http://dx.doi.org/10.1126/science.325_24
- Moroz, LL. On the independent origins of complex brains and neurons. Brain Behav Evol 2009; 74:177-190; PMID:20029182; http://dx.doi.org/10.1159/000258665
- Moroz, LL. Phylogenomics meets neuroscience: how many times might complex brains have evolved? Acta Biol Hung 2012; 63Suppl 2:3-19; PMID:22776469; http://dx.doi.org/10.1556/ABiol.63.2012.Suppl.2.1
- Moroz, LL. Convergent evolution of neural systems in Ctenophores. J Exp Biol in press 2015
- Moroz, L. L. Kocot KM, Citarella MR, Dosung S, Norekian TP, Povolotskaya IS, Grigorenko AP, Dailey C, Berezikov E, Buckley KM et al. The ctenophore genome and the evolutionary origins of neural systems. Nature 2014; 510:109-114; PMID:24847885; http://dx.doi.org/10.1038/nature13400
- Pennisi E. Nervous system may have evolved twice. Society for integrative and comparative biology annual meeting. Science 2013; 339:391; PMID:23349266; http://dx.doi.org/10.1126/science.339.6118.391-a
- Rokas A. My oldest sister is a sea walnut? Science 2013; 342:1327-1329; PMID:24337283; http://dx.doi.org/10.1126/science.1248424
- Ryan TJ, Grant SG. The origin and evolution of synapses. Nat Rev Neurosci 2009; 10, 701-712; PMID:19738623; http://dx.doi.org/10.1038/nrn2717
- Darwin C. The Expression of the Emotions in Man and Animals John Murray, 1872
- Jennings HS. Behavior of the Lower Organisms 1-366 Macmillan, 1906.
- Parker GH. The Elementary Nervous Systems. Lippincott, 1919.
- Bullock TH, Horridge GA. Structure and Function in the Nervous Systems of Invertebrates. Vol. 1-2, 1719 Freeman, 1965.
- Lentz TL. Primitive Nervous Systems. Yale University Press, 1968.
- Sakharov DA. Genealogy of Neurons (in Russian). Nauka, 1974.
- Greenspan RJ. An Introduction to Nervous Systems. CSHL Press, 2007.
- Binet A. The Psychic Life of Micro-Organisms: A Study in Experimental Psychology. 1-121 The Open Court Publishing Company, 1894.
- Baluska F, Mancuso S. Deep evolutionary origins of neurobiology: turning the essence of 'neural' upside-down. Commun Integr Biol 2009; 2:60-5; PMID:19513267; http://dx.doi.org/10.4161/cib.2.1.7620
- Arendt D, Denes AS, Jekely G, Tessmar-Raible K. The evolution of nervous system centralization. Philos Trans R Soc Lon B Biol Sci. 2008; 363:1523-8; PMID:18192182; http://dx.doi.org/10.1098/rstb.2007.2242
- Hirth F, Kammermeier L, Frei E, Walldorf U, Noll M, Reichert H. An urbilaterian origin of the tripartite brain: developmental genetic insights from Drosophila. Development 2003; 130:2365-73; PMID:12702651; http://dx.doi.org/10.1242/dev.00438
- Hirth F, Reichert H. in Evolution of Nervous Systems Vol. 1 ed JH Kaas 55-72 Academic Press, 2007.
- Arendt D. The evolution of cell types in animals: emerging principles from molecular studies. Nature Rev Genet. 2008; 9:868-82; PMID:18927580; http://dx.doi.org/10.1038/nrg2416
- Holland ND. Early central nervous system evolution: an era of skin brains? Nat Rev Neurosci 2003; 4:617-27; PMID:12894237; http://dx.doi.org/10.1038/nrn1175
- Arendt J, Reznick D Convergence and parallelism reconsidered: what have we learned about the genetics of adaptation? Trends Ecol Evol 2008; 23:26-32; PMID:18022278; http://dx.doi.org/10.1016/j.tree.2007.09.011
- Leander BS. Different modes of convergent evolution reflect phylogenetic distances: a reply to Arendt and Reznick. Trends Ecol Evol 2008; 23:481-82; author reply 483-484; PMID:18657879; http://dx.doi.org/10.1016/j.tree.2008.04.012
- Stern DL. The genetic causes of convergent evolution. Nature Revi 2013; 14:751-64; PMID:24105273; http://dx.doi.org/10.1038/nrg3483
- Kleinenberg N. Hydra - Eine anatomisch-entwicklungsgeschichtliche untersuchung An Anatomical-Evolutionary Investigation of Hydra. Wilhelm Engelmann, 1872
- Hertwig O, Hertwig R. Das Nervensystem und die Sinnesorgane der Medusen The Nervous System and the Sensory Organs of the Medusa. Vogel, 1878
- Claus C. Studien uber Polypen und Quallen der Ardia. Denkschr Acad Wiss Wien 1878; 1-64
- Chun C. Die Ctenophoren des Golfes von Neapel. Fauna Flora Neapel, Monogr 1880; 1-313
- Pantin CFA. The origin of the nervous system. Pubbl Staz Zool Napoli 1956; 28:171-81
- Passano, LM. Primitive Nervous Systems. Proc Natl Acad Sci U S A 1963; 50:306-13; PMID:14060649; http://dx.doi.org/10.1073/pnas.50.2.306
- Mackie GO. Neuroid conduction and the evolution of conducting tissues. The Quarterly Rev Biol 1970; 45:319-32; PMID:4395914; http://dx.doi.org/10.1086/406645
- Mackie GO. The elementary nervous systems revisited. American Zoologist 1990; 30:907-20
- Cook ND, Carvalho GB, Damasio A. From membrane excitability to metazoan psychology. Trends Neurosci S0166-2236(14)00128-3 2014; 37:698-705; PMID:25176475; http://dx.doi.org/10.1016/j.tins.2014.07.011
- Keijzer F, van Duijn M, Lyon P. What nervous systems do: early evolution, input-output, and the skin brain thesis. Adaptive Behavior 2013; 21:67-85; DOI: 10.1177/1059712312465330
- Anderson PA. Physiology of a bidirectional, excitatory, chemical synapse. J Neurophysiol 1985; 53:821-835; PMID:2984356
- Anderson PA, Grunert U. Three-dimensional structure of bidirectional, excitatory chemical synapses in the jellyfish Cyanea capillata. Synapse 1988; 2:606-13; PMID:2905537; http://dx.doi.org/10.1002/syn.890020605
- Satterlie RA. Do jellyfish have central nervous systems? J Exp Biol 2011; 214:1215-23; PMID:21430196; http://dx.doi.org/10.1242/jeb.043687
- Westfall JA. Ultrastructural evidence for a granule-containing sensory-motor-interneuron in Hydra littoralis. J Ultrast Res 1973; 42:268-82; PMID:4702922; http://dx.doi.org/10.1016/S0022-5320(73)90055-5
- Westfall JA, Kinnamon JC. A second sensory–motor–interneuron with neurosecretory granules in Hydra. J Neurocytol 1978; 7:365-79; PMID:207827; http://dx.doi.org/10.1007/BF01176999
- Horridge GA. in Coelenterate Biology eds L Muscatine, HM Lenhoff 439-68 Academic Press, 1974
- Horridge GA. in Structure and Function of Nervous Tissue ed GH Bourne 1-33 Academic Press, 1968
- Jorgensen EM. Animal evolution: looking for the first nervous system. Curr Biol 2014; 24:R655-8; PMID:25050965; http://dx.doi.org/10.1016/j.cub.2014.06.036
- Smith C L., Varoqueaux F, Kittelmann M, Azzam RN, Cooper B, Winters CA, Eitel M, Fasshauer D, Reese TS. Novel cell types, neurosecretory cells, and body plan of the early-diverging metazoan Trichoplax adhaerens. Curr Biol 2014; 24:1565-72; PMID:24954051; http://dx.doi.org/10.1016/j.cub.2014.05.046
- Haldane JBC. La signalization animale. Anne Biol 1954; 58:89-98
- Baluska F. Recent surprising similarities between plant cells and neurons. Plant Signal Behav 2010; 5:87-9, ; PMID:20150757; http://dx.doi.org/10.4161/psb.5.2.11237
- Grundfest H. in Evolution of Nervous Control from Primitive Organisms to Man ed AD Bass 43-86 American Association for Advancement of Science, 1959
- Grundfest H. in Essays on Physiological Evolution ed JWS Pringle 107-38 Pergamon Press, 1965
- Clark RB On the transformation of neurosecretory cells into ordinary nerve cells. Fysiogr Sallsk Lund, Forth 1956; 26:82-89
- Clark RB. On the origin of neurosecretory cells. Ann Sci Nat Zool 1956; 18:199-207
- Sakharov DA. Cellular aspects of invertebrate neuropharmacology. Annu Rev Pharmacol 1970; 10:335-52; PMID:4315688; http://dx.doi.org/10.1146/annurev.pa.10.040170.002003
- Sakharov DA. Principal approaches to the systematization of nerve cells (in Russian). Zh Obsch Biol 1970; 31:449-457; PMID:5492133
- Sakharov DA. Why are neurons different? (in Russian). Priroda 1972; 52-62.
- Sakharov DA Evolutionary aspects of transmitter heterogeneity. J Neural Transm 1974; Suppl 11:43-59; PMID:4154076
- Zachos FE , Hossfeld U. Adolf Remane (1898-1976) and his views on systematics, homology and the modern synthesis. Theory Biosci 2006; 124:335-48; PMID:17046364; http://dx.doi.org/10.1016/j.thbio.2005.09.006
- Hossfeld U, Olsson L The history of the homology concept and the "Phylogenetisches Symposium”. Theory Biosci 2005; 124:243-53; PMID:17046358; http://dx.doi.org/10.1007/BF02814486
- Buhner S, Schemann M. Mast cell-nerve axis with a focus on the human gut. Biochim Biophys Acta 2012; 1822:85-92, ; PMID:21704703; http://dx.doi.org/10.1016/j.bbadis.2011.06.004
- Nautiyal KM, Dailey CA, Jahn JL, Rodriquez E, Son NH, Sweedler JV, Silver R. Serotonin of mast cell origin contributes to hippocampal function. Eur J Neurosci 2012; 36:2347-59); PMID:22632453; http://dx.doi.org/10.1111/j.1460-9568.2012.08138.x
- Yong LC. The mast cell: origin, morphology, distribution, and function. Exp Toxicol Pathol 1997; 49:409-24; PMID:9495641; http://dx.doi.org/10.1016/S0940-2993(97)80129-7
- Guillery RW. Observations of synaptic structures: origins of the neuron doctrine and its current status. Philos Trans R Soc Lond B Biol Sci 2005; 360:1281-1307; PMID:16147523; http://dx.doi.org/10.1098/rstb.2003.1459
- Guillery RW. Relating the neuron doctrine to the cell theory. Should contemporary knowledge change our view of the neuron doctrine? Brain Res Rev 2007; 55:411-21; PMID:17300841; http://dx.doi.org/10.1016/j.brainresrev.2007.01.005
- Bullock TH, Bennett MV, Johnston D, Josephson R, Marder E, Fields RD Neuroscience. The neuron doctrine, redux. Science 2005; 310:791-793; PMID:16272104; http://dx.doi.org/10.1126/science.1114394
- Erwin DH, Valentine JW. The Cambrian Explosion. The construction of animal biodiversity. 406 Roberts and Company, 2013
- Horridge GA. in The Cnidaria and their Evolution Synposia of the Zoological Society of London. Number 16 ed JV Rees 247-66 Academic Press Inc. (London) Ltd, 1966