Abstract
Among all the strategies displayed by fungi to reproduce and propagate, some species have adopted a peculiar behavior called pseudo-homothallism. Pseudo-homothallic fungi are true heterothallics, i.e., they need 2 genetically-compatible partners to mate, but they produce self-fertile mycelium in which the 2 different nuclei carrying the compatible mating types are present. This lifestyle not only enables the fungus to reproduce without finding a compatible partner, but also to cross with any mate it may encounter. However, to be fully functional, pseudo-homothallism requires maintaining heterokaryosis at every stage of the life cycle. We recently showed that neither the structure of the mating-type locus nor hybrid-enhancing effect due to the presence of the 2 mating types accounts for the maintenance of heterokaryosis in the pseudo-homothallic fungus P. anserina. In this addendum, we summarize the mechanisms creating heterokaryosis in P. anserina and 2 other well-known pseudo-homothallic fungi, Neurospora tetrasperma and Agaricus bisporus. We also discuss mechanisms potentially involved in maintaining heterokaryosis in these 3 species.
To be able to colonize nearly all ecosystems on earth, fungi have developed many dispersal strategies, including the lack of all kinds of dispersal propagules (mycelia sterilia), exclusive asexual reproduction, exclusive sexual reproduction and finally both asexual and sexual reproductions depending upon internal (e.g., age) or external stimuli (e.g., medium composition, temperature, light…). For those practicing sexual reproduction, the choice of the mating partner may also follow various modalities.Citation1 Many species are called heterothallic, i.e., 2 individuals of compatible mating-types need to meet for the sexual reproduction to occur. Compatible mating may be under the control of one locus (bipolar mating types), often with only 2 alternatives, or 2 loci (tetrapolar mating types), each locus with 2 or more alternatives leading to from 4 to potentially thousands of different mating-types. In other species, 2 genetically-identical nuclei can fuse and undergo meiosis; these self-fertile species are called homothallic. However, for these species lacking mating type, compatibility is in fact universal, meaning that any individual can fertilize any other one.Citation1,2 An interesting observation is that some truly heterothallic fungi are bypassing their obligation to find a proper mate, mimicking homothallics. They may do so by undergoing mating type switching such as in the brewer's and fission yeastsCitation3,4 and some filamentous ascomycetes.Citation5 An alternative method is presented by the so-called pseudo-homothallic or secondarily homothallic species. These fungi develop a self-fertile mycelium thanks to the ability to generate heterokaryotic spores containing mating-compatible nuclei. This lifestyle has been adopted independently several times during evolution and pseudo-homothallic species can be found in many groups of ascomycetes and basidiomycetes. Possibly, pseudo-homothallics benefit from both heterothallism, which favors the creation of genetic variation through recombination during outbreeding and homothalism, which allows the possibility to self-cross when no exogenous partner is present. Three species have been well-studied regarding their pseudo-homothallism: 2 ascomycetes Neurospora tetrasperma and Podospora anserina and one basidiomycete Agaricus bisporus, the well-known and edible “button mushroom” or “champignon de Paris.”
The Many Ways to Create Heterokaryons After Sexual Reproduction
Most researches on pseudo-homothallics have concentrated upon how the sexually-compatible nuclei end up in the same spore after meiosis.Citation6,7 In is summarized what is happening for N. tetrasperma, P. anserina and A. bisporus. In P. anserina, the mating type locus is not centromere linked and undergoes Second Division Segregation (SDS) in 99% of the cases, indicative that a single crossover always occurs between the centromere and the mating type locus.Citation8,9 Furthermore, the spindles of the post-meiotic mitosis are perpendicular to those of the meiosis. This allows the spores to individualize around 2 nuclei of opposite mating-type. In Neurospora tetrasperma, the mating type locus is located close to a centromere and thus undergoes First Division Segregation (FDS). However, unlike P. anserina, the spindles of meiosis II are overlapping.Citation6,10,11 As a result, the 2 sister nuclei are separated. Then, after the post-meiotic mitosis, the spindles are also perpendicular and the spores can be formed around 2 nuclei carrying the 2 different mating types. In basidiomycetes, the process is not as well-understood as in the ascomycetes. The major difference being that unlike in ascomycetes, where the sporogenesis takes place within the ascus and the spores are formed in situ, the nuclei in the basidia can move and often undergo several migrations before getting into the spores.Citation12 In A. bisporus, the life cycle can be homothallic, pseudo-homothallic or heterothallic, which renders even more complex the problem.Citation13 Random migration of the nuclei was shown to be sufficient to explain the pseudo-homothallic breeding in Coprinus bilanatus.Citation14 The same model may be applied to A. bisporus.Citation14 However, genetic evidences indicate that 2 non-sister nuclei migrate preferentially into the same basidiospores in A. bisporus.Citation15 How this is achieved is not well understood, but asynchronous second meiotic division could contribute to the presence of genetically different nuclei in the basidiospores of A. bisporus.Citation13
Figure 1. Creation of mating type heterokaryosis during sexual reproduction in P. anserina, N. tetrasperma and A. bisporus. For the 3 species, at meiosis I, the spindles are aligned in the longitudinal axis of the ascus for P. anserina and N. tetrasperma or of the basidium for A. bisporus. (A) In P. anserina, the mating type locus undergoes 99% of SDS. (B) In meiosis II, the spindles are aligned and oriented in the same way as in meiosis I. (C) After meiosis, a post meiotic mitosis occurs for which the spindles are parallel and oriented across the ascus. (D) The ascospores are formed within the ascus, each one engulfing 2 non-sister nuclei. (E) About 1% of the asci show abnormal spore formation where 5 ascospores are present, 2 of them carrying only one nucleus. (F) In N. tetrasperma, the mating type locus undergoes FDS. (G) In meiosis II, the spindles are parallel and partially overlapping. This overlap leads to the same situation as in P. anserina after meiosis. (H) A post-meiotic mitosis takes place in the same way as in P. anserina and (I) the ascospores are also formed around 2 non-sister nuclei and rare 5-spored asci may be obtained. (J) In A. bisporus var. bisporus, the mating type locus likely undergoes FDS. (K) In meiosis II, the spindles may be in the diagonal of the basidiumCitation38 as depicted here or adopt other conformations.Citation13 After meiosis, 2 non-sister nuclei migrate preferentially through the sterigamata to the basidiospores.Citation15 This may be facilitated by the fact that the second meiotic division is not synchronous for the 2 nuclei issued from the first meiotic division.Citation13 (L) The 4 nuclei undergo a post-meiotic mitosis into the basidiospores.
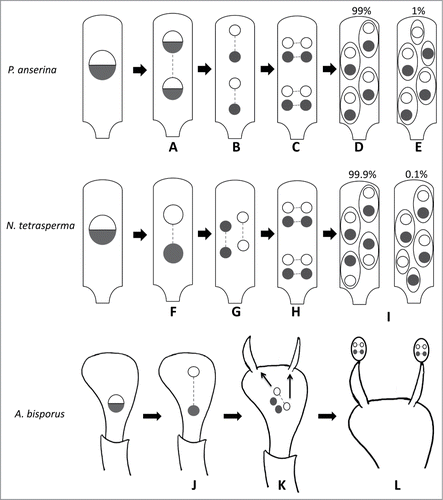
Lack of Recombination Around the Mating Type
More recently, attempts at understanding the structure of the mating type locus and its surrounding regions have been undertaken in N. tetrasperma and P. anserina. Indeed, while the idomorphs (alternative dissimilar sequences) controlling the mating compatibility have been known for quite some time in these species,Citation16,17 only the new sequencing technologies have enabled to access the sequences surrounding the idomorphs, the “mat regions.” In both species, recombination is abolished around the mating type locus, creating a large haplotype that may accumulate genetic polymorphisms, but the solutions found by the 2 fungi to inhibit it are quite different. Indeed, in N. tetrasperma,Citation18 several inversions prevent recombination in a large region of ≈7 Mb. On the contrary, we recently showedCitation19 in P. anserina that no rearrangement can explain the ≈0.8 Mb region lacking recombination that surround the mating type. The mechanisms by which recombination is inhibited in P. anserina remains a mystery, as the mat region contains many transcribed genes and does not obviously differ in its coding capacity from the rest of the genome. Moreover, elimination of a large cluster of ripped transposons present in the mat region and which could potentially act as a cis signal for trans recombination-inhibiting factors had no effect on the recombination rate.Citation19 Interestingly, while the 7 Mb mat region of N. tetrasperma differ by about 2.5% between the mata and matA strains, mat+ and mat− strains of P. anserina differ by only 0.1%. Although this could be due to a difference in the timing of the appearance of the recombination inhibition, we favored the hypothesis that recombination, although severely inhibited in the P. anserina mat region, still occur at low rate,Citation19 enabling genetic homogenization by conversion.
The reason why recombination around the mating type in P. anserina is inhibited is not clear. Indeed, in N. tetrasperma, presence of a crossing-over in the mat region would result in the recovery of homokaryotic spores carrying a single mat locus ().Citation6 In the case of P. anserina, the number of crossing-overs between the mat locus and the centromere is the important feature: only one must occur if 4 heterokaryotic ascospores are to be obtained. There is thus no obvious reason why recombination should be inhibited around the mating type. It must however be tightly regulated if only a single recombination event is to happen. Possibly, inhibition of recombination in the mat region helps to control this. Note that segregation analysis in A. bisporus detected recombination irregularities around the mating type,Citation20 which in this species, is bipolar.Citation20,21 Chromosomal rearrangements appear numerous in A. bisporus,Citation21 however, their possible involvement in the irregularities around the mating type region has not yet been proven.Citation21
The Regions Around the Mating Type are Heterozygous
Another possibility to explain the recombination inhibition in P. anserina and N. tetrasperma is that it permits the accumulation of genetic differences between the mat+/mat− or mata/matA strains, which could impact on their phenotype. Indeed, it is known that recombination blockage is the first step of the creation of sex-determining chromosomes.Citation22 Although P. anserina and N. tetrasperma are hermaphrodites, differences between opposite mating types may facilitate sexual reproduction. We did not noticed any difference between the P. anserina mat+ and mat− strains in their ability to engage sexual reproduction when grown in optimal conditions: they produce equal number of male and female gametes, fruiting body development are differentiated in equal numbers and timing. On the contrary, Branch Howe reportedCitation23 that the mata strain of N. tetrasperma had intensely pigmented conidia and mycelium and differentiated numerous small protoperithecia (the female gametangia), while matA mycelium and conidia were lighter in color and differentiated fewer protoperithecia, but some of which were large. The genetic basis for these differences is unknown, but the phenotypes co-segregated with the mating type in crosses. Furthermore, differences in the expression profile of the mata and matA strain was recently evidenced,Citation24 with an excess of matA-biased gene when grown on the medium used to induce protoperithecium formation. This leads Samils et al.24 to conclude that the matA strain could be optimized for promoting sexual female development, while the mata could be more dedicated to vegetative and male development. To validate this hypothesis, additional experiments should be performed, especially in the view that the matA differentiate less female structures.Citation23 Moreover, differentiation of gametes in fungi, especially the female one is strongly influence by temperature, medium composition etc. and could thus differ in the mata versus matA in an environment-dependent manner. This is evidenced clearly in the P. anserina S (big S) strain. Indeed, although there is no obvious difference for reproduction between the S mat+ and S mat− strains in optimal conditions, the mat+ cultures lives longer than the mat− ones, but are more sensitive to high temperature. The temperature-sensitivity difference is due to a polymorphism in the rmp1 gene, which is tightly linked to the mating type, while the genetic basis of the difference in aging, also highly-linked to the mating type, is unknown.Citation25 Since senescence and temperature inhibit sexual reproduction, we thus expect the S mat+ strain to be less efficient than the S mat− strain when used as female at high temperatures, but to overall produce more female gametes at low ones! Additionally, the polymorphism in rmp1 is not present in all P. anserina strains,Citation25 suggesting that these potential differences in gamete productions may not be universal among P. anserina isolates.
Like described for N. tetrasperma, we evidencedCitation19 transcriptional differences between S mat+ and S mat−. Intriguingly the differentially-expressed genes were not enriched in the mat region as seen in N. tetrasperma when grown on sexual-reproduction-inducing medium,Citation24 although we grew P. anserina on the medium optimal for its sexual reproduction. We however found that most genes encoded enzymes of the primary metabolism, suggesting that the S mat+ and S mat− strains could have different metabolic profiles, accounting for their different longevity. This led us to hypothesize that metabolic complementation could confer an advantage during the vegetative phase of P. anserina. Such complementation could for example maintain heterokaryosis, which is a prerequisite to enjoy the advantage of a pseudo-homothallic life cycle. We tested this hypothesis, but surprisingly found that mat+/mat+ and mat−/mat− heterokaryons were as stable as mat−/mat+ ones. A phenomenon similar to the nuclear mixing described for N. crassaCitation26 appears thus sufficient to maintain P. anserina heterokaryosis, at least in controlled laboratory conditions. We however cannot exclude that in nature where conditions are harsher and more variable, nuclear mixing could not be sufficient to maintain heterokaryosis in the absence of metabolic complementation. Maintenance of heterokaryosis appears to not have been experimentally addressed in mycelia of N. tetrasperma, but Corcoran et al. showedCitation27 that the ratio of conidia of either mating type is genetically controlled in this species. Note that in A. bisporus, nuclear mixing may also operate to preserve heterokaryosis since this species lack the typical clamps that enable the maintenance of the binucleated dikaryotic state in the mycelia of most basidiomycetes.Citation28,29
Breaking Down Heterokaryosis
Pseudo-homothallic fungi produce almost only heterokaryotic spores but there can be some exceptions. Random pairing of nuclei in basidiomycetes can lead to spores homoallelic for the mating type.Citation14 A. bisporus has some truly heterothallic strains producing 4 homokaryotic basidiospores.Citation13,30 In P. anserina and N. tetrasperma, the creation of heterokaryosis during ascus formation is also not 100% efficient.Citation9,31,32 This may result from nuclear loss in some ascospores as shown in N. tetrasperma.Citation33 In some P. anserina meioses, there is no crossing-over between the centromere and the mat locus (or an even number of them on the same chromatids), leading to the formation of 2 homokaryotic mat+ and 2 homokaryotic mat− ascospores.Citation9 Likewise, malformation of spores happens in about 1% of the asci in P. anserina and 0.1% in N. tetrasperma, resulting in the formation of 5-spored asci containing 2 small uninucleated spores.Citation10,11,34 As luck would have it, P. anserina may have an additional mechanism that ensures the maintenance of heterokaryosis from the few asci that contain homokaryotic ascospores. Ascospores stick together when they are expelled thanks to the presence of a strand or “rope” that connects the ascospore. There are few reports on the structure and function of this rope in ascomycetes.Citation35,36 It contains actin microfilaments in Sordaria macrospora.Citation36 The proposed roles for the rope are to participate in the proper alignment of the ascospore with the ascus pore to enable proper dischargeCitation35,36 and/or to enable the 4 ascospores to be expelled together, facilitating dispersal.Citation35 In P. anserina, this strand is quite resistant, as seen on the movie S1, which shows that the whole ascus follows when one ascospore is pulled. In Sordaria macrospora, a homothallic species, this rope is not as resistant as ascospores are easily separated (movie S1). However, the spore discharge in this species is rather similar to the one of P. anserina. We propose that this rope may play an additional role in P. anserina and that its strengthening may allow the spores of the same ascus to stick together. In this way, the ascospores are most likely to germinate close to each other. Thus, homokaryotic or uninucleated spores have a chance to quickly found a mating partner with which to fuse and then formed a heterokaryotic mycelium. In addition to maintaining heterokaryosis, the sticking of ascospores together may help the fungus to form a viable thallus.Citation37
In conclusion, pseudo-homothallic fungi have evolved multiple mechanisms to create and maintain the dikaryotic state allowing both selfing and crosses with any other encountered partners, while maintaining an obligate cross between 2 genetically different mates. These mechanisms operate during sexual reproduction for the creation of heterokaryosis, but also more unexpectedly during vegetative growth for its maintenance. Moreover, in P. anserina, ascospore dispersal in groups appears also optimized for keeping a dikaryotic structure, even when homokaryotic ascospores are created. It now remains to establish whether these fungi use more selfing or more outbreeding in natural ecosystems. Thanks to the new sequencing technologies, proving selfing could now be easily done in P. anserina and N. tetrasperma by resequencing the 8 genomes issued from a single ascus obtained directly from nature: all the 8 sequences should be identical apart in the region around the mating type, which should come as 2 different haplotypes. In fact sequencing only 2 genomes, one mat+ and the other mat− will provide the same information. If both sequences are identical, this would indicate selfing. Sequences from multiple asci should then provide clues whether selfing is the rule or the exception in both fungi.
Disclosure of Potential Conflicts of Interest
No potential conflicts of interest were disclosed.
Supplemental_video.wmv
Download (9 MB)References
- Billiard S, Lopez-Villavicencio M, Devier B, Hood ME, Fairhead C, Giraud T. Having sex, yes, but with whom? Inferences from fungi on the evolution of anisogamy and mating types. Biol Rev Camb Philos Soc 2011; 86:421–42; PMID:21489122; http://dx.doi.org/10.1111/j.1469-185X.2010.00153.x
- Attanayake RN, Tennekoon V, Johnson DA, Porter LD, Del Rio-Mendoza L, Jiang D, Chen W. Inferring outcrossing in the homothallic fungus Sclerotinia sclerotiorum using linkage disequilibrium decay. Heredity (Edinb) 2014; 113:353–63; PMID:24781807; http://dx.doi.org/10.1038/hdy.2014.37
- Haber JE. Mating-type genes and MAT switching in Saccharomyces cerevisiae. Genetics 2012; 191:33–64; PMID:22555442; http://dx.doi.org/10.1534/genetics.111.134577
- Klar AJ. Regulation of fission yeast mating-type interconversion by chromosome imprinting. Dev Suppl 1990:3–8; PMID:2090428; http://dev.biologists.org/content/108/Supplement/3.full.pdf+html
- Debuchy R, Berteaux-Lecellier V, Silar P. Mating Systems and Sexual Morphogenesis in Ascomycetes. In: Borkovich K, Ebbole D, eds. Cellular and Molecular Biology of Filamentous Fungi. Washington, DC: ASM Press, 2010:501–35.
- Raju NB, Perkins DD. Diverse programs of ascus development in pseudohomothallic species of Neurospora, Gelasinospora, and Podospora. Dev Genet 1994; 15:104–18; PMID:8187347; http://dx.doi.org/10.1002/dvg.1020150111
- Langton FA, Elliott TJ. Genetics of secondarily homothallic basidiomycetes. Heredity 1980; 45:99–106; http://dx.doi.org/10.1038/hdy.1980.53
- Dodge BO. Spermatia and nuclear migration in Pleurage anserina. Mycologia 1936; 28:284–91; http://dx.doi.org/10.2307/3754278
- Rizet G, Engelmann C. Contribution à l'étude génétique d'un Ascomycète tétrasporé : Podospora anserina (Ces.) Rehm. Rev Cytol Biol Veg 1949; 11:201–304.
- Dodge B. Nuclear phenomena associated with heterothallism and homothallism in the ascomycete Neurospora. J Agr Res 1927; 35:289–305.
- Colson B. The Cytology and Morphology of Neurospora tetrasperma Dodge. Annals of Botany 1934; os-48:211–24.
- Duncan EG, Galbraith M. Post-meiotic events in the Homobasidiomycetidae. Trans Brit Mycol Soc 1972; 58:387–92; http://dx.doi.org/10.1016/S0007-1536(72)80088-3
- Kamzolkina OV, Volkova VN, Kozlova MV, Pancheva EV, Dyakov YT, Callac P. Karyological evidence for meiosis in the three different types of life cycles existing in Agaricus bisporus. Mycologia 2006; 98:763–70; PMID:17256579; http://dx.doi.org/10.3852/mycologia.98.5.763
- Challen MP, Elliott TJ. Segregation of genetic markers in the two-spored secondarily homothallic basidiomycete Coprinus bilanatus. Theor Appl Genet 1989; 78:601–7; PMID:24225691; http://dx.doi.org/10.1007/BF00290848
- Summerbell RC, Castle AJ, Horgen PA, Anderson JB. Inheritance of restriction fragment length polymorphisms in Agaricus brunnescens. Genetics 1989; 123:293–300; PMID:2573557.
- Poggeler S. Phylogenetic relationships between mating-type sequences from homothallic and heterothallic ascomycetes. Curr Genet 1999; 36:222–31; PMID:10541860; http://dx.doi.org/10.1007/s002940050494
- Picard M, Debuchy R, Coppin E. Cloning the mating types of the heterothallic fungus Podospora anserina: developmental features of haploid transformants carrying both mating types. Genetics 1991; 128:539–47; PMID:1831427.
- Ellison CE, Stajich JE, Jacobson DJ, Natvig DO, Lapidus A, Foster B, Aerts A, Riley R, Lindquist EA, Grigoriev IV, et al. Massive changes in genome architecture accompany the transition to self-fertility in the filamentous fungus Neurospora tetrasperma. Genetics 2011; 189:55–69; PMID:21750257; http://dx.doi.org/10.1534/genetics.111.130690
- Grognet P, Bidard F, Kuchly C, Tong LC, Coppin E, Benkhali JA, Couloux A, Wincker P, Debuchy R, Silar P. Maintaining Two Mating Types: Structure of the Mating Type Locus and Its Role in Heterokaryosis in Podospora anserina. Genetics 2014; 197:421–32; PMID:24558260; http://dx.doi.org/10.1534/genetics.113.159988
- Xu J, Kerrigan RW, Horgen PA, Anderson JB. Localization of the Mating Type Gene in Agaricus bisporus. Appl Environ Microbiol 1993; 59:3044–9; PMID:16349046.
- Morin E, Kohler A, Baker AR, Foulongne-Oriol M, Lombard V, Nagy LG, Ohm RA, Patyshakuliyeva A, Brun A, Aerts AL, et al. Genome sequence of the button mushroom Agaricus bisporus reveals mechanisms governing adaptation to a humic-rich ecological niche. Proc Natl Acad Sci U S A 2012; PMID:23045686.
- Charlesworth D, Charlesworth B, Marais G. Steps in the evolution of heteromorphic sex chromosomes. Heredity (Edinb) 2005; 95:118–28; PMID:15931241; http://dx.doi.org/10.1038/sj.hdy.6800697
- Branch Howe Jr H. Vegetative Traits Associated with Mating Type in Neurospora tetrasperma. Mycologia 1964; 56:519–25; http://dx.doi.org/10.2307/3756357
- Samils N, Gioti A, Karlsson M, Sun Y, Kasuga T, Bastiaans E, Wang Z, Li N, Townsend JP, Johannesson H. Sex-linked transcriptional divergence in the hermaphrodite fungus Neurospora tetrasperma. Proc Biol Sci 2013; 280:20130862; PMID:23782882; http://dx.doi.org/10.1098/rspb.2013.0862
- Contamine V, Zickler D, Picard M. The Podospora rmp1 gene implicated in nucleus-mitochondria cross-talk encodes an essential protein whose subcellular location is developmentally regulated. Genetics 2004; 166:135–50; PMID:15020413; http://dx.doi.org/10.1534/genetics.166.1.135
- Roper M, Simonin A, Hickey PC, Leeder A, Glass NL. Nuclear dynamics in a fungal chimera. Proc Natl Acad Sci U S A 2013; 110:12875–80; PMID:23861490; http://dx.doi.org/10.1073/pnas.1220842110
- Corcoran P, Jacobson DJ, Bidartondo MI, Hickey PC, Kerekes JF, Taylor JW, Johannesson H. Quantifying functional heterothallism in the pseudohomothallic ascomycete Neurospora tetrasperma. Fungal Biol 2012; 116:962–75; PMID:22954339; http://dx.doi.org/10.1016/j.funbio.2012.06.006
- Colson B. The Cytology of the Mushroom Psalliota campestris Quel. Annals of Botany 1935; os-49:1–18.
- Kligman AM. Some Cultural and Genetic Problems in the Cultivation of the Mushroom, Agaricus campestris Fr. American Journal of Botany 1943; 30:745–63; http://dx.doi.org/10.2307/2437548
- Callac P, Billette C, Imbernon M, Kerrigan RW. Morphological, Genetic, and Interfertility Analyses Reveal a Novel, Tetrasporic Variety of Agaricus bisporus from the Sonoran Desert of California. Mycologia 1993; 85:835–51; http://dx.doi.org/10.2307/3760617
- Dodge BO. Inheritance of the Albinistic Non-Conidial Characters in Interspecific Hybrids in Neurospora. Mycologia 1931; 23:1–50.
- Howe HB, Jr. Markers and centromere distances in Neurospora tetrasperma. Genetics 1963; 48:121–31; PMID:13955153.
- Howe HB, Jr. Sources of Error in Genetic Analysis in Neurospora Tetrasperma. Genetics 1964; 50:181–9; PMID:14191349.
- Dowding ES. The sexuality of the normal, giant and dwarf spores of Pleurage anserina. (Ces) Kuntze. Ann Bot 1931; 45:1–14.
- Beckett A. The ascus with an apical pore: Development, Composition, and Function. In: DR R, ed. Ascomycetes Systematics - The Luttrellian concept. New York, Heildelberg, Berlin: Springer verlag, 1981:7–26.
- Thompson-Coffe C, Zickler D. Cytoskeleton interactions in the ascus development and sporulation of Sordaria macrospora. J Cell Sci 1993; 104:883–98.
- Richard F, Glass NL, Pringle A. Cooperation among germinating spores facilitates the growth of the fungus, Neurospora crassa. Biol Lett 2012; 8:419-22; PMID: 22258449; http://dx.doi.org/10.1098/rsbl.2011.1141
- Hou H, Wu L. Nuclear behaviour of cultivated mushroom. Bot Bull Academia Sinica 1972; 13:82–91.