Abstract
Immediately following birth, the gastrointestinal tract is colonized with a complex community of bacteria, which helps shape the immune system. Under conditions of health, the immune system is able to differentiate between innocuous antigens, including food protein and commensals, and harmful antigens such as pathogens. However, patients with celiac disease (CD) develop an intolerance to gluten proteins which results in a pro-inflammatory T-cell mediated immune response with production of anti-gluten and anti-tissue transglutaminase antibodies. This adaptive immune response, in conjunction with activation of innate inflammatory cells, lead to destruction of the small intestinal mucosa. Overall 30% of the global population has genetic risk to develop CD. However, only a small proportion develop CD, suggesting that additional environmental factors must play a role in disease pathogenesis. Alterations in small intestinal microbial composition have recently been associated with active CD, indicating a possible role for the microbiota in CD. However, studies demonstrating causality are lacking. This review will highlight the recent data on the potential role of the microbiota in CD pathogenesis, the potential mechanisms, and discuss future research directions.
Abbreviations
CD | = | celiac disease |
GALT | = | gut associated lymphoid tissue |
IBD | = | inflammatory bowel disease |
GRD | = | gluten related disorders |
MLN | = | mesenteric lymph node |
IEL | = | intraepithelial lymphocyte |
Tregs | = | regulatory T cells |
DC | = | dendritic cell |
SFB | = | segmented filamentous bacteria |
SCFA | = | short chain fatty acids |
WT | = | wild-type |
GFD | = | gluten-free diet |
TG2 | = | tissue transglutaminase |
CTL | = | cytotoxic T lymphocytes |
FISH | = | fluorescence in situ hybridization |
PBMC | = | peripheral blood mononuclear cell |
EC | = | epithelial cell. |
Introduction
Our intestine is exposed to a vast amount of foreign material, including pathogenic and commensal bacteria, as well as food antigens. We ingest more than 100 grams of foreign protein per day in the diet, and the density of the microbiota reaches up to 1012 bacteria per gram of gut content in the colon.Citation1 The immune system must therefore be able to discriminate between harmful, pathogenic bacteria, while remaining tolerant to harmless antigens such as food proteins and commensal bacteria. This concept of oral tolerance is a key feature of the gut associated lymphoid tissue (GALT) whereby a state of local and systemic unresponsiveness to food protein is induced through the oral administration of an innocuous antigen.Citation1 A number of immune adaptations also exist to maintain host-microbial homeostasis and systemic ignorance of commensal bacteria.Citation2
The concept of oral tolerance has been known for over a century,Citation3 but why some individuals develop intolerances to normally innocuous antigens is not fully understood. Inappropriate immune responses to members of the microbiota are thought to contribute to inflammatory bowel disease (IBD).Citation4 On the other hand, food allergy and celiac disease (CD) are thought to result from the failure to induce oral tolerance or loss of tolerance to food protein, such as peanut or gluten, respectively.Citation1 The prevalence of those suffering from intolerances or sensitivity to food proteins, including CD, is increasing every year and the mechanisms underlying this phenomenon are not clearly understood.Citation5-10 The microbiota plays an important role in immune maturation and homeostasis; alterations in microbial composition or colonization may influence intestinal homeostasis and consequently, immune responses to food antigens. While alterations in microbial composition (dysbiosis) have been shown in patients with food allergy and CD,Citation11,12 whether dysbiosis is a disease-promoting factor is unknown. Here we will discuss the role of the microbiota in immune maturation and intestinal homeostasis, and factors that influence intestinal colonization. We will review emerging data involving the microbiota in the development of abnormal immune responses to food antigens. Although gluten related disorders (GRD) include all conditions that are triggered by gluten, including CD, gluten ataxia, non-celiac gluten sensitivity, and dermatitis herpetiformis,Citation13 we will focus on CD as a specific example in this review to highlight possible mechanisms through which the microbiota may play a pathogenic role in the development of adverse reactions to food components. The reason for this being that CD, unlike other GRD, is a well-defined entity with known pathophysiological mechanisms.
Overview of the Intestinal Microbiota
During birth, mammals are colonized with a complex community of bacteria. The diversity of the infant microbiota is lower than a healthy adult microbiota, but gradually increases with time.Citation14 Initially, the neonatal gastrointestinal tract is colonized by facultative anaerobes, which deplete the oxygen supply over a few days and allows colonization of strict anaerobes including Bifidobacterium, Clostridium, and Bacteroides.Citation15 The intestinal microbiota fluctuates over the first 2 years of life, with high interindividual variability, but becomes more stable and converges to resemble that of an adult microbiota by 2 to 3 years of age.Citation16,17 A number of factors, which include mode of delivery, feeding pattern, and antibiotic use, can influence this initial colonization. These first few months of life are a critical window during which the microbiota can modulate key events in the host, including immune development, which may have long-lasting health implications.
The adult microbiota contains more than 10Citation14 microorganisms, about 10 times as many cells as the human body. Molecular profiling has shown that the adult microbiota still has high interindividual variability at the species level. However, when compared at higher-taxa levels, the microbiobota are highly conserved.Citation18 The adult microbiota is typically dominated by members of the Firmicutes and Bacteroidetes phyla, with members from Proteobacteria, Actinobacteria, Fusobacteria and Verrucomicrobia accounting for less than 10% of the total population.Citation2 Alterations in the composition of the microbiota, termed dysbiosis, are associated with a number of inflammatory diseases including CD, raising the question whether the microbiota plays a role in disease pathogenesis.
Role of the Microbiota on Immune Development and Homeostasis
Animal studies using gnotobiotic mice have demonstrated that host-microbial interactions are crucial for the development of a fully functioning immune system. Germ-free mice, which are reared in a completely sterile environment, have a number of mucosal and systemic innate and adaptive immunological defects.Citation19-21 Germ-free mice have a thinner mucus layerCitation22 as well as reduced levels of the antimicrobial peptide RegIIIγ.Citation23,24 The development of secondary lymphoid structures is also impaired in germ-free mice. The development of Peyer's patches and mesenteric lymph node (MLN) are initiated prenatally. However, their maturation is dependent on microbial colonization as germ-free mice have underdeveloped Peyer's patches and MLNs, with no germinal centers.Citation19,22 The microbiota is also crucial for modulating T cell differentiation and secretory IgA production. Germ-free mice have a reduced number of lamina propria CD4+ T cells,Citation25 intraepithelial lymphocytes (IELs) bearing the αβ TCR,Citation26,27 and colonic regulatory T cells (Tregs),Citation20,28 which are restored following conventionalization. The differentiation of B cells into IgA-producing plasma cells is induced through the sensing of microbiota-derived flagellin by lamina propria dendritic cells (DCs).Citation29 It has become clear that the microbiota plays an important role in the development of the immune system, and in shaping the nature of innate and adaptive immune responses. However, the effects of the microbiota on modulation of innate immune cells has not been as well characterized as the effects on adaptive immune cells. For example, the effect of the microbiota on the development of IEL subsets and their activation isn't fully understood.Citation30 Furthermore, most studies have focused on microbial-induced effects in the colon, and thus the effects of the microbiota on immune cell development in the small intestine is not as clear. There may be differences in the proportion of Tregs in germ-free versus colonized mice, depending on both the location (small intestine vs. large intestine) and strain of the mouse.Citation31 This may have important implications for the development of oral tolerance to food antigens which occurs in the small intestine.
Particular members of the microbiota may simulate immune responses differently, and this may have implications for susceptibility or resistance to disease. For example, segmented filamentous bacteria (SFB) have been suggested to be potent inducers of the pro-inflammatory Th17 cellsCitation32,33 whereas Clostrium and Bacteroides fragilis (B. fragilis) have been shown to stimulate Tregs.Citation31,34,35 Microbial-derived products, such as LPS and short-chain fatty acids (SCFA) have also been shown to stimulate Tregs in germ-free mice.Citation36-39 Thus, induction of particular immune responses may not solely depend on the presence or absence of live bacteria, but on the relative abundance of particular members of the microbiota or the presence of microbial-derived products.Citation40 This also suggests that imbalances in the microbiota may lead to dysregulated pro-inflammatory (pathological) and regulatory (protective) immune responses which may influence tolerance to food proteins ().Citation41
Figure 1. Microbiota and immune homeostasis in oral tolerance. (A) In the healthy gut there is a balance between beneficial bacteria and potentially harmful bacteria (pathobionts). This results in intestinal homeostasis with a balance between the pro-inflammatory Th1 and Th17 cells and regulatory T cells, allowing the generation of oral tolerance to food proteins such as gluten. (B) A disruption of early microbial colonization (C-section, neonatal antibiotic use) or a disruption of barrier function or microbial ecology (changes in diet, infections, or drugs) can results in intestinal dysbiosis. This can lead to imbalances between pro-inflammatory and regulatory immune cells. In genetically susceptible individuals, immune imbalances may promote loss of tolerance to food proteins, such as gluten.
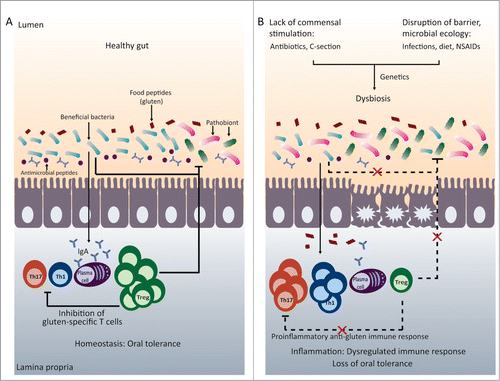
Many recent studies have suggested that the microbiota may be a disease promoting factor in food intolerances or sensitivities. Evidence from animal models show that germ-free mice are more susceptible to oral sensitization to cow's milk protein,Citation42,43 and OVA-induced allergy.Citation44 Oral sensitization to OVA led to altered intestinal microbial composition in genetically susceptible mice, which was not evident in wild-type (WT) mice. Interestingly, the transfer of microbiota from OVA-sensitized mice to germ-free mice also transfers disease susceptibly.Citation45 These studies suggest that the lack of bacteria can exacerbate intolerance to proteins. Germ-free studies are crucial for understanding the fundamental role of the microbiota in disease pathogenesis; however, it is difficult to extrapolate conclusions from germ-free studies to human disease. Several studies have suggested the use of antibiotics increases the severity of OVA and peanut-sensitization in mice.Citation46,47 The mechanisms involved in food allergy pathogenesis are very different from those involved in CD, and therefore drawing conclusions from these studies may not be of relevance to CD. Therefore, experimental studies exploring how alterations in microbial composition or the presence of particular bacterial species can play a causative role in CD pathogenesis and intolerance to gluten are critical for understanding the link between the microbiota and CD.
The next sections will review the known factors that play a role in the pathogenesis of CD with emphasis on the possible role of microbial factors as disease modulators.
Celiac Disease
CD is a chronic immune mediated enteropathy caused by the lack of oral tolerance to gluten in genetically susceptible individuals.Citation11,48 The incidence of CD has continued to rise,Citation5,49,50 and serological based studies estimate that the prevalence in the United States and European countries is 1%.Citation51-53 The prevalence rates within Europe vary from 2.4% in Finland, to 0.3% in Germany.Citation51 Although the lack of biopsy-proven CD diagnosis in serological based studies is a limitation, serology may be optimal for screening large populations in which small intestinal biopsies for all participants is not feasible. Furthermore, sequential serological testing of IgA anti-tissue transglutaminase (TG2) and IgA endomysial antibody (EMA) has been shown to have excellent diagnostic performanceCitation6,54 The only treatment for CD is a life-time adherence to a gluten-free diet (GFD). However, there are many problems associated with a GFD, including high non-adherence rates and high costs.Citation55,56 HLA-DQ2 and DQ8 are the major susceptibility genes, which are necessary, but not sufficient for disease development. Only a subset of genetically susceptible individuals develop CD, suggesting additional environmental factors must play a role in disease pathogenesis.Citation57 Recently, the composition of the microbiota has been implicated in the pathogenesis of CD.Citation11 However, whether alterations in microbial composition (dysbiosis) contributes to CD pathogenesis or is a consequence of the disease is unclear. Unlike many other chronic inflammatory diseases, the trigger (gluten) and the genetics of CD are well-defined, making it an ideal disease to study the relationship between intestinal microbiota, host genetics and inflammation.
Celiac Disease Pathogenesis
The hallmark of CD is an immune-mediated enteropathy that involves both innate and adaptive immune responses (). The interplay between gluten, HLA-DQ2/DQ8 and TG2 plays a key role in the adaptive anti-gluten immune response. Two properties of gluten may explain their toxicity for CD patients. First, gluten peptides are highly resistant to degradation by proteolytic enzymes due to their high proline content.Citation58 This leaves large fragments that can reach the mucosa. Second, the high proline and glutamine content renders them excellent substrates for TG2. TG2 converts glutamine residues to negatively charged glutamate residues in a process known as deamidation, which increases binding avidity to HLA-DQ2/DQ8 molecules.Citation48 Additionally, the proline residues in gluten peptides allow preferential binding in the HLA-DQ2/DQ8 peptide grooves.Citation59 The HLA-DQ haplotype plays an important role in determining CD risk.Citation60 The strongest association occurs with HLA-DQ2.5, which is encoded by the HLA-DQA1∗05 (α-chain) and HLA-DQB1∗02 (β-chain) alleles, whereas HLA-DQ2.2 confers a much lower risk.Citation61 More than 90% of CD patients carry the HLA-DQ2.5 heterodimer.Citation60 Unlike the HLA-DQ2.2 heterodimer, DQ2.5 is able to form strong complexes with a larger repertoire of gliadin peptides due to the presence of a proline reside at position 3 of many gluten epitopes.Citation61 Furthermore, the presence of a tyrosine residue at position 22 of the α chain of DQ2.5 allows for sustained antigen presentation to gluten-specific T cells.Citation62 Together, these properties allow the activation of HLA-DQ2/DQ8 restricted gluten-specific T cells within the gut which produce inflammatory cytokines IFN-γ and IL-21.Citation63,64 Importantly, gluten-reactive T cells are present in the gut of CD patients and they preferentially recognize deamidated gliadin peptides.Citation65 In addition, CD4+ gluten-specific T cells provide help to activate B cells to become anti-gluten and anti-TG2 antibody producing plasma cells.Citation57 While antibodies specific for TG2 are useful for the diagnosis of CD, the role that they play in CD pathogenesis and tissue damage remains unclear.Citation60 TG2 is normally present in the gut; however, under homeostatic conditions it remains inactive.Citation66,67 Its regulation is not fully understood, but may be modulated by tissue damage, infections, or the microbiota.Citation67
Figure 2. For figure legend, see next page.Figure 2 (See previous page). Celiac disease (CD) pathogenesis and potential microbial role as a disease modulator. (A) CD Pathogenesis. Gluten peptides in the small intestinal lumen translocate the epithelial barrier, either through paraceullular or transcellular mechansisms. Once in the lamina propria (LP), deamidation occurs, during which tissue transglutaminase (TG2) introduces negatively charged residues into the gluten peptides. Once gluten peptides are deamidated they can bind strongly and preferentially to DQ2/DQ8 molecules that are present on DCs. After migrating to sites of induction (mesenteric lymph nodes (MLN)), mature DCs present the gluten peptides to gluten-specific CD4+ T cells resulting in their activation and a gluten-specific Th1 response (production of IFNγ and IL-21). Gluten peptides and TG2 can also form complexes, which can be taken up by TG2 specific B cells or gluten-specific B cells. The presentation of gluten peptides by B cells to gluten-specific T cells results in B cell activation and the formation of anti-gliadin and anti-TG2 producing plasma cells. In the lumen, secretory anti-gliadin antibodies can bind gluten and transport gluten to the lamina propria via CD71-mediated transcytosis. Increased epithelial cell (EC) stress, triggered by gluten peptides, bacteria, or viruses, can upregulate stress molecules on epithelial cells (HLA-E, MICA/B) and induce IL-15 production from ECs. IL-15 can induce DC maturation and upregulate NK receptors (NKG2D) on IELs. The binding of NK receptors on IELs to their ligands (HLA-E and MICA/B) on ECs results in cytotoxic killing of ECs leading to tissue damage. IL-15 can also inhibit the regulatory effects of Tregs. (B) Pathogenic and protective role of microbiota in CD. Potential pathobionts, including E. coli and Shigella, may promote pro-inflammatory anti-gluten immune responses. First, E. coli strains isolated from CD patients were shown to have increased virulence. Second, E. coli and Shigella can induce the maturation of DCs and the production of pro-inflammatory cytokines (IL-12, TNFα) after gliadin stimulation. Finally, Shigella and E. coli can increase intestinal permeability and alter tight junction (TJ) protein expression. On the other hand, potentially beneficial bacteria, such as bifidobacteria, may reverse pathogenic, gluten-induced responses. Bifidobacteria can reverse gluten-induced increased permeability and altered TJ expression. Bifidobacterium species may also reduce the number of toxic, immunogenic gliadin peptides generated in the lumen. Finally, bifidobacteria can promote the production of IL-10 from DCs.
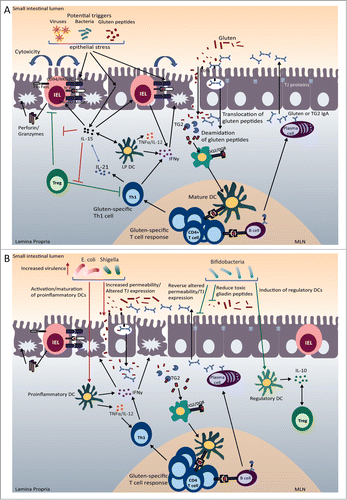
In addition to this adaptive anti-gluten response, an innate immune response is critical for epithelial cell damage in CD. Intraepithelial cytotoxic T lymphocytes (intraepithelial CTLs) expand in patients with CD, but return to normal levels on a GFD, correlating with the presence of villous atrophy.Citation68 While the proliferation and activation of the CTLs seems to depend on the presence of gluten, exactly how their activation is triggered or regulated in CD is not fully understood.Citation57 Several triggers, including bacteria, viruses, or gluten itself may be involved in the activation or regulation of cytotoxic IELs through the production of IL-15 from epithelial cells and DCs.Citation57 IL-15 can activate CTLs and upregulate the expression of activating NK receptors (NKG2D) on intraepithelial CTLs.Citation69 However, high levels of IL-15 have been described in only a proportion of CD patients raising the possibility that other mediators can activate intraepithelial CTLs.Citation70 In active CD, IELs express high levels of these activating receptors. CD patients also have increased epithelial expression of stress-induced ligands, such as HLA-E and MICA, which are ligands for NKG2D. The interaction between NKG2D on CTLs and MICA on epithelial cells leads to epithelial cell death, contributing to villous atrophy.Citation69,71,72 Another subset of IELs which express the γδ TCR are upregulated in CD patients, but remain increased on a GFD,Citation68 leading to the hypothesis that these cells play a regulatory or anti-inflammatory role.Citation73 However, whether these cells can mediate tissue damage during active disease has not been excluded.Citation60 The exact role of these different IEL phenotypes in CD and their regulation is not completely understood.
Many of the immunological mechanisms involved in CD pathogenesis have been well characterized. However, it remains unclear why only a proportion of genetically susceptible individuals develop CD. About 30–35% of the population carry the HLA-DQ2 or DQ8 susceptibility genes; however, only 2–5% of genetically susceptible individuals will go on to develop CD, suggesting that additional environmental factors besides gluten introduction are involved in CD pathogenesis.Citation74 Furthermore, the rate at which the prevalence of CD is increasing is greater than what can be explained by genetic factors alone, again suggesting a role for environmental factors in CD pathogenesis.Citation75 Evidence showing an association between intestinal dysbiosis and CD suggests that the microbiota could be an additional environmental factor that may promote breakdown of tolerance to gluten in genetically susceptible individuals.
Is there a Role of Microbiota in Celiac Disease?
Factors influencing intestinal colonization that could modify risk for celiac disease
Many factors influence microbial colonization, including birth mode, neonatal antibiotic use and diet.Citation15 Given the importance of microbial exposure on educating the immune system, the initial microbiota that colonizes the gastrointestinal tract may have important consequences on shaping the immune system in the neonatal gut. This, in turn may influence the development of tolerance to food antigens (). The association between perturbations in intestinal microbial composition early in life and later development of CD provides further evidence that intestinal dysbiosis may play a pathogenic role in CD.
The mode of delivery has been shown to have a great impact on the initial colonizers of the gastrointestinal tract, and these changes persist beyond the first days and months following birth.Citation76-78 Infants born by caesarean section (C-section) have delayed colonization of members of the microbiota, lower overall microbial diversity, and alterations in immune function compared to infants born vaginally.Citation76,77,79,80 These studies have important implications as the rate of caesarean deliveries around the world is increasing, reaching over 30% in the United States in 2007.Citation81 There have been 2 case-control studies exploring the link between caesarean delivery and later development of CD. The first study included children with CD, inflammatory bowel disease, other gastrointestinal diseases and healthy controls. A significantly higher rate of caesarean delivery was found only in children with CD.Citation82 The second case-control study concluded that there was a positive association between elective caesarean delivery and later CD.Citation83 There was no association between later CD and emergency C-section. However, children born by emergency C-section may have been in contact with the birth canal, where initial intestinal colonization of the infant would occur.Citation83 These associations need to be confirmed with additional studies; however, they suggest that altered microbial colonization following caesarean delivery may impair the establishment of host-microbe homeostasis and impair generation of tolerance to gluten.
Antibiotics are designed to target pathogenic bacteria; however, they also affect related commensals or co-dependent bacteria, which can lead to long-lasting shifts in the microbial community.Citation84,85 Most members of the microbiota will return to pre-treatment levels within days or weeks after antibiotic treatment has ended.Citation86,87 However, some members do not return and are lost from the community, resulting in long-term changes in bacterial diversity as well as changes in immune function.Citation14,46,88-90 These antibiotic-induced changes in the microbiota and immune function may have important implications in food intolerances. A recent case-control study linked antibiotic use at any time in life to CD. This risk increased with a greater number of antibiotic courses. However, the limited time window included in the study was a major limitation making it difficult to determine whether antibiotic use truly preceded CD onset.Citation91 A recent population-based birth cohort study that included over 200,000 babies investigated perinatal variables, infections, and antibiotic use during the first year of life as possible risk factors for CD.Citation92 The study found that infections during the first year of life were associated with later CD diagnosis. An even stronger association was found when only intestinal infections were considered. Antibiotic use during the first year of life was also significantly associated with subsequent CD diagnosis. The risk of developing CD increased significantly with increasing number of antibiotic courses.Citation92 An important finding of the paper was that the positive association between CD and antibiotic use depends on the type of antibiotic. The use of penicillin and cephalosporin during the first year of life were associated with later CD development whereas macrolide had no association with later CD onset. The use of cephalosporin during the first 6 months of life was also significantly associated with later CD.Citation92 An earlier study failed to find an association between antibiotic use and later CD.Citation93 However, the type or class of antibiotics used in this study were not identified. Antibiotics can have a different effect on the microbiota depending on their spectrum and pharmacokinetic profile.Citation88,94 Future studies on the early use of antibiotics, their effect on the microbiota, as well as investigation on the basic mechanisms through which they could affect CD development are necessary.
It is well known that diet can affect the composition of the intestinal microbiota.Citation95,96 In line with this, feeding patterns in early life can influence the development of the microbiota. Breast milk may be an important source of early colonizers as well as pre and probiotics.Citation15,97 Several recent studies have explored the link between early feeding practices, the microbiota, and the subsequent development of CD in at-risk children. In a prospective study of 164 newborn, both the HLA-DQ genotype and milk-feeding type influenced the early intestinal microbiota composition. Infants with a high genetic risk of CD had increased numbers of B. fragilis group and Staphylococcus spp., and reduced numbers of Bifidobacterium spp and B. longum. Breast-feeding genetically susceptible children decreased B. fragilis numbers and increased Bifidobacterium spp, whereas feeding practices had no influence on Staphylococcus spp.Citation98 Sellitto et al.Citation99 also concluded that infants at risk for developing CD had a different microbiota than children with no genetic predisposition. At risk infants had an overall decrease in bacteria belonging to the Bacteroidetes phylum and an increase in Firmicutes.Citation99
The interaction between feeding practices and microbiota was further hypothesized to influence CD risk after rod shaped bacteria were detected in CD children from the so-called “Swedish epidemic.” In Sweden the annual incidence rate of CD in children was 4 times higher from 1985–1995,Citation100 which coincided with changes in feeding practice guidelines. At the start of the epidemic, national feeding recommendations for infants postponed the introduction of gluten from 4 to 6 months. As a result, a larger proportion of children received gluten without the protection of breastfeeding. At the same time, the average consumption of gluten from commercial milk products increased in children under 2 years of age. Rod-shaped bacteria constituted a large fraction of the small intestinal microbiota of children born during this epidemic and this interaction between changes in feeding practices and the presence of rod-shaped bacteria may be an important risk factor that contributed to the increase in disease incidence.Citation101 These studies suggest that host genotype, as well as feeding practices may influence colonization patterns early in life; introduction of gluten to an immature or dysbiotic microbiota could trigger or accelerate the loss of gluten tolerance.
Together, these studies demonstrate that the period during which intestinal microbial colonization is established may critical for the establishment of appropriate immune responses toward dietary antigens. Disruptions of this process may influence the developing immune system, which may have consequences on the development of oral tolerance.
Dysbiosis and celiac disease
In the past 6 years, a number of studies have explored the overall differences in fecal and duodenal microbial composition in patients with active CD, treated CD, and healthy children and adults. One of the first studies exploring microbial differences between CD children and healthy controls found the ratio of harmless bacteria (Lactobacillus—Bifidobacterium) to potentially harmful bacteria (Bacteroides-E. coli) was significantly reduced in the duodenum of CD patients compared with controls.Citation102 These changes were independent of disease activity as no differences were seen in patients who had been on a GFD. Patients with active disease also had increases in total gram-negative bacteria as well as Bacteroides and E. coli groups, which were normalized following a GFD.Citation102 In another study, a higher ratio of fecal gram-positive to gram-negative bacteria was found in healthy controls compared to children with treated and untreated CD.Citation103 Analysis of specific microbial composition in feces and duodenal biopsies by real-time PCR showed that the Bacteroides and Clostridium leptum (C. leptum) groups were more abundant in treated and untreated CD children compared to controls. Untreated CD children also had higher counts of E. coli and Staphylococcus that normalized after treatment with GFD.Citation104 In the same cohort of children, lower total Bifidobacterium and B. longum counts were seen in feces and duodenal biopsies from active and inactive CD children compared with controls.Citation105 Similarly, using fluorescence in situ hybridization (FISH) De Palma et al. found that Bifidobacterium proportions were lower and the abundance of C. histolyticum, C. lituseburense and F. prausnitzii groups was higher in the feces of untreated celiac children compared to healthy children whereas patients with treated CD had intermediate values.Citation103 Fecal samples from children with active or inactive disease were also shown to have increased abundance of B. fragilis.Citation106 Culture-dependent methods also showed significant differences in fecal microbiota between treated celiac children and healthy controls. Lactobacillus, Enterococcus and bifidobacteria were significantly higher in fecal samples from healthy children compared to treated CD patients. Bacteroides, Staphylococcus, Salmonella, Shigella and Klebsiella were higher in treated CD children compared to controls.Citation107 Culture-dependent methods also detected differences in duodenal microbial composition between CD children and healthy children. Children with active CD had a higher abundance of Proteobacteria and lower abundance of Firmicutes compared to treated CD children and healthy controls. Active CD was also associated with increases in Enterobacteriaceae and Staphylococcaceae.Citation108
In adult patients, analysis of fecal microbiota showed that CD patients have a different microbial composition than healthy controls.Citation109 Additionally, CD adults with gastrointestinal symptoms had a higher amount of Proteobacteria and lower abundance of Firmicutes and Bacteroidetes than the patients with another manifestation of the disease or the control subjects.Citation110
Differences in diversity have also been detected between CD patients and healthy controls. Increased diversity of dominant microbial species was reported in patients with active disease, compared to the same patients after 9-months of a gluten free diet and compared to healthy controls.Citation111 Analysis of duodenal microbiota by DGGE demonstrated that the diversity was higher in treated CD children compared to healthy children.Citation107 Culture-dependent techniques also showed patients with active CD had the highest diversity in duodenal microbiota, followed by treated CD and controls.Citation108 Differences in species diversity have also been reported. Increased Bacteroides diversity was reported in controls compared to CD children whereas Bifidobacterium diversity was higher in CD children compared to controls.Citation112 In adults, there was a higher diversity of the Lactobacillus and Bifidobacterium in untreated CD patients and in healthy controls, compared to treated patients, suggesting the GFD may have an effect on microbiota.Citation109 Indeed, in healthy subjects, a GFD was shown to affect the fecal microbial composition, including Lactobacillus and Bifidobacterium species.Citation113
Finally, the metabolic function of the microflora in CD has been characterized by assessing the presence and composition of fecal SCFA. Children and adults with active or inactive CD have increased fecal SCFA.Citation109,114 Increases in levels of acetic, valeric, and butyric acid were detected in children with CD and remained increased after treatment with GFD for 3 months.Citation114 In contrast, a recent study concluded that total SCFA levels normalized after treatment with a GFD for greater than one year, whereas shorter treatment duration had no effect.Citation115 Butyric acid has anti-inflammatory properties and positive metabolic effects on intestinal enterocytes, while acetic acid has pro-inflammatory effects.Citation115 A fermentation index has recently been demonstrated to illustrate the inflammatory properties of SCFA in Crohn's disease (amount of acetic acid minus propionic acid and n-butyric acid, together divided by the total amount of SCFAs). In adults with untreated CD, the fermentation index, and thus inflammatory properties of SCFAs, was significantly higher compared to healthy adults and CD patients treated with GFD for greater than one year.Citation115 In another study, patients with treated CD were found to have higher fecal levels of acetic acid, while isocaproic, butyric and proprionic acids were higher in healthy controls compared to treated CD patients.Citation107 These changes in SCFA may be a result of altered intestinal microbiota, but could also be a result of increased fermentable substrates reaching the colon due to villous atrophy and reduced nutrient absorption in active CD patients. Further studies exploring the link between dysbiosis, SCFA and their effect on the host in CD are needed.
The balance between pathogenic and protective bacteria in celiac disease
It has been described that patients with active CD have an altered small intestinal microbial composition compared to healthy controls, and some of these changes are reversed, or partially reversed after treatment with a gluten free diet. However, it is still unclear whether these alterations in microbial composition play a pathogenic role in CD. Whether and how dysbiosis could initiate or exacerbate loss of tolerance to gluten, the anti-gluten CD4+ T cell response, and the full activation of intraepithelial CTLs is unknown. Studies exploring the link between bacterial species and gluten-induced immune responses have shed more light on the potential pathogenic role of bacteria in CD ().
A number of studies have detected increases in virulence gene expression in CD microbiota. E. coli clones isolated from the feces of celiac children had increases in virulence-gene carriage when compared to those from healthy controls.Citation116 Given the increased abundance of B. fragilis in CD patients, the potential pathogenicity was explored by Sanchez et al. and it was found that B. fragilis clones carrying virulence genes for metalloproteases were more abundant in CD patients than in controls.Citation106 Interestingly, applying this strain to Caco-2 monolayers led to increased permeability.Citation106 Finally, the presence of virulence genes was higher in Staphylococcus epidermidis clones isolated from CD patients compared to controls.
The link between CD and the pathogenic role of bacteria has recently been investigated in a number of studies. The different immunomodulatory effects of commensals or potential pathogens isolated from CD patients have been explored on monocytes, DCs, and epithelial cells. Feces from active CD patients applied to peripheral blood mononuclear cells (PBMCs) in vitro led to increased TNF-α and IFN-γ production compared to feces from control subjects. Feces from controls lead to increased IL-10 production by PBMCs. The addition of Bifodobacterium strains reduced the pro-inflammatory effects of CD feces and led to increased IL-10 production.Citation117 In another 2 studies, the effects of B. longum, B. bifidum, B. fragilis, E. coli and Shigella on PBMCsCitation118 or DCsCitation119 with or without gliadin stimulation were evaluated. E. coli and Shigella induced high levels of IFN-γ and IL-12 whereas the Bifidobacterium strains induced IL-10 production from PBMCs and DCs. The different bacterial strains also induced different cell surface markers on PBMCs. In co-cultures with Caco-2 monolayers and PBMCs or DCs, stimulation with Shigella increased TNF-α and IFN-γ production from PBMCs and DCs, which was further increased with the addition of gliadin and IFN-γ. These studies suggest that enterobacteria have the ability to induce pro-inflammatory signals through an intact epithelial barrier and could play a potentially pathogenic role in CD.Citation118,119 Finally, a recent study also suggested that CD-associated bacteria may influence the IL-17A response by intestinal T cells as well as the level of anti-gluten T cells response in CD patients.Citation120
The potential beneficial effects of commensals and probiotics have been further investigated in vitro and in vivo. Bifidobacterium strains were shown to reduce the number of toxic gliadin peptides generated in vitro and reverse their adverse effects on Caco-2 monolayers.Citation121 In an animal model, administration of B. longum reduced pro-inflammatory cytokine production and increased IL-10 production following sensitization and gliadin feeding.Citation122 In an in vitro study, B. lactis, but not L. fermentum reversed gliadin-induced increased permeability and altered expression of the tight junction protein ZO-1 in Caco-2 cells.Citation123 Using a model of intestinal loops in germ-free rats, it was similarly shown that B. bifidum could reverse gliadin-induced altered ZO-1 expression in the small intestine. On the other hand, E. coli and Shigella induced altered ZO-1 expression and increased the translocation of gliadin peptides to the lamina propria.Citation124
Given the beneficial role of Bifidobacterium strains on toxic gluten-induced effects in vitro and the barrier enhancing properties of secreted factors from B. infantis, a recent clinical trial assessed the effect of B. infantis as a therapeutic intervention in patients with untreated CD consuming gluten.Citation125 CD patients were randomized to receive capsules containing B. infantis or placebo before each meal, consuming at least 12 mg gluten per day for 3 weeks. The randomized, double-blind, placebo-controlled study failed to see any changes in intestinal permeability in those treated with the probiotic. However, patients receiving probiotic treatment had a significant improvement in gastrointestinal symptoms and decreased anti-TG2 IgA antibodies.Citation125 This is the first clinical trial assessing the therapeutic potential of a probiotic for CD; future studies are needed to confirm these results and explore whether strains of probiotics can modulate the gluten-induced responses in vivo.
Together these studies demonstrate that members of the microbiota may participate in the generation of toxic gliadin peptides and can differentially influence gliadin-induced immune responses. Alterations in intestinal microbial composition may therefore have profound effects on the type of immune response that is generated against gliadin and may play a role in the loss of gluten tolerance. This may have particular importance during the colonization process during which the immune system is undergoing education and maturation. The use of germ-free and gnotobiotic animal models are useful for evaluating the importance of microbial colonization on subsequent immune responses. An early study in germ-free rats demonstrated that a single high dose of gliadin to 10 day old germ-free rats induced mild epithelial damage. In addition, long-term gliadin, but not albumin feeding to germ-free rats induced moderate small intestinal damage, including crypt hyperplasia, villous atrophy, and increased IELs.Citation126 Although this study lacks appropriate colonized controls to draw major conclusions, it supports that gliadin, but not albumin, can induce mucosal damage in the absence of gut microbes. The transgenic NOD/DQ8 mouse, which expresses the human DQ8 gene, is a validated animal model to study gluten-induced immune responses following sensitization to gluten.Citation127 Interestingly, colonization with an ultra-clean microbiota known to induce balanced immune responses in germ-free mice,Citation20 had a protective effect during gliadin-sensitization and challenge in NOD/DQ8 mice.Citation128 In comparison to mice colonized with an ultra-clean microbiota free from any opportunistic pathogens, germ-free mice developed a heightened gluten-induced response. These results suggest that the background microbiota can influence the development of both innate and adaptive gluten-induced responses. Further studies exploring mechanisms and whether particular microbial factors play a key role in the development of oral tolerance to gluten in genetically susceptible individuals are necessary.
Future Directions and Conclusions
The evidence for an association between intestinal dysbiosis and CD is clear; however, evidence demonstrating causality is lacking. It remains unclear whether general changes in microbial composition or the presence of absence of particular members of the microbiota play a direct role in CD pathogenesis. Furthermore, the mechanisms through which the microbiota may influence gluten intolerance are unclear. Recent in vitro data has shed some light into the potential role of the microbiota on influencing gluten-induced immune responses. Changes in microbial composition may therefore influence whether the immune system becomes tolerant to gluten or recognizes the protein as foreign, mounting an anti-gluten pro-inflammatory immune response. However, in vivo data demonstrating how altered intestinal colonization may promote intolerance to gluten and subsequent CD is lacking. The continued use of gnotobiotic animal models will be a valuable tool to explore this complex relationship between the intestinal microbiota, host genetics, and gluten. These models can be used to explore whether the lack of stimulation by particular microbial species during immune development, or the presence of potential pathogens during immune education can promote the development of gluten related disorders.
Disclosure of Potential Conflicts of Interest
No potential conflicts of interest were disclosed.
Funding
The work was supported by a CIHR grant (MOP-123282) to EFV and a CCA award to HG. EFV holds a Canada Research Chair.
References
- Pabst O, Mowat A. Oral tolerance to food protein. Mucosal Immunol 2012; 5:232-9; PMID:22318493; http://dx.doi.org/10.1038/mi.2012.4
- Hooper LV, Macpherson AJ. Immune adaptations that maintain homeostasis with the intestinal microbiota. Nat Rev Immunol 2010; 10:159-69; PMID:20182457; http://dx.doi.org/10.1038/nri2710
- Weiner HL, da Cunha AP, Quintana F, Wu H. Oral tolerance. Immunol Rev 2011; 241:241-59; PMID:21488901; http://dx.doi.org/10.1111/j.1600-065X.2011.01017.x
- Cerf-Bensussan N, Gaboriau-Routhiau V. The immune system and the gut microbiota: friends or foes? Nat Rev Immunol 2010; 10:735-44; PMID:20865020; http://dx.doi.org/10.1038/nri2850
- Ludvigsson JF, Rubio-Tapia A, van Dyke CT, Melton LJ, Zinsmeister AR, Lahr BD, Murray JA. Increasing incidence of celiac disease in a North American population. Am J Gastroenterol 2013; 108:818-24; PMID:23511460; http://dx.doi.org/10.1038/ajg.2013.60
- Rubio-Tapia A, Ludvigsson JF, Brantner TL, Murray JA, Everhart JE. The prevalence of celiac disease in the United States. Am J Gastroenterol 2012; 107:1538-44; PMID:22850429; http://dx.doi.org/10.1038/ajg.2012.219
- Skripak JM, Matsui EC, Mudd K, Wood RA. The natural history of IgE-mediated cow's milk allergy. J Allergy Clin Immunol 2007; 120:1172-7; PMID:17935766; http://dx.doi.org/10.1016/j.jaci.2007.08.023
- Branum AM, Lukacs SL. Food allergy among children in the United States. Pediatrics 2009; 124:1549-55; PMID:19917585; http://dx.doi.org/10.1542/peds.2009-1210
- Catassi C, Gatti S, Fasano A. The new epidemiology of celiac disease. J Pediatr Gastroenterol Nutr 2014; 59:S7-S9; PMID:24979197; http://dx.doi.org/10.1097/01.mpg.0000450393.23156.59
- Catassi C, Kryszak D, Bhatti B, Sturgeon C, Helzlsouer K, Clipp SL, Gelfond D, Puppa E, Sferruzza A, Fasano A. Natural history of celiac disease autoimmunity in a USA cohort followed since 1974. Ann Med 2010; 42:530-8; PMID:20868314; http://dx.doi.org/10.3109/07853890.2010.514285
- Sanz Y, Pama GD, Laparra M. Unraveling the ties between celiac disease and intestinal Microbiota. Int Rev Immunol 2011; 30:207-18; PMID:21787226; http://dx.doi.org/10.3109/08830185.2011.599084
- Nakayama J, Kobayashi T, Tanaka S, Korenori Y, Tateyama A, Sakamoto N, Kiyohara C, Shirakawa T, Sonomoto K. Aberrant structures of fecal bacterial community in allergic infants profiled by 16S rRNA gene pyrosequencing. FEMS Immunol Med Microbiol 2011; 63:397-406; PMID:22029688; http://dx.doi.org/10.1111/j.1574-695X.2011.00872.x
- Ludvigsson JF, Leffler DA, Bai JC, Biagi F, Fasano A, Green PH, Hadjivassiliou M, Kaukinen K, Kelly CP, Leonard JN, et al. The Oslo definitions for coeliac disease and related terms. Gut 2013; 62:45-52; http://dx.doi.org/10.1136/gutjnl-2011-301346
- Clemente JC, Ursell LK, Parfrey LW, Knight R. The impact of the gut microbiota on human health: an integrative view. Cell 2012; 148:1258-70; PMID:22424233; http://dx.doi.org/10.1016/j.cell.2012.01.035
- Matamoros S, Gras-Leguen C, Le Vacon F, Potel G, de La Cochetiere M-F. Development of intestinal microbiota in infants and its impact on health. Trends Microbiol 2013; 21:167-73; PMID:23332725; http://dx.doi.org/10.1016/j.tim.2012.12.001
- Koenig JE, Spor A, Scalfone N, Fricker AD, Stombaugh J, Knight R, Angenent LT, Ley RE. Succession of microbial consortia in the developing infant gut microbiome. Proc Natl Acad of Sci U S A 2011; 108:4578-85; http://dx.doi.org/10.1073/pnas.1000081107
- Yatsunenko T, Rey FE, Manary MJ, Trehan I, Dominguez-Bello MG, Contreras M, Magris M, Hidalgo G, Baldassano RN, Anokhin AP, et al. Human gut microbiome viewed across age and geography. Nature 2012; 486:222-7; PMID:22699611
- Cho I, Blaser MJ. The human microbiome: at the interface of health and disease. Nat Rev Genet 2012; 13:260-70; PMID:22411464
- Smith K, McCoy KD, Macpherson AJ. Use of axenic animals in studying the adaptation of mammals to their commensal intestinal microbiota. Semin Immunol 2007; 19:59-69; PMID:17118672; http://dx.doi.org/10.1016/j.smim.2006.10.002
- Geuking MB, Cahenzli J, Lawson MA, Ng DC, Slack E, Hapfelmeier S, McCoy KD, Macpherson AJ. Intestinal bacterial colonization induces mutualistic regulatory T cell responses. Immunity 2011; 34:794-806; PMID:21596591; http://dx.doi.org/10.1016/j.immu-ni.2011.03.021
- Round JL, Mazmanian SK. The gut microbiota shapes intestinal immune responses during health and disease. Nat Rev Immunol 2009; 9:313-23; PMID:19343057; http://dx.doi.org/10.1038/nri2515
- Sommer F, Bäckhed F. The gut microbiota—masters of host development and physiology. Nat Rev Microbiol 2013; 11:227-38; PMID:23435359; http://dx.doi.org/10.1038/nrmicro2974
- El Aidy S, van Baarlen P, Derrien M, Lindenbergh-Kortleve DJ, Hooiveld G, Levenez F, Doré J, Dekker J, Samsom JN, Nieuwenhuis EE, et al. Temporal and spatial interplay of microbiota and intestinal mucosa drive establishment of immune homeostasis in conventionalized mice. Mucosal Immunol 2012; 5:567-79; PMID:22617837; http://dx.doi.org/10.1038/mi.2012.32
- Natividad JM, Hayes CL, Motta J-P, Jury J, Galipeau HJ, Philip V, Garcia-Rodenas CL, Kiyama H, Bercik P, Verdu EF. Differential induction of antimicrobial REGIII by the intestinal microbiota and Bifidobacterium breve NCC2950. Appl Environ Microbiol 2013; 79:7745-54; http://dx.doi.org/10.1128/AEM.02470-13
- Macpherson A, Martinic M, Harris N. The functions of mucosal T cells in containing the indigenous commensal flora of the intestine. Cell Mol Life Sci 2002; 59:2088-96; PMID:12568335; http://dx.doi.org/10.1007/s000180200009
- Imaoka A, Matsumoto S, Setoyama H, Okada Y, Umesaki Y. Proliferative recruitment of intestinal intraepithelial lymphocytes after microbial colonization of germ-free mice. Eur I Immunol 1996; 26:945-8; http://dx.doi.org/10.1002/eji.1830260434
- Helgeland L, Vaage J, Rolstad B, Midtvedt T, Brandtzaeg P. Microbial colonization influences composition and T-cell receptor Vβ repertoire of intraepithelial lymphocytes in rat intestine. Immunology 1996; 89:494-501; PMID:9014812; http://dx.doi.org/10.1046/j.1365-2567.1996.d01-783.x
- Round JL, Mazmanian SK. Inducible Foxp3– regulatory T-cell development by a commensal bacterium of the intestinal microbiota. Proc Natl Acad Sci U S A 2010; 107:12204-9; PMID:20566854; http://dx.doi.org/10.1073/pnas.0909122107
- Uematsu S, Fujimoto K, Jang MH, Yang B-G, Jung Y-J, Nishiyama M, Sato S, Tsujimura T, Yamamoto M, Yokota Y, et al. Regulation of humoral and cellular gut immunity by lamina propria dendritic cells expressing Toll-like receptor 5. Nat Immunol 2008; 9:769-76; PMID:18516037; http://dx.doi.org/10.1038/ni.1622
- Kamada N, Núñez G. Role of the gut microbiota in the development and function of lymphoid cells. J Immunol 2013; 190:1389-95; PMID:23378581; http://dx.doi.org/10.4049/jimmunol.1203100
- Atarashi K, Tanoue T, Shima T, Imaoka A, Kuwahara T, Momose Y, Cheng G, Yamasaki S, Saito T, Ohba Y, et al. Induction of colonic regulatory T cells by indigenous Clostridium species. Science 2011; 331:337-41; PMID:21205640; http://dx.doi.org/10.1126/science.1198469
- Gaboriau-Routhiau V, Rakotobe S, Lécuyer E, Mulder I, Lan A, Bridonneau C, Rochet V, Pisi A, De Paepe M, Brandi G, et al. The key role of segmented filamentous bacteria in the coordinated maturation of gut helper T cell responses. Immunity 2009; 31:677-89; PMID:19833089; http://dx.doi.org/10.1016/j.immuni.2009.08.020
- Ivanov II, Atarashi K, Manel N, Brodie EL, Shima T, Karaoz U, Wei D, Goldfarb KC, Santee CA, Lynch SV, et al. Induction of intestinal Th17 cells by segmented filamentous bacteria. Cell 2009; 139:485-98; PMID:19836068; http://dx.doi.org/10.1016/j.cell.2009.09.033
- Round JL, Lee SM, Li J, Tran G, Jabri B, Chatila TA, Mazmanian SK. The Toll-like receptor 2 pathway establishes colonization by a commensal of the human microbiota. Science 2011; 332:974-7; PMID:21512004; http://dx.doi.org/10.1126/science.1206095
- Atarashi K, Tanoue T, Oshima K, Suda W, Nagano Y, Nishikawa H, Fukuda S, Saito T, Narushima S, Hase K, et al. Treg induction by a rationally selected mixture of Clostridia strains from the human microbiota. Nature 2013; 500:232-6; PMID:23842501; http://dx.doi.org/10.1038/nature12331
- Hrncir T, Stepankova R, Kozakova H, Hudcovic T, Tlaskalova-Hogenova H. Gut microbiota and lipopolysaccharide content of the diet influence development of regulatory T cells: studies in germ-free mice. BMC Immunol 2008; 9:65; PMID:18990206; http://dx.doi.org/10.1186/1471-2172-9-65
- Arpaia N, Campbell C, Fan X, Dikiy S, van der Veeken J, deRoos P, Liu H, Cross JR, Pfeffer K, Coffer PJ, et al. Metabolites produced by commensal bacteria promote peripheral regulatory T-cell generation. Nature 2013; 504:451-5; PMID:24226773; http://dx.doi.org/10.1038/nature12726
- Furusawa Y, Obata Y, Fukuda S, Endo TA, Nakato G, Takahashi D, Nakanishi Y, Uetake C, Kato K, Kato T, et al. Commensal microbe-derived butyrate induces the differentiation of colonic regulatory T cells. Nature 2013; 504:446-50; PMID:24226770; http://dx.doi.org/10.1038/nature12721
- Smith PM, Howitt MR, Panikov N, Michaud M, Gallini CA, Bohlooly-Y M, Glickman JN, Garrett WS. The microbial metabolites, short-chain fatty acids, regulate colonic Treg cell homeostasis. Science 2013; 341:569-73; PMID:23828891; http://dx.doi.org/10.1126/science.1241165
- Berin MC, Sampson HA. Mucosal immunology of food allergy. Curr Biol 2013; 23:R389-R400; PMID:23660362; http://dx.doi.org/10.1016/j.cub.2013.02.043
- Cotter PD, Stanton C, Ross RP, Hill C. The impact of antibiotics on the gut microbiota as revealed by high throughput DNA sequencing. Discov Med 2012; 13:193-9; PMID:22463795
- Hazebrouck S, Przybylski-Nicaise L, Ah-Leung S, Adel-Patient K, Corthier G, Wal JM, Rabot S. Allergic sensitization to bovine β-lactoglobulin: comparison between germ-free and conventional BALBc mice. Int Arch Allergy Immunol 2008; 148:65-72; PMID:18716405; http://dx.doi.org/10.1159/000151507
- Rodriguez B, Prioult G, Bibiloni R, Nicolis I, Mercenier A, Butel MJ, Waligora-Dupriet AJ. Germ-free status and altered caecal subdominant microbiota are associated with a high susceptibility to cow's milk allergy in mice. FEMS Microbiol Ecol 2011; 76:133-44; PMID:21223329; http://dx.doi.org/10.1111/j.1574-6941.2010.01035.x
- Herbst T, Sichelstiel A, Schär C, Yadava K, Bürki K, Cahenzli J, McCoy K, Marsland BJ, Harris NL. Dysregulation of allergic airway inflammation in the absence of microbial colonization. Am J Respir Crit Care Med 2011; 184:198-205; PMID:21471101; http://dx.doi.org/10.1164/rccm.201010-1574OC
- Noval Rivas M, Burton OT, Wise P, Zhang Y-q, Hobson SA, Garcia Lloret M, Chehoud C, Kuczynski J, DeSantis T, Warrington J, et al. A microbiota signature associated with experimental food allergy promotes allergic sensitization and anaphylaxis. J Allergy Clin Immunol 2012; 131:201-12; PMID:23201093; http://dx.doi.org/10.1016/j.jaci.2012.10.026
- Russell SL, Gold MJ, Hartmann M, Willing BP, Thorson L, Wlodarska M, Gill N, Blanchet MR, Mohn WW, McNagny KM et al. Early life antibiotic-driven changes in microbiota enhance susceptibility to allergic asthma. EMBO Rep 2012; 13:440-7; PMID:22422004; http://dx.doi.org/10.1038/embor.2012.32
- Stefka AT, Feehley T, Tripathi P, Qiu J, McCoy K, Mazmanian SK, Tjota MY, Seo GY, Cao S, Theriault BR, et al. Commensal bacteria protect against food allergen sensitization. Proc Natl Acad Sci USA 2014; 111: 13145-50.
- Jabri B, Sollid LM. Tissue-mediated control of immunopathology in coeliac disease. Nat Rev Immunol 2009; 9:858-70; PMID:19935805; http://dx.doi.org/10.1038/nri2670
- White LE, Merrick VM, Bannerman E, Russell RK, Basude D, Henderson P, Wilson DC, Gillett PM. The rising incidence of celiac disease in Scotland. Pediatrics 2013; 132:e924-e31; PMID:24019416; http://dx.doi.org/10.1542/peds.2013-0932
- Murray JA, Dyke CV, Plevak MF, Dierkhising RA, Zinsmeister AR, Melton LJ. Trends in the identification and clinical features of celiac disease in a North American community, 1950-2001. Clin Gastroenterol Hepatol 2003; 1:19-27; PMID:15017513; http://dx.doi.org/10.1053/jcgh.2003.50004
- Mustalahti K, Catassi C, Reunanen A, Fabiani E, Heier M, McMillan S, Murray L, Metzger MH, Gasparin M, Bravi E, et al. The prevalence of celiac disease in Europe: results of a centralized, international mass screening project. Ann Med 2010; 42:587-95; PMID:21070098; http://dx.doi.org/10.3109/07853890.2010.505931
- Fasano A, Berti I, Gerarduzzi T, Not T, Colletti RB, Drago S, Elitsur Y, Green PH, Guandalini S, Hill ID, et al. Prevalence of celiac disease in at-risk and not-at-risk groups in the United States: a large multicenter study. Arch Intern Med 2003; 163:286-92; PMID:12578508; http://dx.doi.org/10.1001/archinte.163.3.286
- Green PH, Cellier C. Celiac disease. N Engl J Med 2007; 357:1731-43; PMID:17960014; http://dx.doi.org/10.1056/NEJMra071600
- Walker MM, Murray JA, Ronkainen J, Aro P, Storskrubb T, D’Amato M, Lahr B, Talley NJ, Agreus L. Detection of celiac disease and lymphocytic enteropathy by parallel serology and histopathology in a population-based study. Gastroenterology 2010; 139:112-9; PMID:20398668; http://dx.doi.org/10.1053/j.gastro.2010.04.007
- Hall NJ, Rubin G, Charnock A. Systematic review: adherence to a gluten-free diet in adult patients with coeliac disease. Aliment Pharmacol Ther 2009; 30:315-30; PMID:19485977; http://dx.doi.org/10.1111/j.1365-2036.2009.04053.x
- Black JL, Orfila C. Impact of coeliac disease on dietary habits and quality of life. J Hum Nutr Diet 2011; 24:582-7; PMID:21615555; http://dx.doi.org/10.1111/j.1365-277X.2011.01170.x
- Sollid LM, Jabri B. Triggers and drivers of autoimmunity: lessons from coeliac disease. Nat Rev Immunol 2013; 13:294-302; PMID:23493116; http://dx.doi.org/10.1038/nri3407
- Shan L, Molberg Ø, Parrot I, Hausch F, Filiz F, Gray GM, Sollid LM, Khosla C. Structural basis for gluten intolerance in celiac sprue. Science 2002; 297:2275-9; PMID:12351792; http://dx.doi.org/10.1126/science.1074129
- Bergseng E, Xia J, Kim C-Y, Khosla C, Sollid LM. Main chain hydrogen bond interactions in the binding of proline-rich gluten peptides to the celiac disease-associated HLA-DQ2 molecule. J Biol Chem 2005; 280:21791-6; PMID:15826953; http://dx.doi.org/10.1074/jbc.M501558200
- Meresse B, Malamut G, Cerf-Bensussan N. Celiac disease: an immunological jigsaw. Immunity 2012; 36:907-19; PMID:22749351; http://dx.doi.org/10.1016/j.immuni.2012.06.006
- Vader W, Stepniak D, Kooy Y, Mearin L, Thompson A, van Rood JJ, Spaenij L, Koning F. The HLA-DQ2 gene dose effect in celiac disease is directly related to the magnitude and breadth of gluten-specific T cell responses. Proc Natl Acad Sci U S A 2003; 100:12390-5; PMID:14530392; http://dx.doi.org/10.1073/pnas.2135229100
- Fallang L-E, Bergseng E, Hotta K, Berg-Larsen A, Kim C-Y, Sollid LM. Differences in the risk of celiac disease associated with HLA-DQ2. 5 or HLA-DQ2. 2 are related to sustained gluten antigen presentation. Nat Immunol 2009; 10:1096-101; PMID:19718029; http://dx.doi.org/10.1038/ni.1780
- Bodd M, Ráki M, Tollefsen S, Fallang L, Bergseng E, Lundin K, Sollid LM. HLA-DQ2-restricted gluten-reactive T cells produce IL-21 but not IL-17 or IL-22. Mucosal Immunol 2010; 3:594-601; PMID:20571486; http://dx.doi.org/10.1038/mi.2010.36
- Nilsen EM, Jahnsen FL, Lundin KE, Johansen FE, Fausa O, Sollid LM, Jahnsen J, Scott H, Brandtzaeg P. Gluten induces an intestinal cytokine response strongly dominated by interferon gamma in patients with celiac disease. Gastroenterology 1998; 115:551-63; PMID:9721152; http://dx.doi.org/10.1016/S0016-5085(98)70134-9
- Molberg Ø, McAdam SN, Körner R, Quarsten H, Kristiansen C, Madsen L, Fugger L, Scott H, Norén O, Roepstorff P, et al. Tissue transglutaminase selectively modifies gliadin peptides that are recognized by gut-derived T cells in celiac disease. Nat Med 1998; 4:713-7; PMID:9623982; http://dx.doi.org/10.1038/nm0698-713
- Klöck C, DiRaimondo TR, Khosla C. Role of transglutaminase 2 in celiac disease pathogenesis. Semin Immunopathol 2012; 34:513-22; PMID:22437759; http://dx.doi.org/10.1007/s00281-012-0305-0
- Siegel M, Strnad P, Watts RE, Choi K, Jabri B, Omary MB, Khosla C. Extracellular transglutaminase 2 is catalytically inactive, but is transiently activated upon tissue injury. PLoS One 2008; 3:e1861; PMID:18365016; http://dx.doi.org/10.1371/journal.pone.0001861
- Kutlu T, Brousse N, Rambaud C, Le Deist F, Schmitz J, Cerf-Bensussan N. Numbers of T cell receptor (TCR) alpha beta– but not of TcR gamma delta– intraepithelial lymphocytes correlate with the grade of villous atrophy in coeliac patients on a long term normal diet. Gut 1993; 34:208-14; PMID:8432475; http://dx.doi.org/10.1136/gut.34.2.208
- Meresse B, Chen Z, Ciszewski C, Tretiakova M, Bhagat G, Krausz TN, Raulet DH, Lanier LL, Groh V, Spies T, et al. Coordinated induction by IL15 of a TCR-independent NKG2D signaling pathway converts CTL into lymphokine-activated killer cells in celiac disease. Immunity 2004; 21:357-66; PMID:15357947; http://dx.doi.org/10.1016/j.immuni.2004.06.020
- DePaolo RW, Abadie V, Tang F, Fehlner-Peach H, Hall JA, Wang W, Marietta EV, Kasarda DD, Waldmann TA, Murray JA, et al. Co-adjuvant effects of retinoic acid and IL-15 induce inflammatory immunity to dietary antigens. Nature 2011; 471:220-4; PMID:21307853; http://dx.doi.org/10.1038/nature09849
- Meresse B, Curran SA, Ciszewski C, Orbelyan G, Setty M, Bhagat G, Lee L, Tretiakova M, Semrad C, Kistner E, et al. Reprogramming of CTLs into natural killer–like cells in celiac disease. J Exp Med 2006; 203:1343-55; PMID:16682498; http://dx.doi.org/10.1084/jem.20060028
- Hüe S, Mention J-J, Monteiro RC, Zhang S, Cellier C, Schmitz J, Verkarre V, Fodil N, Bahram S, Cerf-Bensussan N, et al. A direct role for NKG2DMICA interaction in villous atrophy during celiac disease. Immunity 2004; 21:367-77; PMID:15357948; http://dx.doi.org/10.1016/j.immuni.2004.06.018
- Bhagat G, Naiyer AJ, Shah JG, Harper J, Jabri B, Wang TC, Green PH, Manavalan JS. Small intestinal CD8– TCRγδ– NKG2A– intraepithelial lymphocytes have attributes of regulatory cells in patients with celiac disease. J Clin Invest 2008; 118:281-93; PMID:18064301; http://dx.doi.org/10.1172/JCI30989
- Rossi M, Schwartz KB. Editorial: Celiac disease and intestinal bacteria: not only gluten? J Leukoc Biol 2010; 87:749-51; PMID:20430780; http://dx.doi.org/10.1189/jlb.1209784
- Lohi S, Mustalahti K, Kaukinen K, Laurila K, Collin P, Rissanen H, Lohi O, Bravi E, Gasparin M, Reunanen A, et al. Increasing prevalence of coeliac disease over time. Aliment Pharmacol Ther 2007; 26:1217-25; PMID:17944736; http://dx.doi.org/10.1111/j.1365-2036.2007.03502.x
- Grölund M-M, Lehtonen O-P, Eerola E, Kero P. Fecal microflora in healthy infants born by different methods of delivery: permanent changes in intestinal flora after cesarean delivery. J Pediatr Gastroenterol Nutr 1999; 28:19-25; PMID:9890463; http://dx.doi.org/10.1097/00005176-199901000-00007
- Jakobsson HE, Abrahamsson TR, Jenmalm MC, Harris K, Quince C, Jernberg C, Björkstén B, Engstrand L, Andersson AF. Decreased gut microbiota diversity, delayed Bacteroidetes colonisation and reduced Th1 responses in infants delivered by caesarean section. Gut 2013; 63:559-66; PMID:23926244; http://dx.doi.org/10.1136/gutjnl-2012-303249
- Salminen S, Gibson G, McCartney A, Isolauri E. Influence of mode of delivery on gut microbiota composition in seven year old children. Gut 2004; 53:1388-9; PMID:15306608; http://dx.doi.org/10.1136/gut.2004.041640
- Dominguez-Bello MG, Costello EK, Contreras M, Magris M, Hidalgo G, Fierer N, Knight R. Delivery mode shapes the acquisition and structure of the initial microbiota across multiple body habitats in newborns. Proc Natl Acad Sci U S A 2010; 107:11971-5; PMID:20566857; http://dx.doi.org/10.1073/pnas.1002601107
- Huurre A, Kalliomäki M, Rautava S, Rinne M, Salminen S, Isolauri E. Mode of delivery–effects on gut microbiota and humoral immunity. Neonatology 2007; 93:236-40; PMID:18025796; http://dx.doi.org/10.1159/000111102
- MacDorman MF, Menacker F, Declercq E. Cesarean birth in the United States: epidemiology, trends, and outcomes. Clin perinatol 2008; 35:293-307; PMID:18456070; http://dx.doi.org/10.1016/j.clp.2008.03.007
- Decker E, Engelmann G, Findeisen A, Gerner P, Laaβ M, Ney D, Posovszky C, Hoy L, Hornef MW. Cesarean delivery is associated with celiac disease but not inflammatory bowel disease in children. Pediatrics 2010; 125:e1433-e40; PMID:20478942; http://dx.doi.org/10.1542/peds.2009-2260
- Mårild K, Stephansson O, Montgomery S, Murray JA, Ludvigsson JF. Pregnancy outcome and risk of celiac disease in offspring: a nationwide case-control study. Gastroenterology 2012; 142:39-45. e3; http://dx.doi.org/10.1053/j.gastro.2011.09.047
- Jakobsson HE, Jernberg C, Andersson AF, Sjölund-Karlsson M, Jansson JK, Engstrand L. Short-term antibiotic treatment has differing long-term impacts on the human throat and gut microbiome. PLoS One 2010; 5:e9836; PMID:20352091; http://dx.doi.org/10.1371/journal.pone.0009836
- Jernberg C, Löfmark S, Edlund C, Jansson JK. Long-term ecological impacts of antibiotic administration on the human intestinal microbiota. ISME J 2007; 1:56-66; PMID:18043614; http://dx.doi.org/10.1038/ismej.2007.3
- Dethlefsen L, Huse S, Sogin ML, Relman DA. The pervasive effects of an antibiotic on the human gut microbiota, as revealed by deep 16S rRNA sequencing. PLoS Biol 2008; 6:e280; PMID:19018661; http://dx.doi.org/10.1371/journal.pbio.0060280
- De La Cochetiere M, Durand T, Lepage P, Bourreille A, Galmiche J, Dore J. Resilience of the dominant human fecal microbiota upon short-course antibiotic challenge. J Clin Microbiol 2005; 43:5588-92; PMID:16272491; http://dx.doi.org/10.1128/JCM.43.11.5588-5592.2005
- Willing BP, Russell SL, Finlay BB. Shifting the balance: antibiotic effects on host–microbiota mutualism. Nat Rev Microbiol 2011; 9:233-43; PMID:21358670; http://dx.doi.org/10.1038/nrmicro2536
- Cash HL, Whitham CV, Behrendt CL, Hooper LV. Symbiotic bacteria direct expression of an intestinal bactericidal lectin. Science 2006; 313:1126-30; PMID:16931762; http://dx.doi.org/10.1126/science.1127119
- Brandl K, Plitas G, Mihu CN, Ubeda C, Jia T, Fleisher M, Schnabl B, DeMatteo RP, Pamer EG. Vancomycin-resistant enterococci exploit antibiotic-induced innate immune deficits. Nature 2008; 455:804-7; PMID: 18724361; http://dx.doi.org/10.1038/nature07250
- Mårild K, Ye W, Lebwohl B, Green PH, Blaser MJ, Card T, Ludvigsson JF. Antibiotic exposure and the development of coeliac disease: a nationwide case–control study. BMC Gastroenterol 2013; 13:109; http://dx.doi.org/10.1186/1471-230X-13-109
- Canova C, Zabeo V, Pitter G, Romor P, Baldovin T, Zanotti R, Simonato L. Association of maternal education, early infections, and antibiotic use with celiac disease: a population-based birth cohort study in Northeastern Italy. Am J Epidemiol 2014; 180:76-85; PMID:24853109; http://dx.doi.org/10.1093/aje/kwu101
- Myléus A, Hernell O, Gothefors L, Hammarström M-L, Persson L-Å, Stenlund H, Ivarsson A. Early infections are associated with increased risk for celiac disease: an incident case-referent study. BMC Pediatr 2012; 12:194; http://dx.doi.org/10.1186/1471-2431-12-194
- Jernberg C, Löfmark S, Edlund C, Jansson JK. Long-term impacts of antibiotic exposure on the human intestinal microbiota. Microbiology 2010; 156:3216-23; PMID:20705661; http://dx.doi.org/10.1099/mic.0.040618-0
- Wu GD, Chen J, Hoffmann C, Bittinger K, Chen Y-Y, Keilbaugh SA, Bewtra M, Knights D, Walters WA, Knight R, et al. Linking long-term dietary patterns with gut microbial enterotypes. Science 2011; 334:105-8; PMID:21885731; http://dx.doi.org/10.1126/science.1208344
- Turnbaugh PJ, Ridaura VK, Faith JJ, Rey FE, Knight R, Gordon JI. The effect of diet on the human gut microbiome: a metagenomic analysis in humanized gnotobiotic mice. Sci Transl Med 2009; 1:6ra14; PMID:20368178; http://dx.doi.org/10.1126/scitranslmed.3000322
- Martín V, Maldonado-Barragán A, Moles L, Rodriguez-Baños M, del Campo R, Fernández L, Rodríguez JM, Jiménez E. Sharing of bacterial strains between breast milk and infant feces. J Hum Lact 2012; 28:36-44; http://dx.doi.org/10.1177/0890334411424729
- De Palma G, Capilla A, Nova E, Castillejo G, Varea V, Pozo T, Garrote JA, Polanco I, López A, Ribes-Koninckx C, et al. Influence of milk-feeding type and genetic risk of developing coeliac disease on intestinal microbiota of infants: the PROFICEL study. PLoS One 2012; 7:e30791; PMID:22319588; http://dx.doi.org/10.1371/journal.pone.0030791
- Sellitto M, Bai G, Serena G, Fricke WF, Sturgeon C, Gajer P, White JR, Koenig SS, Sakamoto J, Boothe D, et al. Proof of concept of microbiome-metabolome analysis and delayed gluten exposure on celiac disease autoimmunity in genetically at-risk infants. PLoS One 2012; 7:e33387; PMID:22432018; http://dx.doi.org/10.1371/journal.pone.0033387
- Ivarsson A, Persson L, Nyström L, Ascher H, Cavell B, Danielsson L, Dannaeus A, Lindberg T, Lindquist B, Stenhammar L, et al. Epidemic of coeliac disease in Swedish children. Acta Paediatr 2000; 89:165-71; PMID:10709885; http://dx.doi.org/10.1111/j.1651-2227.2000.tb01210.x
- Ou G, Hedberg M, Hörstedt P, Baranov V, Forsberg G, Drobni M, Sandström O, Wai SN, Johansson I, Hammarström ML, et al. Proximal small intestinal microbiota and identification of rod-shaped bacteria associated with childhood celiac disease. Am J Gastroenterol 2009; 104:3058-67; PMID:19755974; http://dx.doi.org/10.1038/ajg.2009.524
- Nadal I, Donant E, Ribes-Koninckx C, Calabuig M, Sanz Y. Imbalance in the composition of the duodenal microbiota of children with coeliac disease. J Med Microbiol 2007; 56:1669-74; PMID:18033837; http://dx.doi.org/10.1099/jmm.0.47410-0
- De Palma G, Nadal I, Medina M, Donat E, Ribes-Koninckx C, Calabuig M, Sanz Y. Intestinal dysbiosis and reduced immunoglobulin-coated bacteria associated with coeliac disease in children. BMC Microbiol 2010; 10:63; PMID:20181275; http://dx.doi.org/10.1186/1471-2180-10-63
- Collado MC, Donat E, Ribes-Koninckx C, Calabuig M, Sanz Y. Specific duodenal and faecal bacterial groups associated with paediatric coeliac disease. J Clin Pathol 2009; 62:264-9; PMID:18996905; http://dx.doi.org/10.1136/jcp.2008.061366
- Collado M, Donat E, Ribes-Koninckx C, Calabuig M, Sanz Y. Imbalances in faecal and duodenal Bifidobacterium species composition in active and non-active coeliac disease. BMC Microbiol 2008; 8:232; PMID:19102766; http://dx.doi.org/10.1186/1471-2180-8-232
- Sánchez E, Laparra J, Sanz Y. Discerning the role of Bacteroides fragilis in celiac disease pathogenesis. Appl Environ Microbiol 2012; 78:6507-15; http://dx.doi.org/10.1128/AEM.00563-12
- Di Cagno R, De Angelis M, De Pasquale I, Ndagijimana M, Vernocchi P, Ricciuti P, Gagliardi F, Laghi L, Crecchio C, Guerzoni ME, et al. Duodenal and faecal microbiota of celiac children: molecular, phenotype and metabolome characterization. BMC Microbiol 2011; 11:219; PMID:21970810; http://dx.doi.org/10.1186/1471-2180-11-219
- Sánchez E, Donat E, Ribes-Koninckx C, Fernández-Murga ML, Sanz Y. Duodenal-mucosal bacteria associated with celiac disease in children. Appl Environ Microbiol 2013; 79:5472-9; http://dx.doi.org/10.1128/AEM.00869-13
- Nistal E, Caminero A, Vivas S, Ruiz de Morales JM, Sáenz de Miera LE, Rodríguez-Aparicio LB, Casqueiro J. Differences in Faecal bacteria populations and Faecal bacteria metabolism in healthy adults and celiac disease patients. Biochimie 2012; 94:1724-9; PMID:22542995; http://dx.doi.org/10.1016/j.biochi.2012.03.025
- Wacklin P, Kaukinen K, Tuovinen E, Collin P, Lindfors K, Partanen J, Mäki M, Mättö J. The duodenal microbiota composition of adult celiac disease patients is associated with the clinical manifestation of the disease. Inflamm Bowel Dis 2013; 19:934-41; PMID:23478804; http://dx.doi.org/10.1097/MIB.0b013e31828029a9
- Schippa S, Iebba V, Barbato M, Di Nardo G, Totino V, Checchi M, Longhi C, Maiella G, Cucchiara S, Conte MP. A distinctive ‘microbial signature’ in celiac pediatric patients. BMC Microbiol 2010; 10:175; PMID:20565734; http://dx.doi.org/10.1186/1471-2180-10-175
- Sánchez E, Donat E, Ribes-Koninckx C, Calabuig M, Sanz Y. Intestinal Bacteroides species associated with coeliac disease. J Clin Pathol 2010; 63:1105-11; http://dx.doi.org/10.1136/jcp.2010.076950
- De Palma G, Nadal I, Collado MC, Sanz Y. Effects of a gluten-free diet on gut microbiota and immune function in healthy adult human subjects. Br J Nutr 2009; 102:1154-60; PMID:19445821; http://dx.doi.org/10.1017/S0007114509371767
- Tjellström B, Stenhammar L, Högberg L, Fälth-Magnusson K, Magnusson KE, Midtvedt T, Sundqvist T, Norin E. Gut microflora associated characteristics in children with celiac disease. Am J Gastroenterol 2005; 100:2784-8; PMID:16393236; http://dx.doi.org/10.1111/j.1572-0241.2005.00313.x
- Tjellström B, Högberg L, Stenhammar L, Fälth-Magnusson K, Magnusson K-E, Norin E, Sundqvist T, Midtvedt T. Faecal short-chain fatty acid pattern in childhood coeliac disease is normalised after more than one year's gluten-free diet. Microb Ecol Health Dis 2013; 24:20905; PMID:24082880[
- Sánchez E, Nadal I, Donat E, Ribes-Koninckx C, Calabuig M, Sanz Y. Reduced diversity and increased virulence-gene carriage in intestinal enterobacteria of coeliac children. BMC Gastroenterol 2008; 8:50; http://dx.doi.org/10.1186/1471-230X-8-50
- Medina M, De Palma G, Ribes-Koninckx C, Calabuig M, Sanz Y. Bifidobacterium strains suppress in vitro the pro-inflammatory milieu triggered by the large intestinal microbiota of coeliac patients. J Inflamm (Lond) 2008; 5:19; PMID:18980693; http://dx.doi.org/10.1186/1476-9255-5-19
- De Palma G, Cinova J, Stepankova R, Tuckova L, Sanz Y. Pivotal advance: Bifidobacteria and Gram-negative bacteria differentially influence immune responses in the proinflammatory milieu of celiac disease. J Leukoc Biol 2010; 87:765-78; PMID:20007908; http://dx.doi.org/10.1189/jlb.0709471
- De Palma G, Kamanova J, Cinova J, Olivares M, Drasarova H, Tuckova L, Sanz Y. Modulation of phenotypic and functional maturation of dendritic cells by intestinal bacteria and gliadin: relevance for celiac disease. J Leukoc Biol 2012; 92:1043-54; PMID:22891290; http://dx.doi.org/10.1189/jlb.1111581
- Sjöberg V, Sandström O, Hedberg M, Hammarström S, Hernell O, Hammarström M-L. Intestinal T-cell responses in celiac disease–impact of celiac disease associated bacteria. PLoS One 2013; 8:e53414; PMID:23326425; http://dx.doi.org/10.1371/journal.pone.0053414
- Laparra JM, Sanz Y. Bifidobacteria inhibit the inflammatory response induced by gliadins in intestinal epithelial cells via modifications of toxic peptide generation during digestion. J Cell Biochem 2010; 109:801-7; PMID:20052669
- Laparra JM, Olivares M, Gallina O, Sanz Y. Bifidobacterium longum CECT 7347 modulates immune responses in a gliadin-induced enteropathy animal model. PLoS One 2012; 7:e30744; PMID:22348021; http://dx.doi.org/10.1371/journal.pone.0030744
- Lindfors K, Blomqvist T, Juuti-Uusitalo K, Stenman S, Venäläinen J, Mäki M, Kaukinen K. Live probiotic Bifidobacterium lactis bacteria inhibit the toxic effects induced by wheat gliadin in epithelial cell culture. Clin Exp Immunol 2008; 152:552-8; PMID:18422736; http://dx.doi.org/10.1111/j.1365-2249.2008.03635.x
- Cinova J, De Palma G, Stepankova R, Kofronova O, Kverka M, Sanz Y, Tuckova L. Role of intestinal bacteria in gliadin-induced changes in intestinal mucosa: study in germ-free rats. PLoS One 2011; 6:e16169; PMID:21249146; http://dx.doi.org/10.1371/journal.pone.0016169
- Smecuol E, Hwang HJ, Sugai E, Corso L, Cherñavsky AC, Bellavite FP, González A, Vodánovich F, Moreno ML, Vázquez H, et al. Exploratory, randomized, double-blind, placebo-controlled study on the effects of Bifidobacterium infantis natren life start strain super strain in active celiac disease. J Clin Gastroenterol 2013; 47:139-47; PMID:23314670; http://dx.doi.org/10.1097/MCG.0b013e31827759ac
- Štepánková R, Tlaskalova-Hogenova H, Šinkora J, Jodl J, Fric P. Changes in jejunal mucosa after long-term feeding of germfree rats with gluten. Scand J Gastroenterol 1996; 31:551-7; http://dx.doi.org/10.3109/00365529609009127
- Galipeau HJ, Rulli NE, Jury J, Huang X, Araya R, Murray JA, David CS, Chirdo FG, McCoy KD, Verdu EF. Sensitization to gliadin induces moderate enteropathy and insulitis in nonobese diabetic-DQ8 mice. J Immunol 2011; 187:4338-46; PMID:21911598; http://dx.doi.org/10.4049/jimmunol.1100854
- Galipeau H, McCarville JL, Moeller S, Murray JA, Alaedini A, Jabri B, Verdu EF. Gluten-induced responses in NODDQ8 mice are influenced by bacterial colonization. Gastroenterology 2014; 146:S-833; http://dx.doi.org/10.1016/S0016-5085(14)63025-0