Abstract
The nuclear lamina has been shown to interact with the genome through lamina-associated domains (LADs). LADs have been identified by DamID, a proximity labeling assay, and more recently by chromatin immunoprecipitation-sequencing (ChIP-seq) of A- and B-type lamins. LADs form megabase-size domains at the nuclear periphery, they are gene-poor and mostly heterochromatic. Here, we show that the mode of chromatin fragmentation for ChIP, namely bath sonication or digestion with micrococcal nuclease (MNase), leads to the discovery of common but also distinct sets of lamin-interacting domains, or LiDs. Using ChIP-seq, we show the existence of lamin A/C (LMNA) LiDs with distinct gene contents, histone composition enrichment and relationships to lamin B1-interacting domains. The extent of genome coverage of lamin A/C (LMNA) LiDs in sonicated or MNase-digested chromatin is similar (∼730 megabases); however over half of these domains are uniquely detected in sonicated or MNase-digested chromatin. Sonication-specific LMNA LiDs are gene-poor and devoid of a broad panel of histone modifications, while MNase-specific LMNA LiDs are of higher gene density and are enriched in H3K9me3, H3K27me3 and in histone variant H2A.Z. LMNB1 LiDs are gene-poor and show no or little enrichment in these marks. Comparison of published LMNB1 DamID LADs with LMNB1 and LMNA LiDs identified here by ChIP-seq further shows that LMNA can associate with ‘open’ chromatin domains displaying euchromatin characteristics, and which are not associated with LMNB1. The differential genomic and epigenetic properties of lamin-interacting domains reflect the existence of distinct LiD populations identifiable in different chromatin contexts, including nuclease-accessible regions presumably localized in the nuclear interior.
Abbreviations
ChIP | = | chromatin immunoprecipitation |
ChIP-seq | = | ChIP and high-throughput sequencing |
DamID | = | DNA adenine methyltransferase identification |
hPTM | = | histone post-translational modification |
IGV | = | integrated genomic viewer |
LAD | = | lamina-associated domain |
LiD | = | lamin-interacting domain |
MNase | = | micrococcal nuclease |
Introduction
The nuclear lamina, a meshwork of intermediate filament proteins called lamins, encloses the genome of higher eukaryotes and provides anchor points for chromatin at the nuclear periphery.Citation1 Interactions of nuclear lamins with chromatin occur through large genomic areas of ∼0.1–10 megabases (mb). These domains have been termed lamina-associated domains (LADs) with the implication that lamins associate with chromatin only at the nuclear lamina, that is, at the nuclear peripheryCitation2-5 (reviewed inCitation6-8). LADs have initially been discovered by DamID (DNA adenine methyltransferase [Dam] identification, a proximity assay).Citation2 Features of LADs include residence in overall transcriptionally silent heterochromatinCitation2,9 consistent with enrichment of this compartment at the nuclear periphery,Citation5,10,11 and a gene-poor content.Citation2 Large chromatin domains with similar properties have also been identified by chromatin immunoprecipitation (ChIP) of lamin A/C (referred to here as LMNA) or lamin B1 (LMNB1) coupled to array hybridizationCitation12 or high-throughput sequencing (ChIP-seq).Citation13-15 The published datasets reveal significant overlap in genomic coverage of lamin-interacting domains discovered by DamID and ChIPCitation15 despite differences between the 2 methods.Citation16
In the ChIP assay,Citation17 chromatin is fractionated into ∼200–500 base pair (bp) fragments usually by sonication in buffer containing an ionic detergent such as sodium dodecylsulfate,Citation18 or by enzymatic digestion with micrococcal nuclease (MNase).Citation19-23 MNase digests linker DNA between nucleosomes and only starts ‘nibbling’ nucleosomal DNA when digestion is prolonged.Citation24,25 Whereas sonication results in a DNA smear detectable by electrophoresis,Citation18 MNase produces a nucleosome laddering pattern.Citation19,26 Not all nuclear DNA is however equally accessible to MNase: nucleosomes released by MNase are the most easily accessible, reflecting their location in an ‘open’ euchromatic environment. This compartment tends to be enriched in the nuclear interior. In contrast, DNA within more compact chromatin domains is not as easily accessible to MNase and may be under-represented in ChIP assays from nuclease-digested chromatin. Even though some bias may occur in the sensitivity of chromatin to sonication, sonication is arguably less discriminative of various chromatin environments than MNase. In particular, the more compact heterochromatin located at the nuclear periphery and susceptible to interact with the lamina, may be more readily available in chromatin fragmented by sonication than with MNase. This leads to the question of whether the identification of lamin interacting domains by ChIP-seq may be influenced by chromatin fragmentation protocol.
Here, we examine the distribution of chromatin domains interacting with LMNA by a comparative ChIP-seq analysis from chromatin prepared by sonication or MNase digestion. Using an algorithm we designed for the detection of broad enriched genomic domains,Citation15 we show that nuclease digestion or physical fragmentation of chromatin reveals different compartment interacting with LMNA, with distinct gene contents, histone patterns and overlaps with domains interacting with LMNB1. We collectively refer to nuclear lamin-interacting domains as LiDs, so as not to imply any specific localization at the nuclear lamina. We show that LiDs can harbor distinct chromatin properties, and that LMNA LiDs can be found in euchromatic regions not necessarily associated with LMNB1.
Results
Sonication and MNase digestion generate chromatin fragments suitable for identification of lamin-interacting domains by ChIP
We generated ChIP-seq data sets for LMNA in HeLa cells after sonication-based or MNase digestion-based chromatin fragmentation. These procedures produce the expected smears and laddering DNA patterns, respectively (). Note that whereas MNase digestion by itself releases mono-nucleosomes, release lamins into the chromatin supernatant also requires a brief sonication pulse (; Supplementary Figs. S1A, B). We restricted the size of fragments generated by sonication to mainly 160–320 bp (1–2 nucleosomes; ), over a background of longer fragments. MNase digestion produces fragments of mostly one nucleosome, but also poly-nucleosomes (). Western blotting shows that lamins A and C are recovered in each chromatin fraction () and can be immunoprecipitated (). Thus, both sonicated and MNase-digested chromatin preparations are suitable for identification of lamin-interacting domains by ChIP.
Figure 1. Chromatin fragmentation by sonication or digestion with micrococcal nuclease. (A, B) Chromatin fragmentation patterns after (A) Bioruptor® sonication and (B) MNase digestion (0.37 U/106 cells) followed by a brief sonication pulse as described in Materials and Methods. (C) Western blot analysis of lamin A/C in chromatin and pellet fractions after sonication- or MNase-based fragmentation. Total, cell lysate before fragmentation. (D) Western blot of lamin A/C immunoprecipitated (IP) from sonicated or MNase-digested chromatin. Total, cell lysate; input, chromatin before immunoprecipitation; beads-only were used as negative controls.
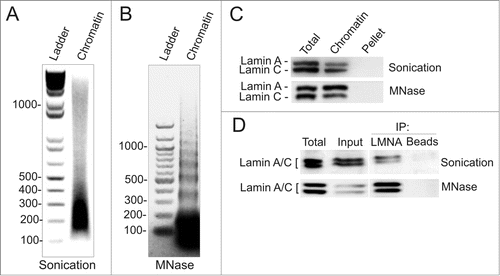
Sonication and MNase digestion generate chromatin fractions differentially enriched in LMNA-interacting domains
To visualize LMNA enrichment profiles genome-wide, we calculated the ratios of ChIP/input sequence reads in consecutive 10 kilobase (kb) bins throughout the genome. Integrated Genomics Viewer (IGV) browser views of these ratios reveal domains of LMNA enrichment with regions of overlap between sonicated and MNase-digested chromatin (). Using enriched domain detector (EDD), an algorithm we developed for detection of wide genomic domains,Citation15 we identify LMNA LiDs in both chromatin preparations (). LiD coverage in both chromatin preparations is similar (730 mb; ), representing 23% of the genome, and consistent with our earlier analysis of fibroblasts.Citation15 The overlap between ‘sonication LiDs’ and ‘MNase LiDs’ (326.1 mb) is, however, surprisingly moderate, accounting for 45% of the total sonication or MNase LiD coverage (). We also identify ∼400 mb that are unique to either sonication or MNase LiDs, with individual domains extending to tens of megabases (, boxed areas). We nonetheless find that sonication LiDs in HeLa cells more robustly overlap sonication LiDs in fibroblastsCitation15 than MNase LiDs do (P < 10−4, Fisher's exact test; ). These data indicate that chromatin fragmentation protocol influences the detection of LiDs by ChIP-seq, and that domains of LMNA interaction exist in an MNase-accessible chromatin environment.
Figure 2. Identification of LMNA LiDs from sonicated and MNase-digested chromatin. (A) IGV browser views of LMNA profiles (log IP/Input ratios) and LMNA LiDs discovered using EDD in chromosome 4 in HeLa cells and AD04DF fibroblasts. RefSeq genes are shown. (B) Venn diagram of genome coverage by LMNA LiDs in sonicated and MNase-digested HeLa chromatin, and in sonicated fibroblast chromatin. AD04DF data are from Lund et al.Citation15 (C) IGV browser views of domains differentially enriched in LMNA depending on chromatin preparation procedure. Scale shown is for (A) and (C).
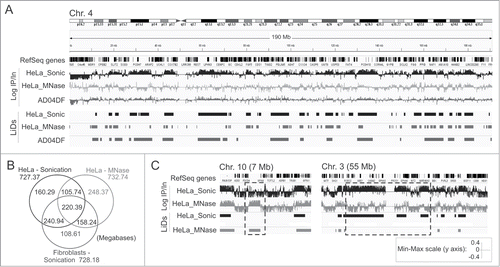
Validation of common and distinct LMNA enrichment sites in sonicated and MNase-digested chromatin
The detection of LiDs common or unique to MNase-digested and sonicated chromatin fractions was validated by ChIP-qPCR analysis of LMNA. We examined 5 promoter regions for which we previously reported interaction patterns with LMNA,Citation12 3 gene bodies and 3 intergenic regions. We determined whether these regions mapped to LiDs in our HeLa ChIP-seq datasets (Supplementary Table S1, LiD information). Using IGV, we also assessed whether our PCR amplicons were localized in 10 kb bins enriched in LMNA or not enriched in LMNA, regardless of whether a bin is found within a LiD or not (Supplementary Table S1, LMNA-bound; ).
Figure 3. ChIP-qPCR validation of LMNA-associated sites detected by ChIP-seq from sonicated and MNase-digested chromatin. (A) Enlarged IGV browser views of LMNA association with indicated genes, detected by ChIP-seq. Upper two tracks show log IP/Input ratios in 10 kb bins (one “block” represents one 10 kb bin). LiD segments are shown in the lower tracks. Arrows show position of amplicons examined by qPCR. (B) ChIP-qPCR analysis of LMNA association with indicated loci, from sonicated and MNase-digested chromatin. See Supplementary Figure S2 for views of LMNA enrichment in additional genes and intergenic regions.
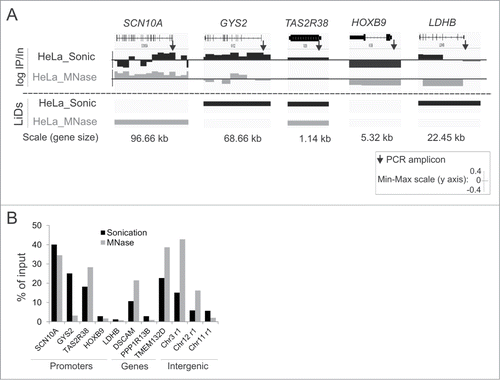
shows, for MNase-digested and sonicated chromatin, ChIP/Input log ratios for LMNA in consecutive 10 kb bins for indicated genes (top panels), and whether these genes are localized in a LiD or not (bottom panels; bar [LiD] or no bar [no LiD]). Consistent with our earlier findings,Citation12 a gene may be partially associated with LMNA and yet be localized outside a LiD (e.g. SCN10A, Sonic) or within a LiD (e.g., LDHB, Sonic), or a gene may be entirely bound by LMNA and found in a LiD (e.g. SCN10A, MNase; GYS2, sonic; TAS2R38, Sonic and MNase). This is also observed for entire gene bodies or intergenic regions (Supplementary Fig. S2). We also note that a given genomic site, such as a PCR amplicon (, arrows), may map to a LiD even though the 10 kb bin within which it localizes is not enriched in LMNA (e.g., LDHB, Sonic). Conversely, a site outside a LiD may be in a 10 kb bin enriched in LMNA (e.g. SCN10A, Sonic); however this does not imply that this particular site itself interacts with LMNA, because a bin may be enriched in LMNA even though a particular locus within that bin does not by itself interact with LMNA.
Keeping this in mind, ChIP-qPCR data show robust consistency with ChIP-seq data (; Supplementary Fig. S2). We identify in both analyses sites interacting with LMNA in sonicated and MNase-digested chromatin (SCN10A and TAS2R38 promoters, DSCAM and TMEM132D genes), sites consistently not interacting with LMNA (HOXB9 and LDHB promoters, PPP1R13B gene, chr11r1 intergenic region), and sites uniquely interacting with LMNA in sonicated or MNase-digested chromatin (GYS2 promoter, chr3r1 and chr12r1 intergenic regions). These results confirm the identification of LiDs common, but also unique, to chromatin prepared by sonication or MNase digestion. Our data further reinforce the view that LMNA can associate with domains of chromatin accessible to MNase, and thus in an ‘open’ configuration. This is consistent with the existence of a pool of intranuclear lamin A/C,Citation27,28 which cannot be excluded from interacting with chromatin.
LiDs identified in MNase-digested chromatin have distinct properties from LiDs mapped to sonicated chromatin
LADs identified by DamIDCitation2,4 are overall gene-poor and enriched in repressive chromatin modifications. We find that LMNA sonication LiDs contain 1.9 genes per mb, which is below genome average (6.18 genes/mb); however gene density of MNase-specific LiDs is twice that of sonication-specific LiDs (3.6 genes/mb; ). We find 770 genes within sonication-specific LiDs (), mainly involved in receptor signaling, while genes found in MNase-specific LiDs (1465 genes) are involved in transcription factor binding (Supplementary Table S2). Genes in LiDs common to MNase and sonicated chromatin (631 genes) are primarily involved in signaling functions. The clear distinction between gene ontology terms enriched among genes found in sonication- vs. MNase-specific LiDs and the robustness of P-values associated with these terms further support the existence of LiDs localized in distinct chromatin compartments.
Figure 4. Genomic, epigenetic and gene expression characterization of LMNA LiDs mapped to sonicated and MNase-digested chromatin. (A) Number of protein-coding RefSeq genes per megabase of genome in LiDs unique or common to sonicated or MNase-digested chromatin. Genes were attributed to LiDs based on localization of the TSS within or outside a LiD. Since genes may have more than one TSS, one gene may be attributed to more than one group, explaining why numbers of genes in “all, only or common” categories do not add up. (B) Observed/expected ratios of enrichment in indicated chromatin marks mapped by ChIP-seq in HeLa cells, determined from public ENCODE data. Genome coverage by each mark is shown in . (C) Median expression level of expressed RefSeq genes (FPKM > 0) included in these LiDs, relative to expression level of all RefSeq genes in the genome. *P < 10−38, Wilcoxon rank-sum test, relative to all other categories.
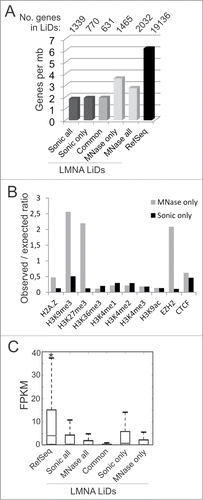
To assess chromatin composition in these LiDs, we determined the extent of co-enrichment in a panel of histone post-translational modifications (hPTMs) and other chromatin marks, using ENCODE ChIP-seq data for HeLa cells (GEO accession number GSE29611). Genome coverage by histone variant H2A.Z, H3K9me3, H3K27me3, H3K36me3, H3K4me1, H3K4me2, H3K4me3, H3K9ac and CTCF is reported in . By computing the observed/expected ratios of enrichment of these marks in the various LiDs, we find that MNase-specific LiDs are strikingly enriched in H3K9me3, H3K27me3 and in the H3K27 histone methyltransferase EZH2; this is in sharp contrast to sonication-specific LiDs which show low levels of these marks (). We also note the enrichment of MNase-specific LiDs in H2A.Z, in line with the localization of H2A.Z in euchromatin or facultative heterochromatinCitation29 and its role in transcription elongation.Citation30,31 We find little or no enrichment in H3K4me1, H3K4me2, H3K4me3, H3K9ac, H3K36me3 or CTCF (), which likely reflects the spatially restricted coverage of these marks. In addition, using RNA-seq data for HeLa cells (GEO accession number GSM958735), we show that a proportion of genes within MNase or sonication LMNA LiDs can be expressed (), again consistent with an association of LMNA with both repressed and active genes.Citation12
Table 1. Genome coverage of LMNA MNAse LiDs and LMNA sonication LiDs by chromatin modifications, EZH2 and CTCF-bound sites in HeLa cells.
Collectively, our results reveal a specific chromatin composition of a subset of LMNA LiDs that are only detected in MNase-digested chromatin. They also indicate that MNase-digested chromatin contains a significant set of lamin-interacting domains identifiable by ChIP and under-represented in sonicated chromatin.
LMNA can associate with chromatin domains harboring euchromatic features and not enriched in LMNB1
The identification of distinct sets of LMNA LiDs suggests that some of these domains may be predominantly euchromatic (accessible to MNase) and enriched in the nuclear interior, while others may be more heterochromatic and concentrated at the nuclear periphery. To address this possibility, we first compared the genome overlap of sonication and MNase LMNA LiDs with LMNB1 LADs recently mapped by DamID in a fibrosarcoma cell line,Citation4 as these domains would be restricted to the nuclear periphery (, LAD tracks). shows that DamID LMNB1 LADs overlap to a greater extent with LMNA sonication LiDs than with LMNA MNase LiDs. With the premise that LMNB1 LADs are restricted to the nuclear periphery, this indicates that a significant proportion of LMNA associates with a chromatin compartment not accessible to LMNB1, and likely enriched in the nuclear interior. This is consistent with independent evidence for the existence of an intranuclear pool of LMNA.Citation27,28,32
Figure 5. LMNA and LMNB1 associate with similar but also distinct chromatin domains. (A) IGV browser views of LMNA and LMNB1 ChIP-seq LiDs, and LMNB1 DamID LADs, on chromosome 2. LiDs are shown for HeLa cells (this study) and DamID LADs are shown for HT1080 cellsCitation4 (1) and fibroblastsCitation2 (2). RefSeq genes are shown. (B, C) Venn diagrams of genome coverage by LMNA LiDs in sonicated and MNase-digested chromatin, and (B) LMNB1 LADs identified by DamID in HT1080 cells or (C) LMNB1 LiDs mapped by ChIP-seq in HeLa cells.
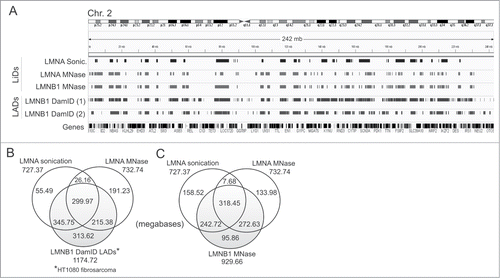
To gain additional insight on chromatin domains interacting with lamins, we next mapped LMNB1-interacting domains by ChIP-seq from MNase-digested chromatin using anti-LMNB1 antibodies (Supplementary Fig. S3A). We identify > 900 mb enriched in LMNB1 in HeLa cells (LMNB1 LiDs; ), which are gene-poor and essentially transcriptionally silent (Supplementary Fig. S3B, C). We find that only ∼10% of LMNB1 LiD coverage is unique to LMNB1, i.e. excluded from LMNA LiDs altogether (), indicating that the vast majority of LMNB1 LiDs are shared with LMNA. Assuming that LMNB1-associated domains mostly represent inactive heterochromatin,Citation2,5 our finding that 272 mb (30%) of LMNB1 MNase LiDs are shared with LMNA MNase-specific LiDs suggests that these LMNA domains also contain this type of chromatin. Nevertheless, the identification of a significant unique fraction of LMNA MNase LiDs (133.98 mb; ) indicates that LMNA can associate with MNase-sensitive domains that are not reached by LMNB1. Lastly, a similar proportion of LMNA sonication LiDs (158 mb; 22%) is unique and not detected in LMNA or LMNB1 MNase domains (; Supplementary Fig. S3D, arrows). We infer from this observation that (i) LMNA sonication-only LiDs may be more heterochromatic than LMNA MNase-only LiDs; (ii) they may be localized at the nuclear periphery but be distinct from LMNB1-interacting domains; and (iii) they may also localize to the nuclear interior.
Discussion
We show that the mode of chromatin preparation influences the discovery of genomic domains interacting with lamins by ChIP-seq. We name these domains LiDs, indistinctively for A- and B-type lamin-interacting domains, to distinguish them from the previously designated lamina-associated domains (LADs), which stricto sensu refer to domains interacting with lamins at the nuclear lamina (as opposed to all nuclear lamins), and hence at the nuclear periphery. Given evidence put forward here and earlierCitation12 that A-type lamins can interact with chromatin not only at the nuclear periphery but also in the nuclear interior, LiDs are by definition less spatially restricted in the nucleus than LADs. We identify here LMNA LiDs specific to MNase-digested or sonicated chromatin, which are differentially enriched in gene density, histone modifications and H2A.Z. LMNA can also associate with chromatin domains with euchromatin features not necessarily associated with LMNB1. Thus LiDs exist in distinct chromatin environments, including a heterochromatic compartment (predominantly at the nuclear periphery), and a more ‘open’ nuclease-accessible compartment.
MNase requires direct access to DNA, thus it preferentially releases nucleosomes from ‘open’ accessible chromatin over more compact heterochromatin. Sonication is probably less discriminative, although bias toward more easily accessible chromatin may also occur, yielding a chromatin fraction likely to contain MNase-resistant and MNase-sensitive domains. Consistent with a localization of LiDs in an MNase-sensitive chromatin environment is their higher gene content as well as enrichment in hPTMs (H3K9me3 and H3K27me3) and in histone variant H2A.Z, relative to sonication LiDs. Since peripheral chromatin is more compact and thereby largely MNase-resistant, our data suggest that at least some MNase-specific LiDs are localized in the nuclear interior. This view is supported by our observations that a significant fraction of LMNA LiDs does not overlap with LMNB1 domains identified by ChIP-seq or DamID. Although it is currently difficult to demonstrate, the concept of ‘internal LADs’ has been proposedCitation5,12 based on fluorescence tagging of lamin-interacting sequences by DamID,Citation5 fluorescence in situ hybridization of LMNA-associated loci,Citation12,33 and the existence of a nucleoplasmic pool of LMNACitation27,28,32 which may interact with chromatin. Interaction of the lamina-associated polypeptide LAP2α with intranuclear A-type laminsCitation27 and association of LAP2α with chromosomesCitation34,35 strongly argue in favor of an intranuclear pool of LMNA directly or indirectly interacting with chromatin.
Features of MNase-specific LiDs resemble those of ‘facultative LADs’ identified by DamID in human and mouse cells.Citation4 While ‘constitutive LADs’ are conserved between cell types, facultative LADs are more cell type-specific. Interestingly, compared to constitutive LADs, facultative LADs appear narrower and have a higher gene content, similarly to MNase LiDs. Facultative LADs also show lower coverage by LINE elements, lower A/T content, and higher coverage by SINE elements than constitutive LADs,Citation4 suggesting a localization in more open chromatin domains. It will be interesting to investigate in detail the biochemical and genomic relationship between MNase-specific LiDs and facultative LADs to determine if similarity can be extended to additional features.
Despite the cross-linking of proteins and DNA in the ChIP procedure used in this study, it is possible that sonication disrupts a subset of lamin-genome contacts which are preserved upon MNase digestion, accounting at least some of the MNase LiDs. Not all lamin-chromatin interactions are likely to be of similar strength throughout the genome, thus sonication, especially in the presence of ionic detergent and in most ChIP protocols), may result in a loss of weaker interactions particularly in loose chromatin contexts. Third, higher gene density of MNase LiDs and co-enrichment in hPTMs imply a higher number of interactions with chromatin remodelers and transcription factors than sonication LiDs located in gene-poor areas or in gene deserts. Thus, molecular occupancy of the genome particularly in accessible chromatin regions may result in weaker or more transient interactions with lamins. Again, these interactions may be lost by sonication, contributing to the detection of MNase-specific LiDs.
Molecular occupancy of genes in MNase LiDs is likely to be related to their chromatin configuration. Compared to sonication LiDs, MNase LiDs are enriched in H2A.Z. Interestingly, in vitro reconstituted H2A.Z-containing nucleosomal arrays are more sensitive to MNase than H2A-containing arrays, likely because H2A.Z facilitates intranucleosomal interactions while inhibiting internucleosomal interactions, i.e., the formation of condensed chromatin.Citation36 Several studies also involve H2A.Z in the relaxation of chromatin, explained by instability of H2A.Z-containing nucleosomes.Citation37,38 Altogether, this provides a logic for H2A.Z detection in MNase LiDs. Additionally, although H2A.Z is positively involved in transcription,Citation30,38-40 it also localizes to poised promoters bound by the Polycomb repressor 2 complex.Citation41,42 This is in line with the enrichment of MNase LiDs in H3K27me3 and in the H3K27 methyltransferase EZH2, a component of this complex.
H3K9 methylation has been linked to localization of DNA sequences or LADs at the nuclear periphery. H3K9me2 is enriched at the nuclear peripheryCitation43 and H3K9 di- and trimethylation has been implicated in peripheral recruitment of LADs in human cellsCitation5 and of gene arrays in worms.Citation11 H3K9me3 enrichment of LiDs agrees with the overall inactive state of genes within LiDs and with our recent observations in adipose progenitor cells.Citation12 As H3K9me3 occupies the promoter of inactive genes, enrichment of H3K9me3 in MNase LiDs relative to sonication LiDs may reflect the higher gene density of MNase LiDs.
The distinct genic and epigenetic properties of LiDs discovered in MNase-digested or in sonicated chromatin strongly argue in favor of the existence of distinct LiD populations in different chromatin environments. In light of our findings, whether MNase, by accessing DNA in open chromatin regions, favors the detection of internal LiDs as opposed to peripheral LiDs is an attractive hypothesis worth of further investigation. It is also possible that by preparing chromatin with MNase we have selected heterochromatin areas with a particular folding state permissive to remodeling or transcription factors loading.Citation38 It will be important to determine the impact of local (e.g., promoter levelCitation12) and global (LiD level) LMNA association and dissociation events from the genome on chromatin remodeling processes.
Materials and Methods
Cells
HeLa cells (American Type Culture Collection; CCL-2) were cultured in MEM medium containing Glutamax (Gibco), 1% non-essential amino acids, 10% fetal calf serum and 1% penicillin/streptomycin.
Chromatin preparation by sonication and MNase digestion
For chromatin preparation by sonication, cells (107 per ChIP) were harvested by trypsinization, washed in PBS and fixed with 1% formaldehyde for 10 min before quenching with 125 mM glycine for 5 min. Cells were lysed for 30 min at 4°C on a rotator in RIPA buffer (140 mM NaCl, 10 mM Tris-HCl, pH 8.0, 1 mM EDTA, 0.5 mM EGTA, 1% Triton X-100, 0.1% SDS, 0.1% deoxycholate, 1 mM PMSF, protease inhibitor cocktail) adjusted to 1% SDS, and sonicated 3 × 15 min in a Bioruptor (Diagenode) using a 30 sec on/off program at high power to produce fragments of predominantly ∼200-400 base pairs (bp). After sedimentation at 10,000 g for 10 min, the supernatant was collected and diluted 10x in RIPA buffer without SDS to constitute the input chromatin used for ChIP.
For chromatin preparation by MNase digestion, cells were fixed with 1% formaldehyde for 10 min, washed and formaldehyde quenched with 125 mM glycine for 5 min. After a wash in PBS, cells were scrapped in cold PBS containing 0.5 mM PMSF, sedimented and suspended in hypotonic buffer (10 mM Tris pH 7.5, 5 mM NaCl, 1.5 mM MgCl2, 0.5 mM DTT, 0.5 mM PMSF, protease inhibitors) for 30 min on ice before Dounce homogenization (50 strokes). MgCl2 was adjusted to 5 mM and NP-40 added to 0.1%. The lysate was kept on ice for 10 min before centrifugation at 2400 g for 10 min. The pellet was resuspended in MNase buffer (20 mM Tris pH 7.5, 15 mM NaCl, 1 mM CaCl2) at 25 × 106 cells/ml and pre-incubated at 37°C for 5 min before adding 0.37 U MNase (Sigma; N5386) per 106 cells. Incubation was at 37°C for 5 min and digestion stopped with 10 mM EDTA on ice for 10 min. Samples were diluted to 8 × 106 cells/ml with lysis buffer (50 mM Tris pH 8.0, 10 mM EDTA, 0.5% SDS, 0.5 mM PMSF and 1x protease inhibitors). Release of digested chromatin and lamins was facilitated by pulse-sonication with a 3 mm probe for 6 times 10 sec (1 sec on/off) at 20% amplitude with cooling on ice between each round (see Results). Samples were centrifuged at 10,000 g for 10 min at 4°C and the supernatants (chromatin fraction) collected.
Chromatin immunoprecipitation
Chromatin fragmented by sonication of 107 cells was incubated on a rotator overnight at 4°C with 25 μg mouse anti-lamin A/C antibodies (Santa Cruz sc-7292) pre-coupled for 2 h to magnetic Dynabeads Protein G (Invitrogen) as described.Citation12 The ChIP sample was collected and washed 3x in 1 ml ice-cold RIPA buffer. Crosslink was reversed and DNA was eluted for 6 h on a shaker at 37°C in elution buffer (50 mM NaCl, 20 mM Tris-HCl pH 7.5, 5 mM EDTA, 1% SDS) containing 0.5 μg/ml RNase A and 2 μg/ml Proteinase K added twice. DNA was purified by phenol-chloroform isoamylalcohol extraction and ethanol precipitation, and dissolved in H2O. The sequencing library was prepared as per Illumina protocol and sequenced on an Illumina HiSeq2500.
Chromatin prepared by MNase digestion was diluted 5x in NP-40 buffer (20 mM Tris pH 8.0, 5 mM EDTA, 150 mM NaCl, 1% NP-40, 1x protease inhibitors) without SDS to decrease SDS concentration to 0.1%. Chromatin was pre-cleared for 1 h at 4°C with Protein A/G agarose beads (Santa Cruz sc-2003) and incubated overnight at 4°C with the same anti-lamin A/C antibody as above (6 μg for 6 × 106 cells) or with anti-lamin B1 antibodies (Abcam ab16048; 3 μg for 6 × 106 cells). Immune complexes were collected using Protein A/G agarose beads for 2 h on a rotator at 4°C, washed 6 × 5 min on a rotator at 4°C as follows: once in 20 mM Tris pH 8.0, 5 mM EDTA, 150 mM NaCl, 1% NP-40, 0.1% SDS, protease inhibitors; twice in 20 mM Tris pH 8.0, 5 mM EDTA, 500 mM NaCl, 1% NP-40, 0.1% SDS, protease inhibitors; twice in 10 mM Tris pH 8.0, 1 mM EDTA, 250 mM LiCl, 1% NP-40, 1% deoxycholate, protease inhibitors; and once in TE buffer. Note that the NaCl- and LiCl-based washes used here are recommended in ChIP protocols using agarose beads. Crosslink was reversed and DNA was eluted and purified as above. Library preparation and sequencing were done as above.
Western blotting
Proteins were separated by SDS-PAGE and immunoblotted as describedCitation44 using antibodies to lamin A/C (Santa Cruz sc-7292) or lamin B1 (Abcam; ab16048) and horseradish peroxidase-conjugated secondary antibodies.
ChIP-seq data processing and identification of LADs
Sequence reads were aligned to the HG19 reference genome using Bowtie2 v2.1.0 with default parameters.Citation45 Duplicate reads were removed using Picard's MarkDuplicates program with parameter REMOVE_DUPLICATES set to true. To avoid any normalization bias, we ensured that each pair of aligned input and ChIP read files had the same read depth, by using Picard's DownsampleSam program (on the larger of the 2 files. We used version 1.86 of picard (http://picard.sourceforge.net/). LiDs were mapped using Enhanced Domain Detector (EDD v1.1), a broad peak calling algorithm we recently developed.Citation15 ChIP-seq data were analyzed using a genomic bin size of 10 kb (LMNA) or 6 kb (LMNB1) and a gap penalty of 4 for LMNA MNase-digested chromatin data, 7 for LMNA sonicated chromatin data, 12 for LMNB1 MNase-digested data, and 5 for AD04DF fibroblast data.
ChIP-qPCR
ChIP DNA (30 ng) was used as template for quantitative (q)PCR using primers to genic and intergenic regions (Supplementary Table S3). PCR was done using SYBR® Green (BioRad), with 95°C for 3 min and 40 cycles of 95°C for 30 sec, 60°C for 30 sec, and 72°C for 30 sec.
Data viewing
Browser views of gene tracks, ChIP-seq data and peaks are shown using Integrated Genomics Viewer (IGV; broadinstitute.org/igv).Citation46 Genes are from the Illumina iGenomes gene annotation with UCSC data source for HG19 (http://cufflinks.cbcb.umd.edu/igenomes.html).
Published data sets
RNA-seq data for HeLa cells were downloaded from NCBI GEO accession number GSM958735. Our LMNA ChIP-seq data from AD04DF fibroblasts were downloaded from NCBI GEO accession number GSE54334. hPTM ChIP-seq data for HeLa cells were downloaded from NCBI GEO accession number GSE29611.
Data access
LMNA ChIP-seq data for HeLa cells are available under GEO accession number GSE57149.
Disclosure of Potential Conflicts of Interest
No potential conflict of interest was declared
supplemental_material.zip
Download Zip (528.2 KB)Acknowledgments
We are grateful to Kristin Vekterud for expert technical assistance.
Funding
This work was supported by the Center National de la Recherche Scientifique, University Paris-Diderot Paris 7, and Association Française de lutte contre les Myopathies (BB, ID-G), and by the Research Council of Norway, South-East Health Norway (ARO), and the University of Oslo (PC, EGL).
Supplemental Material
Supplemental data for this article can be accessed on the publisher's website.
References
- Burke B, Stewart CL. The nuclear lamins: flexibility in function. Nat Rev Mol Cell Biol 2013; 14:13-24; PMID:23212477; http://dx.doi.org/10.1038/nrm3488
- Guelen L, Pagie L, Brasset E, Meuleman W, Faza MB, Talhout W, Eussen BH, de KA, Wessels L, de Laat W, et al. Domain organization of human chromosomes revealed by mapping of nuclear lamina interactions. Nature 2008; 453:948-951; PMID:18463634; http://dx.doi.org/10.1038/nature06947
- Peric-Hupkes D, Meuleman W, Pagie L, Bruggeman SW, Solovei I, Brugman W, Graf S, Flicek P, Kerkhoven RM, van Lohuizen M, et al. Molecular maps of the reorganization of genome-nuclear lamina interactions during differentiation. Mol Cell 2010; 38:603-613; PMID:20513434; http://dx.doi.org/10.1016/j.molcel.2010.03.016
- Meuleman W, Peric-Hupkes D, Kind J, Beaudry JB, Pagie L, Kellis M, Reinders M, Wessels L, van Steensel B. Constitutive nuclear lamina-genome interactions are highly conserved and associated with A/T-rich sequence. Genome Res 2013; 23:270-280; PMID:23124521; http://dx.doi.org/10.1101/gr.141028.112
- Kind J, Pagie L, Ortabozkoyun H, Boyle S, de Vries SS, Janssen H, Amendola M, Nolen LD, Bickmore WA, van Steensel B. Single-cell dynamics of genome-nuclear lamina interactions. Cell 2013; 153:178-192; PMID:23523135; http://dx.doi.org/10.1016/j.cell.2013.02.028
- Kind J, van Steensel B. Genome-nuclear lamina interactions and gene regulation. Curr Opin Cell Biol 2010; 22:320-325; PMID:20444586; http://dx.doi.org/10.1016/j.ceb.2010.04.002
- Zuleger N, Robson MI, Schirmer EC. The nuclear envelope as a chromatin organizer. Nucleus 2011; 2:339-349; PMID:21970986; http://dx.doi.org/10.4161/nucl.2.5.17846
- Collas P, Lund EG, Oldenburg AR. Closing the (nuclear) envelope on the genome: how nuclear lamins interact with promoters and modulate gene expression. BioEssays 2014; 36:75-83; PMID:24272858; http://dx.doi.org/10.1002/bies.201300138
- Reddy KL, Zullo JM, Bertolino E, Singh H. Transcriptional repression mediated by repositioning of genes to the nuclear lamina. Nature 2008; 452:243-247; PMID:18272965; http://dx.doi.org/10.1038/nature06727
- Mattout A, Pike BL, Towbin BD, Bank EM, Gonzalez-Sandoval A, Stadler MB, Meister P, Gruenbaum Y, Gasser SM. An EDMD mutation in C. elegans lamin blocks muscle-specific gene relocation and compromises muscle integrity. Curr Biol 2011; 21:1603-1614; PMID:21962710; http://dx.doi.org/10.1016/j.cub.2011.08.030
- Towbin BD, Gonzalez-Aguilera C, Sack R, Gaidatzis D, Kalck V, Meister P, Askjaer P, Gasser SM. Step-wise methylation of histone H3K9 positions heterochromatin at the nuclear periphery. Cell 2012; 150:934-947; PMID:22939621; http://dx.doi.org/10.1016/j.cell.2012.06.051
- Lund E, Oldenburg A, Delbarre E, Freberg C, Duband-Goulet I, Eskeland R, Buendia B, Collas P. Lamin A/C-promoter interactions specify chromatin state-dependent transcription outcomes. Genome Res 2013; 23:1580-1589; PMID:23861385; http://dx.doi.org/10.1101/gr.159400.113
- Sadaie M, Salama R, Carroll T, Tomimatsu K, Chandra T, Young AR, Narita M, Perez-Mancera PA, Bennett DC, Chong H, et al. Redistribution of the Lamin B1 genomic binding profile affects rearrangement of heterochromatic domains and SAHF formation during senescence. Genes Dev 2013; 27:1800-1808; PMID:23964094; http://dx.doi.org/10.1101/gad.217281.113
- Shah PP, Donahue G, Otte GL, Capell BC, Nelson DM, Cao K, Aggarwala V, Cruickshanks HA, Rai TS, McBryan T, et al. Lamin B1 depletion in senescent cells triggers large-scale changes in gene expression and the chromatin landscape. Genes Dev 2013; 27:1787-1799; PMID:23934658; http://dx.doi.org/10.1101/gad.223834.113
- Lund EG, Oldenburg AR, Collas P. Enriched Domain Detector: a program for detection of wide genomic enrichment domains robust against local variations. Nucleic Acids Res 2014; 42:e92; PMID:24782521; http://dx.doi.org/10.1093/nar/gku324
- Greil F, Moorman C, van Steensel B. DamID: mapping of in vivo protein-genome interactions using tethered DNA adenine methyltransferase. Methods Enzymol 2006; 410:342-359; PMID:16938559; http://dx.doi.org/10.1016/S0076-6879(06)10016-6
- Collas P. The state-of-the-art of chromatin immunoprecipitation. Methods Mol Biol 2009; 567:1-25; PMID:19588082; http://dx.doi.org/10.1007/978-1-60327-414-2_1
- Dahl JA, Collas P. A rapid micro chromatin immunoprecipitation assay (mChIP). Nat Protoc 2008; 3:1032-1045; PMID:18536650; http://dx.doi.org/10.1038/nprot.2008.68
- O'Neill LP, Turner BM. Histone H4 acetylation distinguishes coding regions of the human genome from heterochromatin in a differentiation-dependent but transcription- independent manner. EMBO J 1995; 14:3946-3957; PMID:7664735
- Kouskouti A, Talianidis I. Histone modifications defining active genes persist after transcriptional and mitotic inactivation. EMBO J 2005; 24:347-357; PMID:15616580; http://dx.doi.org/10.1038/sj.emboj.7600516
- Comelli L, Marchetti L, Arosio D, Riva S, Abdurashidova G, Beltram F, Falaschi A. The homeotic protein HOXC13 is a member of human DNA replication complexes. Cell Cycle 2009; 8:454-459; PMID:19182517; http://dx.doi.org/10.4161/cc.8.3.7649
- Hirano Y, Hizume K, Kimura H, Takeyasu K, Haraguchi T, Hiraoka Y. Lamin B receptor recognizes specific modifications of histone H4 in heterochromatin formation. J Biol Chem 2012; 287(51):42654-63; PMID:23100253; http://dx.doi.org/10.1074/jbc.M112.397950
- Wal M, Pugh BF. Genome-wide mapping of nucleosome positions in yeast using high-resolution MNase ChIP-Seq. Methods Enzymol 2012; 513:233-250; PMID:22929772; http://dx.doi.org/10.1016/B978-0-12-391938-0.00010-0
- McGhee JD, Felsenfeld G. Another potential artifact in the study of nucleosome phasing by chromatin digestion with micrococcal nuclease. Cell 1983; 32:1205-1215; PMID:6301684; http://dx.doi.org/10.1016/0092-8674(83)90303-3
- Cockell M, Rhodes D, Klug A. Location of the primary sites of micrococcal nuclease cleavage on the nucleosome core. J Mol Biol 1983; 170:423-446; PMID:6631965; http://dx.doi.org/10.1016/S0022-2836(83)80156-9
- Noll M, Kornberg RD. Action of micrococcal nuclease on chromatin and the location of histone H1. J Mol Biol 1977; 109:393-404; PMID:833849; http://dx.doi.org/10.1016/S0022-2836(77)80019-3
- Dechat T, Korbei B, Vaughan OA, Vlcek S, Hutchison CJ, Foisner R. Lamina-associated polypeptide 2alpha binds intranuclear A-type lamins. J Cell Sci 2000; 113:3473-3484; PMID:10984438
- Dechat T, Gesson K, Foisner R. Lamina-independent lamins in the nuclear interior serve important functions. Cold Spring Harb Symp Quant Biol 2010; 75:533-543; PMID:21209392; http://dx.doi.org/10.1101/sqb.2010.75.018
- Sarcinella E, Zuzarte PC, Lau PN, Draker R, Cheung P. Monoubiquitylation of H2A.Z distinguishes its association with euchromatin or facultative heterochromatin. Mol Cell Biol 2007; 27:6457-6468; PMID:17636032; http://dx.doi.org/10.1128/MCB.00241-07
- Weber CM, Henikoff JG, Henikoff S. H2A.Z nucleosomes enriched over active genes are homotypic. Nat Struct Mol Biol 2010; 17:1500-1507; PMID:21057526; http://dx.doi.org/10.1038/nsmb.1926
- Weber CM, Ramachandran S, Henikoff S. Nucleosomes Are Context-Specific, H2A.Z-Modulated Barriers to RNA Polymerase. Mol Cell 2014; 53:819-830; PMID:24606920; http://dx.doi.org/10.1016/j.molcel.2014.02.014
- Kolb T, Maass K, Hergt M, Aebi U, Herrmann H. Lamin A and lamin C form homodimers and coexist in higher complex forms both in the nucleoplasmic fraction and in the lamina of cultured human cells. Nucleus 2011; 2:425-433; PMID:22033280; http://dx.doi.org/10.4161/nucl.2.5.17765
- Kubben N, Voncken JW, Misteli T. Mapping of protein- and chromatin-interactions at the nuclear lamina. Nucleus 2010; 1:460-471; PMID:21327087; http://dx.doi.org/10.4161/nucl.1.6.13513
- Dechat T, Gotzmann J, Stockinger A, Harris CA, Talle MA, Siekierka JJ, Foisner R. Detergent-salt resistance of LAP2a in interphase nuclei and phosphorylation-dependent association with chromosomes early in nuclear assembly implies functions in nuclear structure dynamics. EMBO J 1998; 17:4887-4902; PMID:9707448; http://dx.doi.org/10.1093/emboj/17.16.4887
- Zhang S, Schones DE, Malicet C, Rochman M, Zhou M, Foisner R, Bustin M. High Mobility Group Protein N5 (HMGN5) and Lamina Associated Polypeptide 2 alpha (LAP2alpha) Interact and Reciprocally Affect Their Genome-wide Chromatin Organization. J Biol Chem 2013; 288:18104-18109; PMID:23673662; http://dx.doi.org/10.1074/jbc.C113.469544
- Fan JY, Gordon F, Luger K, Hansen JC, Tremethick DJ. The essential histone variant H2A.Z regulates the equilibrium between different chromatin conformational states. Nat Struct Biol 2002; 9:172-176; PMID:11850638; http://dx.doi.org/10.1038/nsb0402-316b
- Bonisch C, Hake SB. Histone H2A variants in nucleosomes and chromatin: more or less stable? Nucleic Acids Res 2012; 40:10719-10741; PMID:23002134; http://dx.doi.org/10.1093/nar/gks865
- Weber CM, Henikoff S. Histone variants: dynamic punctuation in transcription. Genes Dev 2014; 28:672-682; PMID:24696452; http://dx.doi.org/10.1101/gad.238873.114
- Nekrasov M, Amrichova J, Parker BJ, Soboleva TA, Jack C, Williams R, Huttley GA, Tremethick DJ. Histone H2A.Z inheritance during the cell cycle and its impact on promoter organization and dynamics. Nat Struct Mol Biol 2012; 19:1076-1083; PMID:23085713; http://dx.doi.org/10.1038/nsmb.2424
- Santisteban MS, Hang M, Smith MM. Histone variant H2A.Z and RNA polymerase II transcription elongation. Mol Cell Biol 2011; 31:1848-1860; PMID:21357739; http://dx.doi.org/10.1128/MCB.01346-10
- Creyghton MP, Markoulaki S, Levine SS, Hanna J, Lodato MA, Sha K, Young RA, Jaenisch R, Boyer LA. H2AZ is enriched at polycomb complex target genes in ES cells and is necessary for lineage commitment. Cell 2008; 135:649-661; PMID:18992931; http://dx.doi.org/10.1016/j.cell.2008.09.056
- Illingworth RS, Botting CH, Grimes GR, Bickmore WA, Eskeland R. PRC1 and PRC2 are not required for targeting of H2A.Z to developmental genes in embryonic stem cells. PLoS One 2012; 7:e34848; PMID:22496869
- Wu R, Terry AV, Singh PB, Gilbert DM. Differential subnuclear localization and replication timing of histone H3 lysine 9 methylation states. Mol Biol Cell 2005; 16:2872-2881; PMID:15788566; http://dx.doi.org/10.1091/mbc.E04-11-0997
- Duband-Goulet I, Woerner S, Gasparini S, Attanda W, Konde E, Tellier-Lebegue C, Craescu CT, Gombault A, Roussel P, Vadrot N, et al. Subcellular localization of SREBP1 depends on its interaction with the C-terminal region of wild-type and disease related A-type lamins. Exp Cell Res 2011; 317:2800-2813; PMID:21993218; http://dx.doi.org/10.1016/j.yexcr.2011.09.012
- Langmead B, Trapnell C, Pop M, Salzberg SL. Ultrafast and memory-efficient alignment of short DNA sequences to the human genome. Genome Biol 2009; 10:R25; PMID:19261174; http://dx.doi.org/10.1186/gb-2009-10-3-r25
- Robinson JT, Thorvaldsdottir H, Winckler W, Guttman M, Lander ES, Getz G, Mesirov JP. Integrative genomics viewer. Nat Biotechnol 2011; 29:24-26; PMID:21221095; http://dx.doi.org/10.1038/nbt.1754