Abstract
The use of biomolecules as coatings on biomaterials is recognized to constitute a promising approach to modulate the biological response of the host. In this work, we propose a coating composed by 2 biomolecules susceptible to provide complementary properties for cardiovascular applications: fibronectin (FN) to enhance endothelialization, and phosphorylcholine (PRC) for its non thrombogenic properties. Polytetrafluoroethylene (PTFE) was selected as model substrate mainly because it is largely used in cardiovascular applications. Two approaches were investigated: 1) a sequential adsorption of the 2 biomolecules and 2) an adsorption of the protein followed by the grafting of phosphorylcholine via chemical activation. All coatings were characterized by immunofluorescence staining, X-Ray Photoelectron Spectroscopy and Scanning Electron Microscopy analyses. Assays with endothelial cells showed improvement on cell adhesion, spreading and metabolic activity on FN-PRC coatings compared with the uncoated PTFE. Platelets adhesion and activation were both reduced on the coated surfaces when compared with uncoated PTFE. Moreover, clotting time tests exhibited better hemocompatibility properties of the surfaces after a sequential adsorption of FN and PRC. In conclusion, FN-PRC coating improves cell adhesion and non-thrombogenic properties, thus revealing a certain potential for the development of this combined deposition strategy in cardiovascular applications.
Introduction
When biomaterials as stents are implanted into humans, the interaction of their surfaces with the living system plays a crucial role in regard to the clinical performances and the medical success. Unhappily, a number of clinical complications - as early and late restenosis - frequently occur and lead to critical physiopathological scenarios. Recent studies show that early restenosis develops in 35–45% of the cases after 3–6 months of implantation while late restenosis appears in 10–12% of the cases after 5 y of implantation.Citation1,2 Although antiplatelet therapies are frequently applied,Citation3,4 they do not succeed ideally against some major clinical complications. Hence, efforts are made to modulate the surface/interface interaction of stents and to minimize the antiplatelet treatments. Different approaches are explored, including coating the biomaterial surface with biologically active molecules. The bioactive molecules involved at the interface are expected 1) to provide hemocompatibility (avoiding thrombus formation during the first weeks after implantation) and 2) to prevent late restenosis (mainly enhancing endothelial cell adhesion and proliferation to modulate the formation of a natural non-thrombogenic surface). Different strategies have been explored over the last decades to improve the performance of stents by modifying their surface. summarizes these studies. Despite the large number of works carried out and the numerous efforts deployed to develop suitable strategies, rare are the works that were successfully translated into clinics.
Table 1. Strategies explored to modify stents surface and improve their biocompatiblity
Late thrombosis with adverse clinical outcomes occurs when stent endothelialization is delayed or absent.Citation5 Therefore the necessity of improving the endothelialization process and avoiding thrombus formation while this process is completed appears as a key strategy. Despite the number of works carried out, as well as the efforts deployed to develop suitable strategies, rare are the works that were successfully translated into clinics. Biological processes are complex and synergistic, and few studies are devoted to explore this synergy through the development of combined strategies. Related to hemocompatibility properties, most of the studies performed some tests of isolated events of the coagulation cascade (as platelet adhesion and activation). Tests involving complete blood as thromboelastography or clotting time could provide a better understanding of the interaction of the biomaterial with the biological fluid.
In order to provide hemocompatibility properties from the time of the first contact of the biomaterial with blood, and at the same time to promote endothelialization process, we proposed the preparation of coatings with 2 biomolecules that have already been studied separately for cardiovascular applications: fibronectin and phosphorylcholine. To the best of our knowledge these molecules have never been studied together to synergize their properties on a single coating. Fibronectin (FN) is a large glycoprotein of the extracellular matrix, found in solution in corporal fluids and immobilized in various tissues. It is composed of 2 subunits, each of an approximate molecular weight of 250 kDa, linked together by disulfide bonds. Fibronectin is known to promote cell attachment and spreading, and in biomedical applications, biomaterial-tissue integration.Citation6-13 Besides, the structure and stability of the human plasma fibronectin are well known.Citation10-14 Indeed, the adsorption and grafting of this protein have been already studied on model surfaces,Citation15–18 showing the feasibility of having a stable coating for biomaterials. For these reasons, fibronectin was proposed here as a support to enhance endothelialization process. However, some other studies have concluded that plasma fibronectin interacts with platelets in important ways to form arterial thrombi,Citation17 which could be a disadvantage for cardiovascular devices. Therefore, we propose to combine fibronectin with a key protagonist known for its non thrombogenic properties: phosphorylcholine. This molecule is an electrically neutral, zwitterionic head group that represents the bulk of the phospholipid head groups present on the external surface of blood cells. It is inert in coagulation assays, decreases platelet adhesion and delays the thrombus formation.Citation19,20
Coatings with these 2 biomolecules are therefore expected to lead to a twofold action: on one hand, limit thrombus formation in the short term and on the other hand, stimulate the endothelialization of the biomaterial surface to provide long term stability. Moreover, the original approach of this work is also to use a fluorocarbon nanocoating, called CFx, as interlayer between the biomolecules and the stainless substrate from stent. Indeed, the CFx coating deposited by plasma has already exhibited good adhesion and cohesion under deformation, such as the one induced during stent implantation procedure,Citation21,22 and in pseudo-physiological medium.Citation23 Since CFx coatings implies a multi-step process to be prepared and has a chemical structure close to polytetrafluoroethylene (PTFE), this last polymer was thus used as surface model in this work. Furthermore, in order to optimize the biological effect expected, 2 different strategies were explored for the coating process of fibronectin and phosphorylcholine adsorption versus grafting. The coating created by the sequential adsorption of the 2 biomolecules is called AA (for Adsorption-Adsorption) and the one including the adsorption of FN followed by the grafting of PRC is called AG (for Adsorption-Grafting), as summarized in .
Results and Discussion
Surfaces characterization by XPS, SEM and immunostaining
X-Ray Photoelectron Spectroscopy: The chemical compositions of all surfaces were assessed by XPS and the results are presented in . The presence of the FN coating was confirmed by the augmentation of carbon concentration and the decrease of the fluorine one, combined with the detection of oxygen and nitrogen, elements that are not present in PTFE substrate. Furthermore, after the adsorption or grafting of PRC, the detection of phosphorus clearly evidenced the presence of this molecule. However, high variations in F, C, N and O concentrations were noticed after the addition of PRC (). This could be explained by FN rearrangement or desorption that could lead to uncoated or less coated zones meaning more fluorine detected by XPS (analysis depth 5 nm). It is also observed that chemical composition was very similar on coatings containing FN/PRC no matter the process applied to prepare both surfaces (AA or AG). This fact implies that whether if PRC is activated or not, desorption and/or rearrangement of the protein occurred. Moreover, the adsorption process of PRC on FN coating seemed to derive in non-covalent interaction that are strong enough to lead to approximately the same concentration of PRC at the surface than in the coating where covalent bonding was induced.
Table 2. Chemical composition of the different surfaces.
Thus, XPS results clearly demonstrated that FN and PRC coatings were efficient. However, PRC either adsorbed or grafted, apparently led to chemical reorganization as evidenced by the high variation on chemical composition when compared to FN coating, which was further investigated by confocal and electron microscopy.
Scanning Electron Microscopy: Surfaces were therefore analyzed under SEM. No difference was detected between FN and AA coatings through this technique. Indeed, both coatings appeared homogeneous. However, in the case of the grafting process AG, complexes distributed all over the surface were detected which were not observed on the other conditions. This fact revealed that the grafting process induced reactions that led to the formation of macromolecular complexes (). Different controls were tested: PTFE in interaction with N-(3-dimethylaminopropyl)-N′-ethylcarbodiimide hydrochloride (EDC) and imidazole, FN coating in interaction with EDC and imidazole, PRC in interaction with EDC and imidazole (data not shown). As no complexes were observed on controls, it was confirmed that they were formed as result of the interaction of the 2 molcules only when PRC was chemically activated to induce covalent bonds. PRC reaction by EDC and imidazole led to the activation of (P=O)OH terminal group in order to react with amino moieties of FN. However, this process could also activate carboxylic groups ((C=O)OH) which are largely present in FN molecule. Thus, even if our controls showed that no complex is formed without PRC, it is possible that the presence of PRC, EDC and imidazole molecules stimulates an interaction between FN molecules themself that provokes the complex formation. Conversely, according to the expected reaction between PRC and FN, several molecules of PRC can also react with one FN, since the protein has several amine groups available for the reaction. The formation of complexes could be related to the interaction of a number of PRC molecules with FN.
Figure 2. SEM images for the different coatings. Two different magnifications are shown for each surface. PTFE) Uncoated PTFE; FN) Fibronectin adsorption; AA) Fibronectin and phosphorylcholine adsorption; AG) Fibronectin adsorption-phosphorylcholine grafting.
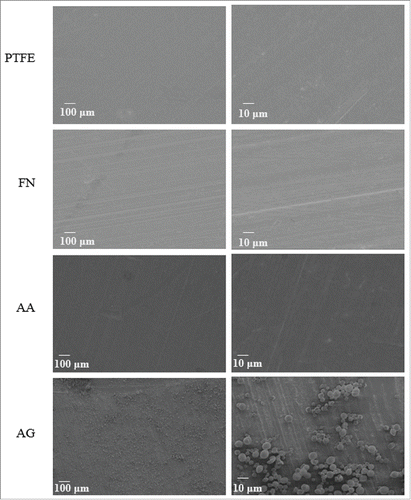
The formation of FN/PRC complexes, as seen in SEM images, permit to explain the high variation observed in XPS analyses for AG condition. Indeed, keeping in mind that the surface area analyzed in XPS is 0.5 mm2, such complexes led to areas rich in FN/PRC whereas some others became less coated. As this particular interaction between the 2 molcules and FN itself could have consequences on the protein conformation, and in order to have a better understanding of this behavior, FN distribution as well as conformation have been studied by immunofluorescence staining.
Immunofluorescence staining: Representative images of the staining of FN are observed on . No staining was observed in absence of FN neither on uncoated PTFE, PTFE treated with PRC or PTFE treated with EDC and imidazole solution (data not shown). The immunostaining of FN coating with the polyclonal antibody evidenced the presence of the protein, as fluorescence was observed all over the surfaces containing FN (, left). On the other hand, a specific monoclonal antibody was also used to assess the availability of the FN cell-binding domain which is responsible of cell adhesion (, right). After observation under confocal microscopy, surfaces showed that the FN cell binding domain at the surface remained available. In AA condition, no apparent difference was observed when compared with FN coating either on the polyclonal and monoclonal antibody staining. However, in the case of the grafting process, the formation of complexes was clearly observed in fluorescence. Furthermore, in some areas no complex was noticed and a decrease in fluorescence intensity was observed. In AA condition, it seems that the PRC adsorption does not affect the FN distribution nor the protein conformation. Nevertheless, this observation may appear in contradiction with XPS results which evidenced higher variations on chemical composition when adding PRC, meaning that either the fluorocarbon substrate was less covered, either FN coating was not homogeneous. Such apparent discrepancy can be explained by the non-pertinence of the fluorescence technique to address precisely the spatial molecular distribution patterning at the surfaces. Indeed, the signal of the fluorescence of the labels grafted on the molecular species diffuses largely around the atomic molecular structure. Consequently, a simple direct exploration of the whole fluorescent signal observed can lead to an overestimation of the portion of the surface truly physically coated. Therefore a surface saturated with a homogeneous coating appears homogeneously fluorescent, but even a non-homogeneous coating -in the limits where the lateral gap between the molecules is not too important and undercover by the fluorescence diffusion- may appear homogeneous if we consider only the fluorescent signal. In AG condition, since no complex was observed when FN or PRC with and without EDC/imidazole are alone, it could be confirmed, that the detected complexes are thus associated to the strong interaction of these 2 molecules, leading to the formation of macromolecular complexes. As well as SEM images, these results could also justify the variation observed on XPS analyses.
Figure 3. Immunostaining of FN. Polyclonal and monoclonal antibodies were used to characterize the presence of the protein (left) and specifically the availability of its cell binding domain (right). PTFE) Uncoated PTFE; FN) Fibronectin adsorption; AA) Fibronectin and phosphorylcholine adsorption; AG) Fibronectin adsorption-phosphorylcholine grafting.
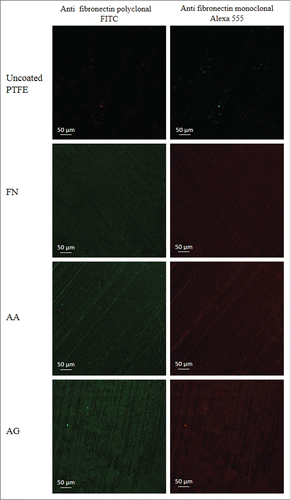
In order to assess more information on the FN/PRC interaction, other techniques such as AFM, contact angle as well as angle-resolved XPS analyses have been tested. Nevertheless, mainly due to the high roughness of PTFE film, these techniques did not seem to be appropriate for ensuring accurate and reproducible results. In the present work, after surface characterization of the coatings on PTFE films, biological performances were studied through endothelial cells and hemocompatibility tests.
Cell response
As the main objective of using FN coatings is to improve the endothelialization process, the first biological tests performed were focused on the interactions of the surfaces with endothelial cells. These interactions were evaluated through cell adhesion, spreading and metabolic activity tests. On one hand, the adhesion and spreading of cells were studied through immunostaining of nuclei and actin filaments after 1 h of adhesion and on the other hand, resazurin tests were performed to measure cell metabolic activity after 24 h. The quantities of adhered cells evaluated from immunostaining images, are summarized in . The adhesion of endothelial cells was significantly improved on surfaces containing FN when compared with uncoated PTFE. However, even if a slight diminution on cell adhesion was noticed on AG surfaces, high variability was observed and no significant difference was detected between AA, AG and FN coatings. This high variability could be justified by the variation on chemical composition and distribution of FN/PRC complexes observed during AG surface characterizations.
Figure 4. Adhesion of endothelial cells after 1 h. Cells counting after immunostaining. Significant difference was found (*) between coated PTFE vs. the uncoated one. PTFE) Uncoated PTFE; FN) Fibronectin adsorption; AA) Fibronectin and phosphorylcholine adsorption; AG) Fibronectin adsorption-phosphorylcholine grafting.
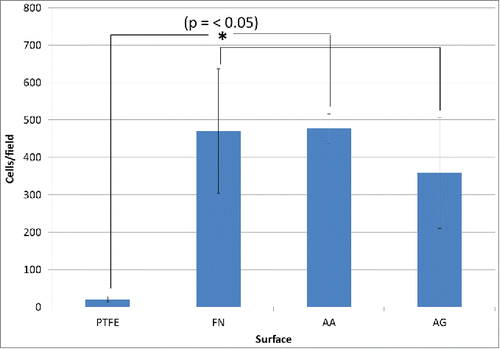
In order to study the cell spreading, F-actin was stained and hence, it was possible to observe their cytoskeleton. Firstly, it was observed a more organized cytoskeleton on FN containing surfaces whereas on uncoated PTFE, the cytoskeleton was not prominent (). Furthermore, although the quantity of adherent cells after 1 h appeared similar in the 3 different coatings, this was not the case for their morphology. Interestingly, cells appeared as widely spread on AA condition as on FN one. In contrast, on AG condition cells were poorly spread and F-actin was concentrated along the plasma membrane. These results confirmed that PRC adsorption on FN coating has no effect on the interaction of the surface with endothelial cells as their spreading was not compromised and the quantity of adherent cells was very similar to the FN surface one. However PRC grafting process seemed to have a negative effect on cells behavior as both a diminution on adherent cells and a lack of spreading were detected. This lack of spreading could be associated to non-viable cells; hence, further studies were performed through resazurin tests in order to measure cell metabolic activity after 24 h. In this study, higher cell viability was observed on FN and AA surfaces with significant difference when compared with AG and PTFE ones. Nevertheless, no significant difference was detected between AG and PTFE nor between AA and FN surfaces (). The notable improvement on cell adhesion and metabolic activity in our AA coating could be consequence of the interaction of these 2 molcules with the surface and cells. This interaction must be different when PRC is grafted, e.g. changes in conformation and rearrangement of the protein could happen. Indeed, the decrease in cell metabolic activity on AG when compared to AA and FN surfaces is coherent to the lack of spreading observed in adherent cells after 1 h, which could be related to a desorption, rearrangement or changes in conformation of fibronectin as suggested previously on coating characterizations. These changes in FN coating could even lead to PTFE uncoated zones or a non-homogeneous distribution of FN. Indeed, XPS results have shown high variation on FN/PRC coating composition, while immunostaining and SEM images have clearly evidenced a rearrangement of FN coating with PTFE uncoated areas and non-homogeneous distribution of FN/PRC complexes all over the surface. All these variations evidenced by the surface characterizations could justify the variability observed on cell adhesion. Moreover, the long-term interaction of the coatings with cells as well as their retention under flow must be considered for further experiments. In this work, after performing cell tests, and even if no further difference has been detected when PRC is adsorbed, an effect on blood response was expected because of the non-thrombogenic properties of this molecule, hence hemocompatibility tests were performed.
Figure 5. Spreading of endothelial cells after 1 h of adhesion. PTFE) Uncoated PTFE; FN) Fibronectin adsorption; AA) Fibronectin and phosphorylcholine adsorption; AG) Fibronectin adsorption-phosphorylcholine grafting.
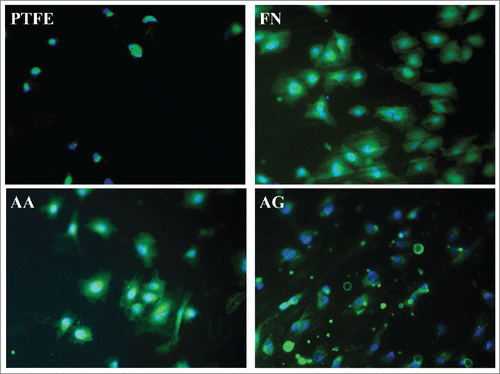
Figure 6. Endothelial cells metabolic activity after 24 h through resazurin test. Significant difference (*) was found between the pairs FN-AG, FN-PTFE and PTFE-AA. No significant difference was determined between the pairs FN-AA and AA-AG. PTFE) Uncoated PTFE; FN) Fibronectin adsorption; AA) Fibronectin and phosphorylcholine adsorption; AG) Fibronectin adsorption-phosphorylcholine grafting.
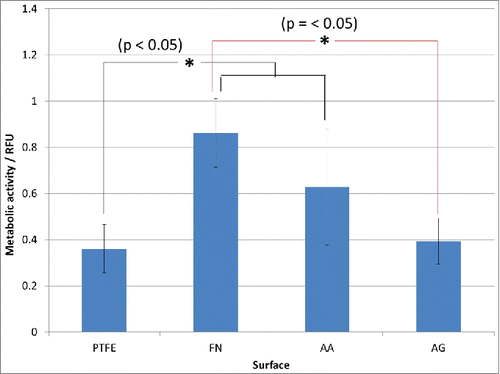
Blood response
The main goal of studying the use of phosphorylcholine onto biomaterials is to improve blood compatibility, by preventing or minimizing the adverse effects induced by the coagulation cascade. As platelets are involved in this process, their adhesion and aggregation on the different surfaces were first investigated. These experiments were followed by evaluating the clot formation when complete blood was in contact with the surfaces.
Platelet adhesion and activation: In order to evaluate the interaction of the different surfaces with platelets, SEM pictures of the surfaces in contact with platelets during 2 h were taken. These pictures allowed the evaluation of the adhesion, activation and aggregation of platelets qualitatively. Indeed, platelet shape changes - depending on the activation stage - from spherical to spheroid then to small bulbous protrusions and at last to pseudopodia network. In the case of uncoated PTFE, zones with activated platelets were observed, linked together through a pseudopodia network (). For FN coating, platelets displayed more the first stage of activation, long spheroids with some pseudopods and some networks. Whereas after phosphorylcholine adsorption, the adherent platelets exhibit spherical and smooth discoid shapes meaning that they are neither activated nor aggregated. In AG condition, platelets displayed activation with change on form; the apparition of pseudopods and some aggregates were also observed. Therefore, SEM analyses showed that platelets are more isolated and less aggregated on the coated surfaces compared with uncoated PTFE. PRC containing coatings increased hemocompatibility properties with less activation of platelets.Citation20,24,25 Indeed Chevallier et al. have shown that PRC grafted on PTFE surfaces improves hemocompatibility properties. Our results show that even in the presence of FN, the PRC coating preserves these properties. The AA condition had a higher impact on decreasing platelet activation and aggregation compared with the AG one. This fact could be related to a better availability of PRC in AA condition, thus supposing that in this condition, PRC molecules is organized at the surface, preserving therefore its non-thrombogenic properties. On the opposite, on AG condition, PRC/FN complexes are formed which should lead to a different organization of both PRC and FN. This different organization might provoke a decrease in the PRC benefit effect. Therefore, in order to obtain further information related to the thrombogenic properties of the surfaces, the kinetic of thrombus formation was studied.
Figure 7. SEM images for platelets adhesion on the different coatings after 2 h of contact. Two different magnifications are shown for each surface. PTFE) Uncoated PTFE; FN) Fibronectin adsorption; AA) Fibronectin and phosphorylcholine adsorption; AG) Fibronectin adsorption-phosphorylcholine grafting.
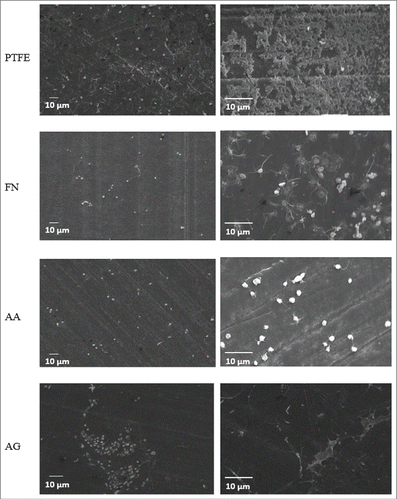
Clotting time analysis: Thrombus formation was followed after 20, 40, 60 and 80 minutes of contact of whole blood with the different surfaces (). At 20 minutes, no significant difference between surfaces was noted and the thrombus barely started to form. However, after 40 minutes, the thrombus formation was significantly different on AA condition compared with the other conditions. Despite no significant difference was detected after 60 minutes, AA still presented a higher concentration of free hemoglobin in the solution which was also persistent after 80 minutes. These results are in accordance with previous results with platelet adhesion. Indeed, in AA condition platelets were isolated and not activated compared with the other surfaces, meaning that the coagulation cascade should be delayed. On the other hand, the differences observed at 60 and 80 min are not as important as at 40 min, hence, the effect of PRC when adsorbed on FN was evidenced at short time, but at longer time it appears that its contribution is less significant compared with FN alone. PRC, at least, delayed the platelets activation and had therefore an effect on the thrombus formation. However, due to the presence of the FN, the zwitterionic nature of the lipid polar head group (PRC) that normally minimizes electrostatic interactions with charge bearing molecules could be reduced at long-term due to blood protein adsorption for example. It must be taken into account that it has been reported that FN plays also a role in the cascade coagulation,Citation17 so it is not surprising having best thrombogenic properties when PRC is present than in FN coatings. It should also be emphasized that AG sample did not seem to keep the hemocompatibility properties of the PRC molecule, which is in accordance with the previous tests with platelets. In addition, the effect of PRC on thrombogenic properties implies an organization of the molecule at the surface that can mimic the lipids organization on cell membranes. Moreover the non-thrombogenic properties of PRC may perhaps be intrinsically related to the molecule organization at the top layer of the surface. In AG condition, PRC could be entrapped in FN network avoiding an organization that permits the molecule to preserve its biological activity. Further experiments are required to better study the distribution and organization of PRC and FN in the prepared coatings, which could lead not only to the finding of a more efficient non-thrombogenic surface but also to the elucidation of the mechanism of action of PRC to delay the coagulation cascade.
Materials and Methods
Preparation of PTFE substrates
Polytetrafluoroethylene (Goodfellow, FP301350) substrate of 250 μm thickness was used. They were cut using a puncher into samples of 10 mm of diameter for surface characterization, endothelial cells and platelet tests. Samples of 15 mm of diameter were punched for clotting time test. Films were washed successively with acetone, deionized water and methanol for 10 min each in an ultrasonic bath and air-dried between each step.
Fibronectin purification and preparation
Fibronectin was purified from human plasma according to a well-established protocol based on a 3-step affinity chromatography.Citation26 This purification process has been shown to provide a protein solution exhibiting a purity of about 98% (w/w). The purified FN was filtered through a 0.2 μm filter and stored until use at ±8 ºC in 10 mM tris(hydroxymethyl) aminomethane (Tris) buffer, pH 7.4.
Coatings preparation
Adsorption process: PTFE samples were placed in sterile plates (48-well for samples of 10 mm of diameter and 24-well for samples of 15 mm of diameter). Samples were immersed in FN solution (50 μg/ml, the equivalent to a molarity of order 10−7 M) at 37 °C for 30 minutes. These parameters were defined after previous studies of our group.Citation15 Afterwards they were washed 3 times with buffer solution (Tris 10 mM pH 7.4). Subsequently, samples were immersed in 0.1 M solution of PRC (Sigma Aldrich, P0378) at 37 °C for 30 minutes, followed by 3 washes with the same buffer solution. Surfaces coated under these conditions are called “AA” (for Adsorption-Adsorption).
Grafting process: Hermanson proposes a reaction in which a phosphate-containing compound (which will be PRC in our case) reacts with EDC and immediately after, with imidazole to create a phosphorimidazole intermediate which will further react with an amine-containing compound (FN in our case) to create a phosphoramidate linkage.Citation27 In order to perform this procedure a 0.1 M PRC and 0.1 M N-(3-Dimethylaminopropyl)-N′-ethylcarbodiimide hydrochloride (EDC, Sigma Aldrich, E6383) solution on TRIS buffer (pH 7.4) was prepared and agitated during 5 minutes. Then, imidazole (Sigma Aldrich, I0250) was added in excess and after 30 minutes of agitation, the solution of activated PRC, containing the phosphorimidazole intermediate, was deposited on a previously coated FN on PTFE at 37 °C and let reacted with FN for 30 minutes in order to create a phosphoramidate linkage with primary amine groups of FN. Surfaces were washed 3 times with buffer solution. Surfaces coated under this condition are called “AG” (for Adsorption-Grafting).
Coatings characterization
Surface chemical characterization by XPS: The surface chemical composition of coated and uncoated substrates was assessed by XPS (depth analysis of 5 nm). The analyses were carried out using an X-Ray Photoelectron Spectrometer (XPS-PHI 5600-ci Spectrometer-Physical Electronics, Eden Prairie, MN, USA). Survey spectra were acquired using the Kα line of standard aluminum (Kα = 1486.6 eV) at 200 W with charge compensation while detecting the emitted photoelectrons at 45º with respect to the surface normal. The surface area analyzed was 0.005 cm2.
Scanning Electron Microscopy: Scanning Electron Microscopy (SEM) of surfaces and platelets test were assessed using a Leica s430i (Nanterre, France), Secondary Electrons Detector, current sensor 150 pA, high voltage 15 kV. Dry surfaces were glued on a stub and sputtered with gold.
Conformation of fibronectin by immunofluorescence staining: The presence of FN on PTFE substrates as well as the protein conformation exploring the accessibility of its cell binding domain were characterized by immunofluorescence staining. Samples were immersed in PBS-BSA saturation buffer 0.5% (w/v) for 1 hat room temperature. After saturation, samples were incubated for 45 minutes at room temperature with a solution of 2 primary antibodies: 1) rabbit anti-human plasma fibronectin polyclonal antibody (Sigma Aldrich, F-3648), to study the presence of the protein at the surface and its homogeneity and 2) anti-fibronectin, cell binding domain monoclonal antibody (Merk Millipore, MAB1926) diluted (1:500) to study the conformation of the protein through the availability of the cell binding domain at the different surfaces. Both antibodies were diluted in the saturation buffer. Samples were rinsed 3 times with the same saturation buffer and incubated again for 45 minutes at room temperature with 2 secondary antibodies: 1) fluorescein isothiocyanate (FITC)-conjugated goat anti-rabbit IgG (Sigma Aldrich, F-0382) for polyclonal antibody and 2) Alexa 555 (Invitrogen, A21424) for monoclonal antibody, both diluted in the saturation buffer (1:500). Samples were washed 3 times with the saturation solution and the fluorescence images were recorded with a confocal microscope LSM710 Carl Zeiss Microscopy (Marly le Roi, France) LSM, 710 Laser Argon ray 488 nm for FITC and Diode DPSS 561 nm for Alexa 555.
Biological performances
Adhesion and spreading of endothelial cells.
HUVEC were isolated from an umbilical cordon kindly provided by the Hospital of Saint François d’Assise with the previous consent of donor mothers. The vein was rinsed with PBS, filled with trypsin 10x and incubated 15 minutes at 37 ºC. Afterwards, PBS was added to inhibit trypsin effect and the solution was recuperated and centrifuged at 1000 rpm during 5 min. The supernatant was removed and cells were cultured in a flask (previously coated with gelatin) with M199 (Thermo Scientific, SH30253.01) culture media containing 10% of fetal bovine serum (Fisher Thermo Scientific, SH30396.03) and 1% of Penicillin-Streptomycin (Gibco, 15140–122). Culture media was changed after 24 h and then each 48 h until confluence. Cells were characterized by immunostaining and third to eighth generation were used to evaluate the interaction with the coated surfaces.
Adhesion cells test
Cells were removed from the culture plates by rinsing in PBS and incubating in a trypsin solution. After trypsin inactivation with complete medium the cells were centrifuged for 5 min at 1000 rpm. The resulting pellet was resuspended in complete medium. 15000 cells in M199 culture media containing serum and penicillin-streptomycin were deposited on PTFE surfaces previously treated and incubated at 37 °C. After this step, 2 different procedures were performed in order to study the cells adhesion and metabolic activity:
Immunostaining: After one hour of incubation, non-adherent cells were removed by washing 3 times with serum-free medium. Cells were fixed for 15 minutes with glutaraldehyde 1% (Sigma Aldrich, G5882) followed by 3 washes with deionized water and permeabilized with a triton (Sigma Aldrich, X100) solution 1% in PBS for 15 min. Samples were then saturated with casein 1% in PBS during 30 min, washed 3 times with PBS and immersed in a solution of DAPI (Sigma Aldrich, 32670) (1:3000) and phalloidin TRITC labeled (Sigma Aldrich, P5282) (1:300) in PBS during 45 min at room temperature. Cells were rinsed 3 times with PBS and images were recorded under a fluorescence microscope Olympus BX51 (Olympus America Corp., Tokyo, Japan) using 10x and 20x objectives. Three representative images were taken per sample and cells were counted using Image J software. Cell spreading was also studied through this technique.
Resazurin test: After adhesion for 24 h and rinsing with culture medium, cells were rinsed once again but with PBS in order to eliminate all the rests of culture medium. 300 μl of solution of resazurin on culture medium (1:10) was then added to the samples and let react during 4 h. 150 μl were taken from each sample and fluorescence was measured at 570 nm using a spectrophotometer ELISA reader (BioRad mod.450, Mississauga, Ontario, Canada).
Hemocompatibility tests
Blood of 6 healthy donors were used to perform the hemocompatibility tests.
Platelets adhesion, activation and aggregation
Platelets rich plasma was obtained by centrifugation of peripheral human blood at 1000 rpm for 10 min. Platelets were purified by hypotonic lysis using Thrombotic pure plus - Test kit for PLT (Fisher Scientific, BTP-4015) and adjusted to a concentration of 3 x 108 platelets / ml. Samples were immersed on platelets solution for 2 h at 37 °C. Samples were rinsed 3 times with deionized water and fixed during 30 min in a 2% isotonic glutaraldehyde solution, rinsed again with deionized water 3 times and dehydrated by immersion in solutions of increasing ethanol concentration (20, 50, 95 and 100%), followed by drying in hexamethyl disilazane. The experiment was performed in triplicate with blood from different donor.
Clotting time
In order to study the coagulation time when blood is in contact with the different surfaces, hemoglobin free methodology was used. The methodology was slightly modified from previous works in our laboratoryCitation28 (a kinetic of the reaction was performed to choose the most representative times for our surfaces as well as the volume of water to be added after interaction with blood). 100 μl of citrated blood were dropped onto the surfaces and 20 μl of CaCl2 (Sigma Aldrich, 2114) were added immediately. Samples were incubated at 37 ºC and 20, 40, 60 and 80 min were chosen as the most representative for our study. After these times of incubation, 2 ml of distilled water were added to each surface. Red blood cells not entrapped in a thrombus were hemolyzed and free hemoglobin molecules in the water were colorimetrically measured by monitoring the absorbance at 540 nm using a spectrophotometer ELISA reader (BioRad mod.450, Mississauga, Ontario, Canada). The test was performed in triplicate with 6 samples per condition using a different donor for each triplicate.
Statistical analysis
For XPS analyses, the data were represented as the mean +/− standard deviation of 9 measurements, corresponding to 3 points on 3 different samples. For adhesion tests (both immunofluorescence and resazurin) and for clotting time tests, the data were represented as the mean +/− standard deviation of 9 measurements, corresponding to 3 experiments repeated 3 times. Significant differences were determined running a one-way ANOVA followed by Tukey's post-hoc method to test all possible pairwise comparisons and determine where the differences lied. A value of p-value < 0.05 was considered significant (*).
Conclusions
In this work, 2 different coatings based on phosphorylcholine and fibronectin for PTFE substrates have been investigated. Physicochemical characterizations and biochemical analyses confirmed the presence of both biomolecules at the surface and evidenced different interactions of the molecules depending on the preparation process, i.e. sequential adsorption and grafting. Subsequent adsorption of FN and PRC resulted in a homogeneous coating for both biomolecules while the grafting process of PRC exhibited the formation of macromolecular complexes. Sequential adsorption of the biomolecules exhibited the best interaction with both endothelial cells and blood. Regardless of the fundamental tests performed compared with the complex biological system, the results are promising as a potential material to delay the coagulation cascade in the first minutes of interaction biomaterial-blood and at the same time to enhance the endothelialization process which is expected to create a non-thrombogenic surface stable in longer periods. However, to validate the applicability of this process and to be able to propose in fine a new generation of bioactive and biofunctionalized stents further experiments are required. Preliminary results made on fluorocarbon nanofilms deposited by plasma on flat stainless substrate have suggested the FN-PRC coatings feasibility on this metallic substrate. Complementary tests are required to elucidate their stability for blood-contact applications; particular attention should be paid to the consequences generated through the material geometrical modifications that occur during stents expansion and the constraint due to the flow pressure of the circulating blood, especially in regard to the stability and adhesion of the biocoating, which remains the main challenges for bioactive stent applications.
Disclosure of Potential Conflicts of Interest
No potential conflicts of interest were disclosed.
Acknowledgment
The authors would like to express their gratitude for their collaboration on the performance of experiments and/or their technical assistance to Rémy Agniel, Emeline Camand, Sana Hammour and Caroline Loy as well as the blood donor volunteers.
Funding
This work was supported by the Natural Science and Engineering Research Council (NSERC) of Canada, Samuel de Champlain Program, Center de Recherche sur les Matériaux Avancés (CERMA-ULaval) and Research Center of the CHU de Québec. Vanessa Montaño-Machado is a recipiendary of a PhD scholarship from CONACYT Mexico and a mobility scholarship from Ile de France region.
References
- Choussat R, Black A, Bossi I, Fajadet J, Marco J. Vascular complications and clinical outcome after coronary angioplasty with platelet IIb/IIIa receptor blockade - Comparison of transradial vs transfemoral arterial access. Eur Heart J 2000; 21:662-7; PMID:10731404; http://dx.doi.org/10.1053/euhj.1999.1945
- Cutlip DE, Chhabra AG, Baim DS, Chauhan MS, Marulkar S, Massaro J, Bakhai A, Cohen DJ, Kuntz RE, Ho KKL. Beyond restenosis - Five-year clinical outcomes from second-generation coronary stent trials. Circulation 2004; 110:1226-30; PMID:15337693; http://dx.doi.org/10.1161/01.CIR.0000140721.27004.4B
- Bhala N, Taggar JS, Rajasekhar P, Banerjee A. Anticipating and managing bleeding complications in patients with coronary stents who are receiving dual antiplatelet treatment. Brit Med J 2011; 343; PMID:21778196; http://dx.doi.org/10.1136/bmj.d4264
- Helqvist S, Kelbak H, Thuesen L, Klovgaard L, Krusell LR, Jorgensen E, Botker HE, Jensen GVH, Lassen JF, Thayssen P, et al. Efficiency and safety of the sirolimus eluting stent in complex coronary artery lesions after cessation of dual antiplatelet therapy: fifteen months clinical outcome of the randomised Stenting Coronary Arteries in Non-stress/benestent Disease (SCANDSTENT) trial. EuroIntervention 2007; 2:309-14; PMID:19737710; http://dx.doi.org/10.4244/EIJV3I3A57
- Kipshidze N, Dangas G, Tsapenko M, Moses J, Leon MB, Kutryk M, Serruys P. Role of the endothelium in modulating neointimal formation - Vasculoprotective approaches to attenuate restenosis after percutaneous coronary interventions. J AM Coll Cardiol 2004; 44:733-9; PMID:15312851
- Grinnell F, Feld MK. Adsorption characteristics of plasma fibronectin in relationship to biological activity. J Biomed Mater Res 1981; 15:363-81; PMID:7348271; http://dx.doi.org/10.1002/jbm.820150308
- Pettit DK, Horbett TA, Hoffman AS. Influence of the substrate binding characteristics of fibronectin on corneal epithelial-cell outgrowth. J Biomed Mater Res 1992; 26:1259-75; PMID:1429748; http://dx.doi.org/10.1002/jbm.820261002
- Iuliano DJ, Saavedra SS, Truskey GA. Effect of the conformation and orientation of adsorbed fibronectin on edothelial-cell spreading and the strenght of adhesion. J Biomed Mater Res 1993; 27:1103-13; PMID:8408123; http://dx.doi.org/10.1002/jbm.820270816
- Garcia AJ, Vega MD, Boettiger D. Modulation of cell proliferation and differentiation through substrate-dependent changes in fibronectin conformation. Mol Biol Cell 1999; 10:785-98; PMID:10069818; http://dx.doi.org/10.1091/mbc.10.3.785
- Patel S, Chaffotte AF, Goubard F, Pauthe E. Urea-induced sequential unfolding of fibronectin: a fluorescence spectroscopy and circular dichroism study. Biochemistry 2004; 43:1724-35; PMID:14769050; http://dx.doi.org/10.1021/bi0347104
- Patel S, Chaffotte AF, Amana B, Goubard F, Pauthe E. In vitro denaturation-renaturation of fibronectin. Formation of multimers disulfide-linked and shuffling of intramolecular disulfide bonds. Int J Biochem Cell Biol 2006; 38:1547-60; PMID:16697243; http://dx.doi.org/10.1016/j.biocel.2006.03.005
- Pauthe E, Pelta J, Patel S, Lairez D, Goubard F. Temperature-induced beta-aggregation of fibronectin in aqueous solution. Biochim Biophys Acta 2002; 1597:12-21; PMID:12009397; http://dx.doi.org/10.1016/S0167-4838(02)00271-6
- Pelta J, Berry H, Fadda GC, Pauthe E, Lairez D. Statistical conformation of human plasma fibronectin. Biochemistry 2000; 39:5146-54; PMID:10819982; http://dx.doi.org/10.1021/bi992770x
- Cai G, Lian J, Shapiro SS, Beacham DA. Evaluation of endothelial cell migration with a novel in vitro assay system. Meth Cell Sci 2000; 22:107-14; PMID:11264960; http://dx.doi.org/10.1023/A:1009864613566
- Baujard-Lamotte L, Noinville S, Goubard F, Marque P, Pauthe E. Kinetics of conformational changes of fibronectin adsorbed onto model surfaces. Colloid Surf B Biointerfaces 2008; 63:129-37; PMID:18249527; http://dx.doi.org/10.1016/j.colsurfb.2007.11.015
- Vallieres K, Petitclerc E, Laroche G. Covalent grafting of fibronectin onto plasma-treated PTFE: influence of the conjugation strategy on fibronectin biological activity. Macromol Biosci 2007; 7:738-45; PMID:17457945; http://dx.doi.org/10.1002/mabi.200600267
- Cho JH, Mosher DF. Enhancement of thrombogenesis by plasma fibronectin cross-linked to fibrin and assembled in platelet thrombi. Blood 2006; 107:3555-63; PMID:16391013; http://dx.doi.org/10.1182/blood-2005-10-4168
- Sima F, Davidson P, Pauthe E, Sima LE, Gallet O, Mihailescu IN, Anselme K. Fibronectin layers by matrix-assisted pulsed laser evaporation from saline buffer-based cryogenic targets. Acta Biomater 2011; 7:3780-8; PMID:21704740; http://dx.doi.org/10.1016/j.actbio.2011.06.016
- Durrani AA, Hayward JA, Chapman D. Biomembranes as models for polymer surfaces .2. The Synth of reactive species for covalent coupling of phosphorylcholine to polymer surfaces Biomaterials 1986; 7:121-5; PMID:3708063
- Hayward JA, Durrani AA, Lu Y, Clayton CR, Chapman D. Biomembranes as models for polymer surfaces .4. ESCA analysis of a phosphorylcholine surface covalently bond to hydroxylated substrates. Biomaterials 1986; 7:252-6; PMID:3741959; http://dx.doi.org/10.1016/0142-9612(86)90045-1
- Haïdopoulos M, Turgeon S, Laroche G, Mantovani D. Chemical and Morphological Characterization of Ultra-Thin Fluorocarbon Plasma-Polymer Deposition on 316 Stainless Steel Substrates: A First Step Toward the Improvement of the Long-Term Safety of Coated-Stents. Plasma Proc Polym 2005; 2:424-40; http://dx.doi.org/10.1002/ppap.200400066
- Lewis F, Horny P, Hale P, Turgeon S, Tatoulian M, Mantovani D. Study of the adhesion of thin plasma fluorocarbon coatings resisting plastic deformation for stent applications. J Phys D Appl Phys 2008; 41.
- Holvoet S, Chevallier P, Turgeon S, Mantovani D. Toward High-Performance Coatings for Biomedical Devices: Study on Plasma-Deposited Fluorocarbon Films and Ageing in PBS. Materials 2010; 3:1515-32; http://dx.doi.org/10.3390/ma3031515
- Lewis AL, Tolhurst LA, Stratford PW. Analysis of a phosphorylcholine-based polymer coating on a coronary stent pre- and post-implantation. Biomaterials 2002; 23:1697-706; PMID:11922473; http://dx.doi.org/10.1016/S0142-9612(01)00297-6
- Chevallier P, Janvier R, Mantovani D, Laroche G. In vitro biological performances of phosphorylcholine-grafted ePTFE prostheses through RFGD plasma techniques. Macromol Biosci 2005; 5:829-39; PMID:16134089; http://dx.doi.org/10.1002/mabi.200500088
- Poulouin L, Gallet O, Rouahi M, Imhoff JM. Plasma fibronectin: Three steps to purification and stability. Protein Expr Purif 1999; 17:146-52; PMID:10497080; http://dx.doi.org/10.1006/prep.1999.1103
- Hermarson G. Bioconjugate techniques. Academic Press: San Diego, CA; 1996.
- Boccafoschi F, Habermehl J, Vesentini S, Mantovani D. Biological performances of collagen-based scaffolds tor vascular tissue engineering. Biomaterials 2005; 26:7410-7; PMID:15998538; http://dx.doi.org/10.1016/j.biomaterials.2005.05.052
- Teichmann J, Morgenstern A, Seebach J, Schnittler H-J, Werner C, Pompe T. The control of endothelial cell adhesion and migration by shear stress and matrix-substrate anchorage. Biomaterials 2012; 33:1959-69; PMID:22154622; http://dx.doi.org/10.1016/j.biomaterials.2011.11.017
- Lei Y, Remy M, Labrugere C, Durrieu M-C. Peptide immobilization on polyethylene terephthalate surfaces to study specific endothelial cell adhesion, spreading and migration. J Mater Sci-Mater Med 2012; 23:2761-72; PMID:22878726; http://dx.doi.org/10.1007/s10856-012-4736-x
- Meng S, Liu Z, Shen L, Guo Z, Chou LL, Zhong W, Du Q, Ge J. The effect of a layer-by-layer chitosan-heparin coating on the endothelialization and coagulation properties of a coronary stent system. Biomaterials 2009; 30:2276-83; PMID:19168214; http://dx.doi.org/10.1016/j.biomaterials.2008.12.075
- Parkinson RJ, Demers CP, Adel JG, Levy EI, Sauvageau E, Hanel RA, Shaibani A, Guterman LR, Hopkins LN, Batjer HH, et al. Use of heparin-coated stents in neurovascular interventional procedures: Preliminary experience with 10 patients. Neurosurgery 2006; 59:812-21; PMID:17038945; http://dx.doi.org/10.1227/01.NEU.0000232836.66310.46
- Kuiper KKJ, Nordrehaug JE. Early mobilization after protamine reversal of heparin following implantation of phosphorylcholine-coated stents in totally occluded coronary arteries. Am J Cardiol 2000; 85:698-702; PMID:12000042; http://dx.doi.org/10.1016/S0002-9149(99)00843-7
- Li G, Yang P, Qin W, Maitz MF, Zhou S, Huang N. The effect of coimmobilizing heparin and fibronectin on titanium on hemocompatibility and endothelialization. Biomaterials 2011; 32:4691-703; PMID:21463893; http://dx.doi.org/10.1016/j.biomaterials.2011.03.025
- Wang HG, Yin TY, Ge SP, Zhang Q, Dong QL, Lei DX, Sun DM, Wang GX. Biofunctionalization of titanium surface with multilayer films modified by heparin-VEGF-fibronectin complex to improve endothelial cell proliferation and blood compatibility. J Biomed Mater Res A 2013; 101A:413-20; PMID:22865832; http://dx.doi.org/10.1002/jbm.a.34339
- Tang C, Wang G, Wu X, Li Z, Shen Y, Lee JCM, Yu Q. The impact of vascular endothelial growth factor-transfected human endothelial cells on endothelialization and restenosis of stainless steel stents. J Vasc Surg 2011; 53:461-71; PMID:21129910; http://dx.doi.org/10.1016/j.jvs.2010.08.020
- Luo C, Zheng Y, Diao Z, Qiu J, Wang G. Review: Research Progress and Future Prospects for Promoting Endothelialization on Endovascular Stents and Preventing Restenosis. J Med Biol Eng 2011; 31:307-16; http://dx.doi.org/10.5405/jmbe.958
- Hall B, Bird RL, Kojima M, Chapman D. Biomembranes as models for polymer surfaces .5. Thromboelastographic studies of polymeric lipids and polyesters. Biomaterials 1989; 10:219-24; PMID:2525934; http://dx.doi.org/10.1016/0142-9612(89)90096-3
- Chen J, Cao J, Wang J, Maitz MF, Guo L, Zhao Y, Li Q, Xiong K, Huang N. Biofunctionalization of titanium with PEG and anti-CD34 for hemocompatibility and stimulated endothelialization. J Colloid Interface Sci 2012; 368:636-47; PMID:22172691; http://dx.doi.org/10.1016/j.jcis.2011.11.039
- Kim JH, Shin JH, Shin DH, Moon MW, Park K, Kim TH, Shin KM, Won YH, Han DK, Lee KR. Comparison of diamond-like carbon-coated nitinol stents with or without polyethylene glycol grafting and uncoated nitinol stents in a canine iliac artery model. Brit J Radiol 2011; 84:210-5.; PMID:21325363;http://dx.doi.org/10.1259/bjr/21667521