Abstract
Mitochondria regulate metabolism and homeostasis within cells. Mitochondria are also very dynamic organelles, constantly undergoing fission and fusion. The importance of maintaining proper mitochondrial dynamics is evident in the various diseases associated with defects in these processes. Protein kinase A (PKA) is a key regulator of mitochondrial dynamics. PKA is spatially regulated by A-Kinase Anchoring Proteins (AKAPs). We completed cloning of a novel AKAP350 isoform, AKAP350C. Immunostaining for endogenous AKAP350C showed localization to mitochondria. The carboxyl-terminal 54-amino acid sequence unique to AKAP350C contains a novel amphipathic alpha helical mitochondrial-targeting domain. AKAP350C co-localizes with Mff (mitochondrial fission protein) and mitofusins 1 and 2 (mitochondrial fusion proteins), and likely regulates mitochondrial dynamics by scaffolding PKA and mitochondrial fission and fusion proteins.
Introduction
Mitochondria have crucial roles within cells, regulating metabolism, decisions between cell survival and death, redox biochemistry, and calcium homeostasis.Citation1 Mitochondria also play a role in calcium signaling, in concert with and independently of the endoplasmic reticulum (ER).Citation2,3 Because of their many roles, mitochondria are critical locations for signal integration. Mitochondria also exhibit localized cyclic AMP (cAMP)/Protein Kinase A (PKA) signaling, and cAMP is involved in many aspects of cell function and survival.Citation4 Decisions between cell survival and cell death, through apoptosis or autophagy, are largely regulated by mitochondria.Citation5 Mitochondrial biogenesis and activity can be regulated by changes in cAMP/PKA signaling. PKA-regulated ion channels also exist in the mitochondrial membranes.Citation6 Some cAMP/PKA-regulated calcium and potassium channels play roles in cardio protection.Citation6
Mitochondria are highly dynamic, constantly undergoing fission and fusion and maintaining a balance between the two processes.Citation7 When the balance is shifted toward increased fusion or decreased fission, mitochondria become elongated and hyperfused.Citation7 When the balance is shifted toward decreased fusion or increased fission, the mitochondria become fragmented.Citation7 This dynamic nature of mitochondria morphology also affects nearly every aspect of mitochondrial function. The importance of proper mitochondrial dynamics is evident in the various diseases associated with defects in mitochondrial dynamics. Mutations in mitofusin 2 (Mfn2), a mitochondrial outer membrane fusion protein, are known to cause Charcot-Marie-Tooth type 2A.Citation8 Autosomal dominant Optic Atrophy is caused by mutations in optic atrophy 1 (Opa1), a mitochondrial inner membrane fusion protein.Citation9 Defects in mitochondrial dynamics have also been associated with Parkinson, Alzheimer, and Huntington's diseases.Citation7,10
Because of the importance of mitochondrial dynamics, the processes of fission and fusion are highly regulated. PKA is an important regulator of mitochondrial dynamics.Citation11 Drp1 (dynamin-related protein 1), a mitochondria fission protein, is inactivated by PKA phosphorylation, resulting in decreased fission and increased mitochondria elongation,Citation12 a process that promotes cell survival. Mfn2 is also phosphorylated by PKA, and again this promotes cell survival.Citation13 PKA signaling at the mitochondria regulates mitochondrial dynamics, but it also is involved in other signaling pathways as well. The complete details of signal integration at mitochondria remain unclear.
A-Kinase Anchoring Proteins (AKAPs) were discovered as PKA anchors that establish localized cAMP/PKA signaling through sequestration of PKA, but they play many other roles in protein scaffolding.Citation14,15 AKAPs function as anchors for targeting proteins to specific subcellular locations, and the localization and composition of AKAP complexes is dynamic. AKAPs comprise a very diverse family of proteins, with 50 AKAPs identified to date.Citation16 The importance of AKAP function is evident in the embryonic lethality of most AKAP knockout mice.Citation17 At least one AKAP is found in every tissue in the body.Citation18
Previous studies have identified AKAPs involved with the mitochondria. AKAP149 (also known as D-AKAP1 and AKAP121 in mouse) localizes to both the ER and mitochondria and plays a role in stress response in cardiomyocytes.Citation19-21 When AKAP149 is displaced from the mitochondria it induces mitochondrial dysfunction.Citation21 This causes an increase in reactive oxygen species, and therefore induced oxidative stress, in cardiomyocytes, smooth muscle cells, and hypertrophic mouse hearts in vivo.Citation21 AKAP149 anchors proteins and RNAs at the mitochondrial outer membrane, and plays an important role in cAMP signaling. Overexpression of AKAP149 reduces apoptosis.Citation22 Rab32, also an AKAP, interacts with the ER and mitochondria. Rab32 localizes to mitochondria-associated membranes (MAMs), where it serves to regulate MAM properties and interacts with Drp1.Citation23 High expression levels of Rab32 delay apoptosis, while low expression levels of Rab32 accelerate apoptosis. It is possible other AKAPs localize to mitochondria and serve as regulators of their dynamics and functions.
We have investigated the functions of splice variants of AKAP350, also known as AKAP450, AKAP9, and CG-NAP.Citation24-26 There are several known splice isoforms of AKAP350: yotiao, AKAP350A, AKAP350B, and AKAP350C. Yotiao, associated with plasma membranes in excitable cells, and AKAP350A, associated with centrosomes and the Golgi apparatus, are the most studied isoforms. We previously identified AKAP350B and AKAP350C isoforms as potential splice variants, but their functions have not been studied further.Citation27
In this study, we completed cloning of AKAP350C and identified it as a mitochondria-targeted AKAP. AKAP350C lacks the centrosome- and Golgi-targeting domains found in AKAP350A, but AKAP350C has a unique mitochondrial targeting sequence in its carboxyl terminus consisting of an amphipathic alpha helix. This alpha helix is necessary and sufficient for mitochondrial localization of AKAP350C. AKAP350C co-localizes with Mff (mitochondria fission factor) and mitofusins 1 and 2, suggesting a role for AKAP350C in regulating mitochondrial dynamics.
Results
Characterization of AKAP350C
AKAP350C was previously identified using 3′-rapid amplification of cDNA ends (RACE) in a human lung cDNA.Citation24,27 We have now completed cloning of AKAP350C from CaCo-2 (human colorectal adenocarcinoma) RNA. The AKAP350C cDNA contains a 9378 nt open reading frame that codes for a protein of 3126 amino acids. AKAP350C lacks the carboxyl-terminal region containing centrosomal- and Golgi-targeting domains found in AKAP350A ().Citation24,27,28 Instead, the carboxyl terminus of AKAP350C contains a unique 54-amino acid sequence (). An amphipathic alpha helix is predicted in the first half of this sequence ( and ). AKAP350C is ubiquitously expressed among tissues and cell lines investigated (data not shown).
Figure 1. Characterization of AKAP350C. (A) The AKAP350 splice variant is determined by the ending of the protein (red). Both variants contain two PKA RIIα-binding domains (yellow). AKAP350A contains one leucine zipper region (green), while AKAP350C contains only one. Other domains include YHR (Yotiao homology region; gray), Golgi (Golgi-targeting domain; purple), and PACT (pericentrin and AKAP350 centrosomal-targeting domain; blue). (B) AKAP350C has a unique 54-amino acid sequence, which contains a predicted alpha helix (Protean). (C) The alpha helix predicted is amphipathic. White circles are hydrophobic residues, black are charged, and gray are polar. (D) HeLa cells were stained for endogenous AKAP350C (green), MTCO2 (mitochondria; blue), and calreticulin (ER; red). (Scale bar: 20 μm).
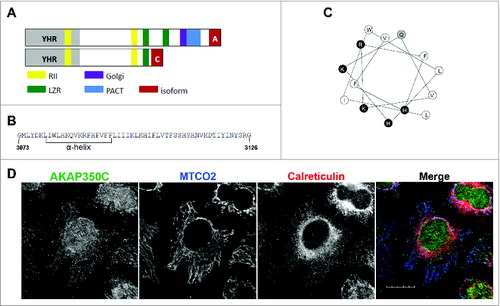
To examine the endogenous localization of AKAP350C, we made 2 isoform-specific antibodies against different epitopes in the AKAP350C-specific carboxyl terminal sequence and validated the specificity of these antibodies (). In immunofluorescence staining, both antibodies showed mitochondrial localization of AKAP350C in all cells that we examined (). In the experiments in this study we used the antibody produced against the AKAP350C specific peptide sequence DKLIWLHKQVKRFHFV.
Figure 2. Specificity of AKAP350C antibodies. (A) CaCo2 (colon adenocarcinoma) cells and HeLa cells (B) were grown on collagen-coated coverslips. Cells were stained for AKAP350C (green), mitochondria (MTCO2, red), and nuclei (DAPI, blue). The AKAP350C antibody was pre-incubated with either PBS or 20 μM blocking antigen peptide. (Scale bars: 20 μm).
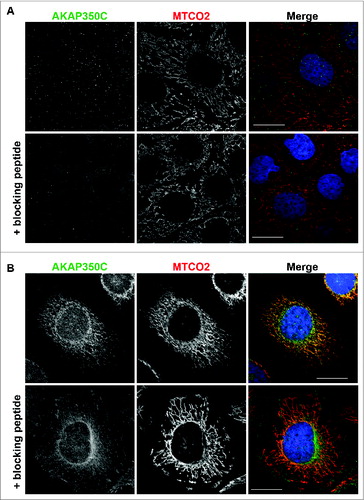
An amphipathic alpha helix is required for targeting to mitochondria
The AKAP350C sequence contains no known mitochondrial-targeting motifs. The carboxyl-terminal 54 amino acids is the only sequence unique to AKAP350C, so we hypothesized that the mitochondrial-targeting domain is contained within that unique sequence. The only identifiable structural feature within the unique sequence was an amphipathic alpha helix ( and ). Therefore, we evaluated whether the amphipathic alpha helix was required for mitochondrial localization of AKAP350C using synthetic constructs to overexpress GFP-tagged AKAP350C constructs (). We created several mutant constructs for GFP-chimeras of amino acids 2644–3126 of AKAP350C (AKAP350C-F3) following the schematic in . The overexpressed GFP-AKAP350C-F3 localized in a distribution that overlaped with mitochondria and the ER (), suggesting that the carboxyl-terminal amino acids can specify targeting to mitochondria.
Figure 3. The AKAP350C carboxyl-terminal amphipathic alpha helix is required for targeting to mitochondria. (A) Schematic of GFP-AKAP350C truncation and deletion constructs. (B) HeLa cells were grown on collagen-coated coverslips. Cells were transfected with various AKAP350C constructs using Polyjet and stained for MTCO2 (mitochondria; blue) and calreticulin (ER; red). (Scale bars: 20 μm).
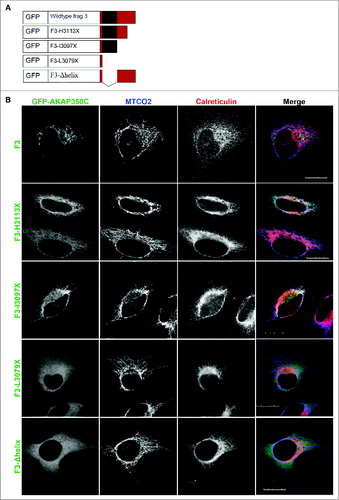
We next examined several truncation and point mutants for their ability to target to mitochondria. The truncation mutant F3-L3079X, which only contains 6 of the 54 carboxyl terminal amino acids, abolished targeting to the mitochondria (), supporting the importance of the unique AKAP350C carboxyl terminus in specifying mitochondrial targeting. The truncation mutant F3-I3097X, which lacks the amino acids after the alpha helix, retained an enhanced mitochondria targeting (). Finally, removal of the alpha helix (F3-Δhelix) also blocked targeting to the mitochondria (). Thus, any mutation that deleted or disrupted the alpha helix abolished mitochondrial targeting of AKAP350C.
Interestingly, the truncation mutant F3-H3113X, which is missing the last 14 amino acids, overlaped with both mitochondria and the ER (). This suggests that an ER retention or targeting sequence exists within amino acids 3097 to 3113, although there is no known targeting motif within this region. Double mutants F3-L3082P-I3097X and F3-V3086P-I3097X no longer targeted to the mitochondria (). Proline substitution mutants F3-L3082P and F3-V3086P, which disrupt the alpha helical structure, also abolished mitochondrial targeting of AKAP350C ().
Figure 4. The amphipathic alpha helix structure is required for targeting to mitochondria. (A) Schematic of GFP-AKAP350C point mutation constructs. (B) HeLa cells were grown on collagen-coated coverslips. Cells were transfected with various AKAP350C constructs using Polyjet and stained for MTCO2 (mitochondria; blue) and calreticulin (ER; red). (Scale bars: 20 μm).
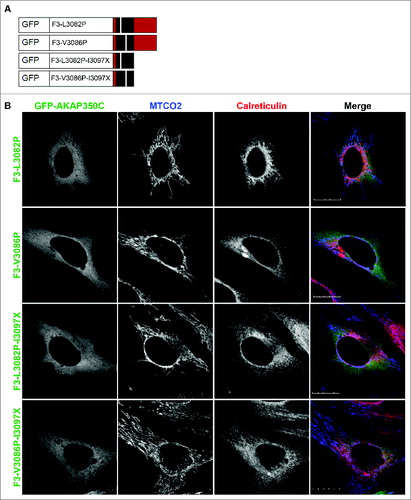
Biochemical confirmation that the amphipathic alpha helix is required for association with mitochondria
We next utilized a biochemical approach to evaluate the subcellular distribution of the various AKAP350C mutants. Mitochondria were isolated from HEK-293T cells expressing the AKAP350C mutants and compared to other subcellular fractions. As predicted based on data in , the mutant lacking the amphipathic alpha helix, F3-Δhelix, abolished association with mitochondria. Surprisingly, this mutant was predominantly found in microsomal fraction, which may suggest some degree of association with the ER (). AKAP350C constructs that retained an intact amphipathic helix localized with mitochondria, including F3, F3-I3097X, and F3-H3113X (). Again, both wildtype F3 and F3-H3113X localized in both mitochondrial and microsomal fractions. These findings suggest that amino acids 3082–3097 contain the mitochondrial-targeting domain, while amino acids 3097–3113 may contain an ER retention or targeting sequence. All of these results confirm that the amphipathic alpha helix is required for AKAP350C association with the mitochondria.
Figure 5. Biochemical confirmation that the amphipathic alpha helix is required for AKAP350C mitochondrial association. Mitochondria were isolated from HEK-293 cells transfected with GFP-AKAP350C constructs. In each case, Lane 1 contains the mitochondrial fraction; Lane 2 contains the microsomal fraction (pellet of 100,000 × g centrifugation); and Lane 3 contains the supernatant of the 100,000 × g centrifugation. In each lane, 12.5 μg of protein were loaded. Membranes were probed for MTCO2 (mitochondria), calreticulin (ER), and GFP (AKAP350C construct). The GFP westerns shown were taken at different exposures due to differences in levels of expression.
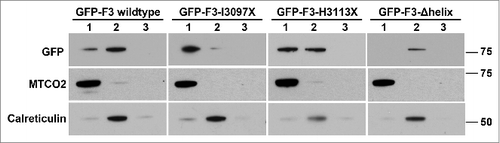
AKAP350C co-localizes with mitochondrial fission and fusion proteins
Given that AKAP350C can scaffold Protein kinase A, which is a regulator of mitochondrial fission/fusion dynamics,Citation11 we sought to determine whether AKAP350C is associated with fission regulator proteins such as Mff and fusion regulator proteins such as Mfn1 and Mfn2. We examined immunostaining of endogenous AKAP350C in HeLa cells overexpressing mCherry-Mff, mCherry-Mfn1 or mCherry-Mfn2 (). As described previously,Citation29,30 Mff overexpression resulted in short, fragmented mitochondria, whereas overexpression of mitofusins induced a spectrum of mitochondrial fusion leading to eventual mitochondrial collapse and formation of perinuclear clusters with enlarged mitochondria.Citation31,32 We observed extensive co-localization of AKAP350C with Mff (), Mfn1 ( and ) and Mfn2 ( and ).
Figure 6. Endogenous AKAP350C co-localizes with overexpressed fission/fusion proteins (Mff/Mitofusins). HeLa cells were transfected with either Cherry-Mff (A), Cherry-Mfn1 (B, C), or Cherry-Mfn2 (D, E) and dual-stained for endogenous AKAP350C (green) and mitochondria marker MTCO2 (blue). Note, that higher levels of expression of mitofusins ((C) and (E) for Mfn1 and Mfn2, respectively) alter the pattern of endogenous AKAP350C staining. The degree of co-localization between endogenous AKAP (green) and overexpressed Mff/Mfn1/Mfn2 (red) were quantified using Pearson's Correlation Coefficient (PCC). PCCs were determined using JACOP plug-in of ImageJ software. PCC: (A) 0.918; (B) 0.908; (C) 0.920; (D) 0.938; (E) 0.880. (Scale bars: 20 μm).
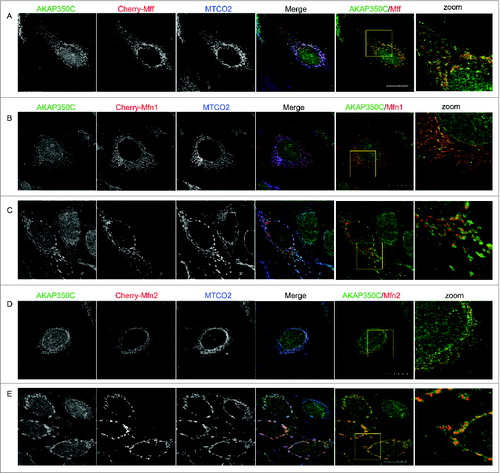
The extent of mitochondrial fusion, and therefore mitochondrial shape, varied depending on the level of mitofusin protein expression. Low levels of mitofusin expression resulted in an elongated, fused mitochondrial network with extensive co-localization of endogenous AKAP350C (see and for Mfn1 and Mfn2, respectively). Higher levels of overexpression of mitofusins encouraged formation of further fused mitochondrial clusters (see and for Mfn1 and Mfn2, respectively) that eventually collapsed around the perinuclear region. We also noted that overexpression of mitofusins changed the pattern of endogenous AKAP350C staining, with endogenous AKAP350C accumulating with the fused mitochondria ( and ). The co-localization of AKAP350C with both mitochondria fission and fusion proteins supports the concept of a dynamic organization of AKAP350C scaffolding complexes, which may scaffold fission/fusion proteins at the mitochondria.
Overexpressed GFP-AKAP350C-F3-I3097X also significantly overlapped with overexpressed Cherry-Mff, Cherry-Mfn1 and Cherry-Mfn2, whereas the F3-Δhelix (a non-targeting mutant) was no longer targeted to mitochondria and did not co-localize with either Mff or mitofusins (). Finally, the GFP-AKAP350C-F3-I3097X construct, but not the F3-Δhelix mutant, also greatly overlapped with endogenous Mff on mitochondria. ().
Figure 7. Overexpressed AKAP350C co-localizes with fission/fusion proteins (Mff/Mitofusins). HeLa cells were transfected with GFP-F3-I3097X or GFP-F3-Δhelix AKAP350C (green) and either Cherry-Mff (A), Cherry-Mfn1 (B), or Cherry-Mfn2 (C) (red) and then imunostained for the mitochondrial marker MTCO2 (blue). The degree of co-localization between AKAP (green) and Mff/Mfn1/Mfn2 (red) was quantified using Pearson's Correlation Coefficient (PCC). PCCs were determined using the JACOP plug-in for ImageJ software. PCCs were 0.943 for GFP-F3-I3097X with Cherry-Mff, 0.775 for GFP-F3-I3097X with Cherry-Mfn1, and 0.970 for GFP-F3-I3097X with Cherry-Mfn2. F3-Δhelix mutant of AKAP350C was not co-localized with mitochondria. (Scale bars: 20 μm).
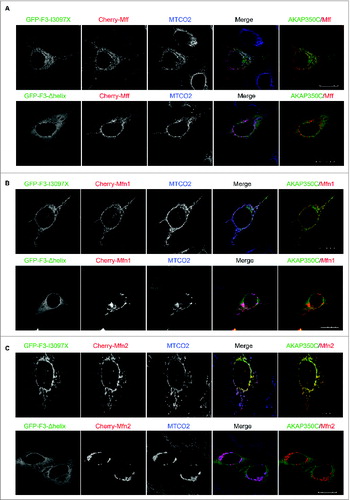
Figure 8. Overexpressed F3-I3097X, but not the F3-Δhelix mutant, co-localized with endogenous Mff. HeLa cells were transfected with GFP-AKAP360C (F3-I3097X or F3-Δhelix) (green) and dual-stained with antibodies against Mff (red) and the mitochondrial marker MTCO2 (blue). The degree of co-localization between AKAP (green) and Mff (red) was quantified using Pearson's Correlation Coefficient (PCC). PCCs were determined using the JACOP plug-in for ImageJ software. The PCC = 0.932 for GFP-F3-I3097X with endogenous Mff (red). F3-Δhelix mutant of AKAP350C was not co-localized with mitochondria. (Scale bars: 20 μm).
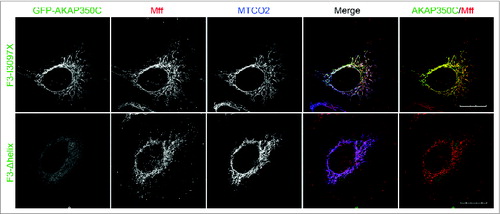
Discussion
In this study, we identified a novel AKAP350 splice variant, AKAP350C,that localizes to mitochondria. We completed cloning of AKAP350C, which contains 54 amino acids of unique protein sequence, with the remaining sequence being homologous to AKAP350A. This relatively small unique sequence greatly differentiates AKAP350C from AKAP350A in terms of subcellular distribution and role. We also identified a novel mitochondrial-targeting domain within AKAP350C. The carboxyl-terminal 54-amino acid region contains an amphipathic alpha helix that is required for mitochondrial targeting of AKAP350C. AKAP350C also co-localized with Mff and mitofusins, suggesting its possible role in the regulation of mitochondrial fusion and fission dynamics.
While it is clear that the amphipathic alpha helix in the unique carboxyl terminal of AKAP350C is required for targeting to the mitochondria, the interacting partner for this helix remains unclear. It is possible that AKAP350C can interact with lipids on the mitochondrial outer membrane through either hydrophobic interactions or electrostatic charge. In preliminary studies, we have not been able to identify any direct interactions with either phospholipids or phosphatidyl-inositides (data not shown). Proteins such as hexokinase I contain domains of charged and hydrophobic regions that lay upon the surface of the outer membrane.Citation33,34 Additionally a similar complex binding mechanism has been proposed for D-AKAP1 localization to mitochondria.Citation35 Further investigations will be required to determine the discrete molecular mechanisms responsible for AKAP350C association with mitochondria.
Mitochondria are critical signaling integration centers, and AKAPs serve to scaffold many proteins in close proximity for sequestration of coordinated signaling within cells, thereby allowing greater spatial and temporal control of signaling cascades. Loss of an AKAP protein, and subsequently the signal integration it provides, is typically embryonic lethal in knockout mice.Citation17 AKAP350C likely serves as a signaling focal point for the regulation of mitochondrial dynamics, scaffolding PKA and mitochondria fission and fusion proteins in close proximity. Upon Mfn1 or Mfn2 overexpression, AKAP350C colocalized with mitofusins. AKAP350C-mediated scaffolding could lead to local coordination of phosphorylation and dephosphorylation of fusion and fission proteins. Though AKAP350C knock-down studies could aid in this investigation, all attempts at knocking down AKAP350C have been unsuccessful. There is limited AKAP350C-specific sequence available as shRNA or siRNA targets.
Mitochondrial dynamics and the balance between fission and fusion is an important process in regulating cellular stress response.Citation36 Our data showed that AKAP350C co-localizes with mitochondrial fission/fusion proteins and likely aids in regulating mitochondrial dynamics. Another splice variant, AKAP350A, serves dual roles, localizing to the Golgi apparatusCitation27 and centrosomeCitation24 during non-stress conditions while re-localizing to stress granules during stress response.Citation37,38
Other AKAP proteins are known to localize to mitochondria, including AKAP149 and Rab32. Seemingly redundant, these AKAPs likely function uniquely under steady-state conditions. For example, AKAP149 is involved in mitochondrial RNA processing, but there is no known role for Rab32 or AKAP350C involving RNA. It is possible these mitochondria-targeted AKAPs are both redundant and distinct, meaning that they play different roles when all are present, but can compensate at least partially if one is lost. Mitofusins 1 and 2 are one example of this distinct and redundant strategy employed for mitochondrial dynamics.Citation39 Mfn1 and Mfn2 are 60% homologousCitation31 and have shared functions, but each mitofusin also functions uniquely. When Mfn1 is knocked down or knocked out, Mfn2 can only partially compensate, and vice versa. Given the crucial role for mitochondria in cell survival, this redundancy could account for the localization of multiple AKAPs at mitochondria. This redundant and distinct theory can also account for the difficulty of studying the cAMP/PKA signaling changes induced by alterations in AKAP expression. The regulation of PKA scaffolded by other AKAPs at the mitochondria could compensate for the increase or decrease of AKAP350C-scaffolded PKA induced by AKAP350C mutant overexpression.
In summary, we have identified AKAP350C as a mitochondrial-associated A-kinase scaffolding protein, which also co-localizes with mitochondrial fusion and fission proteins. Since mitochondrial fission and fusion proteins participate in neurodegenerative, cardiovascular, and other diseases,Citation40 AKAP350C acting as a signal integration center, could provide an important future therapeutic target for regulation of mitochondrial function in stress and disease.
Materials and Methods
Cloning of AKAP350C
We previously cloned the 3′-end of AKAP350C from a 3′-RACE strategy using a human lung cDNA.Citation24,27 To amplify the complete sequence, we synthesized a cDNA from 5 μg of CaCo-2 cell total RNA using an anti-sense primer specific for AKAP350C (AS1: CGTAGCTGTTTAAACCAATTAGACAATCCTC). The resulting cDNA was then used for as template for amplification using Advantage Taq polymerase (Clontech) of the AKAPA350C sequence with a nested AKAP350C anti-sense primer (AS2: CTAACCCCTAGAGTAATTAATATAGATTGTATC) and Sense primers from all 50 exons of the known AKAP9 gene. The largest polymerized fragment was obtained with a sense primer from Exon 5 (Exon 5-S: CTGAACAAGGAGCACAAGACAGTCCGAC) and AS2. To complete the 5′ sequence, we performed created a 5′-RACE linkered RNA from CaCo-2 RNA (Ambion RLM-RACE) and synthesized cDNA primer with AS1. The 5′ ends were then amplified with a universal primer from the linker region (5′-RACE inner) and an antisense primer against Exon 5. The resulting PCR fragments were cloned into pTOPO and sequenced to determine authentic 5′ sequences. The complete DNA sequence was then assembled using DNASTAR software. The resulting sequence was deposited in Genbank as an update of the original AKAP350C 3′-cDNA sequence AF247727.
AKAP350C-specific antibodies
Two AKAP350C-specific antibodies were made. The first antibody was produced against SSHSHNVKDTIYINYSRG (Antibody Facility at the University of Georgia). The second antibody was produced against CDKLIWLHKQVKRFHFV (Covance). The peptide used for antibody production was used to block the antibody during staining to show specificity of antibodies.
Cell culture
HeLa cells (American Type Culture Collection, ATCC) were maintained at 37°C in 5% CO2 using complete RPMI media supplemented with 10% fetal bovine serum (FBS). HEK-293 cells (American Type Culture Collection, ATCC) were maintained at 37°C in 5% CO2 using complete DMEM media supplemented with 10% fetal bovine serum (FBS). Polyjet was used for transfections according to the manufacturer's protocol (SignaGen).
GFP-AKAP350C assembly
Synthetic constructs of AKAP350C fragments were synthesized by GeneART. Amino acids 1–1400 were received as fragment 1 (F1) in a modified pEGFP vector missing the HindIII site normally in the multicloning site. Amino acids 1360–2653 were received as fragment 2 (F2) in pMA vector. Finally, amino acids 2644–3126 were received as fragment 3 (F3) in pMA vector. AKAP350C-F3 was subcloned into pEFGP-C3 using XhoI and BamHI restriction site. Assembly of full length AKAP350C was achieved via triple ligation at 16C overnight using pEGFP-AKAP350C-F1 (cut with HindII and BamHI), AKAP350C-F2 (cut from pMA with HindIII and XhoI), and AKAP350C-F3 (cut with XhoI and BamHI).
Mutagenesis
Point mutations were created using Quickchange PCR techniques (Agilent Technologies). Primers were designed using the Quickchange primer design tool. The synthetic sequence of AKAP350C (GeneART) was used for all mutagenesis.
Plasmid construction
Human Mff was amplified from human heart tissue cDNA using primers 5′-GAGCGTCGACATGAGTAAAGGAACAAGCAGTGACAC-3′ and 5′-GAGCGGATCCCTAGCGGCGAAACCAGAGC-3′ and was ligated as a SalI/BamHI fragment into SalI/BamHI sites of pmCherry-C1 (a gift from Dr. Roger Tsien, University of California, San Diego). Human Mfn1 and Mfn2 were also amplified from human heart tissue cDNA using primers: 5′-GAGCGAATTC ATGGCAGAACCTGTTTCTCCACT-3′ and 5′-GAGCGGATCCTTAGGATTCT TCATTGCTTGAAGG-3′ (Mfn1) or 5′-GAGCGAATTCATGTCCCTGCTCTTCTC TCGATG-3′ and 5′-GAGCGGATCCCTATCTGCTGGGCTGCAGGTACT-3′ (Mfn2) and were ligated into EcoR1/BamH1 sites of pmCherry-C1.
Fluorescence microscopy and analysis
HeLa cells grown on collagen-coated coverslips were fixed at room temperature for 15 minutes using 4% paraformaldehyde (PFA) supplemented with 0.1% Triton X-100, 80 mM K-PIPES pH 7.2, 1 mM EGTA, 1 mM MgSO4, and 30% glycerol. Cells were permeabilized with 0.25% Triton X-100 and blocked with 5% normal serum for one hour at room temperature. The cells were incubated with primary antibodies for one hour at room temperature: rabbit-anti-AKAP350C serum (1:300), mouse-anti-MTCO2 (1:400, Abcam), chicken-anti-calreticulin (1:750, Abcam), mouse-anti-Mfn1 (1:200, Abcam), mouse-anti-Mfn2 (1:200, Abcam), and rabbit-anti-Mff (1:200, PTG Lab). This was followed by incubation at room temperature for one hour with species-specific fluorescent secondary antibodies (1:500; Invitrogen or Jackson Immunoresearch). Coverslips were mounted using Prolong Gold with DAPI (Invitrogen). Cells were imaged using a 60x oil immersion lens on Olympus FV-1000 confocal fluorescence microscope (Vanderbilt Cell Imaging Shared Resource. All experiments were repeated at least three times. Statistics for colocalization are detailed in the figure legends.
Mitochondria preparation and analysis
HEK-293 cells were transfected with AKAP350C constructs. Mitochondria were isolated using the Qproteome Mitochondria Isolation Kit (Qiagen) following the protocol for high-purity preparations. An additional 100,000 x g centrifugation step was performed on the microsomal fraction from the kit to further separate the ER and cytosolic fractions. Proteins from the ER and cytosol fractions were precipitated using the protein precipitation method in the Qproteome Mitochondria Isolation kit handbook. Protein concentrations of each fraction were taken using the BCA Protein Assay kit (Pierce), and 12.5 μg protein from each fraction was run in a 10% polyacrylamide gel. Proteins were transferred to nitrocellulose membrane. Primary antibodies used for western blotting were mouse-anti-MTCO2 (1:1,000; Abcam), rabbit-anti-calreticulin (1:1,000, Abcam), and rabbit-anti-GFP (1:5,000, Abcam). Secondary antibodies were donkey-anti-mouse-HRP (1:5,000) and donkey-anti-rabbit-HRP (1:5,000). Signal was detected using ECL Western Blotting Substrate (Pierce).
Disclosure of Potential Conflicts of Interest
No potential conflicts of interest were disclosed.
Additional information
Funding
References
- Horbinski C, Chu CT. Kinase signaling cascades in the mitochondrion: a matter of life or death. Free Radic Biol Med 2005; 38:2-11; PMID: 15589366; http://dx.doi.org/10.1016/j.freeradbiomed.2004.09.030
- Gunter TE, Buntinas L, Sparagna G, Eliseev R, Gunter K. Mitochondrial calcium transport: mechanisms and functions. Cell Calcium 2000; 28:285-96; PMID: 11115368; http://dx.doi.org/10.1054/ceca.2000.0168
- Dorn GW, 2nd, Scorrano L. Two close, too close: sarcoplasmic reticulum-mitochondrial crosstalk and cardiomyocyte fate. Circ Res 2010; 107:689-99; PMID: 20847324; http://dx.doi.org/10.1161/CIRCRESAHA.110.225714
- Carlucci A, Lignitto L, Feliciello A. Control of mitochondria dynamics and oxidative metabolism by cAMP, AKAPs and the proteasome. Trends Cell Biol 2008; 18:604-13; PMID: 18951795; http://dx.doi.org/10.1016/j.tcb.2008.09.006
- Fulda S, Gorman AM, Hori O, Samali A. Cellular stress responses: cell survival and cell death. Int J Cell Biol 2010; 2010:214074; PMID: 20182529
- Nishida H, Sato T, Ogura T, Nakaya H. New aspects for the treatment of cardiac diseases based on the diversity of functional controls on cardiac muscles: mitochondrial ion channels and cardioprotection. J Pharmacol Sci 2009; 109:341-7; PMID: 19270424; http://dx.doi.org/10.1254/jphs.08R24FM
- Chen H, Chan DC. Mitochondrial dynamics–fusion, fission, movement, and mitophagy–in neurodegenerative diseases. Hum Mol Genet 2009; 18:R169-76; PMID: 19808793; http://dx.doi.org/10.1093/hmg/ddp326
- Zuchner S, Mersiyanova IV, Muglia M, Bissar-Tadmouri N, Rochelle J, Dadali EL, Zappia M, Nelis E, Patitucci A, Senderek J, Parman Y, et al. Mutations in the mitochondrial GTPase mitofusin 2 cause Charcot-Marie-Tooth neuropathy type 2A. Nat Genet 2004; 36:449-51; PMID: 15064763; http://dx.doi.org/10.1038/ng1341
- Eiberg H, Kjer B, Kjer P, Rosenberg T. Dominant optic atrophy (OPA1) mapped to chromosome 3q region. I. Linkage analysis. Hum Mol Genet 1994; 3:977-80; PMID: 7951248; http://dx.doi.org/10.1093/hmg/3.6.977
- Liesa M, Palacin M, Zorzano A. Mitochondrial dynamics in mammalian health and disease. Physiol Rev 2009; 89:799-845; PMID: 19584314; http://dx.doi.org/10.1152/physrev.00030.2008
- Rambold AS, Lippincott-Schwartz J. Mechanisms of mitochondria and autophagy crosstalk. Cell Cycle 2011; 10:4032-8; PMID: 22101267; http://dx.doi.org/10.4161/cc.10.23.18384
- Chang CR, Blackstone C. Cyclic AMP-dependent protein kinase phosphorylation of Drp1 regulates its GTPase activity and mitochondrial morphology. J Biol Chem 2007; 282:21583-7; PMID: 17553808; http://dx.doi.org/10.1074/jbc.C700083200
- Chen KH, Guo X, Ma D, Guo Y, Li Q, Yang D, Li P, Qiu X, Wen S, Xiao RP, Tang J. Dysregulation of HSG triggers vascular proliferative disorders. Nat Cell Biol 2004; 6:872-83; PMID: 15322553; http://dx.doi.org/10.1038/ncb1161
- Theurkauf WE, Vallee RB. Molecular characterization of the cAMP-dependent protein kinase bound to microtubule-associated protein 2. J Biol Chem 1982; 257:3284-90; PMID: 6277931
- Lohmann SM, DeCamilli P, Einig I, Walter U. High-affinity binding of the regulatory subunit (RII) of cAMP-dependent protein kinase to microtubule-associated and other cellular proteins. Proc Natl Acad Sci U S A 1984; 81:6723-7; PMID: 6093118; http://dx.doi.org/10.1073/pnas.81.21.6723
- Wong W, Scott JD. AKAP signalling complexes: focal points in space and time. Nat Rev Mol Cell Biol 2004; 5:959-70; PMID: 15573134; http://dx.doi.org/10.1038/nrm1527
- Carnegie GK, Means CK, Scott JD. A-kinase anchoring proteins: from protein complexes to physiology and disease. IUBMB Life 2009; 61:394-406; PMID: 19319965; http://dx.doi.org/10.1002/iub.168
- Welch EJ, Jones BW, Scott JD. Networking with AKAPs: context-dependent regulation of anchored enzymes. Mol Interv 2010; 10:86-97; PMID: 20368369; http://dx.doi.org/10.1124/mi.10.2.6
- Ma Y, Taylor SS. A molecular switch for targeting between endoplasmic reticulum (ER) and mitochondria: conversion of a mitochondria-targeting element into an ER-targeting signal in DAKAP1. J Biol Chem 2008; 283:11743-51; PMID: 18287098; http://dx.doi.org/10.1074/jbc.M710494200
- Rogne M, Stokka AJ, Tasken K, Collas P, Kuntziger T. Mutually exclusive binding of PP1 and RNA to AKAP149 affects the mitochondrial network. Hum Mol Genet 2009; 18:978-87; PMID: 19074462
- Perrino C, Feliciello A, Schiattarella GG, Esposito G, Guerriero R, Zaccaro L, Del Gatto A, Saviano M, Garbi C, Carangi R, et al. AKAP121 downregulation impairs protective cAMP signals, promotes mitochondrial dysfunction, and increases oxidative stress. Cardiovasc Res 2010; 88:101-10; PMID: 20511238; http://dx.doi.org/10.1093/cvr/cvq155
- Affaitati A, Cardone L, de Cristofaro T, Carlucci A, Ginsberg MD, Varrone S, Gottesman ME, Avvedimento EV, Feliciello A. Essential role of A-kinase anchor protein 121 for cAMP signaling to mitochondria. J Biol Chem 2003; 278:4286-94; PMID: 12427737; http://dx.doi.org/10.1074/jbc.M209941200
- Bui M, Gilady SY, Fitzsimmons RE, Benson MD, Lynes EM, Gesson K, Alto NM, Strack S, Scott JD, Simmen T. Rab32 modulates apoptosis onset and mitochondria-associated membrane (MAM) properties. J Biol Chem 2010; 285:31590-602; PMID: 20670942; http://dx.doi.org/10.1074/jbc.M110.101584
- Schmidt PH, Dransfield DT, Claudio JO, Hawley RG, Trotter KW, Milgram SL, Goldenring JR. AKAP350, a multiply spliced protein kinase A-anchoring protein associated with centrosomes. J Biol Chem 1999; 274:3055-66; PMID: 9915845; http://dx.doi.org/10.1074/jbc.274.5.3055
- Witczak O, Skalhegg BS, Keryer G, Bornens M, Tasken K, Jahnsen T, Orstavik S. Cloning and characterization of a cDNA encoding an A-kinase anchoring protein located in the centrosome, AKAP450. Embo J 1999; 18:1858-68; PMID: 10202149; http://dx.doi.org/10.1093/emboj/18.7.1858
- Takahashi M, Shibata H, Shimakawa M, Miyamoto M, Mukai H, Ono Y. Characterization of a novel giant scaffolding protein, CG-NAP, that anchors multiple signaling enzymes to centrosome and the golgi apparatus. J Biol Chem 1999; 274:17267-74; PMID: 10358086; http://dx.doi.org/10.1074/jbc.274.24.17267
- Shanks RA, Steadman BT, Schmidt PH, Goldenring JR. AKAP350 at the Golgi apparatus. I. Identification of a distinct Golgi apparatus targeting motif in AKAP350. J Biol Chem 2002; 277:40967-72; PMID: 12163481; http://dx.doi.org/10.1074/jbc.M203307200
- Gillingham AK, Munro S. The PACT domain, a conserved centrosomal targeting motif in the coiled-coil proteins AKAP450 and pericentrin. EMBO Rep 2000; 1:524-9; PMID: 11263498; http://dx.doi.org/10.1093/embo-reports/kvd105
- Otera H, Wang C, Cleland MM, Setoguchi K, Yokota S, Youle RJ, Mihara K. Mff is an essential factor for mitochondrial recruitment of Drp1 during mitochondrial fission in mammalian cells. J Cell Biol 2010; 191:1141-58; PMID: 21149567; http://dx.doi.org/10.1083/jcb.201007152
- Otera H, Mihara K. Discovery of the membrane receptor for mitochondrial fission GTPase Drp1. Small GTPases 2011; 2:167-72; PMID: 21776419; http://dx.doi.org/10.4161/sgtp.2.3.16486
- Santel A, Frank S, Gaume B, Herrler M, Youle RJ, Fuller MT. Mitofusin-1 protein is a generally expressed mediator of mitochondrial fusion in mammalian cells. J Cell Sci 2003; 116:2763-74; PMID: 12759376; http://dx.doi.org/10.1242/jcs.00479
- Pich S, Bach D, Briones P, Liesa M, Camps M, Testar X, Palacin M, Zorzano A. The Charcot-Marie-Tooth type 2A gene product, Mfn2, up-regulates fuel oxidation through expression of OXPHOS system. Hum Mol Genet 2005; 14:1405-15; PMID: 15829499; http://dx.doi.org/10.1093/hmg/ddi149
- Gelb BD, Adams V, Jones SN, Griffin LD, MacGregor GR, McCabe ER. Targeting of hexokinase 1 to liver and hepatoma mitochondria. Proc Natl Acad Sci U S A 1992; 89:202-6; PMID: 1309605; http://dx.doi.org/10.1073/pnas.89.1.202
- Sui D, Wilson JE. Structural determinants for the intracellular localization of the isozymes of mammalian hexokinase: intracellular localization of fusion constructs incorporating structural elements from the hexokinase isozymes and the green fluorescent protein. Arch Biochem Biophys 1997; 345:1-11-25; http://dx.doi.org/10.1006/abbi.1997.0241
- Ma Y, Taylor S. A 15-residue bifunctional element in D-AKAP1 is required for both endoplasmic reticulum and mitochondrial targeting. J Biol Chem 2002; 277:27328-36; PMID: 11994283; http://dx.doi.org/10.1074/jbc.M201421200
- Youle RJ, van der Bliek AM. Mitochondrial fission, fusion, and stress. Science 2012; 337:1062-5; PMID: 22936770; http://dx.doi.org/10.1126/science.1219855
- Kolobova E, Efimov A, Kaverina I, Rishi AK, Schrader JW, Ham AJ, Larocca MC, Goldenring JR. Microtubule-dependent association of AKAP350A and CCAR1 with RNA stress granules. Exp Cell Res 2009; 315:542-55; PMID: 19073175; http://dx.doi.org/10.1016/j.yexcr.2008.11.011
- Mason TA, Kolobova E, Liu J, Roland JT, Chiang C, Goldenring JR. Darinaparsin is a multivalent chemotherapeutic which induces incomplete stress response with disruption of microtubules and Shh signaling. PLoS One 2011; 6:e27699; PMID: 22110729; http://dx.doi.org/10.1371/journal.pone.0027699
- Chen H, Detmer SA, Ewald AJ, Griffin EE, Fraser SE, Chan DC. Mitofusins Mfn1 and Mfn2 coordinately regulate mitochondrial fusion and are essential for embryonic development. J Cell Biol 2003; 160:189-200; PMID: 12527753; http://dx.doi.org/10.1083/jcb.200211046
- Joseph AM, Joanisse DR, Baillot RG, Hood DA. Mitochondrial dysregulation in the pathogenesis of diabetes: potential for mitochondrial biogenesis-mediated interventions. Exp Diabetes Res 2012; 2012:642038; PMID: 22203837; http://dx.doi.org/10.1155/2012/642038