Abstract
This study examined the alterations in triglyceride (TG) breakdown and storage in subcutaneous inguinal (SC Ing) and epididymal (Epid) fat depots following chronic endurance training. Male Wistar rats were either kept sedentary (Sed) or subjected to endurance training (Ex) at 70–85% peak VO2 for 6 weeks. At weeks 0, 3, and 6 blood was collected at rest and immediately after a bout of submaximal exercise of similar relative intensity to assess whole-body lipolysis. At week 6, adipocytes were isolated from Epid and SC Ing fat pads for the determination of lipolysis under basal or isoproterenol- and forskolin-stimulated conditions, basal and insulin-stimulated glucose incorporation into lipids, and fatty acid oxidation (FAO). Body weight, fat pad mass, and insulin were reduced by endurance training. Also, circulating non-esterified fatty acids (NEFAs) were 33% lower in Ex than Sed rats when exercising at the same relative intensity. This coincided with reduced isoproterenol-stimulated lipolysis in the Epid (27%) and SC Ing (25%) adipocytes in Ex rats. Similarly, forskolin-stimulated lipolysis was reduced in Epid (51%) and SC Ing (49%) adipocytes from Ex rats. Insulin-stimulated glucose incorporation into lipids in adipocytes from both fat depots from Ex rats was also lower (∼43%) than Sed controls. Conversely, FAO was increased in Epid (1.71-fold) and SC Ing (1.82-fold) adipocytes of Ex rats. In conclusion, chronic endurance exercise reduced lipolysis and lipogenesis while increasing FAO in Epid and SC Ing adipocytes. These are compatible with an energy-sparing adaptive response to reduced adiposity under chronic endurance training conditions.
Introduction
The white adipose tissue (WAT) allows the organism to meet daily oscillations in energy demand and helps to regulate whole-body energy homeostasis. It does so by constantly adjusting its metabolism according to the various conditions that can potentially alter adiposity such as exercise. Several human studies have reported that circulating NEFAs during submaximal exercise of the same relative intensity are reduced after a period of endurance training.Citation1-4 Importantly, analyses of NEFA rate of appearance (Ra)Citation4-6 during exercise revealed that this variable was significantly reduced as a result of training, which is also in support of a reduction in WAT lipolysis with endurance training. However, at odds with these findings are the results of studies measuring catecholamine-stimulated glycerol release in isolated adipocytes from humansCitation7-11 and rats,Citation12-15 which report increased WAT lipolysis after a period of endurance training. Additional studies have reported lipolysis to be either reduced in isolated rat adipocytesCitation16 or unaltered when assessed in situ by the microdialysis technique in human WATCitation17 following a period of endurance training.
It is often difficult to reconcile apparent discrepant findings regarding the effects of chronic endurance training on WAT lipolysis when large methodological variability exists among studies. In vivo studies essentially assess whole-body lipolysis without discriminating the origin of fatty acids and glycerol measured in the circulation. Considering that IMTG has been estimated to provide more than 50% of the fat utilized during exerciseCitation4 and that skeletal muscle lipolysis is required for this to occur, lipolysis defined as 3× glycerol Ra in the circulationCitation18 does not necessarily reflect TG breakdown that actually takes place in the WAT. Additionally, alterations in WAT blood flow and catecholamine delivery,Citation17 adiposity,Citation6 and insulin sensitivityCitation19 could confound the in vivo adaptive lipolytic responses of the WAT to chronic endurance training. Lastly, fasting can alter the hormonal milieu in a way that is more conducive to lipolysis (e.g. elevated catecholamine and reduced insulinemia).Citation6 Thus, studies in which lipolysis was assessed under fasting conditionsCitation20,21 could, to some extent, reflect these effects.
Studies in isolated adipocytes avoid most of these in vivo confounders, but have limitations of their own that can make comparisons and interpretation of lipolysis data challenging. A critical issue when comparing lipolysis in adipocytes isolated from sedentary and endurance-trained conditions is the method used for normalization of data. Alterations in adiposity induced by chronic endurance training and/or diet lead to alterations in fat cell volume and number within each preparation, which could greatly affect the estimation of lipolysis under basal and catecholamine-stimulated conditions. In fact, there are studies in which the volume of adipocytes in endurance-trained subjects is significantly lower than sedentary controls and lipolysis is expressed per amount of lipid in each incubation.Citation8,9 In such cases, lipolysis values in the endurance trained condition could derive from a much higher number of fat cells than that of control sedentary adipocytes, making it difficult to establish a clear direct comparison between the conditions. Lipolysis between sedentary and endurance-trained conditions has at times been compared by incubating pieces of rat WAT instead of isolated cells,Citation12 which does not take into account differences in cell content in each preparation either, despite major differences in fat mass between the 2 groups.Citation12
In this context, the aim of this study was twofold: (a) to investigate the effects of endurance training on WAT lipolysis by assessing this variable in a time-dependent manner during submaximal exercise of same relative intensity in rats, and (b) to assess basal and stimulated lipolysis in adipocytes isolated from visceral (VC) and subcutaneous (SC) fat depots after a period of endurance training. The combination of in vivo and in vitro approaches to assess lipolysis within the same group of animals had not been previously done and is crucial to reconcile in vivo and in vitro lipolysis data. The number of fat cells in each preparation was equalized not only to determine how chronic endurance training regulates WAT lipolysis, but also to test if SC and VC fat depots would respond differently to chronic endurance training. The mass of VC and SC fat depots was measured and samples of these tissues were used for microscopy analysis and determination of adipocyte size. Finally, since lipogenesis and oxidative capacity of adipocytes affect adiposity and the release and storage of fatty acids within the WAT, glucose incorporation into lipids and palmitate oxidation were also measured in VC and SC adipocytes from sedentary and endurance-trained rats. Here, we provide a detailed analysis of the effects of chronic endurance training on release and storage of fatty acids by the WAT.
Results
Effects of chronic endurance training on peak VO2 and resting corticosterone
Peak VO2 was 19% greater in exercise than sedentary animals after 6 weeks of treadmill running (3603 ± 189.90 ml/kg/hr vs. 4289 ± 106.20 ml/kg/hr for sedentary and exercised animals, respectively). To further characterize the training model, plasma corticosterone concentrations were assessed in samples obtained under resting conditions to determine whether rats exposed to treadmill running had higher stress levels compared to sedentary counterparts. These measurements revealed that plasma corticosterone did not differ between sedentary and endurance-trained rats at weeks 0, 3, and 6 (). In fact, we found that plasma concentrations of corticosterone markedly dropped at weeks 3 and 6, reaching values equivalent to ∼10% of week 0 values (). These values were similar to what has been reported for male rats under resting conditions during the morning hours.Citation22 This suggests that the endurance training program used in this study did not induce chronically elevated stress levels in the animals.
Table 1. Effects of chronic endurance training on blood parameters of sedentary (Sed) and endurance-trained (Ex) rats in the fed state under resting conditions
Effects of chronic endurance training on body mass, food intake, and adiposity
By the end of the 6 week endurance-training period, body mass (average ± SEM) was 6.1% lower in Ex (422.55 g ± 5.17) than Sed (450.15 g ± 6.05) rats. Energy intake did not differ between the Sed and Ex rats throughout the study with values corresponding to 92.62 ± 2.56 and 91.02 ± 2.37 kcal/rat/day (n = 8) at week 6. Analysis of fat mass revealed that the Epid () and SC Ing fat () depots were 30% and 21% smaller in trained animals than sedentary controls, respectively. Similarly, the average adipocyte area of Ex rats was also reduced in the Epid fat depot by 40% () and in the SC Ing fat depot by 25% () when compared to sedentary animals. These findings demonstrate that the endurance training program was effective in causing a fat-reducing effect.
Figure 1. Chronic endurance training reduces visceral and subcutaneous adiposity. The mass of the Epid (epididymal) (A) and SC Ing (subcutaneous inguinal) (B) fat depots of sedentary (Sed) and endurance-trained (Ex) rats was measured at the end of the study (week 6). Samples of each tissue were also used for microscopy analysis and quantification of epididymal (C) and inguinal (D) adipocyte area. *P < 0.05 vs. Sed. t-test, n = 8.
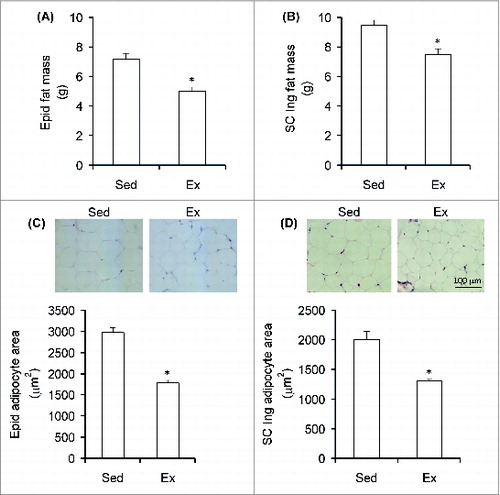
Effects of chronic endurance training on circulating NEFAs and glycerol during sub-maximal exercise
Rats assigned to the Sed and Ex groups did not differ in submaximal running speed at baseline (week 0, ). However, Ex animals ran at 1.3- and 1.4-fold higher speeds than Sed animals at weeks 3 and 6, respectively (). To confirm that the intensity was indeed similar between groups, plasma lactate was measured and no significant differences were found between Sed and Ex animals at weeks 0, 3, and 6 of the study (). In agreement with this finding, neither plasma epinephrine () nor norepinephrine () differed between Sed and Ex rats at weeks 0, 3, and 6 of the study. At week 0, circulating NEFAs were similar between rats in the Sed and Ex groups when exercising at the same relative exercise intensity (). However, at weeks 3 and 6 of the study, circulating NEFA levels in Ex rats were significantly reduced by 38% and 34%, respectively (). Similarly, baseline (week 0) circulating glycerol did not differ between Sed and Ex rats (), but was decreased in Ex rats by 22% and 33% after 3 and 6 weeks of endurance training, respectively, when compared to Sed rats (). At week 0, fed-state glycemia was also the same for both groups of rats, although a divergent pattern of progressive elevation and reduction was found for Sed and Ex rats, respectively, at weeks 3 and 6 ().
Effects of chronic endurance training on lipolysis in isolated adipocytes
Our in vivo data suggested that endurance training caused a reduction in adipose tissue lipolysis. To provide further evidence of this endurance training effect, an in vitro model was used to directly assess basal and catecholamine-stimulated lipolysis in adipocytes. Under basal conditions, the rate of glycerol release in Epid and SC Ing adipocytes did not differ between Sed and Ex rats (). Isoproterenol (10 μM) significantly increased the release of glycerol by Epid and SC Ing adipocytes isolated from both groups of rats, however this variable was found to be reduced by 27% and by 25% in Epid and SC Ing adipocytes from Ex when compared to Sed rats, respectively (). Similarly, forskolin-stimulated glycerol release was reduced by 51% in Epid adipocytes and by 49% in SC Ing adipocytes of Ex rats when compared to sedentary controls (). These in vitro findings support the reduction in circulating NEFAs and glycerol found in vivo in rats exposed to chronic endurance training.
Figure 4. Chronic endurance training reduces isoproterenol (Iso)- and forskolin (Forsk)-stimulated lipolysis. Glycerol release was measured in epididymal (A and B) and subcutaneous inguinal (C and D) under basal conditions and also in the absence or presence of Iso or Forsk. *P < 0.05 vs. Sed. Two-way ANOVA, n = 8 per group.
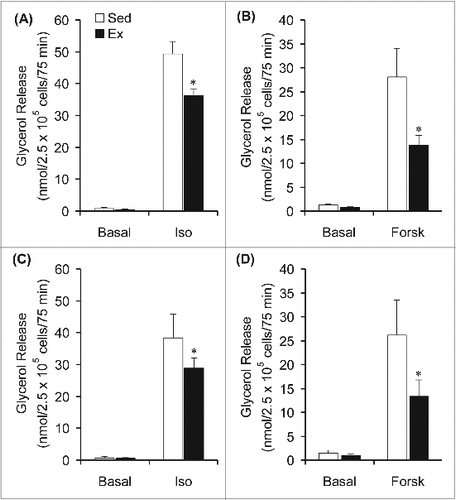
Glucose incorporation into lipids and fatty acid oxidation in Epid and SC Ing isolated adipocytes
Since there was significantly less adiposity in Ex than Sed rats, despite a reduction in WAT lipolysis found in the former, we assessed lipogenesis and fatty acid oxidation to explore potential mechanisms for the decrease in fat mass in Ex rats. Interestingly, after 6 weeks of endurance training the incorporation of glucose into lipids in Epid adipocytes was reduced by 39% and by 44% under basal- and insulin-stimulated conditions, respectively (). In SC Ing adipocytes, the basal incorporation of glucose into lipids was not affected by endurance training; however, under insulin-stimulated conditions this variable was reduced by 42% in the Ex when compared to Sed rats (). Also, in Epid and SC Ing isolated adipocytes from Ex rats, palmitate oxidation was increased by 1.71- and by 1.82-fold, respectively, when compared to Sed rats ().
Figure 5. Chronic endurance training reduces lipogenesis and increases fatty acid oxidation in epididymal (Epid) and subcutaneous inguinal (Ing) adipocytes. The incorporation of glucose into lipids was assessed in Epid (A) and Ing (B) adipocytes extracted from sedentary (Sed) or endurance-trained (Ex) rats after incubation of the cells for 1h either in the absence (Basal) or presence of insulin (Ins, 100 nm). Palmitate oxidation (C) was measured during 1h incubation of isolated adipocytes from Sed and Ex rats. *P < 0.05 vs. Sed. #P < 0.05 vs. Sed Basal and Ex Ins. Two-way ANOVA, n = 8.
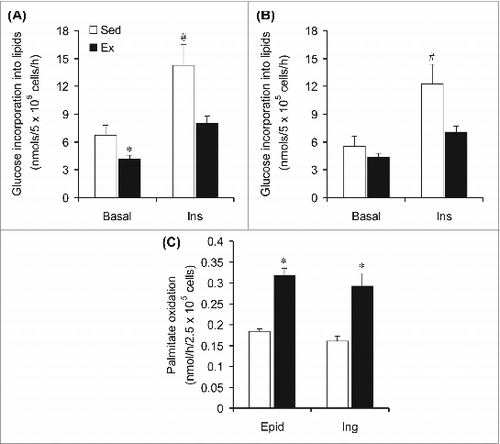
Discussion
One of the main findings of this study was that chronic endurance training caused an adaptive response that reduced lipolysis in both Epid and SC Ing adipocytes, an effect that was also accompanied by a reduction in circulating NEFAs and glycerol under submaximal exercise conditions. Even though Epid adipocytes had a higher lipolytic response than the SC Ing adipocytes in response to isoproterenol and forskolin, endurance training caused a similar reduction in the lipolytic response to these agents in both fat depots. Furthermore, while the incorporation of glucose into lipids under basal conditions was reduced, palmitate oxidation was markedly increased by endurance training in Epid and SC Ing adipocytes. A higher rate of lipid oxidation would help maintain intracellular energy levels while leaving fewer fatty acids available for re-esterification within the cell. Re-esterification in the WAT has been reported to be directly proportional to the rate of lipolysisCitation24,25 and its precise control is considered to play an important role in enabling a rapid response of fatty acid metabolism to major changes in energy demand.Citation26 However, the energy required for acylation has been reported to be the largest single drain of ATP in adipocytes stimulated by lipolytic hormones.Citation27 Thus, it would be expected that under conditions of chronic exposure to increased TG breakdown the organism would elicit an adaptive response that modulates the lipolytic response to more effectively match NEFA release with its peripheral utilization. This would serve to attenuate the high energy cost of re-esterification while still allowing for rapid adjustments to changes in energy demand to occur.
Fat mass reduction is a potential mechanism by which chronic endurance training could lead to downregulation of lipolysis in the WAT. In fact, previous studies have demonstrated that basal and stimulated hormone sensitive lipase (HSL) activity showed high direct correlation with fat cell size in rats.Citation28 This is also compatible with other human studies reporting reduced fatty acid RaCitation6 and WAT HSL contentCitation29 in subjects undergoing weight loss. Interestingly, weight loss with diet alone or in combination with exercise caused similar reductions in fatty acid Ra,6 indicating that fat loss plays a major role in the downregulation of fatty acid mobilization in the WAT. In this study, Epid and SC Ing fat masses and adipocyte area were indeed significantly lower in Ex than Sed rats. Conversely, previous studies in isolated adipocytes from ratsCitation30 and humansCitation11 reporting an increase in lipolysis in response to chronic endurance training did not show any significant alteration in body weight or adiposity with endurance training, which could be the reason for the apparent discrepant findings from this and other studies in isolated rat adipocytesCitation16 in which adiposity is reduced with chronic endurance training.
Another important aspect directly associated with exercise-induced fat loss and altered lipolysis is insulin sensitivity. Circulating NEFAs have been reported to be lower in endurance-trained than sedentary rats during an euglycemic hyperinsulinemic clamp.Citation19 In humans, plasma glycerol was suppressed to a greater extent by insulin after 10 days of endurance training compared to the pre-training condition,Citation31 and interstitial glycerol concentration in the periumbilical subcutaneous adipose tissue has been reported to decrease faster in trained than in sedentary subjects during a hyperinsulinemic euglycemic clamp.Citation32 These findings indicate that the antilipolytic action of insulin is enhanced by endurance training. In this study, we measured plasma insulin under resting conditions and found it to be significantly lower in Ex than Sed rats after 3 (35%) and 6 (28%) weeks of endurance training, although no differences in plasma glucose were found between the 2 groups. This indicates that insulin sensitivity is higher in Ex than Sed rats. We also measured glycemia during submaximal exercise of similar relative intensity and found it to be significantly lower in Ex than Sed rats after 3 and 6 weeks (14% and 18%, respectively) of endurance training, which is again consistent with higher insulin sensitivity and glucose utilization in Ex rats than Sed rats. The fact that the rats were exercised in the fed state must also have contributed to this effect, since the levels of circulating insulin were much higher in the fed (2.11 ± 0.21 ng/ml) than in the fasted (1.09 ± 0.15 ng/ml) state. Therefore, reduced circulating NEFAs and glycerol during submaximal exercise of the same relative intensity in Ex rats could be because of a higher antilipolytic response to insulin in rats in which the sensitivity to this hormone was already increased by chronic endurance training. Fed animals were also used for adipocyte isolation and a similar enhanced antilipolytic response due to exercise-induced increased insulin sensitivity could have contributed to lower lipolytic rates in Ex than Sed rats.
We used isoproterenol and forskolin to stimulate lipolysis in adipocytes isolated from Epid and Sc Ing fat depots and found that the lipolytic effect of both agents was attenuated by endurance training. The main difference between isoproterenol and forskolin is that while the former exerts its effects through activation of β-adrenergic receptors, the latter induces lipolysis by directly activating adenylyl cyclase. Shepherd et al.Citation33 have reported that adenylyl cyclase activity in response to norepinephrine was lower in trained than untrained rat fat cell ghosts, which is in line with the findings of reduced norepinephrine-stimulated cAMP accumulationCitation34,35 and increased ratio of activity of phosphodiesterase relative to adenyl cyclase in adipocytes of endurance trained rats.Citation34 These could at least partially explain our findings of lower circulating NEFA and glycerol levels in Ex rats than Sed counterparts under submaximal exercise of same relative intensity, as well as the reduced lipolysis in Epid and SC Ing isolated adipocytes from Ex rats. Increased insulin action could again lead to this effect, since stimulation of the insulin signaling pathway increases the activity of phosphodiesterase 3B (PDE3B) which inactivates cAMP by converting it to 5′AMP. This leads to a reduction in protein kinase A activity and suppression of lipolysis.Citation36 Because inactivation of cAMP by PDE3B is an event that takes place downstream of both β-adrenergic receptors and adenylyl cyclase activation, improved insulin action could justify the endurance training-induced impairment of lipolysis under both isoproterenol and forskolin stimulation. However, the breakdown of TG is regulated by a complex orchestration of numerous enzymes and proteins in adipocytes. As such, there are many levels of regulation in the lipolytic cascade that could potentially mediate the observed training induced attenuation of glycerol release in the present study. In this context, adipose triglyceride lipase (ATGL) and HSL (the major TG and DAG lipases, respectively) along with their associated regulatory proteins perilipin and comparative gene identification-58 (CGI-58) could also have been affected by endurance training. Additional studies are required to explore these possibilities.
It is interesting to note that the insulin-stimulated increase in glucose incorporation into lipids was impaired with endurance training. We originally thought that this would increase given the fact that insulin sensitivity is improved with endurance training. However, it is possible that the training-induced downregulation of lipolysis and increased oxidative capacity in the WAT reduced the availability of NEFAs for esterification within the adipocytes, and contributed to the reduced use of glucose for the formation of the glycerol moiety by these cells. This is consistent with previous observations that moderate exercise increased intra-adipocyte free fatty acid levels under basal and epinephrine-stimulated conditions.Citation30 This is particularly relevant under conditions of chronic endurance training in which an increased pool of intracellular NEFAs can be quickly mobilized to supply energy to peripheral tissues without requiring extensive WAT lipolysis. Such an adaptive response is also compatible with the observations that endurance-trained skeletal muscles rely more on their IMTG pool than on circulating NEFAs for energy production.Citation3-5,Citation18 Furthermore, reduced glucose metabolism in the WAT may reflect a lower uptake and storage of fuel in adipose tissue, which may facilitate glucose uptake and metabolism in other peripheral tissues such as skeletal muscles and liver. In fact, glycogen storage has been demonstrated to be enhanced in skeletal muscles and liver of endurance trained rats.Citation37,38 Lastly, in the present study food intake did not differ in both groups of animals, although adiposity was significantly reduced in Ex when compared to Sed rats. Thus, it is clear that Ex rats were in a negative energy balance condition, which must have limited lipogenesis in the WAT and led to a reduction in the incorporation of glucose into lipids in visceral and subcutaneous adipocytes.
In summary, this study assessed the time-dependent alterations in whole-body lipolysis following sub-maximal exercise of the same relative intensity, as well as the lipolytic response of VC and SC fat depots under sedentary and endurance-trained conditions. Both in vivo and in vitro analyses were carried out within the same group of animals, which had not been previously done. We demonstrated that after 6 weeks of chronic endurance exercise, rats displayed well established training-induced adaptations including enhanced VO2 peak, reduced body weight, and decreased adiposity. Our findings provide evidence that circulating NEFAs and glycerol during submaximal exercise of same relative intensity and lipolysis in VC and SC isolated adipocytes are reduced in rats exposed to chronic endurance training. These findings are in support of our original hypothesis that the organism elicits an adaptive response to chronic endurance training that modulates the lipolytic response to more effectively match NEFA release with its peripheral rate of utilization.
Materials and Methods
Reagents
Type II Collagenase (Cat # C6885), isoproterenol (Cat # I2760), forskolin (Cat # F3917), FA-free bovine serum albumin (BSA, Cat # A3803), palmitic acid (Cat # P0500), and free glycerol determination kit (Cat # FG0100) were obtained from Sigma. [1–14C] palmitic acid (Cat # NEC075H050UC) and D-[U14C] glucose (Cat # NEC042V250UC) were from GE Healthcare Radiochemicals. The non-esterified fatty acid (NEFA) kit was from Wako (Wako HR Series NEFA-HR, Cat #s 999–34691, 991–34891, 995–34791, 993–35191). The kit for epinephrine and norepinephrine determination (2-CAT ELISA, Cat # BA E-4500) was from Rocky Mountain Diagnostics, Inc. The lactate kit was from Trinity Biotech (Cat # 735–10). The rat corticosterone kit (Rat CorticosteroneCitation3H Kit) was from MP Biomedicals (Cat # 07120002).
Animals
Male albino rats (Wistar strain) 40–45 d of age (weighing approximately 150g upon commencement of the endurance training protocol) were housed at 22°C on a 12/12-hr light/dark cycle and fed standard laboratory chow (Lab Diet Cat # 5012) ad libitum throughout the entire study. Food intake and body weight were measured on a daily basis and always in the morning (between 09:00 and 10:00 am) throughout the study. The protocol containing all animal procedures described in this study was specifically approved by the Committee on the Ethics of Animal Experiments of York University (York University Animal Care Committee, YUACC, permit number: 2011–14) and performed strictly in accordance with the YUACC guidelines. All surgery was performed under Ketamine/Xylazine anesthesia, and all efforts were made to minimize suffering.
Acclimatization, selection and exercise training
Upon arrival, animals were permitted to acclimatize for one week followed by a one week selection process in which animals unwilling to run were excluded from the study. For selection, animals performed a graded treadmill test for 4 consecutive days. The test consisted of a 5 min warm up at a speed of 10 m/min with 0% inclination followed by a 2 min interval at 14 m/min with 5% inclination. The treadmill was then set at 10% inclination and the speed was increased by 2 m/min every 2 min to a maximum speed of 30 m/min. Inclusion into the study was based on the demonstration of willingness to run continuously beyond a minimum speed of 20 m/min all 4 days of the selection period. This was done to ensure that all animals were capable of completing the endurance exercise training protocol. Only ∼10% of the animals did not meet the inclusion criteria. The selected animals were then randomly divided into sedentary and exercise groups. The rats were endurance-trained by running on treadmills (AccuScan Instruments, Inc., Columbus, OH, USA).
Peak oxygen consumption (peak VO2) determination and training protocol
In order to set the initial training intensity and to adjust it as the animals improved their running ability, peak VO2 tests were conducted at weeks 0, 2, 4, and 6 of the study. Specially designed treadmills connected to the Comprehensive Laboratory Animal Monitoring System (CLAMS) from Columbus Instruments were used to apply an exercise protocol of incremental workloads to determine peak VO2 in rats. To accomplish this, all rats were placed on the treadmill and VO2 was continuously monitored under resting (after 15–20 min of rats being placed in the treadmill chamber) and during the entire exercise-test period. After recording resting VO2 values, the rats were exposed to a 5 min warm-up-period (10 m/min, 0% inclination). Subsequently, inclination was increased to 5% (stage 1) and to 10% (stage 2) and then maintained constant thereafter, while treadmill speed was progressively increased (2 m/min every 2 min) until peak VO2 was reached. Peak VO2 was characterized by the point at which increments in speed were not accompanied by increases in VO2 or the point at which the animals could no longer run continuously (characterized by the rats remaining on the shocking grid for at least 5 consecutive seconds). Rats in the endurance training group were exposed to treadmill running at 70 – 85% of peak VO2, 1h/day, 5 days/week for 6 weeks. In order to assure equal conditions between the sedentary and endurance-trained groups, all rats were placed on the treadmill simultaneously. The treadmill speed for sedentary animals was kept at 1–2 m/min and 0% inclination during the entire duration (1h) of the training session. Treadmill speed was adjusted every 2 weeks to maintain the exercise intensity between 70 – 85% of peak VO2 throughout the study. For weeks 1 and 2, the training sessions started with a warm-up period (3 min at 12 m/min, 0% inclination followed by 2 min at 14 m/min, 5% inclination). Treadmill inclination was then increased to 10% and maintained constant, while the speed was progressively increased in a manner that by the 20th min all animals had reached the 70–85% peak VO2 training-range, and were then exercised at that intensity during the remaining 40 min. The animals quickly adapted to the training protocol, so for weeks 3 to 6 the warm-up lasted only 2 min and started at 24 m/min, 10% inclination with the speed being progressively increased every 2 min in a manner that the training range was reached within 10 min of the start, and then maintained for the remaining 50 min of each training session. The average treadmill speed required to maintain the training intensity also increased from 24 m/min (week 1) to 32 m/min (week 3), and to 38 m/min at week 6. The intensity, frequency, and volume of exercise chosen here have been previously demonstrated to significantly increase peak VO2 in rats.Citation39 This training protocol is also compatible with exercise prescriptions used in humans to improve cardiovascular fitness.Citation40
Determination of circulating glycerol and NEFAs under submaximal exercise
Because the hormonal response to exercise has been shown to be determined by relative rather than absolute exercise intensity,Citation41 exercise in the present study was performed at the same relative exercise intensity as demonstrated by circulating catecholamines (epinephrine and norepinephrine) and lactate. Therefore, the endurance training-induced alterations in lipolysis were not associated with different plasma catecholamine concentrations. In order to assess the effects of chronic endurance training on circulating NEFAs and glycerol, Sed and Ex rats were exposed to a single bout of sub-maximal exercise of similar relative intensities at weeks 0, 3, and 6 of the study. Exercise intensity was determined by calculating the speed corresponding to 75–80% of their peak VO2 in a graded test (described above) carried out prior to the beginning of the study (week 0), and also at weeks 3 and 6 of the training protocol. For blood collection after a sub-maximal exercise bout, all animals warmed up for 5 minutes with a progressively increasing speed that reached 75–80% of their Peak VO2 by the end of the 5th minute with the treadmill inclination set at 10%. This pace was then maintained for additional 30 min and blood was collected immediately upon completion of the protocol. The submaximal exercise test was always conducted at the same time of the day (10:00 am) with all animals in the fed state. After collection, blood samples were centrifuged for 5 min at 4°C and plasma stored at -80°C for subsequent analysis of glycerol and NEFAs. To confirm that the intensity of exercise was similar between the Sed and the Ex rats, blood was also used to measure epinephrine, norepinephrine, and lactate concentrations.
Adipocyte isolation
In order to avoid the acute effect of exercise and assess the chronic effects of endurance training on adipose tissue metabolism, the animals were anesthetized (0.4 mg ketamine and 8 mg xylazine per 100 g body weight) in the fed state 48 – 72h after the last training session. Subcutaneous (SC) inguinal (Ing) and epididymal (Epid) fat pads were then extracted and weighed. A sample of each fat pad was taken for microscopy to assess adipocyte area and the remaining tissue was used for adipocyte isolation as described previously.Citation42 Briefly, the adipose tissue was finely minced in Krebs-Ringer Buffer (0.154M NaCl, 0.154M KCl, 0.11M CaCl2, 0.154M MgSO4, 0.154M KH2PO4, 0.154M NaHCO3, pH 7.4) with 5.5 mM glucose and 30 mM HEPES (KRBH) supplemented with type II collagenase (0.5 mg/ml). The finely minced tissues were then incubated at 37°C with gentle agitation (120 orbital strokes/min) for approximately 25–30 min. Digested tissue was strained using a nylon mesh (pore size 500 μm) and cells were transferred to 50 ml tubes to be carefully washed 3 times and resuspended in KRBH containing 3.5% BSA (KRBH-3.5% BSA). To distribute an equal number of adipocytes in each treatment condition, cell diameters and numbers were measured as described by DiGirolamo and Fine.Citation43
Determination of adipocyte lipolysis
Lipolysis was measured by incubating adipocytes (2.5 × 105 cells) either in the absence or presence of isoproterenol (10 μM), or forskolin (10 μM). Triplicates for each condition were used and the isolated adipocytes were incubated for 75 min at 37°C with gentle agitation (80 orbital strokes/min). These agents were utilized in order to stimulate lipolysis by slightly distinct mechanisms. Isoproterenol elicits the highest lipolytic response by acting as a typical, non-specific β-adrenergic agonistCitation44 while forskolin induces lipolysis bypassing β-adrenergic receptors cyclase activity and increasing discyclase activity and increase intracellular levels of cyclic AMP (cAMP).Citation45,46 After incubation, a 200 μl aliquot of media was taken for the determination of glycerol concentration.
Incorporation of [U–14C]D-glucose into total lipids
As an indication of lipogenesis, glucose incorporation into TG was determined by incubating 0.5 ml cell suspension (2.5×105 isolated adipocytes) in 0.5 ml KRBH-3.5% BSA containing D–[U–14C] glucose (0.5 Ci/ml) either in the absence or presence of insulin (100 nM) for 1 hour at 37°C.Citation47 Cells were subsequently lysed by the addition of 62.5 μl of H2SO4 (5N). Lipids were then extracted using Dole's Reagent (40:10:1 isopropylol:heptane: H2SO4 1N) to determine radioactivity in the total lipid fraction as previously described.Citation48
Tissue fixation and determination of adipocyte area
Since fat mass was significantly reduced by endurance training, we also assessed adipocyte area in Epid and Sc Ing fat depots of both groups of animals. Morphological analysis was performed using light microscopy as previously describedCitation42 with a few modifications. Briefly, upon extraction of the fat pads, small samples (∼50–100 mg) of the SC Ing and Epid white adipose tissues were removed. All fat samples were fixed in 4% paraformaldehyde, 0.1 M phosphate buffer solution (pH 7.4) for 24 hours at room temperature. After fixation, tissue samples were washed 3 times and stored in 70% ethanol. Samples were subsequently sent to the Toronto Center for Phenogenomics (Toronto, ON, CA) where they were embedded in paraffin blocks, sectioned and processed for hemotoxylin and eosin staining. Stained samples were viewed using a Nikon Eclipse TiE inverted microscope (Nikon Canada, Mississauga, ON, CA) under 20× magnification. Average adipocyte area was determined by measuring the area of 150 cells in 3 randomly selected fields of view for each animal. This was done by 2 independent researchers to prevent biased selection of cells for measurement. The results of the 2 independent determinations differed by ∼2%. Area was determined by NIS-elements basic research imaging software (Nikon Canada, Mississauga, ON, CA) and images were captured with a digital Nikon DS-QI1Mc camera (Nikon Canada, Mississauga, ON, CA).
Statistical analysis
Statistical significance was assessed by 2-way analysis of variance (ANOVA) with Bonferroni post-hoc test or by unpaired t-test as indicated. Statistical significance was set at P < 0.05.
Disclosure of Potential Conflicts of Interest
No potential conflicts of interest were disclosed.
Authors Contributions
KEP, DMSK, and SH performed experiments. DMSK analyzed data, prepared figures, and edited the manuscript. RBC designed the study and wrote the manuscript.
Funding
This research was funded by a Discovery Grant from the Natural Science and Engineering Research Council of Canada (NSERC) and by infrastructure grants from the Canada Foundation for Innovation (CFI) and the Ontario Research Fund (ORF) awarded to RBC. DMSK was supported by the Elia Scholarship and the NSERC Alexander Graham Bell Canada Graduate Doctoral Scholarship.
References
- Winder WW, Hickson RC, Hagberg JM, Ehsani AA, McLane JA. Training-induced changes in hormonal and metabolic responses to submaximal exercise. J Appl Physiol 1979; 46:766-71; PMID:457555
- Koivisto V, Hendler R, Nadel E, Felig P. Influence of physical training on the fuel-hormone response to prolonged low intensity exercise. Metabolism 1982; 31:192-7; PMID:7043170; http://dx.doi.org/10.1016/0026-0495(82)90135-4
- Hurley BF, Nemeth PM, Martin WH, Hagberg JM, Dalsky GP, Holloszy JO. Muscle triglyceride utilization during exercise: effect of training. J Appl Physiol 1986; 60:562-7; PMID:3512511
- Martin WH, Dalsky GP, Hurley BF, Matthews DE, Bier DM, Hagberg JM, Rogers MA, King DS, Holloszy JO. Effect of endurance training on plasma free fatty acid turnover and oxidation during exercise. Am J Physiol 1993; 265:E708-14; PMID:8238496
- Phillips SM, Green HJ, Tarnopolsky MA, Heigenhauser GF, Hill RE, Grant SM. Effects of training duration on substrate turnover and oxidation during exercise. J Appl Physiol 1996; 81:2182-91; PMID:9053394
- Schenk S, Harber MP, Shrivastava CR, Burant CF, Horowitz JF. Improved insulin sensitivity after weight loss and exercise training is mediated by a reduction in plasma fatty acid mobilization, not enhanced oxidative capacity. J Physiol 2009; 587:4949-61; PMID:19723783; http://dx.doi.org/10.1113/jphysiol.2009.175489
- Crampes F, Beauville M, Riviere D, Garrigues M. Effect of physical training in humans on the response of isolated fat cells to epinephrine. J Appl Physiol 1986; 61:25-9; PMID:3733612
- Riviere D, Crampes F, Beauville M, Garrigues M. Lipolytic response of fat cells to catecholamines in sedentary and exercise-trained women. J Appl Physiol 1989; 66:330-5; PMID:2537283
- Crampes F, Riviere D, Beauville M, Marceron M, Garrigues M. Lipolytic response of adipocytes to epinephrine in sedentary and exercise-trained subjects: sex-related differences. Eur J Appl Physiol Occup Physiol 1989; 59:249-55; PMID:2583171; http://dx.doi.org/10.1007/BF02388324
- De Glisezinski I, Crampes F, Harant I, Berlan M, Hejnova J, Langin D, Rivière D, Stich V. Endurance training changes in lipolytic responsiveness of obese adipose tissue. Am J Physiol 1998; 275:E951-6; PMID:9843736
- Després JP, Bouchard C, Savard R, Tremblay A, Marcotte M, Thériault G. The effect of a 20-week endurance training program on adipose-tissue morphology and lipolysis in men and women. Metabolism 1984; 33:235-9; PMID:6694563; http://dx.doi.org/10.1016/0026-0495(84)90043-X
- Parizkova J, Stankova L. Influence of physical activity on a treadmill on the metabolism of adipose tissue in rats. Br J Nutr 1964; 18:325-32; PMID:14188292; http://dx.doi.org/10.1079/BJN19640031
- Askew EW, Huston RL, Plopper CG, Hecker AL. Adipose tissue cellularity and lipolysis. Response to exercise and cortisol treatment. J Clin Invest 1975; 56:521-9; PMID:1159071; http://dx.doi.org/10.1172/JCI108120
- Ogasawara J, Sakurai T, Kizaki T, Ishibashi Y, Izawa T, Sumitani Y, Ishida H, Radak Z, Haga S, Ohno H. Higher levels of ATGL are associated with exercise-induced enhancement of lipolysis in rat epididymal adipocytes. PLoS One 2012; 7:e40876; PMID:22815850; http://dx.doi.org/10.1371/journal.pone.0040876
- Suda K, Izawa T, Komabayashi T, Tsuboi M, Era S. Effect of insulin on adipocyte lipolysis in exercise-trained rats. J Appl Physiol 1993; 74:2935-9; PMID:8103512
- Chapados N, Collin P, Imbeault P, Corriveau P, Lavoie J-M. Exercise training decreases in vitro stimulated lipolysis in a visceral (mesenteric) but not in the retroperitoneal fat depot of high-fat-fed rats. Br J Nutr 2008; 100:518-25; PMID:18284712; http://dx.doi.org/10.1017/S0007114508921735
- Stallknecht B, Simonsen L, Bülow J, Vinten J, Galbo H. Effect of training on epinephrine-stimulated lipolysis determined by microdialysis in human adipose tissue. Am J Physiol 1995; 269:E1059-66; PMID:8572197
- Horowitz JF, Klein S. Lipid metabolism during endurance exercise. Am J Clin Nutr 2000; 72:558S-63S; PMID:10919960
- Lavoie JM, Bongbélé J, Cardin S, Bélisle M, Terrettaz J, Van de Werve G. Increased insulin suppression of plasma free fatty acid concentration in exercise-trained rats. J Appl Physiol 1993; 74:293-6; PMID:8444706
- Romijn JA, Klein S, Coyle EF, Sidossis LS, Wolfe RR. Strenuous endurance training increases lipolysis and triglyceride-fatty acid cycling at rest. J Appl Physiol 1993; 75:108-13; PMID:8376256
- Guezennec CY, Serrurier B, Aymonod M, Merino D, Pesquies PC. Metabolic and hormonal response to short term fasting after endurance training in the rat. Horm Metab Res 1984; 16:572-5; PMID:6392054; http://dx.doi.org/10.1055/s-2007-1014854
- Atkinson HC, Waddell BJ. Circadian variation in basal plasma corticosterone and adrenocorticotropin in the rat: sexual dimorphism and changes across the estrous cycle. Endocrinology 1997; 138:3842-8; PMID:9275073
- Atkinson HC, Waddell BJ. Circadian variation in basal plasma corticosterone and adrenocorticotropin in the rat: sexual dimorphism and changes across the estrous cycle. Endocrinology 1997; 138:3842-8; PMID:9275073
- Brooks B, Arch JR, Newsholme EA. Effects of hormones on the rate of the triacylglycerol/fatty acid substrate cycle in adipocytes and epididymal fat pads. FEBS Lett 1982; 146:327-30; PMID:6128259; http://dx.doi.org/10.1016/0014-5793(82)80945-9
- Vaughan M. The production and release of glycerol by adipose tissue incubated in vitro. J Biol Chem 1962; 237:3354-8; PMID:13996476
- Wolfe RR, Klein S, Carraro F, Weber JM. Role of triglyceride-fatty acid cycle in controlling fat metabolism in humans during and after exercise. Am J Physiol 1990; 258:E382-9; PMID:2106269
- Rognstad R, Katz J. The balance of pyridine nucleotides and ATP in adipose tissue. Proc Natl Acad Sci U S A 1966; 55:1148-56; PMID:4381021; http://dx.doi.org/10.1073/pnas.55.5.1148
- Berger JJ, Barnard RJ. Effect of diet on fat cell size and hormone-sensitive lipase activity. J Appl Physiol 1999; 87:227-32; PMID:10409579
- Klein S, Luu K, Gasic S, Green A. Effect of weight loss on whole body and cellular lipid metabolism in severely obese humans. Am J Physiol 1996; 270:E739-45; PMID:8967459
- Owens JL, Fuller EO, Nutter DO, DiGirolamo M. Influence of moderate exercise on adipocyte metabolism and hormonal responsiveness. J Appl Physiol 1977; 43:425-30; PMID:914713
- Hickner RC, Racette SB, Binder EF, Fisher JS, Kohrt WM. Effects of 10 days of endurance exercise training on the suppression of whole body and regional lipolysis by insulin. J Clin Endocrinol Metab 2000; 85:1498-504; PMID:10770188
- Stallknecht B, Larsen JJ, Mikines KJ, Simonsen L, Bülow J, Galbo H. Effect of training on insulin sensitivity of glucose uptake and lipolysis in human adipose tissue. Am J Physiol Endocrinol Metab 2000; 279:E376-85; PMID:10913038
- Shepherd RE, Sembrowich WL, Green HE, Gollnick PD. Effect of physical training on control mechanisms of lipolysis in rat fat cell ghosts. J Appl Physiol 1977; 42:884-8; PMID:195925
- Askew EW, Hecker AL, Coppes VG, Stifel FB. Cyclic AMP metabolism in adipose tissue of exercise-trained rats. J Lipid Res 1978; 19:729-36; PMID:211172
- Shepherd RE, Noble EG, Klug GA, Gollnick PD. Lipolysis and cAMP accumulation in adipocytes in response to physical training. J Appl Physiol 1981; 50:143-8; PMID:6259098
- Langin D. Control of fatty acid and glycerol release in adipose tissue lipolysis. C R Biol 2006; 329:598-607; discussion 653-5; PMID:16860278; http://dx.doi.org/10.1016/j.crvi.2005.10.008
- Murakami T, Shimomura Y, Fujitsuka N, Sokabe M, Okamura K, Sakamoto S. Enlargement glycogen store in rat liver and muscle by fructose-diet intake and exercise training. J Appl Physiol 1997; 82:772-5; PMID:9074962; http://dx.doi.org/10.1063/1.365771
- Holloszy JO, Coyle EF. Adaptations of skeletal muscle to endurance exercise and their metabolic consequences. J Appl Physiol 1984; 56:831-8; PMID:6373687
- Wisløff U, Helgerud J, Kemi OJ, Ellingsen O. Intensity-controlled treadmill running in rats: VO(2 max) and cardiac hypertrophy. Am J Physiol Heart Circ Physiol 2001; 280:H1301-10; PMID:11179077
- Pescatello LS, Arena R, Riebe D, Thompson PD, editors. ACSM's Guidelines for Exercise Testing and Prescription. 9th ed. Baltimore, MD: Wolters Kluwer Health; Lippincott Williams & Wilkins; 2014.
- Galbo H. Sympatho-adrenal activity in exercise and pancreatic hormones. In: Hormonal and Metabolic Adaptation to Exercise. Stuttgart: Georg Thieme Verlag; 1983;2-35.
- Gaidhu MP, Frontini A, Hung S, Pistor K, Cinti S, Ceddia RB. Chronic AMP-kinase activation with AICAR reduces adiposity by remodeling adipocyte metabolism and increasing leptin sensitivity. J Lipid Res 2011; 52:1702-11; PMID:21737753; http://dx.doi.org/10.1194/jlr.M015354
- DiGirolamo M, Fine JB. Cellularity measurements. Methods Mol Biol 2001; 155:65-75; PMID:11293084
- Ahlquist RP. Present state of alpha- and beta-adrenergic drugs I. The adrenergic receptor. Am Heart J 1976; 92:661-4; PMID:10722; http://dx.doi.org/10.1016/S0002-8703(76)80086-5
- Lafontan M, Barbe P, Galitzky J, Tavernier G, Langin D, Carpéné C, Bousquet-Melou A, Berlan M. Adrenergic regulation of adipocyte metabolism. Hum Reprod 1997; 12 Suppl 1:6-20; PMID:9403317; http://dx.doi.org/10.1093/humrep/12.suppl_1.6
- Litosch I, Hudson TH, Mills I, Li SY, Fain JN. Forskolin as an activator of cyclic AMP accumulation and lipolysis in rat adipocytes. Mol Pharmacol 1982; 22:109-15; PMID:6289066
- Gaidhu MP, Fediuc S, Anthony NM, So M, Mirpourian M, Perry RLS, Ceddia RB. Prolonged AICAR-induced AMP-kinase activation promotes energy dissipation in white adipocytes: novel mechanisms integrating HSL and ATGL. J Lipid Res 2009; 50:704-15; PMID:19050316; http://dx.doi.org/10.1194/jlr.M800480-JLR200
- Dole VP, Meinertz H. Microdetermination of long-chain fatty acids in plasma and tissues. J Biol Chem 1960; 235:2595-9; PMID:13817286