Abstract
Fermentation of dietary fibers by colonic microbiota generates short-chain fatty acids (SCFAs), e.g., propionic acid and butyric acid, which have been described to have “anti-obesity properties” by ameliorating fasting glycaemia, body weight and insulin tolerance in animal models. In the present study, we therefore investigate if propionic acid and butyric acid have effects on lipolysis, de novo lipogenesis and glucose uptake in primary rat adipocytes. We show that both propionic acid and butyric acid inhibit isoproterenol- and adenosine deaminase-stimulated lipolysis as well as isoproterenol-stimulated lipolysis in the presence of a phosphodiesterase (PDE3) inhibitor. In addition, we show that propionic acid and butyric acid inhibit basal and insulin-stimulated de novo lipogenesis, which is associated with increased phosphorylation and thus inhibition of acetyl CoA carboxylase, a rate-limiting enzyme in fatty acid synthesis. Furthermore, we show that propionic acid and butyric acid increase insulin-stimulated glucose uptake. To conclude, our study shows that SCFAs have effects on fat storage and mobilization as well as glucose uptake in rat primary adipocytes. Thus, the SCFAs might contribute to healthier adipocytes and subsequently also to improved energy metabolism with for example less circulating free fatty acids, which is beneficial in the context of obesity and type 2 diabetes.
Abbreviations:
- AMPK, AMP-activated protein kinase
- ACC, acetyl-CoA carboxylase
- ADA, adenosine deaminase
- BA, butyric acid
- BSA, bovine serum albumin
- FFAR, free fatty acid receptor
- GLUT, glucose transporter
- GPCR, G-protein-coupled receptor
- HSL, hormone-sensitive lipase
- ISO, isoproterenol
- KRBH, Krebs-Ringer bicarbonate-HEPES
- KRH, Krebs Ringer-HEPES
- PA, propionic acid
- PDE, cyclic nucleotide phosphodiesterase
- SCFAs, short-chain fatty acids
- T2D, type 2 diabetes
Introduction
Type 2 diabetes (T2D) is a heterogenous disorder, involving insulin resistance as well as impaired insulin secretion from pancreatic β-cells.Citation1 In individuals who are genetically predisposed, T2D commonly arises with progressive obesity, in particular visceral obesity.Citation2,3 Despite the fact that it is not exactly known what causes the strong and consistent association between obesity and development of T2D, spillover of fatty acids from white adipose tissue with consequent deposition in non-adipocyte cells, chronic low-grade inflammation and imbalance in the circulating hormones derived from adipose tissue are believed to be contributing factors.Citation2,4,5 Thus, adipose tissue is an important target for prevention and treatment of T2D.
Fermentation of dietary fibers consisting of non-digestible carbohydrates, such as non-starch polysaccharides and resistant starch, by colonic microbiota generates short-chain fatty acids (SCFAs).Citation6,7 Acetic acid, butyric acid and propionic acid are the most abundant SCFAs and they are believed to be important mediators of fermented dietary fibers on metabolism.Citation8 Dietary supplementation or rectal and oral administration of SCFAs to mice and rats decreases free fatty acids and glucose concentrations in blood,Citation9-13 but for studies in human subjects given SCFAs in the diet, the results are yet inconclusive.Citation14,15 Moreover, animal studies show that high-fat diet supplemented with propionic acid and butyric acid improve insulin sensitivity and protect against the development of obesity and insulin resistance.Citation11,13 In addition, obese mice treated with butyric acid decreased their fat content, which was consistent with loss of body weight and improved insulin tolerance, suggesting a role for butyric acid in the treatment of diet-induced obesity.Citation11 The effect of SCFAs are believed to be mediated via the free fatty acid receptor (Ffar) 3 and 2, also known as G-protein-coupled receptor (GPCR) 41 and 43, respectively, which are expressed in adipose tissue.8,16-18 For example, Kimura et al. generated an adipose-specific Ffar2 transgenic mouse model, which was lean, and when challenged by a high-fat diet, these mice were protected against development of obesity and showed improved insulin sensitivity.
Some effects by SCFAs on adipocytes have been described, e.g., inhibition of lipolysis. However, the information is scarce regarding the impact of SCFAs on several aspects of basal and hormone-regulated lipid and glucose metabolism in primary adipocytes, which is addressed in this study.
Results
Short-chain fatty acids inhibit lipolysis
The effects of propionic acid and butyric acid on basal and stimulated lipolysis were studied in primary rat adipocytes. Adenosine deaminase (ADA), isoproterenol (ISO) and the cyclic nucleotide phosphodiesterase (PDE) 3 inhibitor OPC3911 were used to increase cAMP concentrations by different mechanisms, leading to activation of cAMP-dependent protein kinase A (PKA) and activation of hormone-sensitive lipase (HSL), which together with other proteins stimulate lipolysis.Citation19 The PDE3 inhibitor is also used in order to test whether SCFAs mediate anti-lipolytic effects via the same pathway as insulin, which utilizes activation of PDE3B as a key component to inhibit lipolysis.Citation20 As shown in , the presence of propionic acid or butyric acid, inhibit lipolysis stimulated via activation of the stimulatory G protein (Gs) by ISO, removal of the adenosine inhibitory effect on cAMP production by ADA or inhibition of cAMP degradation by the family-selective PDE3 inhibitor. However, the SCFAs did not have an effect on basal lipolysis. A significant inhibition of ADA-stimulated lipolysis was seen at 3 mM for propionic acid whereas 10 mM of either propionic acid or butyric acid were required for a significant inhibition of ADA- and ISO-stimulated lipolysis, as seen in . “In the presence of OPC3911, basal as well as ISO-stimulated lipolysis was significantly enhanced (). Furthermore, OPC3911 did not prevent the anti-lipolytic effect of propionic acid and butyric acid on ISO-stimulated lipolysis ().
Figure 1. Short-chain fatty acids inhibit lipolysis in primary rat adipocytes. Lipolysis was measured after 30 min of stimulation with or without 30 nM isoproterenol (ISO), 10–30 U/ml adenosine deaminase (ADA) and 10 μM OPC3911 in the presence or absence of 3 and 10 mM propionic acid (PA) (A, B and E) or butyric acid (BA) (C-E). In A, C and E, the values are related to BASAL CTRL (condition without lipolytic agent and SCFA). In B and D, the values for PA and BA are related to respective CTRL (control without PA or BA) for each lipolytic condition. Mean ± SD (n = 5–13) were used and significance levels were accepted when *P < 0.05, **P < 0.01 and ***P < 0.001.
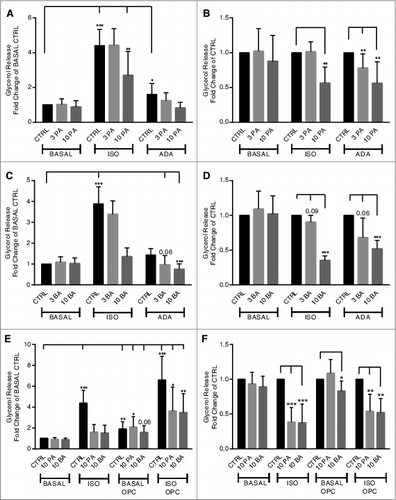
Short-chain fatty acids inhibit insulin-stimulated de novo lipogenesis
The effect of propionic acid and butyric acid on de novo lipogenesis was studied in primary rat adipocytes. It is well established that insulin stimulates the synthesis of fatty acids from e.g. glucose intermediates, known as de novo lipogenesis.Citation21 As shown in , both basal and insulin-stimulated de novo lipogenesis were inhibited by propionic acid and butyric acid, significant inhibition was seen at the lowest concentration (1 mM). To identify the molecular mechanism behind the inhibiting effect of SCFA, we studied the key enzyme ACC1 in de novo lipogenesis. In , we show that propionic acid and butyric acid increased phosphorylation of ACC1 at serine 79. This leads to an inactivation of the enzymatic activity of ACC1.Citation22 Taken together, the effect of propionic acid and butyric acid on phosphorylation of ACC1 is consistent with our finding that SCFA inhibits de novo lipogenesis.
Figure 2. Short-chain fatty acids inhibit de novo lipogenesis in primary rat adipocytes. De novo lipogenesis was measured after 30 min of stimulation with or without 1 nM insulin (INS) in the presence or absence of 1, 3 and 10 mM propionic acid (PA) (A and B) or butyric acid (C and D). In A and C, the values are related to BASAL, condition without insulin and SCFA. In B and D, the values for PA and BA are related to CTRL (BASAL and INS are used as CTRL in respective group), either in a basal or an insulin-stimulated state. Mean ± SD (n = 3–6) were used and significance levels were accepted when *P < 0.05, **P < 0.01 and ***P < 0.001.
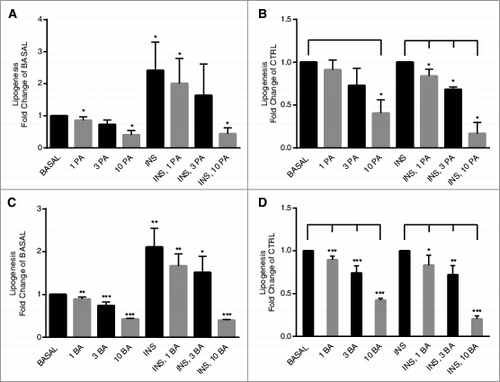
Figure 3. Short-chain fatty acids inhibit de novo lipogenesis via inhibition of ACC1. Primary rat adipocytes were stimulated with insulin (INS) in the presence or absence of 3 and 10 mM propionic acid (PA) and butyric acid (BA) for 10 min. In the CTRL, primary rat adipocytes were kept in a non-stimulatory condition. Homogenates were subjected to immunoblot analysis and membranes were probed for antibodies against ACC1 and phospho-ACC1 at serine 79 (S79). Quantification was made using Image Lab Software (Bio-Rad Laboratories) and data are presented as fold of insulin. Mean ± SD (n = 5–9) were used and significance levels were accepted when *P < 0.05, **P < 0.01 and ***P < 0.001.
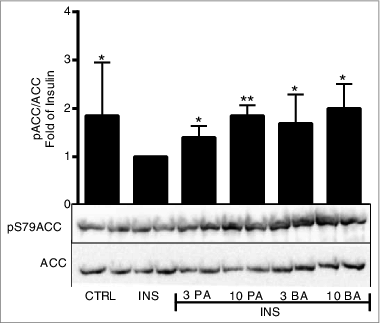
Short-chain fatty acids increase insulin-stimulated glucose uptake
The effect of propionic acid and butyric acid on glucose uptake was studied in primary rat adipocytes. As shown in , basal glucose uptake was significantly decreased by both propionic acid and butyric acid. However, insulin-stimulated glucose uptake was significantly increased in the presence of propionic acid () due to decreased and increased glucose uptake in the absence or presence of insulin, respectively. Butyric acid did also have an effect on insulin-stimulated glucose uptake (), however, the effect of butyric acid, as shown in , is solely due to a decreased basal glucose uptake in the presence of butyric acid (10 mM).
Figure 4. Short-chain fatty acids potentiate insulin-stimulated glucose uptake in primary adipocytes. Glucose uptake was measured after 30 min of stimulation with or without 1 nM insulin (INS) in the presence or absence of 1, 3 and 10 mM propionic acid (PA) (A and B) or butyric acid (BA) (C and D). In A and C, the values are related to BASAL CTRL, the condition without insulin and SCFA. In B and D, the values are based upon the ratio between BASAL and INS-stimulated conditions (shown in A and C). Mean ± SD (n = 4–5) were used and significance levels were accepted when *P < 0.05, **P < 0.01 and ***P < 0.001.
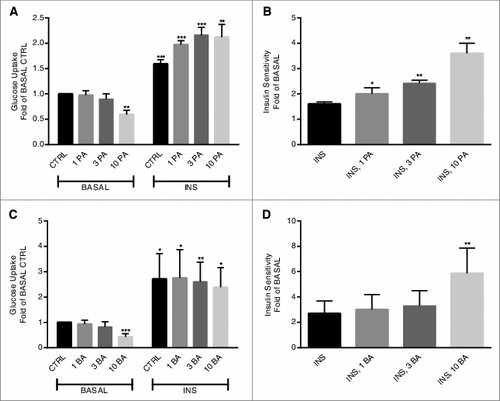
Discussion
In the literature, the beneficial effects seen with dietary fiber intake have partly been ascribed to the SCFAs.Citation8 For example, results from in vivo studies in mice suggest that dietary supplementation of propionic acid and butyric acid protects against high fat diet-induced obesity by e.g., inhibiting food intake, improving insulin sensitivity and increasing energy expenditure.Citation11,13 However, less is known regarding the role of specific cellular targets and mechanisms in mediating the overall beneficial effects on metabolism. Because adipocytes are key target cells concerning the prevention and treatment of T2D, in this study we evaluate the effects of a number of biological functions and mechanisms on primary rat adipocytes of relevance for glucose and lipid metabolism.
In adipose tissue, the hydrolysis of triacylglycerols into free fatty acids and glycerol, a process known as lipolysis, is often dysregulated in obesity, leading to excessive release of fatty acids to the plasma and decreased insulin sensitivity.Citation23 In the present study, we show that propionic acid and butyric acid inhibit lipolysis stimulated by activation of Gs via isoproterenol (ISO) and inhibition of Gi via the removal of adenosine using adenosine deaminase (ADA). Also, we show that lipolysis stimulated by suppression of the cAMP degrading enzyme PDE3 is inhibited by both SCFAs. The ability of SCFAs to inhibit lipolysis when PDE3B is suppressed indicates that SCFAs do not mediate their anti-lipolytic effects via enhanced degradation of cAMP, which is the case for insulin.Citation20 Furthermore, the ability of propionic acid and butyric acid to inhibit lipolysis in rat adipocytes in this process has to our knowledge not been shown previously, which has been discussed by others.Citation16,24 However, it has been shown that acetic acid and propionic acid inhibit basal and ISO- or ADA-potentiated lipolysis in 3T3-L1 adipocytes and primary mouse adipocytes.Citation17,18,24 Inhibition of lipolysis by SCFA could indeed have an important role in preventing ectopic fat accumulation and lipotoxicity, which are important risk factors for the generation of insulin resistance and T2D. The molecular targets for SCFA-mediated inhibition of ISO- and ADA-stimulated lipolysis are currently not known. We have made extensive attempts to demonstrate that SCFAs inhibit phosphorylation of adipose triglyceride lipase (ATGL), HSL, and perilipin (data not shown). Although small effects were obtained, significant effects could not be demonstrated. On the other hand, as expected, insulin efficiently inhibited hormone-stimulated phosphorylation of HSL and perilipin (data not shown). Thus, the downstream targets for the anti-lipolytic action of propionic acid and butyric need further investigation. Interestingly, a recent study by Aberdein et al.Citation25 showed that ISO-potentiated phosphorylation of HSL was decreased by approximately 15% by 4 mM acetic acid in 3T3-L1 adipocytes.
Adipocytes store energy in the form of cellular lipids, and de novo lipogenesis is part of this process, producing fatty acids subsequently used for triacylglycerol synthesis.Citation21 In the present study, under both basal and insulin-stimulated conditions, propionic acid and butyric acid decrease the rate of de novo lipogenesis, measured as the incorporation of [3H]-labeled glucose into cellular lipids, in a dose-dependent manner in rat adipocytes. In rat hepatocytes, it has been shown that propionic acid inhibits whereas acetic acid and butyric acid stimulate fatty acid synthesis using tritiated water as a substrate.Citation26,27 We also show that ACC1 is phosphorylated at serine 79 in the presence of propionic acid or butyric acid, leading to inhibition of the rate-limiting enzyme of de novo lipogenesis, which is consistent with the inhibitory effect of SCFAs on de novo lipogenesis. However, de novo lipogenesis is not the main pathway for the consequent synthesis of triacylglycerol in adipocytes,Citation21 rather triacylglycerols are synthesized from dietary fatty acids. However, during de novo lipogenesis, malonyl CoA, the inhibitor of carnitine palmitoyltransferase controlling the transport of fatty acid into the mitochondria, is produced from acetyl CoA.Citation22 Thus, the ability of SCFAs to decrease basal as well as insulin-stimulated de novo lipogenesis might have an important role to lower the production of malonyl CoA and to increase β-oxidation, a beneficial effect in the context of obesity and insulin resistance.
In adipocytes, basal glucose uptake and insulin-dependent glucose uptake are mediated by glucose transporter (GLUT)1 and GLUT4, respectively.Citation28 In the present study, basal glucose uptake is decreased by both propionic acid and butyric acid in rat primary adipocytes. A decreased glucose uptake by adipocytes is favorable when the body is maintaining euglycemia in a fasting condition. Interestingly, insulin-potentiated glucose uptake was further pronounced in a dose-dependent manner in the presence of propionic acid and to some extent with butyric acid by effects on basal lipolysis, which might have beneficial effects on glucose homeostasis in dysregulated metabolism. Liver and muscles utilize the major proportion of glucose in a postprandial state, but even though only a minor proportion of glucose is utilized by adipose tissue, it is favorable for whole body glucose homeostasis. For example, studies in mouse models with either an increased glucokinase productionCitation29 or with an adipocyte-specific knockdown of GLUT4Citation30 imply the favorable effect of a proper utilization of glucose in adipocytes on glucose tolerance and insulin sensitivity on the systemic level. Interestingly, GLUT4, which mediates insulin-dependent glucose uptake, is increased at the mRNA level in response to propionic acid in omental adipose tissue from overweight individuals.Citation31 Another study has shown that acetic acid inhibits insulin-potentiated glucose uptake and suppresses insulin signaling via PKB inhibition in 3T3-L1 adipocytes and primary adipocytes from mice.Citation32
In the present study, concentrations in the millimolar range were required to obtain effects on primary adipocytes. Concentrations of the SCFAs fluctuate in the circulation throughout the day, which is particularly dependent on fasted and fed state, but also reflected upon time point for meal consumption.Citation33-35 The formation and proportion of SCFAs is also dependent on several other factors, such as amount and type of indigestible carbohydrate and its accessibility in diet, fermentation capability of the residing bacterial populations and gut transit time.Citation36,37 Measures of circulation SCFA concentrations have been done in sudden-death victims,Citation7,34,38 as well as in healthy individualsCitation39,40 and all 3 SCFAs (acetic acid > propionic acid > butyric acid) have been estimated to be in the micromolar range concentration in the systemic circulation. The net uptake (measured as the arteriovenous difference across the tissue times the tissue plasma flow) of the SCFAs by abdominal subcutaneous adipose tissue has been estimated to be 18.2 μmol/100 mL adipose tissue (AT) for acetic acid and 1.53 μmol/100 mL AT for propionic acid in humans received a diet supplemented with dietary fibers or placebo.Citation40 However, in vitro and in the present study, it is not unusual to obtain effects by hormones and nutrient used in supra-physiological concentrations, which is a limiting factor for drawing conclusions. On the other hand, cell models are important tools for evaluating target tissues and molecular mechanism behind an obtained effect in vivo.
Thus, the present study shows a number of effects of propionic acid and butyric acid on glucose and lipid metabolism that could cont-ribute to the beneficial effects of dietary fibers on metabolism. Interestingly, the microbiota appears to be of relevance in this context. Indeed, dietary fiber intake can promote a shift in the colonic microbiota composition,Citation41,42 leading to a change in the production of SCFAs.Citation42 In humans, the microbiota differs between lean and obese individuals but whether a specific phylum is more dominant than another has still not been unraveled.Citation42-44 T2D patients have also an altered microbiota with a notable decrease in butyric acid-generating bacteria, e.g. Roseburia species.Citation45 The advantage of having a lean phenotype of the microbiota has thus been observed in individuals with a metabolic disturbance, as infusion of lean microbiota via a gastroduodenal tube improved insulin sensitivity, which also was associated with a notable increase of butyric acid-generating bacterial species, in these individuals.Citation46 Although it is known that dietary habits can have effects on colonic microbiota composition and thus generation of SCFAs, as described above, the exact physiological impact of these changes needs to be further investigated.
In summary, the obtained effects by SCFAs on glucose and lipid metabolism in primary adipocytes, described herein, might be beneficial for prevention of dysfunctional adipocytes associated with insulin resistance as seen in obese and diabetic individuals. Hence, as SCFAs are prominent targets for development of functional foods for prevention of obesity and T2D, further investigations are needed to estimate the actual concentration of SCFAs that fluctuates around visceral adipose tissue as well as to elucidate the precise underlying mechanism on adipocyte function.
Materials and Methods
Animal model
Sprague Dawley males, 36 d of age, purchased from Charles River Laboratories (Germany), were kept under standardized conditions in the animal house facilities at Lund University. The Committee of ethical animal research in Malmö and Lund has approved all experimental procedures (permission number: M245–12).
Adipocyte isolation
Epididymal white adipose tissue was isolated and digested in collagenase for 45 min at 37°C with agitation and then washed as previously described.Citation47 The total volume of packed cells (lipocrit) in the adipocyte suspension was determined as described by Fine et al.Citation48 The adipocytes were resuspended and diluted to the final cell concentration (1–10%), depending on experimental setup.
Lipolysis
Lipolysis was measured as described by Dole et al.Citation49 Aliquots (300 μl) of a 5% suspension of adipocytes were prepared in Krebs Ringers HEPES (KRH) containing 25 mM HEPES (pH 7.4), 120 mM NaCl, 4.7 mM KCl, 1.2 mM KH2PO4, 1.2 mM MgSO4, 2.5 mM CaCl2, 1% BSA, 2 mM glucose, and 200 nM adenosine and incubated with or without 30 nM DL-Isoproterenol hydrochloride (ISO) (Sigma-Aldrich, St. Louis, MO, USA), 10–30 U/ml adenosine deaminase (ADA) (Roche Diagnostics, Mannheim, Germany), 10 μM OPC3911 (PDE3 inhibitor) (Osaka Inc., Japan) in the presence or absence of 3 and 10 mM propionic acid (Sigma-Aldrich) or butyric acid (Sigma-Aldrich) at 37°C for 30 min in a shaking device (150 rpm). The cell-free medium was transferred to wells and buffer containing 50 mM glycine, 0.05% hydrazinehydrate, 1 mM MgCl2, 0.75 mg/ml ATP (Sigma-Aldrich), 0.375 mg/ml NAD (Roche, Pleasanton, CA, USA), 25 μg/ml glycerol-3-phosphate dehydrogenase (Roche) and 0.5 μg/ml glycerol kinase (Roche) was added. Lipolysis was measured at optical density of 340 nm as the release of glycerol into the cell-free medium.
Lipogenesis
Lipogenesis was measured as described by Moody et al.Citation50 Aliquots (700 μl) of a 2% suspension of adipocytes were prepared in KRH with low glucose (containing 0.55 mM glucose and 3.5% BSA without adenosine) and 14 μl D-[6–3H (N)] glucose (22 μCi/ml) (Perkin Elmer, Waltham, MA, USA) was added as substrate for lipogenesis. Cells were stimulated with 1 nM insulin (Novonordisk, Bagsvaerd, Denmark) in the presence or absence of 1, 3 and 10 mM propionic acid or butyric acid in a shaking device (80 rpm) at 37°C for 30 min. Incubations were stopped by addition of toluene-based scintillation liquid (VWR Chemicals, Radnor, PA, USA) containing 0.3 mg/ml 2.5-diphenyloxazole (PPO) (Sigma-Aldrich) and 5 mg/ml 1.4-bis-[4-methyl-5-phenyl-2-oxazolyl]benzene (POPOP) (Sigma-Aldrich) and the incorporation of 3H into cellular lipids in the non-water soluble phase was determined by scintillation counting.
Glucose uptake
Glucose uptake was measured as described by Foley et al.Citation51 Aliquots (200 μl) of a 5% suspension of adipocytes were prepared in a glucose-free KRBH buffer containing 120 mM M NaCl, 4 mM KH2OP4, 1 mM MgSO4, 0.75 mM CaCl2, 10 mM NaHCO3, 1% BSA and 30 mM HEPES (pH 7.4). 100 μl D-[14C (U)] (57.8 mCi/mmol) was added as substrate for glucose uptake. The adipocyte suspensions were incubated with or without 1 nM insulin in the presence or absence of 1, 3 and 10 mM propionic acid or butyric acid in a shaking device (100 rpm) at 37°C for 30 min. Adipocytes were separated from the medium by addition of 60 μl dinonylphthalate and then briefly centrifuged. Ultima Gold scintillation liquid (PerkinElmer, Waltham, MA, USA) was added to the cell suspensions and uptake of 14C glucose was determined by scintillation counting.
SDS-PAGE and Immunoblot Analysis
Aliquots of a 10% suspension of adipocytes were used for 10 min incubations with 1 nM insulin in the presence or absence of 1, 3 and 10 mM propionic acid or butyric acid. Cells were homogenized with a lysis buffer containing 50 mM Tris-HCl (pH 7.5), 1 mM EGTA, 1 mM EDTA, 1% NP40, 1 mM Na-orthovanadate, 50 mM NaF, 5 mM Na-pyrophosphate, 0.27 M sucrose, Nonidet P 40 (Sigma-Aldrich) and supplemented with NuPAGE sample reducing agent (Novex, Grand Island, NY, USA) and Complete Protease Inhibitor (containing inhibitors for serine-, cysteine-, and metalloproteases as well as calpains) (Roche) and centrifuged at 13,600 × g for 15 min. The total protein amount was determined according to Bradford. Samples (10–15 μg) were subjected to SDS-PAGE. Proteins were electrotransferred to Amersham Hybond-C Extra nitrocellulose membranes (GE Healthcare Bio-Sciences, Pittsburgh, PA, USA) and the membranes were stained with Ponceau S (0.1% in 5% acetic acid) and then blocked with 10% milk in a buffer consisting of 20 mM Tris-HCl, pH 7.6, 137 mM NaCl and 0.1% (v/w) Tween-20 for 60 min. Membranes with proteins were probed with antibodies for acetyl CoA carboxylase 1 (ACC1) (Cell signaling Technology, Danvers, MA, USA) and phospho-ACC1(S79) (Cell signaling Technology) and incubated overnight at 4°C. Proteins were detected using the chemiluminescent Super Signal West Pico Luminol/Enhancer solution (Thermo Fisher Scientific, Waltham, MA, USA) and the ChemiDoc XRS+ Imager (Bio-Rad Laboratories, Hercules, CA, USA.). Image Lab Software (Bio-Rad Laboratories) was used for quantification.
Statistics
Data are presented as mean ± SD from the indicated number of experiments. Statistically significant differences were analyzed using Student´s t-test and significance levels were accepted when *P < 0.05, **P < 0.01 and ***P < 0.001.
Disclosure of Potential Conflicts of Interest
No potential conflicts of interest were disclosed.
Author Contributions
All authors contributed to the design of the study and manuscript writing. EH contributed to the conduct of the study and data collection, whereas all authors contributed with analysis as well as data interpretation.
Acknowledgments
Eva Ohlson is acknowledged for her excellent technical assistance.
Funding
The study was financially supported by the Antidiabetic Food Center (AFC), a VINNOVA VINN Excellence Center at Lund University.
References
- DeFronzo RA. Insulin resistance, lipotoxicity, type 2 diabetes and atherosclerosis: the missing links. The Claude Bernard Lecture 2009. Diabetologia 2010; 53:1270-87; PMID:20361178; http://dx.doi.org/10.1007/s00125-010-1684-1
- Capurso C, Capurso A. From excess adiposity to insulin resistance: the role of free fatty acids. Vascul Pharmacol 2012; 57:91-7; PMID:22609131; http://dx.doi.org/10.1016/j.vph.2012.05.003
- Hajer GR, van Haeften TW, Visseren FL. Adipose tissue dysfunction in obesity, diabetes, and vascular diseases. Eur Heart J 2008; 29:2959-71; PMID:18775919; http://dx.doi.org/10.1093/eurheartj/ehn387
- Mittendorfer B. Origins of metabolic complications in obesity: adipose tissue and free fatty acid trafficking. Curr Opin Clin Nutr Metab Care 2011; 14:535-41; PMID:21849896; http://dx.doi.org/10.1097/MCO.0b013e32834ad8b6
- Henry SL, Bensley JG, Wood-Bradley RJ, Cullen-McEwen LA, Bertram JF, Armitage JA. White adipocytes: more than just fat depots. Int J BiochemCell Biol 2012; 44:435-40; PMID:22222895; http://dx.doi.org/10.1016/j.biocel.2011.12.011
- Everard A, Cani PD. Diabetes, obesity and gut microbiota. Best Prac Res Clin Gastroenterol 2013; 27:73-83; PMID:23768554; http://dx.doi.org/10.1016/j.bpg.2013.03.007
- Al-Lahham SH, Peppelenbosch MP, Roelofsen H, Vonk RJ, Venema K. Biological effects of propionic acid in humans; metabolism, potential applications and underlying mechanisms. Biochim Biophy Acta 2010; 1801:1175-83; PMID:20691280; http://dx.doi.org/10.1016/j.bbalip.2010.07.007
- den Besten G, van Eunen K, Groen AK, Venema K, Reijngoud DJ, Bakker BM. The role of short-chain fatty acids in the interplay between diet, gut microbiota, and host energy metabolism. J Lipid Res 2013; 54:2325-40; PMID:23821742; http://dx.doi.org/10.1194/jlr.R036012
- Boillot J, Alamowitch C, Berger AM, Luo J, Bruzzo F, Bornet FR, Slama G. Effects of dietary propionate on hepatic glucose production, whole-body glucose utilization, carbohydrate and lipid metabolism in normal rats. Br J Nutr 1995; 73:241-51; PMID:7718543; http://dx.doi.org/10.1079/BJN19950026
- Berggren AM, Nyman EM, Lundquist I, Bjorck IM. Influence of orally and rectally administered propionate on cholesterol and glucose metabolism in obese rats. Br J Nutr 1996; 76:287-94; PMID:8813902; http://dx.doi.org/10.1079/BJN19960032
- Gao Z, Yin J, Zhang J, Ward RE, Martin RJ, Lefevre M, Cefalu WT, Ye J. Butyrate improves insulin sensitivity and increases energy expenditure in mice. Diabetes 2009; 58:1509-17; PMID:19366864; http://dx.doi.org/10.2337/db08-1637
- Yamashita H, Fujisawa K, Ito E, Idei S, Kawaguchi N, Kimoto M, Hiemori M, Tsuji H. Improvement of obesity and glucose tolerance by acetate in Type 2 diabetic Otsuka Long-Evans Tokushima Fatty (OLETF) rats. Biosci Biotechnol Biochem 2007; 71:1236-43; PMID:17485860; http://dx.doi.org/10.1271/bbb.60668
- Lin HV, Frassetto A, Kowalik EJ, Jr., Nawrocki AR, Lu MM, Kosinski JR, Hubert JA, Szeto D, Yao X, Forrest G, et al. Butyrate and propionate protect against diet-induced obesity and regulate gut hormones via free fatty acid receptor 3-independent mechanisms. PloS One 2012; 7:e35240; PMID:22506074; http://dx.doi.org/10.1371/journal.pone.0035240
- Wolever TM, Spadafora P, Eshuis H. Interaction between colonic acetate and propionate in humans. Am J Clin Nutr 1991; 53:681-7; PMID:2000822
- Todesco T, Rao AV, Bosello O, Jenkins DJ. Propionate lowers blood glucose and alters lipid metabolism in healthy subjects. Am J Clin Nutr 1991; 54:860-5; PMID:1951157
- Brown AJ, Goldsworthy SM, Barnes AA, Eilert MM, Tcheang L, Daniels D, Muir AI, Wigglesworth MJ, Kinghorn I, Fraser NJ, et al. The Orphan G protein-coupled receptors GPR41 and GPR43 are activated by propionate and other short chain carboxylic acids. J J Biol Chem 2003; 278:11312-9; PMID:12496283; http://dx.doi.org/10.1074/jbc.M211609200
- Hong YH, Nishimura Y, Hishikawa D, Tsuzuki H, Miyahara H, Gotoh C, Choi KC, Feng DD, Chen C, Lee HG, et al. Acetate and propionate short chain fatty acids stimulate adipogenesis via GPCR43. Endocrinology 2005; 146:5092-9; PMID:16123168; http://dx.doi.org/10.1210/en.2005-0545
- Ge H, Li X, Weiszmann J, Wang P, Baribault H, Chen JL, Tian H, Li Y. Activation of G protein-coupled receptor 43 in adipocytes leads to inhibition of lipolysis and suppression of plasma free fatty acids. Endocrinology 2008; 149:4519-26; PMID:18499755; http://dx.doi.org/10.1210/en.2008-0059
- Londos C, Brasaemle DL, Schultz CJ, Adler-Wailes DC, Levin DM, Kimmel AR, Rondinone CM. On the control of lipolysis in adipocytes. Ann N Y Acad Sci 1999; 892:155-68; PMID:10842661; http://dx.doi.org/10.1111/j.1749-6632.1999.tb07794.x
- Castan I, Wijkander J, Manganiello V, Degerman E. Mechanisms of inhibition of lipolysis by insulin, vanadate and peroxovanadate in rat adipocytes. Biochem J 1999; 339 (Pt 2):281-9; PMID:10191258; http://dx.doi.org/10.1042/0264-6021:3390281
- Czech MP, Tencerova M, Pedersen DJ, Aouadi M. Insulin signalling mechanisms for triacylglycerol storage. Diabetologia 2013; 56:949-64; PMID:23443243; http://dx.doi.org/10.1007/s00125-013-2869-1
- Schreurs M, Kuipers F, van der Leij FR. Regulatory enzymes of mitochondrial beta-oxidation as targets for treatment of the metabolic syndrome. Obes Rev 2010; 11:380-8; PMID:19694967; http://dx.doi.org/10.1111/j.1467-789X.2009.00642.x
- Chaves VE, Frasson D, Kawashita NH. Several agents and pathways regulate lipolysis in adipocytes. Biochimie 2011; 93:1631-40; PMID:21658426; http://dx.doi.org/10.1016/j.biochi.2011.05.018
- Zaibi MS, Stocker CJ, O'Dowd J, Davies A, Bellahcene M, Cawthorne MA, Brown AJ, Smith DM, Arch JR. Roles of GPR41 and GPR43 in leptin secretory responses of murine adipocytes to short chain fatty acids. FEBS Lett 2010; 584:2381-6; PMID:20399779; http://dx.doi.org/10.1016/j.febslet.2010.04.027
- Aberdein N, Schweizer M, Ball D. Sodium acetate decreases phosphorylation of hormone sensitive lipase in isoproterenol-stimulated 3T3-L1 mature adipocytes. Adipocyte 2014; 3:121-5; PMID:24719785; http://dx.doi.org/10.4161/adip.27936
- Nishina PM, Freedland RA. Effects of propionate on lipid biosynthesis in isolated rat hepatocytes. J Nutr 1990; 120:668-73; PMID:2366102
- Demigne C, Morand C, Levrat MA, Besson C, Moundras C, Remesy C. Effect of propionate on fatty acid and cholesterol synthesis and on acetate metabolism in isolated rat hepatocytes. Br J Nutr 1995; 74:209-19; PMID:7547838; http://dx.doi.org/10.1079/BJN19950124
- Ebeling P, Koistinen HA, Koivisto VA. Insulin-independent glucose transport regulates insulin sensitivity. FEBS Lett 1998; 436:301-3; PMID:9801136; http://dx.doi.org/10.1016/S0014-5793(98)01149-1
- Munoz S, Franckhauser S, Elias I, Ferre T, Hidalgo A, Monteys AM, Molas M, Cerdan S, Pujol A, Ruberte J, et al. Chronically increased glucose uptake by adipose tissue leads to lactate production and improved insulin sensitivity rather than obesity in the mouse. Diabetologia 2010; 53:2417-30; PMID:20623219; http://dx.doi.org/10.1007/s00125-010-1840-7
- Abel ED, Peroni O, Kim JK, Kim YB, Boss O, Hadro E, Minnemann T, Shulman GI, Kahn BB. Adipose-selective targeting of the GLUT4 gene impairs insulin action in muscle and liver. Nature 2001; 409:729-33; PMID:11217863; http://dx.doi.org/10.1038/35055575
- Al-Lahham S, Roelofsen H, Rezaee F, Weening D, Hoek A, Vonk R, Venema K. Propionic acid affects immune status and metabolism in adipose tissue from overweight subjects. Eur J Clin Invest 2012; 42:357-64; PMID:21913915; http://dx.doi.org/10.1111/j.1365-2362.2011.02590.x
- Kimura I, Ozawa K, Inoue D, Imamura T, Kimura K, Maeda T, Terasawa K, Kashihara D, Hirano K, Tani T, et al. The gut microbiota suppresses insulin-mediated fat accumulation via the short-chain fatty acid receptor GPR43. Nat Commun 2013; 4:1829; PMID:23652017; http://dx.doi.org/10.1038/ncomms2852
- Wolever TM, Josse RG, Leiter LA, Chiasson JL. Time of day and glucose tolerance status affect serum short-chain fatty acid concentrations in humans. Metabolism 1997; 46:805-11; PMID:9225836; http://dx.doi.org/10.1016/S0026-0495(97)90127-X
- Bach Knudsen KE, Serena A, Canibe N, Juntunen KS. New insight into butyrate metabolism. Proc Nutr Soc 2003; 62:81-6; PMID:12740062; http://dx.doi.org/10.1079/PNS2002212
- Nilsson AC, Ostman EM, Knudsen KE, Holst JJ, Bjorck IM. A cereal-based evening meal rich in indigestible carbohydrates increases plasma butyrate the next morning. J Nutr 2010; 140:1932-6; PMID:20810606; http://dx.doi.org/10.3945/jn.110.123604
- Macfarlane S, Macfarlane GT. Regulation of short-chain fatty acid production. Proc Nutr Soc 2003; 62:67-72; PMID:12740060; http://dx.doi.org/10.1079/PNS2002207
- Soldavini J, Kaunitz JD. Pathobiology and potential therapeutic value of intestinal short-chain fatty acids in gut inflammation and obesity. Dig Dis Sci 2013; 58:2756-66; PMID:23839339; http://dx.doi.org/10.1007/s10620-013-2744-4
- Cummings JH, Pomare EW, Branch WJ, Naylor CP, Macfarlane GT. Short chain fatty acids in human large intestine, portal, hepatic and venous blood. Gut 1987; 28:1221-7; PMID:3678950; http://dx.doi.org/10.1136/gut.28.10.1221
- Jakobsdottir G, Bjerregaard JH, Skovbjerg H, Nyman M. Fasting serum concentration of short-chain fatty acids in subjects with microscopic colitis and celiac disease: no difference compared with controls, but between genders. Scand J Gastroenterol 2013; 48:696-701; PMID:23600961; http://dx.doi.org/10.3109/00365521.2013.786128
- Robertson MD, Bickerton AS, Dennis AL, Vidal H, Frayn KN. Insulin-sensitizing effects of dietary resistant starch and effects on skeletal muscle and adipose tissue metabolism. Am J Clin Nutr 2005; 82:559-67; PMID:16155268
- Walker AW, Ince J, Duncan SH, Webster LM, Holtrop G, Ze X, Brown D, Stares MD, Scott P, Bergerat A, et al. Dominant and diet-responsive groups of bacteria within the human colonic microbiota. ISME J 2011; 5:220-30; PMID:20686513; http://dx.doi.org/10.1038/ismej.2010.118
- Duncan SH, Lobley GE, Holtrop G, Ince J, Johnstone AM, Louis P, Flint HJ. Human colonic microbiota associated with diet, obesity and weight loss. Int J Obes (Lond) 2008; 32:1720-4; PMID:18779823; http://dx.doi.org/10.1038/ijo.2008.155
- Ley RE, Turnbaugh PJ, Klein S, Gordon JI. Microbial ecology: human gut microbes associated with obesity. Nature 2006; 444:1022-3; PMID:17183309; http://dx.doi.org/10.1038/4441022a
- Schwiertz A, Taras D, Schafer K, Beijer S, Bos NA, Donus C, Hardt PD. Microbiota and SCFA in lean and overweight healthy subjects. Obesity (Silver Spring) 2010; 18:190-5; PMID:19498350; http://dx.doi.org/10.1038/oby.2009.167
- Qin J, Li Y, Cai Z, Li S, Zhu J, Zhang F, Liang S, Zhang W, Guan Y, Shen D, et al. A metagenome-wide association study of gut microbiota in type 2 diabetes. Nature 2012; 490:55-60; PMID:23023125; http://dx.doi.org/10.1038/nature11450
- Vrieze A, Van Nood E, Holleman F, Salojarvi J, Kootte RS, Bartelsman JF, Dallinga-Thie GM, Ackermans MT, Serlie MJ, Oozeer R, et al. Transfer of intestinal microbiota from lean donors increases insulin sensitivity in individuals with metabolic syndrome. Gastroenterology 2012; 143:913-6. e7; PMID:22728514; http://dx.doi.org/10.1053/j.gastro.2012.06.031
- Honnor RC, Dhillon GS, Londos C. cAMP-dependent protein kinase and lipolysis in rat adipocytes. I. Cell preparation, manipulation, and predictability in behavior. J Biol Chem 1985; 260:15122-9; PMID:2415513
- Fine JB, DiGirolamo M. A simple method to predict cellular density in adipocyte metabolic incubations. Int J Obes Relat Metab Disd 1997; 21:764-8; PMID:9376888; http://dx.doi.org/10.1038/sj.ijo.0800469
- Dole VP, Meinertz H. Microdetermination of long-chain fatty acids in plasma and tissues. J Biol Chem 1960; 235:2595-9; PMID:13817286
- Moody AJ, Stan MA, Stan M, Gliemann J. A simple free fat cell bioassay for insulin. Horm Metab Res 1974; 6:12-6; PMID:4819286; http://dx.doi.org/10.1055/s-0028-1093895
- Foley JE, Kashiwagi A, Verso MA, Reaven G, Andrews J. Improvement in in vitro insulin action after one month of insulin therapy in obese noninsulin-dependent diabetics. Measurements of glucose transport and metabolism, insulin binding, and lipolysis in isolated adipocytes. J Clin Invest 1983; 72:1901-9; PMID:6358258; http://dx.doi.org/10.1172/JCI111153