Abstract
The prevalence of obesity has risen to an unprecedented level. According to World Health Organization, over 500 million adults, equivalent to 10%–14% of the world population, were obese with a body mass index (BMI) of 30 kg/m2 or greater in 2008.Citation1 This rising prevalence and earlier onset of obesity is believed to be resulted from an interplay of genetic factors, over-nutrition and physical inactivity in modern lifestyles. Obesity also increases the susceptibility to metabolic syndromes, hypertension, cardiovascular diseases, Type 2 diabetes mellitus (T2DM) and cancer.Citation2-4 The global obesity epidemic has sparked substantial interests in the biology of adipose tissue (fat). In addition, the skeletal muscle and its secretive factors (myokines) have also been shown to play a critical role in controlling body energy balance, adipose homeostasis and inflammation status.Citation5 Interestingly, skeletal muscle cells share a common developmental origin with brown adipocytes,Citation6,7 which breaks down lipids to generate heat – thus reducing obesity. Here, we provide a brief overview of the basics and recent progress in muscle-fat crosstalk in the context of body energy metabolism, obesity, and diabetes. We summarize the different types of adipocytes, their developmental origins and implications in body composition. We highlight the role of several novel myokines in regulating fat mass and systemic energy balance, and evaluate the potential of skeletal muscles as a therapeutic target to treat obesity.
Characterization and Origin of Brown, White and Beige Adipocytes
White and brown adipocytes are 2 types of commonly known fat cells in mammals.Citation8 White adipocytes are characterized by a spherical shape, a large single lipid droplet that takes up to 90% of the total cell volume, and few mitochondria.Citation9 White adipocytes constitute the bulky white adipose tissues (WAT) located under the skin (subcutaneous fat), in the muscle (intramuscular fat or “marbling” fat), and in the abdominal cavity attached to the visceral mass (visceral fat).Citation10 They store excess food intake (or energy surplus) in the form of triglycerides, which can be utilized to generate energy under energy deficit conditions. In contrast, brown adipocytes contain multilocular lipid droplets and numerous mitochondria.Citation9 They are found in brown adipose tissues (BAT) mainly located in the interscapular regions of rodents and human infants.Citation10,11 Unlike white adipocytes, brown adipocytes break down lipids to produce heat, thus reducing adiposity. The thermogenesis of brown adipocytes is mediated by uncoupling protein 1 (UCP1) that is uniquely located in their inner mitochondria membrane. Of note, uncoupling activity of UCP1 is under control by sympathetic innervationCitation12 as well as other factors like free fatty acids from lipolysis.Citation13 The thermogenic “energy-leaking” feature of brown adipocytes has made BAT an appealing therapeutic target for treating obesity.Citation14
Although brown and white adipocytes share multiple morphological and functional similarities, their developmental origins are surprisingly different (). Recent studies have revealed a vascular or perivascular localization of white adipocyte progenitors, and identified several molecular markers of these cells (for example Zfp423, VE-Cad, PDGFRβ, and PDGFRα).Citation15-18 However, the anatomical localization of brown adipocyte progenitors has not been reported. Interestingly, classic brown fat, but not white fat, shares a developmental origin with the skeletal muscle. Lineage tracing studies demonstrate that muscle and brown fat are from a population of mesodermal progenitors that express myogenic marker genes Myf5, Pax7 and Pax3.Citation6,19,20 Furthermore, brown adipocytes and muscle cells share a similar mitochondrial proteomic signatureCitation21 and both contribute to thermogenesis.Citation22,23 In fact, brown fat precursors express a variety of myogenic genes prior to differentiation.Citation24 This is followed by progressive loss of myogenic signature gene expression during brown fat maturation. Recent studies have begun to elucidate factors that diverge brown adipose from muscle development. Particularly, PRDM16 (PRD1-BF-1-RIZ1 homologous domain-containing protein-16) has been shown to be a bidirectional switch that determines BAT versus muscle fate.Citation6 Loss of PRDM16 in brown preadipocytes leads to a phenotypic switch to muscle cells, while ectopic expression of PRDM16 in myoblasts switches them back to brown adipocytes.Citation6 More recently, it was reported that miR-133 acts as an upstream regulator of PRDM16 to control skeletal muscle vs. BAT fate choice in muscle stem cells (satellite cells).Citation25 Although BAT-specific deletion of PRDM16 does not affect embryonic BAT development, it promotes white fat-specific gene expression in BAT in young mice and attenuates thermogenesis in old mice.Citation26 These lines of evidence suggest that BAT originates differently from WAT, but shares developmental similarities with skeletal muscles.
Figure 1. Origin of brown, white and beige adipocytes. Two types of common precursors of muscle/fat lineage are derived from mesenchymal stem cells. These 2 types of common precursors are distinguished by Myf5, Pax7, Pax3 and PDGFRα expression. Myf5+, Pax7+ and Pax3+ common precursors give rise to specified muscle or brown adipose precursors that differentiate into either mature myocytes (Myf5+, MyoD+, Myogenin+) or brown adipocytes (UCP1+), respectively. Myf5-, Pax3-, Pax7- and PDGFRα+ common precursors give rise to specified beige or white adipose precursors that differentiate into either mature beige adipocytes (Tnfrnfs9+, Tmem26+, Tbx1+) or white adipocytes (Eva1+, Hspb7+, Pdk4+), respectively. The interconversion of mature beige and white adipocytes may occur under environmental stimulus.
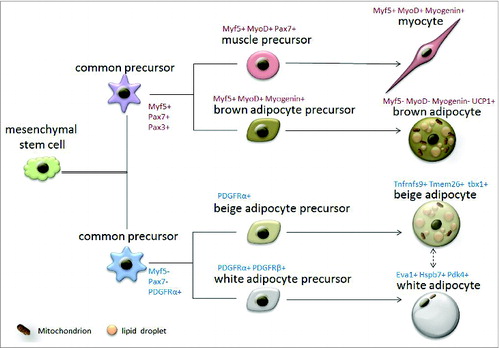
It has long been known that when rodents are exposed to cold stress or β-adrenergic stimulating drugs, certain depots of WAT undergo a “browning” process, giving rise to brown adipocyte-like cells (called beige or brite adipocytes) with multilocular lipid droplets, UCP1 expression and thermogenic activity.Citation27 However, whether adult humans have a similar capacity to form inducible BAT (iBAT) has been unclear. Recently, advanced imaging analysis using positron emission tomography (PET) revealed that in cold conditions, active “brown-like” adipocytes form along the cervical-supraclavicular area in human adults.Citation28–31 These cells retain radioactive fluorodeoxyglucose, suggesting that they actively uptake and utilize glucose. Different from classic WAT and BAT, iBAT is characterized by the presence of beige adipocytes.Citation32 Beige cells specifically express several marker genes (for example, Tnfrnfs9, Tmem26 and Tbx1 in mice and HOXC8, HOXC9 and CITED1 in humans) that are not expressed by white or brown adipocytes. In contrast, brown adipocytes uniquely express several maker genes (for example, Eva1, Hspb7 and Pdk4 in mouse and EPSTI1, LHX8 and ZIC1 in human) that are not shared by beige or white cells.Citation33 Notably, genetic analysis of human iBAT suggests it contains both brown and beige adipocytes.Citation34,35
Beige adipocytes can be differentiated de novo from precursors or transdifferentiate from mature white adipocytes (). Beige cell precursors are believed to be enriched in Pax3− cell lineage.Citation36 A study using Adiponectin-Cre combined with Tert inducible lacZ mice (named Adipo-chaser mice) shows that in response to cold stimulation, preadipocytes in subcutaneous fat undergo de novo differentiation to form beige cells, while abdominal beige fat cells accumulate through proliferation.Citation37 Using EdU labeling combined with β3-adrenergic receptor stimulation, it was shown that the mitotic index was 7.5 times higher in epididymal WAT than inguinal WAT. Interestingly, UCP1 co-localizes with a fraction of the proliferating cells.Citation38 Together, these studies suggest the existence of beige preadipocytes. It is worth mentioning that an independent study identified a population of beige precursors within skeletal muscle that respond to bone morphogenetic protein 7 (BMP7) stimulation.Citation7 On the other hand, interconversion of white and beige adipocytes has also been observed. Even before the characterization of beige cells, it was reported that white adipose precursors do not increase in response to cold acclimation, and iBAT formation is predominantly due to β3-adrenergic receptor-dependent transdifferentiation of white adipocytes.Citation39 Indeed, murine perivascular Pdgfra+ cells differentiate into beige adipocytes upon β-adrenergic receptor stimulation, but become white adipocytes in response to high fat diet (HFD) feeding.Citation40 Based on these studies, cold can induce de novo formation of beige adipocytes from a population of beige precursor cells. These beige adipocytes are plastic: they can become white adipocytes upon warm adaption and switch back to beige adipocytes after additional cold stimulation. Beige cell formation is regulated by many factors. Among these, inhibition of Prdm16 blocks the function of beige adipose and induces redistribution of subcutaneous white fat to unfavorable visceral fat, resulting in obesity and metabolic disorders.Citation41 On the contrary, inhibition of Notch signaling dramatically promotes “browning” of white adipocyte through activating Prdm16 and Pparg (Peroxisome proliferator-activated receptor gamma). Importantly, the Notch inhibitor dibenzazepine (DBZ) effectively reduces adiposity and improves glucose metabolism in ob/ob mice.Citation42 Further understanding of mechanisms underlying beige cell development and adipose “browning” will have a huge impact in the treatment of obesity.
Adipose depots at different anatomical locations not only differ in the relative composition of brown, beige and white adipocytes,Citation8 but are also heterogeneous in developmental origins.Citation43-46 Classic brown adipose depots include interscapular BAT (isBAT), subscapular BAT (sBAT), cervical BAT (cBAT), peri-aortic BAT (paBAT) and renal BAT (rBAT), whereas white adipose depots include anterior subcutaneous WAT (asWAT), inguinal WAT (inWAT), retroperitoneal WAT (rWAT), gonadal WAT (gWAT), mesenteric WAT (mWAT), and intramuscular fat (IMAT)Citation8,44,45,47,48 (). The white adipose depots can also be broadly divided into subcutaneous WAT (asWAT and inWAT) and visceral WAT (sWAT, rWAT and gWAT). IMAT refers to adipocytes located in muscle interstitiium and is different from the intramyocellular triglycerides, which refer to cellular lipid droplets within muscle cells. Developmentally, visceral WAT develops from the lateral plate mesoderm Wnt+ cells whereas no subcutaneous or BAT were found to come from the Wnt+ population.Citation46 A recent in vivo lineage tracing experiment employing the Myf5-Cre, Myod-Cre, Pax3-Cre mice models combined with mTmG dual reporter mice shows that while isBAT depot contains 99% Myf5 lineage adipocytes, cBAT had only ∼60% Myf5 lineage adipocytes. Myf5 lineage cells also contribute to 25% and 50% of asWAT and rWAT progenitors, respectively. Pax3 lineage tracing shows similar results, but interestingly, shows gender differences in various depots especially in rBAT and gWAT.Citation45 These experiments together suggest that depot-specific origin of adipose tissues is under combined influences of genetic, breeds, age, gender and even seasons.
Figure 2. Distribution of various adipose depots Adipose tissues are generally divided into brown adipose depots and white adipose depots. Brown adipose depots include interscapular BAT (isBAT), subscapular BAT (sBAT), cervical BAT (cBAT), peri-aortic BAT (paBAT) and renal BAT (rBAT). White adipose depots include anteriorsubcutaneous WAT (asWAT), inguinal WAT (inWAT), retroperitoneal WAT (rWAT), gonadal WAT (gWAT), mesenteric WAT (mWAT), and intramuscular fat (IMAT).
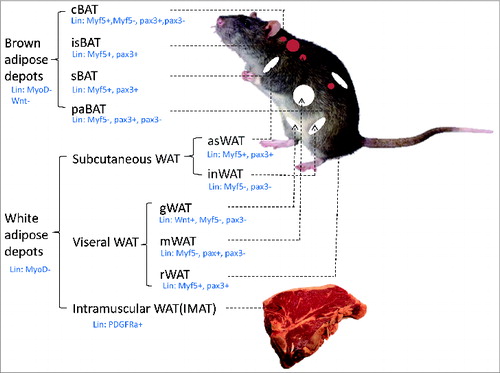
Role of Skeletal Muscle in Body Energy Balance
Obesity results from an energy surplus (i. e. greater energy intake than expenditure). As the largest organ in the body, the skeletal muscle comprises ∼40% of body massCitation49 and serves as one of the major regulators of body energy homeostasis. Even under severe obese conditions, skeletal muscles still account for ∼25% of body mass and remain metabolically active to a certain extent. In order to satisfy the structural and functional needs for skeletal muscle, massive amounts of energy are utilized for muscle protein synthesis and motor function under both resting and exercise conditions. Skeletal muscles mainly utilize glucose as the energy source, but can also utilize free fatty acids (FFA) and amino acids (AA) as fuels or structural “building blocks." Thus, the skeletal muscle can affect not only glucose, but also fat and protein homeostasis in the body.
Skeletal muscles uptake ∼75% of ingested carbohydrate from meals.Citation50 Postprandial plasma glucose is primarily taken up through glucose transporter 4 (GLUT4) facilitated infusion, and then subjected to glycolysis, oxidative phosphorylation, or glycogen synthesis depending on individual activity levels. The process of glucose uptake and catabolism during muscle activity contributes to a negative energy balance. Contraction and insulin stimulation are 2 main activators of muscle glucose uptake.Citation51
Muscle contraction directly stimulates glucose uptake predominantly through activation of AMP-activate protein kinase (AMPK), which increases GLUT4 translocation to the muscle cell membrane (sarcolemma).Citation52,53 Briefly, muscle contraction increases the ratio of AMP/ATP, which is detected by energy sensor AMPK. The activated AMPK then phosphorylates TBC1 (Tre-2/USP6, BUB2, Cdc16) domain family member 4 and 1 (TBC1D 4 and TBC1D1), leading to the release of GLUT4 from cytoplasmic storage vesicles to the sarcolemma.Citation52 Differential phenotypes of mice lacking AMPK α, β, or γ subunit suggest that each subunit has a unique role in regulating muscle glucose uptake.Citation54-58 AMPK-independent mechanisms may also be involved in skeletal muscle glucose uptake. For example, the antioxidant N-acetyl-l-cysteine (NAC) and the nitric oxide synthase (NOS) inhibitor N(G)-monomethyl-l-arginine (l-NMMA) can attenuate contraction stimulated glucose uptake in both wild type and muscle specific AMPKα2 knockout mice.Citation59 As an energy “sensor” and regulatory molecule, AMPK also inhibits glycogen synthesis, promotes fatty acid oxidation, and enhances mitochondria oxidation in contracting muscle cells. AMPK also promotes exercise-mediated improvements in insulin sensitivity through mechanisms involving interleukin 6 (IL-6), adiponectin, IR, IRS, and mTOR.Citation52 Human studies indicate that AMPK mRNA and protein levels are down-regulated in obese subjects but elevated by exercise, suggesting the potential of AMPK mimetic as therapeutic agents to treat obesity and insulin resistance. In fact, metformin, an AMPK activating compound, has been used as the first-line drug in current clinical treatments of diabetes.Citation60,61 The most updated meta-analyses also indicate that metformin also reduces colorectal, liver, pancreatic and stomach cancer risks in diabetic patients.Citation62
Insulin stimulates muscle glucose uptake and disposal through the IR-IRS-PI3K pathway. Insulin binding to the insulin receptor (IR) activates the intrinsic tyrosine kinase of IRβ-subunit, which phosphorylates insulin receptor substrate 1 (IRS-1). Phospho-IRS-1 docks class I phosphatidlyinositol-3-kinase (PI3K), which phosphorylates Akt and atypical protein kinase C zeta (aPKCζ), leading to GLUT4 translocation. Insulin resistance commonly associated with obesity and T2DM describes the condition of reduced cellular responsiveness to circulating insulin, resulting in the hyperinsulinemia, hyperglycinemia and hyperlipidemia. Insulin resistance dampers insulin signaling transduction without damaging the structures needed for glucose uptake, since insulin resistant human skeletal muscles exhibit normal GLUT4 translocation when stimulated by contraction or hypoxia.Citation63,64 As ∼80% of body insulin stimulated glucose uptake is mediated by the skeletal muscle,Citation52 skeletal muscle insulin resistance can be devastating, and can lead to a vicious circle of insulin resistance and metabolic disorders. Thus, exercise therapies and pharmaceutical compounds that improve muscle insulin sensitivity represent a promising direction to treat Type 2 diabetes and other metabolic syndromes.
In addition to glucose, skeletal muscle also utilizes fatty acids and proteins depending on availability of substrates. This adaptive process is known as “metabolic flexibility.” During fasting, fatty acid oxidation (FAO) accounts for up to 90% of muscle energy supply. Healthy human skeletal muscle actively catabolizes non-esterified fatty acid (NEFA) via hormone-sensitive lipoprotein lipase (LPL), while muscles of obese or T2DM patients show nearly diminished NEFA uptake and deficiency in FAO.Citation65 The impaired FFA metabolism potentially causes ectopic lipid accumulation in skeletal muscle, liver, and heart; increasing the risk of insulin resistance and metabolic disorders. The impaired FFA metabolism may be associated with mitochondrial mass reduction or functional disruption, such as inhibition of carnitine palmitoyltransferase 1 (CPT-1) mediated lipid transport and ROS inactivation of mitochondrial membrane enzymes.Citation65,66
The skeletal muscle is also highly active in protein metabolism since it requires rapid protein synthesis and degradation for maintaining muscle turnover. Proteins also serve as a source of energy for muscle contraction under extreme fasting conditions. Increased levels of plasma amino acids (AA) and decreased skeletal muscle protein synthesis were evident in obese animal models.Citation67 Skeletal muscle protein metabolism is regulated by complex mechanisms. Mammalian target of rapamycin (mTOR), especially the mTOR complex 1 (mTORC1), is the most important regulator of skeletal muscle mass and protein synthesis in response to high plasma AA concentration. Activation of mTOR initiates canonical muscle protein synthesis pathways, and interestingly, partially decreases autophagic influx that facilitates protein degradation.Citation49
Myokines Regulate Body Fat Composition and Inflammation Status
Skeletal muscle is not only a motor organ but also an endocrine organ releasing small secretive molecules known as myokines. Broadly speaking, “peptides or proteins that are produced, expressed and released by muscle fibers and exert a paracrine or endocrine effect” are referred to as myokines.Citation68-71 Myokines often alter body energy utilization and inflammatory status, therefore affecting weight gain and fat composition. Common myokines include irisin, myostatin, interleukins (IL6, 7, 8, 15), Leukemia Inhibitory Factor (LIF), among others.Citation5
Irisin is a short peptide cleaved from the extracellular domain of the Fndc5 (fibronectin domain-containing 5). Fndc5 mRNA is abundantly expressed in the heart, brain, rectum and skeletal muscle and moderately in the intracranial artery, tongue and optic nerve.Citation72-74 The expression pattern implies that irisin may exert functions on multiple tissues. One example is that exercising mice have elevated Fndc5 in their hippocampus, suggesting that Fndc5 positively regulates neural activities.Citation75 Another example is the original discovery demonstrating irisin as an “exercise hormone” secreted by skeletal muscle. Once secreted, irisin circulates through the bloodstream to fat tissues, where it promotes browning of white adipocytes.Citation76 The irisin mediated “browning” of WAT depends on proliferator-activated receptor γ coactivator 1 α (PGC1-α).Citation77 The “browning” can be synergistically enhanced by fibroblast growth factor21 (FGF21), a cold-induced myokine.Citation78-80 Irisin also serves as a focal regulator of muscle cell metabolism through promoting mitochondria biogenesis and metabolic gene expression.Citation81 Besides its hormonal function, Fndc5 may serve as a transmembrane signaling protein.Citation82 However, contradictory results regarding association between plasma irisin levels and insulin sensitivity have been reported in human studies.Citation83 For example, obesity and T2DM are reported to be negatively correlated with irisin/ FNDC5 secretion and mRNA expression.Citation84,85 In contrast, high levels of baseline plasma irisin are correlated with insulin resistance in obese patients, implying the possibility of “irisin resistance”.Citation86 In cell culture experiments, palmitate and glucose treatments that partially mimics diabetic and obese situations decrease muscle cell FNDC5 mRNA expression.Citation85 Other studies show that neither acute nor long term exercise significantly improves circulating irisin level or brown fat gene expression, despite elevated irisin/Fndc5 expression in muscle.Citation84,85 Another independent study reports that the human FNCD5 gene start codon is mutated, and irisin treatment does not increase human brite cell formation in vitro.Citation87 Although the majority of studies support the idea that irisin/Fndc5 mediates the “crosstalk” of skeletal muscles to other tissues in rodents,Citation80,88 whether the effect of irisin can be translated to humans remains to be confirmed.
Myostatin (Mstn), also called growth/differentiation factor 8 (GDF8), is another extensively studied and well-established myokine. Mstn belongs to the highly conserved TGF-β family and its absence causes dramatic increases of muscle cell size (hypertrophy) in humans, mice, cattle and other mammals.Citation89-92 Mstn also affects other tissues such as adipose and bone.Citation93 In addition to muscle hypertrophy, reduced adiposity and adipose browning were observed in muscle-specific and whole body Mstn knockout, but not in adipose-specific Mstn knockout mice.Citation93-95 Mechanistically, whole body deletion of Mstn robustly induces Fndc5 expression in skeletal muscle, which in turn increases serum irisin levels and promotes formation of beige fat cells.Citation96 Although Mstn and one of its receptors – activin receptor IIB (ActRIIB) – are expressed by adipocytes, the expression level may be too low to provide physiological relevance,Citation97-99 explaining the lack of phenotype in adipose-specific knockout mice. Consistently, Mstn treatment has no effect on cultured adipocytes.Citation96 Together these lines of evidence suggest that Mstn reduces body fat composition and induces “browning” of WAT likely through an indirect mechanism mediated by the skeletal muscle. In both human and rodents, Mstn is expressed at higher levels in obese individuals and lower levels in exercised subjects.Citation5,Citation99-101 As Mstn seems to partially explain the beneficial effect of exercise, targeting Mstn signaling pathway using soluble ActRIIB decoy receptor ACE-031 is currently in clinical trial.Citation102
The IL family members (IL-4, 6, 7, 8 and 15) are regarded as myokines for their versatile functions in regulating energy homeostasis, immune responses and cell movements.Citation94 They are produced in muscle cells, though some, such as IL-8, are mainly produced by other cell types. Of these IL family myokines, IL-6 appears to have the most promising clinical value. IL6 production is low when cellular glucose import is high, but increases during exerciseCitation103 or when skeletal muscle glycogen content is lowCitation103. IL-6 also promotes muscle growth and regenerationCitation104,105 in addition to its function in improving muscle glucose metabolism.Citation106 Recombinant IL-6 improves glucose metabolism and insulin sensitivity in humans, and helps to shift the source of skeletal muscle energy from glucose to fat.Citation5 However, IL-6 is also produced by adipocytes and has been shown to induce insulin resistanceCitation107-109 and inflammation in adipose tissues.Citation110 Moreover, elevation of the long-term effect of IL-6 seems to suggest some adverse effects.Citation111 Other IL family members IL-7, 8 and 15 are all involved in immune responses that play a key role in adipose tissue function.Citation5 Although they are not yet well-characterized, they do seem to facilitate the maintenance of energy homeostasis or inflammation status. Notably, IL-15 mediates muscle-fat interaction by negatively regulating lipid metabolism.Citation112
Other myokines and potential myokines include LIFs,Citation5 FGF2,Citation5 brain-derived neurotrophic factor,Citation70,113,114 follistatin,Citation5 insulin-like growth factor 1,Citation5,115,116 monocyte chemoattractant protein,Citation117,118 and myonectinCitation119,120 (). Myokines are secreted in different amounts and combinations depending on various physical activity levels. Some of the myokines are also produced in adipose tissue and are known as adipomyokines.Citation70 The adipomyokines are key players involved in bidirentional muscle-fat cross talk.
Table 1. Potential myokines, their secretion and target sites, and functions
More Muscle, Less Fat?
Obesity and sarcopenia (age related loss of muscle) represent 2 typical situations in which fat mass and muscle mass are negatively correlated. Obesity is generally accompanied by increased fat and lean mass, but the fat mass increases at a larger scale, resulting in a smaller lean muscle to fat ratio. The overall increased body mass in obese individuals also leads to skeletal muscle overload, resulting in greater leg and trunk muscle strength but not handgrip or arm strength.Citation121 Sarcopenic obesity, which affects 5–10% of the elderly, is a type of obesity in which patients have normal body mass but little lean mass. Sarcopenic obesity is difficult to detect and treat, because subjects have a normal body weight.Citation122 In addition to sarcopenic obesity, aging is naturally accompanied by sarcopenia and fat deposition,Citation123-125 together with redistribution of body fat from appendicular to stomach and ectopic sites such as liver and muscle.Citation48
Individuals who exercise regularly gain more muscle mass, have better muscle function, and lower risks of obesity and T2DM. In contrast, physical inactivity is associated with decreased muscle mass, increased visceral adiposity, and increased macrophage infiltration, chronic systemic inflammation, insulin resistance, obesity, and T2DM.Citation5 As discussed previously, skeletal muscle exercise increases energy expenditure, stimulates secretion of beneficial myokines, and increases insulin sensitivity. Muscle exercise also attenuates adipogenesis of mesenchymal stem cells through Akt-mediated mechanical signal responses.Citation126,127 An increase in skeletal muscle mass may directly lead to reduced body fat composition, manifested by the fact that body builders often find themselves stuck in an extremely lean condition.Citation128 Consistently, animals with enhanced muscle growth, such as Mstn knockout mice and Callipyge sheep, often have little body fat deposition.Citation95,129 However, moderate to high intensity exercise training increases intramyocellular triglycerides,Citation130-132 a lipid store within muscle cells.Citation133 This phenomenon is referred to as “athlete's paradox”Citation134 and is likely associated with an increase in oxidative metabolism in exercised muscle. The intramyocellular triacylglycerol droplets act as a FFA fuel source for muscle contraction.Citation135 Interestingly, diabetic individuals also have elevated levels intramyocellular triglycerides.Citation132,134
Body fat deposition in turn affects skeletal muscle function. It is widely accepted that inflammatory adipokines secreted by ectopic accumulation of fat leads to muscle insulin resistance.Citation136,137 It was also reported that FFA and exercise mimetics (such as AICAR) impact the secretion of myokines, especially the IL family members.Citation138 Importantly, fat deposition affects muscle homeostasis. Decreases of muscle mass were consistently reported in obese rodents and humans. In accordance, mice with ectopic fat accumulation exhibit impaired muscle regeneration possibly due to lipid toxicity, pro-inflammatory cytokines and compromised muscle stem cell or satellite cell function.Citation139 Adipocytes are nevertheless necessary for muscle regeneration, and in the absence of adipocytes skeletal muscles cannot regenerate after injury.Citation19 This observation suggests that adipocytes or their “crosstalk” to muscle cells may facilitate muscle recovery. Indeed, adiponenctin, an adipokine secreted by adipocytes, has been reported to improve skeletal muscle regeneration by promoting satellite cell proliferation and differentiation.Citation140
Summary and Future Trends
Muscle-fat interaction is a complicated process acting at multiple levels. (1) Developmental origin. Muscle and fat both develop from mesenchymal precursors; brown fat and skeletal muscle share surprisingly similar origins, suggesting a close relationship, even interconversion between adipocytes and muscle cells. (2) Energy balance. Muscle affects global energy balance and inflammatory status through its active metabolism of nutrients. In these processes, muscle substrates and metabolites intricately “talk” to adipose and other tissues. (3) Body composition. Increased muscle mass and muscle exercise reduces fat deposition and increases insulin sensitivity through secretion of beneficial myokines. (4) Adipose tissue affects muscle inflammation, insulin sensitivity and regeneration possibly through adipokine secretion and physical infiltration, providing a novel target to regulate muscle function.
In conclusion, regulating skeletal muscle function represents a potential strategy to counteract obesity and T2DM. Understanding muscle-fat interaction may lead to the development of exercise therapies and molecular targets for obesity treatment. It is also critical to understand how muscle reacts to fat accumulation in order to minimize loss of muscle mass and function in obesity. Areas that need further exploration include the research of beneficial myokines, individually tailored training programs, and mechanisms underlying muscle-fat interactions that can be employed for developing drugs. It is critical to carry out human studies in order to translate animal data into novel therapeutic approaches to benefit mankind.
Disclosure of Potential Conflicts of Interest
No potential conflicts of interest were disclosed.
References
- Malik VS, Willett WC, Hu FB. Global obesity: trends, risk factors and policy implications. Nat Rev Endocrinol 2013; 9:13-27; PMID:23165161; http://dx.doi.org/10.1038/nrendo.2012.199
- Park YW, Zhu S, Palaniappan L, Heshka S, Carnethon MR, Heymsfield SB. The metabolic syndrome: prevalence and associated risk factor findings in the US population from the third national health and nutrition examination survey, 1988-1994. Arch Intern Med 2003; 163:427-36; PMID:12588201; http://dx.doi.org/10.1001/archinte.163.4.427
- Adams KF, Schatzkin A, Harris TB, Kipnis V, Mouw T, Ballard-Barbash R, Hollenbeck A, Leitzmann MF. Overweight, obesity, and mortality in a large prospective cohort of persons 50 to 71 years old. New Eng J Med 2006; 355:763-78; PMID:16926275; http://dx.doi.org/10.1056/NEJMoa055643
- Kabakov E, Norymberg C, Osher E, Koffler M, Tordjman K, Greenman Y, Stern N. Prevalence of hypertension in type 2 diabetes mellitus: impact of the tightening definition of high blood pressure and association with confounding risk factors. J Cardiometabolic Syndrome 2006; 1:95-101; PMID:17679829; http://dx.doi.org/10.1111/j.1559-4564.2006.05513.x
- Pedersen BK, Febbraio MA. Muscles, exercise and obesity: skeletal muscle as a secretory organ. Nat Rev Endocrinol 2012; 8:457-65; PMID:22473333; http://dx.doi.org/10.1038/nrendo.2012.49
- Seale P, Bjork B, Yang W, Kajimura S, Chin S, Kuang S, Scime A, Devarakonda S, Conroe HM, Erdjument-Bromage H, et al. PRDM16 controls a brown fatskeletal muscle switch. Nature 2008; 454:961-7; PMID:18719582; http://dx.doi.org/10.1038/nature07182
- Schulz TJ, Huang TL, Tran TT, Zhang H, Townsend KL, Shadrach JL, Cerletti M, McDougall LE, Giorgadze N, Tchkonia T, et al. Identification of inducible brown adipocyte progenitors residing in skeletal muscle and white fat. Proc Nat Acad Sci U S A 2011; 108:143-8; PMID:21173238; http://dx.doi.org/10.1073/pnas.1010929108
- Cinti S. The adipose organ. Prostaglandins, Leukotrienes, and Essential Fatty Acids 2005; 73:9-15; PMID:15936182; http://dx.doi.org/10.1016/j.plefa.2005.04.010
- Cinti S. The adipose organ at a glance. Dis Model Mech 2012; 5:588-94; PMID:22915020; http://dx.doi.org/10.1242/dmm.009662
- Rosen Evan D, Spiegelman Bruce M. What we talk about when we talk about fat. Cell 2014; 156:20-44; PMID:24439368; http://dx.doi.org/10.1016/j.cell.2013.12.012
- Lidell ME, Betz MJ, Dahlqvist Leinhard O, Heglind M, Elander L, Slawik M, Mussack T, Nilsson D, Romu T, Nuutila P, et al. Evidence for two types of brown adipose tissue in humans. Nat Med 2013; 19:631-4; PMID:23603813; http://dx.doi.org/10.1038/nm.3017
- Cannon B, Nedergaard J. Brown adipose tissue: function and physiological significance. Physiol Rev 2004; 84:277-359; PMID:14715917; http://dx.doi.org/10.1152/physrev.00015.2003
- Fedorenko A, Lishko PV, Kirichok Y. Mechanism of fatty-acid-dependent UCP1 uncoupling in brown fat mitochondria. Cell 2012; 151:400-13; PMID:23063128; http://dx.doi.org/10.1016/j.cell.2012.09.010
- Saito M. Brown adipose tissue as a therapeutic target for human obesity. Obes Res Clin Prac 2013; 7:e432-8; PMID:24459687; http://dx.doi.org/10.1016/j.orcp.2013.09.001
- Tang W, Zeve D, Suh JM, Bosnakovski D, Kyba M, Hammer RE, Tallquist MD, Graff JM. White fat progenitor cells reside in the adipose vasculature. Science 2008; 322:583-6; PMID:18801968; http://dx.doi.org/10.1126/science.1156232
- Gupta RK, Mepani RJ, Kleiner S, Lo JC, Khandekar MJ, Cohen P, Frontini A, Bhowmick DC, Ye L, Cinti S, et al. Zfp423 expression identifies committed preadipocytes and localizes to adipose endothelial and perivascular cells. Cell Met 2012; 15:230-9; PMID:22326224; http://dx.doi.org/10.1016/j.cmet.2012.01.010
- Tran KV, Gealekman O, Frontini A, Zingaretti MC, Morroni M, Giordano A, Smorlesi A, Perugini J, De Matteis R, Sbarbati A, et al. The vascular endothelium of the adipose tissue gives rise to both white and brown fat cells. Cell Met 2012; 15:222-9; PMID:22326223; http://dx.doi.org/10.1016/j.cmet.2012.01.008
- Berry R, Rodeheffer MS. Characterization of the adipocyte cellular lineage in vivo. Nat Cell Biol 2013; 15:302-8; PMID:23434825; http://dx.doi.org/10.1038/ncb2696
- Liu W, Liu Y, Lai X, Kuang S. Intramuscular adipose is derived from a non-Pax3 lineage and required for efficient regeneration of skeletal muscles. Dev Biol 2012; 361:27-38; PMID:22037676; http://dx.doi.org/10.1016/j.ydbio.2011.10.011
- Lepper C, Fan CM. Inducible lineage tracing of Pax7-descendant cells reveals embryonic origin of adult satellite cells. Genesis 2010; 48:424-36; PMID:20641127; http://dx.doi.org/10.1002/dvg.20630
- Forner F, Kumar C, Luber CA, Fromme T, Klingenspor M, Mann M. Proteome differences between brown and white fat mitochondria reveal specialized metabolic functions. Cell Met 2009; 10:324-35; PMID:19808025; http://dx.doi.org/10.1016/j.cmet.2009.08.014
- Schonfeld P, Wojtczak L. Brown adipose tissue mitochondria oxidizing fatty acids generate high levels of reactive oxygen species irrespective of the uncoupling protein-1 activity state. Biochimica et Biophysica Acta 2012; 1817:410-8; PMID:22226918; http://dx.doi.org/10.1016/j.bbabio.2011.12.009
- Rolfe DF, Brand MD. Contribution of mitochondrial proton leak to skeletal muscle respiration and to standard metabolic rate. Am J Physiol 1996; 271:C1380-9; PMID:8897845
- Timmons JA, Wennmalm K, Larsson O, Walden TB, Lassmann T, Petrovic N, Hamilton DL, Gimeno RE, Wahlestedt C, Baar K, et al. Myogenic gene expression signature establishes that brown and white adipocytes originate from distinct cell lineages. Proc Nat Acad Sci U S A 2007; 104:4401-6; PMID:17360536; http://dx.doi.org/10.1073/pnas.0610615104
- Yin H, Pasut A, Soleimani VD, Bentzinger CF, Antoun G, Thorn S, Seale P, Fernando P, van Ijcken W, Grosveld F, et al. MicroRNA-133 controls brown adipose determination in skeletal muscle satellite cells by targeting Prdm16. Cell Met 2013; 17:210-24; PMID:23395168; http://dx.doi.org/10.1016/j.cmet.2013.01.004
- Harms MJ, Ishibashi J, Wang W, Lim HW, Goyama S, Sato T, Kurokawa M, Won KJ, Seale P. Prdm16 is required for the maintenance of brown adipocyte identity and function in adult mice. Cell Met 2014; 19:593-604; PMID:24703692; http://dx.doi.org/10.1016/j.cmet.2014.03.007
- Shabalina IG, Petrovic N, de Jong JM, Kalinovich AV, Cannon B, Nedergaard J. UCP1 in britebeige adipose tissue mitochondria is functionally thermogenic. Cell Rep 2013; 5:1196-203; PMID:24290753; http://dx.doi.org/10.1016/j.celrep.2013.10.044
- Cypess AM, Lehman S, Williams G, Tal I, Rodman D, Goldfine AB, Kuo FC, Palmer EL, Tseng YH, Doria A, et al. Identification and importance of brown adipose tissue in adult humans. New Eng J Med 2009; 360:1509-17; PMID:19357406; http://dx.doi.org/10.1056/NEJMoa0810780
- van Marken Lichtenbelt WD, Vanhommerig JW, Smulders NM, Drossaerts JM, Kemerink GJ, Bouvy ND, Schrauwen P, Teule GJ. Cold-activated brown adipose tissue in healthy men. New Eng J Med 2009; 360:1500-8; PMID:19357405; http://dx.doi.org/10.1056/NEJMoa0808718
- Nedergaard J, Bengtsson T, Cannon B. Unexpected evidence for active brown adipose tissue in adult humans. Am J Physiol Endocrinol Met 2007; 293:E444-52; PMID:17473055; http://dx.doi.org/10.1152/ajpendo.00691.2006
- Saito M, Okamatsu-Ogura Y, Matsushita M, Watanabe K, Yoneshiro T, Nio-Kobayashi J, Iwanaga T, Miyagawa M, Kameya T, Nakada K, et al. High incidence of metabolically active brown adipose tissue in healthy adult humans: effects of cold exposure and adiposity. Diabetes 2009; 58:1526-31; PMID:19401428; http://dx.doi.org/10.2337/db09-0530
- Wu J, Bostrom P, Sparks LM, Ye L, Choi JH, Giang AH, Khandekar M, Virtanen KA, Nuutila P, Schaart G, et al. Beige adipocytes are a distinct type of thermogenic fat cell in mouse and human. Cell 2012; 150:366-76; PMID:22796012; http://dx.doi.org/10.1016/j.cell.2012.05.016
- Bartelt A, Heeren J. Adipose tissue browning and metabolic health. Nat Rev Endocrinol 2014; 10:24-36; PMID:24146030; http://dx.doi.org/10.1038/nrendo.2013.204
- Jespersen NZ, Larsen TJ, Peijs L, Daugaard S, Homoe P, Loft A, de Jong J, Mathur N, Cannon B, Nedergaard J, et al. A classical brown adipose tissue mRNA signature partly overlaps with brite in the supraclavicular region of adult humans. Cell Met 2013; 17:798-805; PMID:23663743; http://dx.doi.org/10.1016/j.cmet.2013.04.011
- Nedergaard J, Cannon B. How brown is brown fat? It depends where you look. Nat Med 2013; 19:540-1; PMID:23652104; http://dx.doi.org/10.1038/nm.3187
- Liu W, Shan T, Yang X, Liang S, Zhang P, Liu Y, Liu X, Kuang S. A heterogeneous lineage origin underlies the phenotypic and molecular differences of white and beige adipocytes. J Cell Sci 2013; 126:3527-32; PMID:23781029; http://dx.doi.org/10.1242/jcs.124321
- Wang QA, Tao C, Gupta RK, Scherer PE. Tracking adipogenesis during white adipose tissue development, expansion and regeneration. Nat Med 2013; 19:1338-44; PMID:23995282; http://dx.doi.org/10.1038/nm.3324
- Lee YH, Petkova AP, Mottillo EP, Granneman JG. In vivo identification of bipotential adipocyte progenitors recruited by beta3-adrenoceptor activation and high-fat feeding. Cell Metab 2012; 15:480-91; PMID:22482730; http://dx.doi.org/10.1016/j.cmet.2012.03.009
- Barbatelli G, Murano I, Madsen L, Hao Q, Jimenez M, Kristiansen K, Giacobino JP, De Matteis R, Cinti S. The emergence of cold-induced brown adipocytes in mouse white fat depots is determined predominantly by white to brown adipocyte transdifferentiation. Am J Physiol Endocrinol Met 2010; 298:E1244-53; PMID:20354155; http://dx.doi.org/10.1152/ajpendo.00600.2009
- Rosenwald M, Perdikari A, Rulicke T, Wolfrum C. Bi-directional interconversion of brite and white adipocytes. Nat Cell Biol 2013; 15:659-67; PMID:23624403; http://dx.doi.org/10.1038/ncb2740
- Cohen P, Levy JD, Zhang Y, Frontini A, Kolodin DP, Svensson KJ, Lo JC, Zeng X, Ye L, Khandekar MJ, et al. Ablation of PRDM16 and beige adipose causes metabolic dysfunction and a subcutaneous to visceral fat switch. Cell 2014; 156:304-16; PMID:24439384; http://dx.doi.org/10.1016/j.cell.2013.12.021
- Bi P, Shan T, Liu W, Yue F, Yang X, Liang XR, Wang J, Li J, Carlesso N, Liu X, et al. Inhibition of Notch signaling promotes browning of white adipose tissue and ameliorates obesity. Nat Med 2014; PMID:25038826
- Wan DC, Longaker MT. Fat or fiction: origins matter. Cell Met 2014; 19:900-1; PMID:24896537; http://dx.doi.org/10.1016/j.cmet.2014.05.007
- Sanchez-Gurmaches J, Guertin DA. Adipocyte lineages: tracing back the origins of fat. Biochimica et Biophysica Acta 2014; 1842:340-51; PMID:23747579; http://dx.doi.org/10.1016/j.bbadis.2013.05.027
- Sanchez-Gurmaches J, Guertin DA. Adipocytes arise from multiple lineages that are heterogeneously and dynamically distributed. Nat Commun 2014; 5:4099; PMID:24942009; http://dx.doi.org/10.1038/ncomms5099
- Chau YY, Bandiera R, Serrels A, Martinez-Estrada OM, Qing W, Lee M, Slight J, Thornburn A, Berry R, McHaffie S, et al. Visceral and subcutaneous fat have different origins and evidence supports a mesothelial source. Nat Cell Biol 2014; PMID:24609269
- Gesta S, Tseng YH, Kahn CR. Developmental origin of fat: tracking obesity to its source. Cell 2007; 131:242-56; PMID:17956727; http://dx.doi.org/10.1016/j.cell.2007.10.004
- Addison O, Marcus RL, Lastayo PC, Ryan AS. Intermuscular fat: a review of the consequences and causes. Int J Endocrinol 2014; 2014:309570; PMID:24527032; http://dx.doi.org/10.1155/2014/309570
- Neel BA, Lin Y, Pessin JE. Skeletal muscle autophagy: a new metabolic regulator. Trends Endocrinol Met: TEM 2013; 24:635-43; PMID:24182456; http://dx.doi.org/10.1016/j.tem.2013.09.004
- Brault JJ, Dohm GL, Houmard JA. Twenty-two skeletal muscle metabolism and obesity. Handbook Obesity: Epidemol, Etiol, Physiopathol 2014; 1:249; http://dx.doi.org/10.1201/b16473-25
- Richter EA, Hargreaves M. Exercise, GLUT4, and skeletal muscle glucose uptake. Physiol Rev 2013; 93:993-1017; PMID:23899560; http://dx.doi.org/10.1152/physrev.00038.2012
- JorgensenO’Neill HM. AMPK and exercise: glucose uptake and insulin sensitivity. Diabetes Met J 2013; 37:1-21; http://dx.doi.org/10.4093/dmj.2013.37.1.1
- Friedrichsen M, Mortensen B, Pehmoller C, Birk JB, Wojtaszewski JF. Exercise-induced AMPK activity in skeletal muscle: role in glucose uptake and insulin sensitivity. Mol Cell Endocrinol 2013; 366:204-14; PMID:22796442; http://dx.doi.org/10.1016/j.mce.2012.06.013
- Barnes BR, Marklund S, Steiler TL, Walter M, Hjalm G, Amarger V, Mahlapuu M, Leng Y, Johansson C, Galuska D, et al. The 5′-AMP-activated protein kinase gamma3 isoform has a key role in carbohydrate and lipid metabolism in glycolytic skeletal muscle. J Biol Chem 2004; 279:38441-7; PMID:15247217; http://dx.doi.org/10.1074/jbc.M405533200
- Jorgensen SB, Viollet B, Andreelli F, Frosig C, Birk JB, Schjerling P, Vaulont S, Richter EA, Wojtaszewski JF. Knockout of the alpha2 but not alpha1 5’-AMP-activated protein kinase isoform abolishes 5-aminoimidazole-4-carboxamide-1-beta-4-ribofuranosidebut not contraction-induced glucose uptake in skeletal muscle. J Biol Chem 2004; 279:1070-9; PMID:14573616; http://dx.doi.org/10.1074/jbc.M306205200
- Steinberg GR, O’Neill HM, Dzamko NL, Galic S, Naim T, Koopman R, Jorgensen SB, Honeyman J, Hewitt K, Chen ZP, et al. Whole body deletion of AMP-activated protein kinase {beta}2 reduces muscle AMPK activity and exercise capacity. J Biol Chem 2010; 285:37198-209; PMID:20855892; http://dx.doi.org/10.1074/jbc.M110.102434
- Dasgupta B, Ju JS, Sasaki Y, Liu X, Jung SR, Higashida K, Lindquist D, Milbrandt J. The AMPK beta2 subunit is required for energy homeostasis during metabolic stress. Mol Cell Biol 2012; 32:2837-48; PMID:22586267; http://dx.doi.org/10.1128/MCB.05853-11
- O’Neill HM, Maarbjerg SJ, Crane JD, Jeppesen J, Jorgensen SB, Schertzer JD, Shyroka O, Kiens B, van Denderen BJ, Tarnopolsky MA, et al. AMP-activated protein kinase (AMPK) beta1beta2 muscle null mice reveal an essential role for AMPK in maintaining mitochondrial content and glucose uptake during exercise. Proc Nat Acad Sci U S A 2011; 108:16092-7; http://dx.doi.org/10.1073/pnas.1105062108
- Merry TL, Steinberg GR, Lynch GS, McConell GK. Skeletal muscle glucose uptake during contraction is regulated by nitric oxide and ROS independently of AMPK. Am J Physiol Endocrinol Met 2010; 298:E577-85; PMID:20009026; http://dx.doi.org/10.1152/ajpendo.00239.2009
- Hadley RD, Whaley JW, Askins DG, Jr. Treatment of type 2 diabetes: a review of metformin in clinical practice. J South Carolina Med Assoc 1998; 94:12-5.
- Dunn CJ, Peters DH. Metformin. A review of its pharmacological properties and therapeutic use in non-insulin-dependent diabetes mellitus. Drugs 1995; 49:721-49; PMID:7601013; http://dx.doi.org/10.2165/00003495-199549050-00007
- Franciosi M, Lucisano G, Lapice E, Strippoli GF, Pellegrini F, Nicolucci A. Metformin therapy and risk of cancer in patients with type 2 diabetes: systematic review. PloS One 2013; 8:e71583; PMID:23936520; http://dx.doi.org/10.1371/journal.pone.0071583
- Dolan PL, Tapscott EB, Dorton PJ, Dohm GL. Contractile activity restores insulin responsiveness in skeletal muscle of obese Zucker rats. Biochem J 1993; 289 (Pt 2):423-6; PMID:8424787
- Azevedo JL Jr, Carey JO, Pories WJ, Morris PG, Dohm GL. Hypoxia stimulates glucose transport in insulin-resistant human skeletal muscle. Diabetes 1995; 44:695-8; PMID:7789635; http://dx.doi.org/10.2337/diab.44.6.695
- Blaak EE. Basic disturbances in skeletal muscle fatty acid metabolism in obesity and type 2 diabetes mellitus. Proc Nutr Soc 2004; 63:323-30; PMID:15294050; http://dx.doi.org/10.1079/PNS2004361
- Wells GD, Noseworthy MD, Hamilton J, Tarnopolski M, Tein I. Skeletal muscle metabolic dysfunction in obesity and metabolic syndrome. Can J Neurol Sci Le J Can Des Sci Neurologiques 2008; 35:31-40; PMID:18380275
- Katsanos CS, Mandarino LJ. Protein metabolism in human obesity: a shift in focus from whole-body to skeletal muscle. Obesity 2011; 19:469-75; PMID:21164506; http://dx.doi.org/10.1038/oby.2010.290
- Pal M, Febbraio MA, Whitham M. From cytokine to myokine: the emerging role of interleukin-6 in metabolic regulation. Immunol Cell Biol 2014; 92:331-9; PMID:24751614; http://dx.doi.org/10.1038/icb.2014.16
- Eckardt K, Gorgens SW, Raschke S, Eckel J. Myokines in insulin resistance and type 2 diabetes. Diabetologia 2014; 57:1087-99; PMID:24676645; http://dx.doi.org/10.1007/s00125-014-3224-x
- Raschke S, Eckel J. Adipo-myokines: two sides of the same coin–mediators of inflammation and mediators of exercise. Mediat Inflamm 2013; 2013:320724; PMID:23861558; http://dx.doi.org/10.1155/2013/320724
- Pedersen L, Hojman P. Muscle-to-organ cross talk mediated by myokines. Adipocyte 2012; 1:164-7; PMID:23700527; http://dx.doi.org/10.4161/adip.20344
- Teufel A, Malik N, Mukhopadhyay M, Westphal H. Frcp1 and Frcp2, two novel fibronectin type III repeat containing genes. Gene 2002; 297:79-83; PMID:12384288; http://dx.doi.org/10.1016/S0378-1119(02)00828-4
- Ferrer-Martinez A, Ruiz-Lozano P, Chien KR. Mouse PeP: a novel peroxisomal protein linked to myoblast differentiation and development. Dev Dyn: An Off Pub Am Assoc Anatomists 2002; 224:154-67; PMID:12112469; http://dx.doi.org/10.1002/dvdy.10099
- Huh JY, Panagiotou G, Mougios V, Brinkoetter M, Vamvini MT, Schneider BE, Mantzoros CS. FNDC5 and irisin in humans: I. Predictors of circulating concentrations in serum and plasma and II. mRNA expression and circulating concentrations in response to weight loss and exercise. Metab: Clin Exp 2012; 61:1725-38; PMID:23018146; http://dx.doi.org/10.1016/j.metabol.2012.09.002
- Wrann CD, White JP, Salogiannnis J, Laznik-Bogoslavski D, Wu J, Ma D, Lin JD, Greenberg ME, Spiegelman BM. Exercise induces hippocampal BDNF through a PGC-1alphaFNDC5 pathway. Cell Metab 2013; 18:649-59; PMID:24120943; http://dx.doi.org/10.1016/j.cmet.2013.09.008
- Bostrom P, Wu J, Jedrychowski MP, Korde A, Ye L, Lo JC, Rasbach KA, Bostrom EA, Choi JH, Long JZ, et al. A PGC1-alpha-dependent myokine that drives brown-fat-like development of white fat and thermogenesis. Nature 2012; 481:463-8; PMID:22237023; http://dx.doi.org/10.1038/nature10777
- Zhang Y, Li R, Meng Y, Li S, Donelan W, Zhao Y, Qi L, Zhang M, Wang X, Cui T, et al. Irisin stimulates browning of white adipocytes through mitogen-activated protein kinase p38 MAP kinase and ERK MAP kinase signaling. Diabetes 2014; 63:514-25; PMID:24150604; http://dx.doi.org/10.2337/db13-1106
- Pedersen BK. Exercise-induced myokines and their role in chronic diseases. Brain, Behav, Immun 2011; 25:811-6; PMID:21354469; http://dx.doi.org/10.1016/j.bbi.2011.02.010
- Keipert S, Ost M, Johann K, Imber F, Jastroch M, van Schothorst EM, Keijer J, Klaus S. Skeletal muscle mitochondrial uncoupling drives endocrine cross-talk through the induction of FGF21 as a myokine. Am J Physiol Endocrinol Metab 2014; 306:E469-82; PMID:24347058; http://dx.doi.org/10.1152/ajpendo.00330.2013
- Lee P, Linderman JD, Smith S, Brychta RJ, Wang J, Idelson C, Perron RM, Werner CD, Phan GQ, Kammula US, et al. Irisin and FGF21 are cold-induced endocrine activators of brown fat function in humans. Cell Metab 2014; 19:302-9; PMID:24506871; http://dx.doi.org/10.1016/j.cmet.2013.12.017
- Vaughan RA, Gannon NP, Barberena MA, Garcia-Smith R, Bisoffi M, Mermier CM, Conn CA, Trujillo KA. Characterization of the metabolic effects of Irisin on skeletal muscle in vitro. Diabetes, Obes Metab 2014; 16:711-8; PMID:24476050; http://dx.doi.org/10.1111/dom.12268
- Erickson HP. Irisin and FNDC5 in retrospect: an exercise hormone or a transmembrane receptor? Adipocyte 2013; 2:289-93; PMID:24052909; http://dx.doi.org/10.4161/adip.26082
- Sanchis-Gomar F, Alis R, Pareja-Galeano H, Romagnoli M, Perez-Quilis C. Inconsistency in circulating irisin levels: what is really happening? Horm Metab Res Hormon- und Stoffwechselforschung 1501; Horm et Metab 2014.
- Norheim F, Langleite TM, Hjorth M, Holen T, Kielland A, Stadheim HK, Gulseth HL, Birkeland KI, Jensen J, Drevon CA. The effects of acute and chronic exercise on PGC-1alpha, irisin and browning of subcutaneous adipose tissue in humans. FEBS J 2014; 281:739-49; PMID:24237962; http://dx.doi.org/10.1111/febs.12619
- Kurdiova T, Balaz M, Vician M, Maderova D, Vlcek M, Valkovic L, Srbecky M, Imrich R, Kyselovicova O, Belan V, et al. Are skeletal muscle & adipose tissue Fndc5 gene expression and irisin release affected by obesity, diabetes and exercise? in vivo & in vitro studies. Journal of Physiol 2014; 592:1091-107; PMID:24297848; http://dx.doi.org/10.1113/jphysiol.2013.264655
- Crujeiras AB, Zulet MA, Lopez-Legarrea P, de la Iglesia R, Pardo M, Carreira MC, Martinez JA, Casanueva FF. Association between circulating irisin levels and the promotion of insulin resistance during the weight maintenance period after a dietary weight-lowering program in obese patients. Metab: Clin Exp 2013; 63:520-31; PMID:24439241; http://dx.doi.org/10.1016/j.metabol.2013.12.007
- Raschke S, Elsen M, Gassenhuber H, Sommerfeld M, Schwahn U, Brockmann B, Jung R, Wisloff U, Tjonna AE, Raastad T, et al. Evidence against a beneficial effect of irisin in humans. PloS One 2013; 8:e73680; PMID:24040023; http://dx.doi.org/10.1371/journal.pone.0073680
- Bostrom PA, Fernandez-Real JM, Mantzoros C. Irisin in humans: recent advances and questions for future research. Metab: Clin Exp 2014; 63:178-80; PMID:24342075; http://dx.doi.org/10.1016/j.metabol.2013.11.009
- McPherron AC, Lee SJ. Double muscling in cattle due to mutations in the myostatin gene. Proc Nat Acad Sci U S A 1997; 94:12457-61; PMID:9356471; http://dx.doi.org/10.1073/pnas.94.23.12457
- Grobet L, Martin LJ, Poncelet D, Pirottin D, Brouwers B, Riquet J, Schoeberlein A, Dunner S, Menissier F, Massabanda J, et al. A deletion in the bovine myostatin gene causes the double-muscled phenotype in cattle. Nat Genet 1997; 17:71-4; PMID:9288100; http://dx.doi.org/10.1038/ng0997-71
- Schuelke M, Wagner KR, Stolz LE, Hubner C, Riebel T, Komen W, Braun T, Tobin JF, Lee SJ. Myostatin mutation associated with gross muscle hypertrophy in a child. New Eng J med 2004; 350:2682-8; PMID:15215484; http://dx.doi.org/10.1056/NEJMoa040933
- Kawada S, Tachi C, Ishii N. Content and localization of myostatin in mouse skeletal muscles during aging, mechanical unloading and reloading. J Muscle Res Cell Motil 2001; 22:627-33; PMID:12222823; http://dx.doi.org/10.1023/A:1016366409691
- Buehring B, Binkley N. Myostatin–the holy grail for muscle, bone, and fat? Curr Osteoporosis Rep 2013; 11:407-14; PMID:24072591; http://dx.doi.org/10.1007/s11914-013-0160-5
- Guo T, Jou W, Chanturiya T, Portas J, Gavrilova O, McPherron AC. Myostatin inhibition in muscle, but not adipose tissue, decreases fat mass and improves insulin sensitivity. PloS One 2009; 4:e4937; PMID:19295913; http://dx.doi.org/10.1371/journal.pone.0004937
- McPherron AC, Lee SJ. Suppression of body fat accumulation in myostatin-deficient mice. J Clin Invest 2002; 109:595-601; PMID:11877467; http://dx.doi.org/10.1172/JCI0213562
- Shan T, Liang X, Bi P, Kuang S. Myostatin knockout drives browning of white adipose tissue through activating the AMPK-PGC1alpha-Fndc5 pathway in muscle. FASEB J: Off Pub Fed Am Soc Exp Biol 2013; 27:1981-9; PMID:23362117; http://dx.doi.org/10.1096/fj.12-225755
- Allen DL, Cleary AS, Speaker KJ, Lindsay SF, Uyenishi J, Reed JM, Madden MC, Mehan RS. Myostatin, activin receptor IIb, and follistatin-like-3 gene expression are altered in adipose tissue and skeletal muscle of obese mice. Am J Physiol Endocrinol Metab 2008; 294:E918-27; PMID: 18334608; http://dx.doi.org/10.1152/ajpendo.00798.2007
- Rebbapragada A, Benchabane H, Wrana JL, Celeste AJ, Attisano L. Myostatin signals through a transforming growth factor beta-like signaling pathway to block adipogenesis. Mol Cell Biol 2003; 23:7230-42; PMID:14517293; http://dx.doi.org/10.1128/MCB.23.20.7230-7242.2003
- Allen DL, Hittel DS, McPherron AC. Expression and function of myostatin in obesity, diabetes, and exercise adaptation. Med Sci Sport Exercise 2011; 43:1828-35; PMID:21364474; http://dx.doi.org/10.1249/MSS.0b013e3182178bb4
- Roth SM, Martel GF, Ferrell RE, Metter EJ, Hurley BF, Rogers MA. Myostatin gene expression is reduced in humans with heavy-resistance strength training: a brief communication. Exp Biol Med 2003; 228:706-9; PMID:12773702
- Konopka AR, Douglass MD, Kaminsky LA, Jemiolo B, Trappe TA, Trappe S, Harber MP. Molecular adaptations to aerobic exercise training in skeletal muscle of older women. J Gerontol Series A, Biol Sci Med Sci 2010; 65:1201-7; PMID:20566734; http://dx.doi.org/10.1093/gerona/glq109
- Attie KM, Borgstein NG, Yang Y, Condon CH, Wilson DM, Pearsall AE, Kumar R, Willins DA, Seehra JS, Sherman ML. A single ascending-dose study of muscle regulator ACE-031 in healthy volunteers. Muscle & Nerve 2013; 47:416-23; PMID:23169607; http://dx.doi.org/10.1002/mus.23539
- Keller C, Steensberg A, Pilegaard H, Osada T, Saltin B, Pedersen BK, Neufer PD. Transcriptional activation of the IL-6 gene in human contracting skeletal muscle: influence of muscle glycogen content. FASEB J: Off Pub Fed Am Soc Exp Biol 2001; 15:2748-50; PMID:11687509
- Spangenburg EE, Booth FW. Leukemia inhibitory factor restores the hypertrophic response to increased loading in the LIF(–) mouse. Cytokine 2006; 34:125-30; PMID:16781162; http://dx.doi.org/10.1016/j.cyto.2006.05.001
- Serrano AL, Baeza-Raja B, Perdiguero E, Jardi M, Munoz-Canoves P. Interleukin-6 is an essential regulator of satellite cell-mediated skeletal muscle hypertrophy. Cell Metab 2008; 7:33-44; PMID:18177723; http://dx.doi.org/10.1016/j.cmet.2007.11.011
- Carey AL, Steinberg GR, Macaulay SL, Thomas WG, Holmes AG, Ramm G, Prelovsek O, Hohnen-Behrens C, Watt MJ, James DE, et al. Interleukin-6 increases insulin-stimulated glucose disposal in humans and glucose uptake and fatty acid oxidation in vitro via AMP-activated protein kinase. Diabetes 2006; 55:2688-97; PMID:17003332; http://dx.doi.org/10.2337/db05-1404
- Bastard JP, Maachi M, Van Nhieu JT, Jardel C, Bruckert E, Grimaldi A, Robert JJ, Capeau J, Hainque B. Adipose tissue IL-6 content correlates with resistance to insulin activation of glucose uptake both in vivo and in vitro. J Clin Endocrinol Metab 2002; 87:2084-9; PMID:11994345; http://dx.doi.org/10.1210/jcem.87.5.8450
- Tsigos C, Papanicolaou DA, Kyrou I, Defensor R, Mitsiadis CS, Chrousos GP. Dose-dependent effects of recombinant human interleukin-6 on glucose regulation. J Clin Endocrinol Metab 1997; 82:4167-70; PMID:9398733; http://dx.doi.org/10.1210/jcem.82.12.4422
- Rotter V, Nagaev I, Smith U. Interleukin-6 (IL-6) induces insulin resistance in 3T3-L1 adipocytes and is, like IL-8 and tumor necrosis factor-alpha, overexpressed in human fat cells from insulin-resistant subjects. J Biol Chem 2003; 278:45777-84; PMID:12952969; http://dx.doi.org/10.1074/jbc.M301977200
- Fontana L, Eagon JC, Trujillo ME, Scherer PE, Klein S. Visceral fat adipokine secretion is associated with systemic inflammation in obese humans. Diabetes 2007; 56:1010-3; PMID:17287468; http://dx.doi.org/10.2337/db06-1656
- Golbidi S, Laher I. Exercise induced adipokine changes and the metabolic syndrome. J Diabetes Res 2014; 2014:726861; PMID:24563869; http://dx.doi.org/10.1155/2014/726861
- Quinn LS. Interleukin-15: a muscle-derived cytokine regulating fat-to-lean body composition. J Anim Sci 2008; 86:E75-83; PMID:17709786; http://dx.doi.org/10.2527/jas.2007-0458
- Zoladz JA, Pilc A. The effect of physical activity on the brain derived neurotrophic factor: from animal to human studies. J Physiol Pharmacol: An Off J Polish Physiol Soc 2010; 61:533-41; PMID:21081796
- Broholm C, Laye MJ, Brandt C, Vadalasetty R, Pilegaard H, Pedersen BK, Scheele C. LIF is a contraction-induced myokine stimulating human myocyte proliferation. J Appl Physiol 2011; 111:251-9; PMID:21527666; http://dx.doi.org/10.1152/japplphysiol.01399.2010
- Laviola L, Natalicchio A, Giorgino F. The IGF-I signaling pathway. Curr Pharm Des 2007; 13:663-9; PMID: 17346182; http://dx.doi.org/10.2174/138161207780249146
- Barton ER, Park S, James JK, Makarewich CA, Philippou A, Eletto D, Lei H, Brisson B, Ostrovsky O, Li Z, et al. Deletion of muscle GRP94 impairs both muscle and body growth by inhibiting local IGF production. FASEB J: Off Pub Fed Am Soc Exp Biol 2012; 26:3691-702; PMID:22649033; http://dx.doi.org/10.1096/fj.11-203026
- Sell H, Dietze-Schroeder D, Kaiser U, Eckel J. Monocyte chemotactic protein-1 is a potential player in the negative cross-talk between adipose tissue and skeletal muscle. Endocrinology 2006; 147:2458-67; PMID:16439461; http://dx.doi.org/10.1210/en.2005-0969
- Yadav A, Saini V, Arora S. MCP-1: chemoattractant with a role beyond immunity: a review. Clinica Chimica Acta; Int J Clin Chem 2010; 411:1570-9; PMID:20633546; http://dx.doi.org/10.1016/j.cca.2010.07.006
- Seldin MM, Peterson JM, Byerly MS, Wei Z, Wong GW. Myonectin (CTRP15), a novel myokine that links skeletal muscle to systemic lipid homeostasis. J Biol Chem 2012; 287:11968-80; PMID:22351773; http://dx.doi.org/10.1074/jbc.M111.336834
- Lim S, Choi SH, Koo BK, Kang SM, Yoon JW, Jang HC, Choi SM, Lee MG, Lee W, Shin H, et al. Effects of aerobic exercise training on C1q tumor necrosis factor alpha-related protein isoform 5 (myonectin): association with insulin resistance and mitochondrial DNA density in women. J Clin Endocrinol Metab 2012; 97:E88-93; PMID:22031510; http://dx.doi.org/10.1210/jc.2011-1743
- Lafortuna CL, Maffiuletti NA, Agosti F, Sartorio A. Gender variations of body composition, muscle strength and power output in morbid obesity. Int J Obes 2005; 29:833-41; PMID:15917862; http://dx.doi.org/10.1038/sj.ijo.0802955
- Sakuma K, Yamaguchi A. Sarcopenic obesity and endocrinal adaptation with age. Int J Endocrinol 2013; 2013:204164; PMID:23690769; http://dx.doi.org/10.1155/2013/204164
- Brady AO, Straight CR, Evans EM. Body Composition, Muscle Capacity and Physical Function in Older Adults: An Integrated Conceptual Model. J Aging Phys Activ 2013; PMID:23945551
- Yamada M, Moriguch Y, Mitani T, Aoyama T, Arai H. Age-dependent changes in skeletal muscle mass and visceral fat area in Japanese adults from 40 to 79 years-of-age. Geriatr Gerontol Int 2014; 14 Suppl 1:8-14; PMID:24450556; http://dx.doi.org/10.1111/ggi.12209
- Schaap LA, Koster A, Visser M. Adiposity, muscle mass, and muscle strength in relation to functional decline in older persons. Epidemiol Rev 2012; PMID:23221972
- Sen B, Guilluy C, Xie Z, Case N, Styner M, Thomas J, Oguz I, Rubin C, Burridge K, Rubin J. Mechanically induced focal adhesion assembly amplifies anti-adipogenic pathways in mesenchymal stem cells. Stem Cells 2011; 29:1829-36; PMID:21898699; http://dx.doi.org/10.1002/stem.732
- Menuki K, Mori T, Sakai A, Sakuma M, Okimoto N, Shimizu Y, Kunugita N, Nakamura T. Climbing exercise enhances osteoblast differentiation and inhibits adipogenic differentiation with high expression of PTHPTHrP receptor in bone marrow cells. Bone 2008; 43:613-20; PMID:18567552; http://dx.doi.org/10.1016/j.bone.2008.04.022
- Bazzarre TL, Kleiner SM, Litchford MD. Nutrient intake, body fat, and lipid profiles of competitive male and female bodybuilders. J Am Coll Nutr 1990; 9:136-42; PMID:2338462; http://dx.doi.org/10.1080/07315724.1990.10720362
- Koohmaraie M, Shackelford SD, Wheeler TL, Lonergan SM, Doumit ME. A muscle hypertrophy condition in lamb (callipyge): characterization of effects on muscle growth and meat quality traits. J Anim Sci 1995; 73:3596-607; PMID:8655433
- Dube JJ, Amati F, Stefanovic-Racic M, Toledo FG, Sauers SE, Goodpaster BH. Exercise-induced alterations in intramyocellular lipids and insulin resistance: the athlete's paradox revisited. Am J Physiol Endocrinol Metab 2008; 294:E882-8; PMID:18319352; http://dx.doi.org/10.1152/ajpendo.00769.2007
- Tarnopolsky MA, Rennie CD, Robertshaw HA, Fedak-Tarnopolsky SN, Devries MC, Hamadeh MJ. Influence of endurance exercise training and sex on intramyocellular lipid and mitochondrial ultrastructure, substrate use, and mitochondrial enzyme activity. Am J Physiol Regul, Integr Comp Physiol 2007; 292:R1271-8; PMID:17095651; http://dx.doi.org/10.1152/ajpregu.00472.2006
- van Loon LJ, Koopman R, Manders R, van der Weegen W, van Kranenburg GP, Keizer HA. Intramyocellular lipid content in type 2 diabetes patients compared with overweight sedentary men and highly trained endurance athletes. Am J Physiol Endocrinol Metab 2004; 287:E558-65; PMID:15165998; http://dx.doi.org/10.1152/ajpendo.00464.2003
- Badin PM, Langin D, Moro C. Dynamics of skeletal muscle lipid pools. Trends Endocrinology Metab: TEM 2013; 24:607-15; PMID:23988586; http://dx.doi.org/10.1016/j.tem.2013.08.001
- Goodpaster BH, He J, Watkins S, Kelley DE. Skeletal muscle lipid content and insulin resistance: evidence for a paradox in endurance-trained athletes. J Clin Endocrinol Metab 2001; 86:5755-61; PMID:11739435; http://dx.doi.org/10.1210/jcem.86.12.8075
- van Loon LJ. Use of intramuscular triacylglycerol as a substrate source during exercise in humans. J Appl Physiol 2004; 97:1170-87; PMID:15358749; http://dx.doi.org/10.1152/japplphysiol.00368.2004
- Romacho T, Elsen M, Rohrborn D, Eckel J. Adipose tissue and its role in organ crosstalk. Acta Physiologica 2014; PMID:24495317
- Kwon H, Pessin JE. Adipokines mediate inflammation and insulin resistance. Front Endocrinol 2013; 4:71; PMID:23781214; http://dx.doi.org/10.3389/fendo.2013.00071
- Sanchez J, Nozhenko Y, Palou A, Rodriguez AM. Free fatty acid effects on myokine production in combination with exercise mimetics. Mol Nutr Food Res 2013; 57:1456-67; PMID:23650203; http://dx.doi.org/10.1002/mnfr.201300126
- Akhmedov D, Berdeaux R. The effects of obesity on skeletal muscle regeneration. Front Physiol 2013; 4:371; PMID:24381559; http://dx.doi.org/10.3389/fphys.2013.00371
- Fiaschi T, Magherini F, Gamberi T, Modesti PA, Modesti A. Adiponectin as a tissue regenerating hormone: more than a metabolic function. Cell Mol Life Sci: CMLS 2013; PMID:24322911