Abstract
Recent data indicate that cell size fluctuation, a key property in adipocyte pathophysiology primarily dependent on lipid storage, is linked to a novel function of lipid droplet organelles acting as mechano-active organelles to regulate cell membrane remodeling and caveolae dynamics.
Adipose tissue is the primary energy storage organ in mammals, and as such, has evolved as a highly efficient and flexible metabolic tissue. Because nutrient availability largely fluctuates, adipose tissue fat stores appropriately provide a continuous energy supply. In this context, the molecular form in which energy is stored within adipose tissue (i.e. esterified fatty acids) ensures optimal packing in neutral lipids within lipid droplet organelles. This lipid storage form provides a highly efficient volume sparing process, over water-dependent storage of carbohydrates as glycogen. During evolution, periods of famine and nutrient scarcity favored adaptive pathways toward optimal filling of adipose stores in prosperous conditions. These include insulin-driven channeling of nutrients to adipose tissue lipids by means of integrated multi-level regulations involving gene transcription, protein synthesis, as well as post transcriptional modifications toward regulated protein abundance, activities and interactions with functional partners. Another important aspect in adipose tissue function is limited basal release of lipids by appropriate coating of lipid droplets to avoid uneven lipolytic mobilization.
Beyond metabolic responses, highly efficient adipocyte lipid storage processes also involve adaptations in cell architecture, since lipid stores in fat cells dominate over other intracellular compartments. Indeed, rapid lipid accretion results in large-scale intracellular volume changes, and requires concomitant adaptive responses by the cell membrane. Conversely, extensive mobilization of stored lipids from adipocytes requires bilayer remodeling to prevent an excess of membrane surface area. These cellular aspects are poorly documented, as the adipocyte is a difficult cell type to apply common cellular biology tools. Physiologists have long observed huge variations in adipose cell size upon fasting-refeeding in rodent models or in humans with extreme leanness or obesity. For example, a 48h-fast in mice can induce an almost complete disappearance of lipids from adipocytes. Depending upon whether an individual is lean or obese, the diameter of a single human adipocyte varies from 70 to 120 μm, which affects cell surface area by nearly 4-fold. Therefore, a major component of metabolic flexibility in adipose tissue is the ability of the adipocyte to respond to volume changes linked to the fluctuations of lipid stores. In the long term, formation of additional lipid reservoirs by means of differentiation of new adipocytes will be required to cope with sustained energy excess.
The exploration of cell responses to size changes and the subsequent physiological consequences primarily rely on manipulation of osmolarity in the cell environment to alter volume.Citation1 When faced with a hypotonic extracellular medium, cells first respond by swelling followed by the so-called “Regulatory Volume Decrease (RVD)” process to recover their original cellular size. Cell swelling has been reported to occur during different clinical situations such as hypoxia, ischemia, hyponatremia, hypothermia, and during intracellular acidosis and diabetic ketoacidosis.Citation1 Therefore osmosensing or osmosignaling is the subject of extensive research. In particular, some TRP (Transient Receptor Potential) channels have been identified as osmosensors involved in controlling the RVD process.Citation2,3
The regulation of cell volume after osmotic changes is similar to that of the response of the adipocyte to changes in lipid store: the mechanical stretching of the lipid bilayer is transiently applied in both situations, potentially threatening cell integrity. Very little is known about the role of TPR channels and associated-osmolyte changes in maintaining energy homeostasis.Citation4 Of interest, one particular TPR, TPRV4, shown to be activated by cellular swellingCitation5,6 and by cellular stretch,Citation7,8 was identified as a negative regulator of adipocyte oxidative metabolism and inflammation.Citation9 Therefore, it is possible that TPRV4 might be a link between adipocyte distension in obesity and cellular dysfunction. In the same line, cell swelling has been reported to directly activate NLRP3 inflammasome in macrophages.Citation10
Apart from ion channels, caveolae are other cell membrane components shown to respond to stretch or swelling-related mechanical stress.Citation11 Caveolae are organized on the cell surface as small flask-shaped invaginations and were shown to disassemble upon membrane stretching, providing a lipid reservoir for membrane extension. Indeed, a specific lipid composition enriched in tightly packed phospholipid/cholesterol molecules resembling lipid rafts is a hallmark of caveolar membranes.Citation12
Because caveolae are particularly abundant structures in adipocytes estimated to cover nearly 30% of the cell surface, and caveolins, which form the caveolar protein coats, are highly induced during adipocyte differentiation, caveolae represent good candidate mediators in lipid-driven fat cell size adaptation. In a recently published paper,Citation13 we provide experimental evidence for this hypothesis. By using retroviral-based stable transfection of caveolin-1 in the well-characterized adipose 3T3-L1 and 3T3F442A cell lines, we produced adipocytes that had higher than normal cell surface caveolae density, which enabled the accommodation of larger intracellular lipid droplets. Furthermore, taking advantage of the ability of 3T3 preadipocytes to drive exogenous formation of adipose tissue in mice, it was confirmed that subcutaneous injection of caveolin-overexpressing preadipocytes could generate fat pads containing larger adipocytes, indicating that caveolin/caveolae are rate limiting for adipocyte enlargement. These findings can also be translated in human physiology. It is well known that adipose tissue expansion can proceed from hyperplasic formation of new adipocytes or hypertrophic growth of existing adipose cells. By examining the response of healthy subjects to a controlled overfeeding trial, it was found that among individuals who responded to overfeeding by adipocyte hypertrophy, caveolin-1 expression at baseline significantly correlated with the degree of adipocyte enlargement. In addition, adipocyte shrinkage due to extensive lipid mobilization was also examined. In particular, prolonged treatment of cell lines in vitro with a lipolytic agent (8Br-cAMP) was found to induce lipid emptying of adipocytes and caveolae disassembly from the cell surface. Similarly, long-term fasting in mice was found to associate with massive lipid mobilization and a reduction in adipocyte caveolae number. Caveolar membrane remodeling was shown to rely on selective destabilization and targeted protein degradation of cavin proteins, which are caveolae adaptors previously identified to critically regulate caveolae formation.Citation14-16
Altogether, these data argue for a role of adipocyte caveolae dynamics in metabolic flexibility and adaptation to lipid store fluctuations (). By identifying the importance of caveolae structures, which are known to respond to mechanical stress in other cell types, this study suggests that adipocyte lipid droplets act as mechano-active intracellular organelles, adding a novel item in the fast-growing list of lipid droplet organelle functions.Citation18 Also, the presence of caveolin proteins onto adipocyte lipid droplets, which are in close vicinity with plasma membrane, has previously been reported.Citation17 Caveolin-deficient mouse models have been shown to display marked insulin-resistance, which has led to the hypothesis that caveolae might serve in the transmission of the insulin signal, as well as vascular dysfunction (reviewed inCitation19). Caveolae-deficient mice also exhibit other adipocyte abnormalities, including impaired lipid storage,Citation20 augmented adipocyte autophagy,Citation21 and metabolic inflexibility.Citation22 Moreover, tissue-specific caveolin reintroduction demonstrated a central contribution of adipocyte caveolae in metabolic dysfunction.Citation23 Accordingly, loss of function mutations in genes coding for caveolar associated-proteins in patients primarily cause lipoatrophic syndromes.Citation24-26 These new data therefore highlight the interweaving of adipocyte metabolic flexibility, insulin response, and caveolae dynamics in adipose tissue physiology.
Figure 1. Caveolae dynamics links to lipid store fluctuations in adipocytes. Fully mature adipocytes engineered for a high caveolae density favor lipid droplet expandability and lipid storage (left). Conversely, forced lipid droplet shrinkage and extensive mobilization induces caveolae disassembly (right). Caveolae are represented as membrane invaginations in cell membrane, cell nucleus is shown in gray, lipid droplets are yellow.
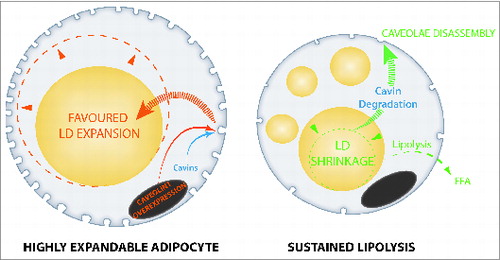
Disclosure of Potential Conflicts of Interest
No potential conflicts of interest were disclosed.
Acknowledgments
We thank Brandon D Kayser for reading the manuscript.
Funding
Funding by EU-FP7 (LipidomicNet-202272) is acknowledged.
References
- Hoffmann EKL. Physiology of cell volume regulation in vertebrates. Physiol Rev 2009; 89:193-277; PMID:19126758; http://dx.doi.org/10.1152/physrev.00037.2007
- Liedtke W. Transient receptor potential vanilloid channels functioning in transduction of osmotic stimuli. J Endocrinol 2006; 191:515-23; PMID:17170210; http://dx.doi.org/10.1677/joe.1.07000
- Muraki K, Iwata Y, Katanosaka Y, Ito T, Ohya S, Shigekawa M, Imaizumi Y. TRPV2 is a component of osmotically sensitive cation channels in murine aortic myocytes. Circ Res 2003; 93:829-38; PMID:14512441; http://dx.doi.org/10.1161/01.RES.0000097263.10220.0C
- Ahern GP. Transient receptor potential channels and energy homeostasis. Trends Endocrinol Metab 2013; 24:554-60; PMID:23891326; http://dx.doi.org/10.1016/j.tem.2013.06.005
- Liedtke W, Choe Y, Marti-Renom MA, Bell AM, Denis CS, Sali A, Hudspeth AJ, Friedman JM, Heller S. Vanilloid receptor-related osmotically activated channel (VR-OAC), a candidate vertebrate osmoreceptor. Cell 2000; 103:525-35; PMID:11081638; http://dx.doi.org/10.1016/S0092-8674(00)00143-4
- Strotmann R, Harteneck C, Nunnenmacher K, Schultz G, Plant TD. OTRPC4, a nonselective cation channel that confers sensitivity to extracellular osmolarity. Nat Cell Biol 2000; 2:695-702; PMID:11025659; http://dx.doi.org/10.1038/35036318
- Mochizuki T, Sokabe T, Araki I, Fujishita K, Shibasaki K, Uchida K, Naruse K, Koizumi S, Takeda M, Tominaga M. The TRPV4 cation channel mediates stretch-evoked Ca2+ influx and ATP release in primary urothelial cell cultures. J Biol Chem 2009; 284:21257-64; PMID:19531473; http://dx.doi.org/10.1074/jbc.M109.020206
- Thodeti CK, Matthews B, Ravi A, Mammoto A, Ghosh K, Bracha AL, Ingber DE. TRPV4 channels mediate cyclic strain-induced endothelial cell reorientation through integrin-to-integrin signaling. Circ Res 2009; 104:1123-30; PMID:19359599; http://dx.doi.org/10.1161/CIRCRESAHA.108.192930
- Ye L, Kleiner S, Wu J, Sah R, Gupta RK, Banks AS, Cohen P, Khandekar MJ, Boström P, Mepani RJ, et al. TRPV4 is a regulator of adipose oxidative metabolism, inflammation, and energy homeostasis. Cell 2012; 151:96-110; PMID:23021218; http://dx.doi.org/10.1016/j.cell.2012.08.034
- Compan V, Baroja-Mazo A, Lopez-Castejon G, Gomez AI, Martínez CM, Angosto D, Montero MT, Herranz AS, Bazán E, Reimers D, et al. Cell volume regulation modulates NLRP3 inflammasome activation. Immunity 2012; 37:487-500; PMID:22981536; http://dx.doi.org/10.1016/j.immuni.2012.06.013
- Sinha B, Koster D, Ruez R, Gonnord P, Bastiani M, Abankwa D, Stan RV, Butler-Browne G, Vedie B, Johannes L, et al. Cells respond to mechanical stress by rapid disassembly of caveolae. Cell 2011; 144:402-13; PMID:21295700; http://dx.doi.org/10.1016/j.cell.2010.12.031
- Simons K, Toomre D. Lipids rafts in cells membranes. Nat Rev Mol Cell Biol 2000; 1:31-9; http://dx.doi.org/10.1038/35036052
- Briand N, Prado C, Mabilleau G, Lasnier F, Le Lièpvre X, Covington JD, Ravussin E, Le Lay S, Dugail I. Caveolin-1 expression and cavins stability regulate caveolae dynamics in adipocyte lipid store fluctuation. Diabetes 2014; in press; PMID:24969108
- Briand N, Dugail I, Le Lay S. Cavin proteins: New players in the caveolae field. Biochimie 2011; 93:71-7; PMID:20363285; http://dx.doi.org/10.1016/j.biochi.2010.03.022
- Hill MM, Bastiani M, Luetterforst R, Kirkham M, Kirkham A, Nixon SJ, Walser P, Abankwa D, Oorschot VM, Martin S, et al. PTRF-Cavin, a conserved cytoplasmic protein required for caveola formation and function. Cell 2008; 132:113-24; PMID:18191225; http://dx.doi.org/10.1016/j.cell.2007.11.042
- Liu L, Brown D, McKee M, Lebrasseur NK, Yang D, Albrecht KH, Ravid K, Pilch PF. Deletion of Cavin/PTRF causes global loss of caveolae, dyslipidemia, and glucose intolerance. Cell Metab 2008; 8:310-7; PMID:18840361; http://dx.doi.org/10.1016/j.cmet.2008.07.008
- Blouin CM, Le LS, Lasnier F, Dugail I, Hajduch E. Regulated association of caveolins to lipid droplets during differentiation of 3T3-L1 adipocytes. Biochem Biophys Res Commun 2008; 376:331-5; PMID:18783721; http://dx.doi.org/10.1016/j.bbrc.2008.08.154
- Farese RV, Jr., Walther TC. Lipid droplets finally get a little R-E-S-P-E-C-T. Cell 2009; 139:855-60; PMID:19945371; http://dx.doi.org/10.1016/j.cell.2009.11.005
- Le Lay S, Blouin M, Hajduch E, Dugail I. Filling up adipocytes with lipids. Lessons from caveolin-1 deficiency. Biochim Biophys Acta 2008; PMID:19038362; .
- Razani B, Combs TP, Wang XB, Frank PG, Park DS, Russell RG, Li M, Tang B, Jelicks LA, Scherer PE, et al. Caveolin-1-deficient mice are lean, resistant to diet-induced obesity, and show hypertriglyceridemia with adipocyte abnormalities. J Biol Chem 2002; 277:8635-47; PMID:11739396; http://dx.doi.org/10.1074/jbc.M110970200
- Le Lay S, Briand N, Blouin CM, Chateau D, Prado C, Lasnier F, Le Liepvre X, Hajduch E, Dugail I. The lipoatrophic caveolin-1 deficient mouse model reveals autophagy in mature adipocytes. Autophagy 2010; 6:754-63; PMID:20574167; http://dx.doi.org/10.4161/auto.6.6.12574
- Asterholm IW, Mundy DI, Weng J, Anderson RG, Scherer PE. Altered mitochondrial function and metabolic inflexibility associated with loss of caveolin-1. Cell Metab 2012; 15:171-85; PMID:22326219; http://dx.doi.org/10.1016/j.cmet.2012.01.004
- Briand N, Le Lay S, Sessa WC, Ferre P, Dugail I. Distinct roles of endothelial and adipocyte caveolin-1 in macrophage infiltration and adipose tissue metabolic activity. Diabetes 2011; 60:448-53; PMID:21270257; http://dx.doi.org/10.2337/db10-0856
- Kim CA, Delepine M, Boutet E, El Mourabit H, Le Lay S, Meier M, Nemani M, Bridel E, Leite CC, Bertola DR, et al. Association of a homozygous nonsense caveolin-1 mutation with Berardinelli-Seip congenital lipodystrophy. J Clin Endocrinol Metab 2008; 93:1129-34; PMID:18211975; http://dx.doi.org/10.1210/jc.2007-1328
- Hayashi YK, Matsuda C, Ogawa M, Goto K, Tominaga K, Mitsuhashi S, Park YE, Nonaka I, Hino-Fukuyo N, Haginoya K, et al. Human PTRF mutations cause secondary deficiency of caveolins resulting in muscular dystrophy with generalized lipodystrophy. J Clin Invest 2009; 119:2623-33; PMID:19726876; http://dx.doi.org/10.1172/JCI38660
- Shastry S, Delgado MR, Dirik E, Turkmen M, Agarwal AK, Garg A. Congenital generalized lipodystrophy, type 4 (CGL4) associated with myopathy due to novel PTRF mutations. Am J Med Genet A 2010; 152A:2245-53; PMID:20684003; http://dx.doi.org/10.1002/ajmg.a.33578