Abstract
Given similarities in metabolic parameters and cardiovascular physiology, the pig is well positioned as a biomedical model for metabolic disease and obesity in humans. Better understanding molecular mechanisms governing porcine adipocyte hyperplasia may provide insight into the regulation of adipose tissue development that is useful both when considering the pig as a commodity and when extrapolating porcine data to human disease. Primary cultures of pig stromal-vascular cells have served as a useful tool for investigating factors that regulate preadipocyte proliferation and differentiation. However, such cultures have generally been maintained at 37°C in vitro despite euthermia being 39°C in pigs. To address potential concerns about the physiological relevance of culturing primary pig preadipocytes under what would be hypothermic conditions in vivo, the objective of this study was to investigate the effect of culture temperature on the proliferation and differentiation of pig preadipocytes in primary culture. Culturing primary preadipocytes at 37 rather than 39°C decreases their proliferation rates based upon cleavage of the tetrazolium salt, MTT (P < 0.001), reduction of resazurin (P < 0.001), and daily cell counts (P < 0.001). Likewise, culturing primary porcine preadipocytes at 37°C suppressed their adipogenic potential based upon monitoring adipogenesis morphologically, biochemically, and via the expression of mRNA encoding adipogenic marker genes. Collectively, these data indicate the proliferation and differentiation of primary pig preadipocytes is suppressed when cultures are incubated at 37°C compared to normal body temperature of pigs. This may confound investigation of factors that impact adipocyte hyperplasia in the pig.
Abbreviations:
- CA3, carbonic anhydrase III
- C/EBPα, CCAAT/enhancer-binding protein α
- C/EBPβ, CCAAT/enhancer-binding protein β
- COUP-TF1, chicken ovalbumin protein transcription factor 1
- COUP-TF2, chicken ovalbumin protein transcription factor 2
- DGAT2, diacylglycerol O-acyltransferase 2
- ECM, extracellular matrix
- FMO1, flavin containing monooxygenase 1
- GPDH, glycerol-3-phosphate dehydrogenase
- ITIH3, inter-α (globulin) inhibitor H3
- MTT, 3-(4, 5-dimethylthiazol-2-yl)-2, 5-diphenyltetrazolium bromide
- OROSM, Oil Red O-stained material
- PFKFB1, 6-phosphofructo-2-kinase/fructose-2, 6-biphosphatase 1
- PPARγ, peroxisome proliferator-activated receptor gamma
- PTFβ, pleiotrophic factor β
- RIN, RNA Integrity Number
- RT-PCR, real-time polymerase chain reaction
- SAL1, salivary lipocalin
- S-V cells, stromal-vascular cells
- TN-X, tenascin-X
- TNFα, tumor necrosis factor α
Introduction
The US is in the midst of an obesity epidemic that is impairing physical fitness and precipitating increased incidences of diabetes and heart failure.Citation1 The current model of obesity-induced disease indicates that excessive adiposity and inappropriate adipose tissue extracellular matrix (ECM) remodeling increases circulating inflammatory signals.Citation2,3 Low grade, chronic inflammation then contributes to the metabolic dysregulation of muscle, heart, liver and the pancreas leading to the development of chronic degenerative diseases.Citation4,5 Thus, preventing the onset of obesity and uncoupling obesity and inflammation represents viable strategies to improve health and fitness. However, factors that regulate adipose tissue development and ECM remodeling need to be better understood in order to accomplish either objective.
Understanding adipose tissue development in the pig is an important goal in its own right given the worldwide importance of the pig as a food animal.Citation6 However, the pig is also well positioned as a biomedical model that can be used to overcome limitations inherent in using rodent models for the study of metabolic syndrome and obesity.Citation7 For instance, pigs are closer phylogenetically to humans, both are omnivores, and like in humans, anatomically discreet depots of brown fat are largely absent in the pig while the vasculature, the proportion of skeletal muscle and adipose tissue to total body mass and circulating levels of glucose are very similar in the pig and humans.Citation7,8 Likewise, there is a well-developed literature concerning the use of swine to study atherosclerosis, cardiovascular disease, and diabetes.Citation9-12 Thus, better understanding molecular mechanisms governing the proliferation and differentiation of porcine preadipocytes may provide insight into the regulation of adipose tissue development that should also inform human obesity and disease.
In that regard, primary cultures of pig stromal-vascular cells have served as a useful tool for investigating factors that regulate preadipocyte proliferation and differentiation. The establishment of optimum culture conditions that promote a high rate of porcine preadipocyte proliferation and differentiation while modeling adipocyte function as it occurs in vivo is crucial for further study of the factors that regulate adipocyte hyperplasia. Adipose tissue consists of various cell types important for its function as a metabolic and endocrine organ. Rodbell first established a procedure whereby the dissociation of cells via collagenase digestion and centrifugation allowed the separation and collection of lipid-filled adipocytes, isolated as the floating fraction, and all other cells in adipose tissue, including preadipocytes and stem cells, isolated as the pelletted stromal-vascular fraction.Citation13,14 Meanwhile, the cloning of the 3T3-L1 adipogenic cell line from dissociated mouse embryos greatly facilitated in vitro studies into the regulation of adipose tissue hyperplasia.Citation15,16 Creation of such clonal cell lines was soon followed by the establishment of primary culture systems for rodents and humans. Elegant studies using adipose tissue from developing depots within the porcine fetus represent initial and important efforts that established culture conditions for the in vitro study of porcine adipose tissue development by elucidating the impact of serum type and amount as well as the role of humoral factors in promoting adipogenesis.Citation17-19 These efforts were followed by the validation of serum-free conditions to further study the hormonal and nutritional regulation of pig preadipocyte differentiation.Citation20-25 However, such culture systems, to date, have utilized incubation temperatures of 37°C presumably to be consistent with conditions applied to human and rodent cultures and to allow the culture of multiple mammalian cell lines simultaneously within limited incubator space.
While maintaining an incubation temperature of 37°C models euthermia in humans and rodents, such conditions are 2°C lower than normal body temperature for pigs and may more faithfully model hypothermia in porcine cell cultures. This raises questions concerning the effect of incubation temperature upon the hyperplasia, metabolism and function of primary pig preadipocytes. Therefore the objective of this study was to investigate the effect of culture temperature, 37 versus 39°C, on the proliferation and differentiation of pig preadipocytes in primary culture using morphological, biochemical and marker gene expression as endpoints. Herein we demonstrate that the proliferation and differentiation of primary pig preadipocytes is suppressed when cultures are incubated at 37°Celsius compared to normal body temperature of pigs.
Results
To test the hypothesis that incubating cultures of primary porcine preadipocytes at a temperature necessary for maintenance of euthermia in vivo modulates the proliferation, differentiation and function of primary preadipocytes, S-V cells were isolated from neonatal porcine adipose tissue and incubated at either 37 or 39°C. The effect of temperature on preadipocyte proliferation was determined using the MTT, resazurin, and cell count assays as markers for proliferation. Differentiation under both serum-containing and serum-free conditions was monitored on d 8 following induction morphologically, biochemically, and by measuring the mRNA abundance of key adipogenic transcription factor, adipokine and adipocyte marker genes via real-time PCR.
Proliferation
To study the impact of incubation temperature on the proliferation of S-V cells, cultures were plated in growth medium and then cell number was monitored based upon cleavage of the tetrazolium salt, MTT, the reduction of resazurin, and physical cell counts measured initially and on days 1, 2 and 3 in cultures incubated at either 37 or 39°C (). As expected, preadipocyte number increased linearly with days in culture regardless of the method used to assess cell number (P < 0.0001). Cell number was increased 30–50% in cultures maintained at 39°C compared to those grown at 37°C based upon the MTT method (P < 0.001), resazurin reduction (P < 0.001), and daily cell counts (P < 0.001).
Figure 1. The effect of incubation temperature on proliferation in primary cultures of pig stromal-vascular cells following treatment for 3 d. Stromal-vascular cells were isolated from porcine adipose tissue, seeded at a concentration of 1.5 × 103 cells/cm2 in plating medium, and incubated for 24 h at 37°C (designated d −1). Cultures were then continuously exposed to either 37°C or 39°C in plating medium from d 0 to d 3. Cell number was determined in designated plates every 24 h by either directly counting trypsinized cell suspensions (A) or by indirectly determining cell number using the MTT method (B) or via resazurin reduction (C) as described in the Materials and Methods section. Data are means ± SEM from 4 experiments, each performed with cells harvested from a different pig. Twenty-4 replicate wells were assayed within treatment for each pig for each day of incubation. Means within a treatment that do not share a common asterisk differ, P < 0.05.
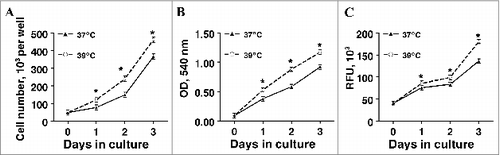
Differentiation
Glycerol-3-phosphate dehydrogenase (GPDH; EC 1.1.1.8) activity was used as an enzymatic marker of differentiation in our primary culture system because GPDH activity is expressed in terminally differentiated, mature fat cells, but it is not present in high degree in undifferentiated SV cells. As expected, GPDH activity was significantly increased on day 8 compared to uninduced cultures regardless of incubation temperature () as GPDH activity was 64-fold higher on d8 in cultures incubated at 37°C (P < 0.001) and 108-fold higher on d8 in cultures incubated at 39°C (P < 0.001). Importantly, consistent with the hypothesis that an incubation temperature modeling euthermic conditions modulates the differentiation of primary porcine preadipocytes, GPDH activity was 64% higher in cultures incubated at 39°C on d 8 post-induction than cultures incubated at 37°C (P < 0.05).
Figure 2. The effect of incubation temperature on glycerol-3-phosphate dehydrogenase (GPDH) activity in primary cultures of differentiating porcine preadipocytes on d 8. Stromal-vascular cells were isolated from porcine adipose tissue, seeded at a concentration of 5 × 104 cells/cm2 in plating medium, and incubated for 24 h at 37°C (designated d −1). Cultures were then continuously treated from d 0 to d 8 with differentiation medium while incubated at either 37°C or 39°C. Cell lysates were harvested after 8 d of treatment and immediately assayed for GPDH activity. Data are means ± SEM from 6 experiments, each performed with cells harvested from a different pig. Three replicate wells were assayed within treatment for each pig. Means within a treatment that do not share a common asterisk differ, P < 0.05.
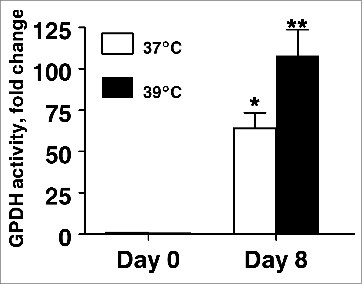
Adipogenesis was also monitored morphologically (). In agreement with the GPDH activity data, induction media significantly increased the number of lipid-filled cells present in cultures on day 8 irrespective of incubation temperature. Likewise, incubating differentiating cultures at 39°C resulted in significantly more lipid-filled cells being present on day 8. This was confirmed spectrophotometrically as extracted OROSM was 10.fold5- and fold17- higher cultures differentiated at 37°C (P < 0.01) and at 39°C (P < 0.001) respectively.
Figure 3. The effect of incubation temperature on the number of lipid-filled adipocytes and accumulation of Oil Red O-stained material (OROSM) present in primary cultures of differentiating porcine preadipocytes on d 8 after induction of differentiation. (A) Stromal-vascular cells were isolated from porcine adipose tissue, seeded at a concentration of 5 × 104 cells/cm2 in plating medium, and incubated for 24 h at 37°C (designated d −1). Differentiating preadipocytes were continuously incubated at either 37°C or 39°C for 8 d after induction. The microscopic magnification was 10× for all images. (B) Stromal-vascular cells were isolated and treated as above. On d 8, plates were stained with Oil Red O, and the extracted stain was quantified spectrophotometrically. The amount of OROSM per well was expressed relative to the protein content of unstained wells receiving similar treatment on the same plate. Data are means ± SEM from 6 experiments, each performed with cells harvested from a different pig.
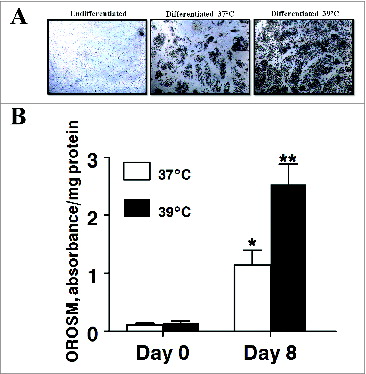
Gene expression
To further study the effect of incubation temperature on the differentiation of porcine preadipocytes, mRNA expression of key adipogenic transcription factors was measured in response to culture conditions (). The expression of, CCAAT/enhancer-binding protein β (C/EBPβ) was highest on d 0 and decreased by day of differentiation under both culture conditions (P < 0.05, ) while the effect was less in cultures that were incubated at 39°C (P < 0.05). The mRNA expression of CCAAT/enhancer-binding protein α (C/EBPα) was induced fold17- in differentiating cultures incubated at 37°C (P < 0.05, ) and 51-fold in cultures incubated at 39°C (P < 0.01). Likewise, consistent with robust differentiation of preadipocytes, peroxisome proliferator-activated receptor gamma (PPARγ) mRNA was upregulated 3.fold3- (P < 0.05, ) and fold13- (P < 0.01), chicken ovalbumin protein transcription factor 1 (COUP-TF1) was upregulated 1.fold7- (P < 0.05, ) and fold2- (P < 0.05), and bone morphogenic protein 4 (BMP4) was upregulated 3.fold3- (P < 0.05, ) and 4.fold4- (P < 0.05) in cultures incubated at 37°C and 39°C respectively. Surprisingly, expression of chicken ovalbumin protein transcription factor 2 (COUP-TF2) was decreased 1.fold6- (P < 0.05, ) in cultures incubated at 37°C but was slightly upregulated 1.fold4- (P < 0.05) in cultures that were incubated at 39°C.
Figure 4. The effect of incubation temperature on mRNA expression in differentiating cultures of primary pig preadipocytes for the transcriptional modulators (A) CCAAT/enhancer-binding protein β (C/EBPβ), (B) CCAAT/enhancer-binding protein α (C/EBPα), (C) peroxisome proliferator-activated receptor gamma (PPARγ), (D) chicken ovalbumin protein transcription factor 1 (COUP-TF1), (E) chicken ovalbumin protein transcription factor 2 (COUP-TF2), and (F) bone morphogenic protein 4 (BMP4). Expression was determined by real-time RT-PCR. Values were normalized to S15 expression. Data is expressed as fold change relative to baseline (d 0) and calculated according to Pfaffl, 2010. Bars denoted by * and ** differ (P < 0.05), n = 6.
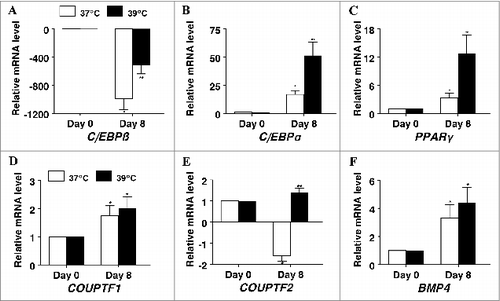
To examine a potential effect of incubation temperature on the endocrine function of differentiating primary porcine preadipocytes, mRNA expression of key adipokines was measures in response to culture temperature (). As expected, the mRNA expression of both adiponectin and leptin were significantly elevated in differentiating cultures regardless of incubation temperature with adiponectin mRNA being upregulated 110-fold (P < 0.05, ) and 745-fold (P < 0.01), and leptin mRNA upregulated 21.fold6- (P < 0.05, ) and 130-fold (P < 0.01) in cultures differentiated at 37°C and 39°C respectively. Meanwhile the mRNA expression of the proinflammatory cytokines interleukin-6 and tumor necrosis factor α (TNFα), were significantly decreased in differentiating cultures regardless of incubation temperature with interleukin-6 mRNA being fold15- (P < 0.05, ) and 8.fold7- (P < 0.01), and TNFα mRNA 4.fold2- (P < 0.05, ) and 2.fold6- (P < 0.01) lower in cultures differentiated at 37°C and 39°C respectively. The consistent differences in magnitude of responses across these genes in cultures differentiated at 39°C vs. 37°C supports the hypothesis that culturing primary porcine S-V cells at euthermic temperature can alter adipogenesis and endocrine function.
Figure 5. The effect of incubation temperature on mRNA expression in differentiating cultures of primary pig preadipocytes for the adipokines (A) adiponectin, (B) leptin, (C) interleukin-6, and (D) tumor necrosis factor α (TNFα). Expression was determined by real-time RT-PCR. Values were normalized to S15 expression. Data is expressed as fold change relative to baseline (d 0) and calculated according to Pfaffl, 2010. Bars denoted by * and ** differ (P < 0.05), n = 6.
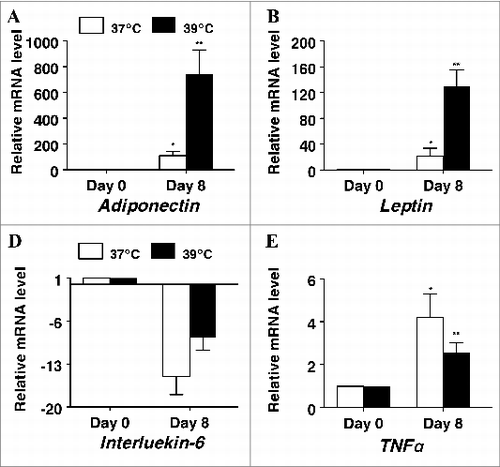
To further assess the potential for incubation temperature to influence cellular function in differentiating cultures of primary porcine S-V cells, the mRNA expression of a survey of genes identified by RNAseq experiments as being differentially regulated during differentiation of porcine preadipocytes (T. Brandebourg, unpublished data) was examined (). Consistent with the effect on transcription factor and adipokine mRNA expression, the magnitude of response to induction medium was greater for carbonic anhydrase III (CA3), flavin containing monooxygenase 1 (FMO1), inter-α (globulin) inhibitor H3 (ITIH3), 6-phosphofructo-2-kinase/fructose-2,6-biphosphatase 1 (PFKFB1), salivary lipocalin (SAL1), diacylglycerol O-acyltransferase 2 (DGAT2), pleiotrophic factor β (PTFβ), and tenascin-X (TN-X) in cultures that were differentiated at 39°C versus 37°C ().
Figure 6. The effect of incubation temperature on mRNA expression in differentiating cultures of primary pig preadipocytes for marker genes (A) carbonic anhydrase III (CA3), (B) flavin containing monooxygenase 1 (FMO1), (C) inter-α (globulin) inhibitor H3 (ITIH3), (D) 6-phosphofructo-2-kinase/fructose-2, 6-biphosphatase 1 (PFKFB1), (E) salivary lipocalin (SAL1), (F) diacylglycerol O-acyltransferase 2 (DGAT2), (G) pleiotrophic factor β (PTFβ), and (H) tenascin-X (TN-X). Expression was determined by real-time RT-PCR. Values were normalized to S15 expression. Data is expressed as fold change relative to baseline (d 0) and calculated according to Pfaffl, 2010. Bars denoted by * and ** differ (P < 0.05), n = 6.
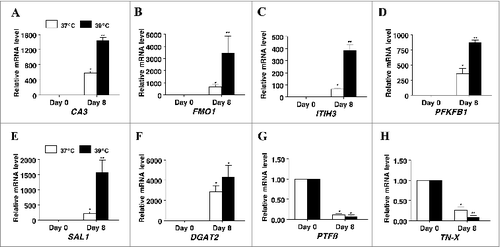
Effect of incubation temperature on markers of differentiation in cultures differentiated under serum-free conditions
It is well established that adipogenic conversion is enhanced by allowing clonal preadipocyte cell lines to reach confluence before differentiation is inducedCitation16. The higher rate of proliferation observed in cultures incubated at 39°C in the present study could potentially confound differentiation studies aimed at examining the effect of incubation temperature by either increasing the number of preadipocytes present in culture or by causing cultures to achieve confluence sooner vs. cultures grown at 37°C. In order to address this issue, cultures were grown to confluence in plating medium at 37°C and switched to serum-free conditions in order to eliminate the mitogenic activity associated with serum-borne factors. Subsequently cultures were induced to differentiate for 8 d at either 37°C or 39°C under serum-free conditions. Consistent with a robust adipogenic response, GPDH activity and the number of lipid-containing cells present in culture were significantly increased in induced cultures on day 8 compared to uninduced cultures regardless of incubation temperature (). Importantly, consistent with differentiation experiments conducted in serum-containing medium, GPDH activity was 2.fold1- higher (P < 0.05) and extracted OROSM was 1.94-fold higher (P < 0.05) on d 8 post-induction in cultures incubated at 39°C versus cultures incubated at 37°C (). Furthermore, in agreement with morphological and biochemical measures, mRNA expression for PPARγ, adiponectin and leptin was 3.3- (P < 0.05), 2.7- (P < 0.05) and 2.fold9- (P < 0.05) higher on d 8 post induction respectively in cultures incubated at 39°C vs. cultures incubated at 37°C.
Figure 7. The effect of incubation temperature on markers of adipogenesis in cultures of primary pig preadipocytes differentiated under serum-free conditions. (A) Photomicrographs depict representative cultures on d 0 and 8 after induction of differentiation. The microscopic magnification was 10× for all images. (B) Amount of extractable OROSM dye from stained cultures on d 0 and 8 after induction of differentiation. (C) Glycerol-3-phosphate dehydrogenase (GPDH) activity on d 0 and 8 after induction of differentiation. (D–F) The abundance of peroxisome proliferator-activated receptor gamma (PPARγ), adiponectin, and leptin mRNA on d 0 and 8 after induction of differentiation. Stromal-vascular cells were isolated from porcine adipose tissue, seeded at a concentration of 5 × 104 cells/cm2 in plating medium and incubated at 37°C until cultures reached full confluence. Confluent cultures were then washed in DME/F12 medium and continuously incubated at either 37°C or 39°C for 8 d in serum-free differentiation medium. Expression of mRNA was determined by real-time RT-PCR. Values were normalized to S15 expression. Data is expressed as fold change relative to baseline (d 0) and calculated according to Pfaffl, 2010. Bars denoted by * and ** differ (P < 0.05), n = 6.
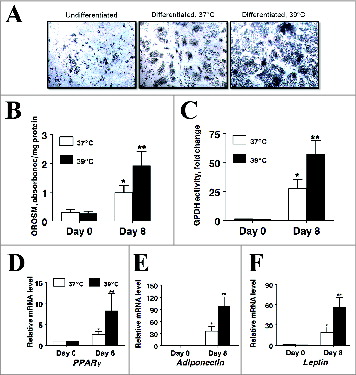
Discussion
Primary cultures of stromal-vascular cells are a vital tool for investigating factors that regulate adipocyte hyperplasia in the pig.Citation26 As such, both serum-containing and serum-free systems have been successfully established that support the proliferation and differentiation of adipose-derived porcine S-V cells in vitro.Citation17-25 However, refining optimum culture conditions that faithfully model adipocyte behavior in vivo and promote robust cellular responses remains a continuous priority in order to speed the rate of discovery concerning factors that regulate adipocyte biology. We report herein for the first time that culturing primary porcine S-V cells at euthermic temperature for the porcine (39°C) rather than the traditional temperature of 37°C alters the behavior of these cells in culture. In the present study, proliferation rate was higher for cells cultured at 39°C compared to those cultured at 37°C based upon 3 separate estimates of cell number. Likewise, culturing primary porcine preadipocytes at 39°C enhanced their adipogenic potential based upon monitoring adipogenesis morphologically, biochemically, and via the expression of mRNA encoding adipogenic marker genes. Collectively these data support the hypothesis that culturing pig preadipocytes at euthermic temperature enhances hyperplasia in these cultures.
Adipose tissue consists of various cell types including fibroblasts, preadipocytes, mesenchymal stem cells, immune cells, and blood vessels which collectively confer its function as a metabolic and endocrine organ.Citation27 Primary cultures more faithfully model adipose cellularity compared to clonal cell lines that are largely homogeneous.Citation26 However, it is likely that culture conditions can impact the cellularity of primary cultures by selecting for subpopulations of cell types or through altering the responsiveness of existing cells to extrinsic signals. This may enrich cultures with preadipocytes or promote the presence of cells within the stromal fraction that do not possess adipogenic potential. Such effects could in turn alter autocrine and paracrine signaling, impact the recruitment of preadipocytes, alter the conversion of preadipocytes to adipocytes, and potentially modulate the endocrine and immune character of such cultures.Citation28-33 Likewise, notable differences exist in regulatory gene expression between cell lines and primary cultures. In the current model of adipogenesis derived from the study of clonal preadipocyte cell lines, the sequential induction of C/EBPβ, PPARγ, and C/EBPα in preadipocytes results in transactivation of adipocyte-specific genes leading to terminal differentiation of the adipocyte.Citation34,35 However, the temporally discreet developmental pattern of transcriptional regulators exhibited by clonal preadipocyte cell lines is obscured in primary porcine S-V cells since such cultures contain preadipocytes at different stages of differentiation and thus often express significant quantities of mRNA and protein for C/EBPα, -β, and -δ, as well as PPARγ even before experimental induction protocols are initiated.Citation26,Citation36-39 Nonetheless, as in murine models, PPARγ and C/EBPα are stimulatory and necessary regulators of porcine preadipocyte differentiation.Citation40 Such differences however highlight the importance of optimizing culture conditions when using primary cultures of pig S-V cells in order to study the regulation of adipose tissue development.
Because incubation temperature could potentially alter hyperplasia in primary porcine preadipocyte cultures, our first objective was to examine cell number and morphological and biochemical markers of adipogenesis in cultures incubated at either 37°C or 39°C. Cell number was increased 30–50% under euthermic conditions as determined by direct cell counts or indirect estimates utilizing the MTT or resazurin reduction methods (). Likewise, cultures displayed greater adipogenic potential when incubated at 39°C as determined using GPDH activity and lipid accumulation as markers of preadipocyte differentiation (; ). These results were verified at the gene level as well by examining the expression of 8 genes randomly chosen from a list of genes identified by RNAseq experiments as being differentially expressed during the differentiation of pig preadipocytes (Brandebourg unpublished data). All genes in this panel where more robustly regulated during differentiation in cultures incubated at 39°C versus cultures incubated at 37°C (). We conclude that incubating primary porcine S-V cells at euthermic temperature potentiates the hyperplasia of these cells in vitro. To the best knowledge of these authors, this is the first time such an affect has been observed and these findings suggest that culturing primary porcine preadipocytes at 39°C may enhance the ability to study factors that regulate adipogenesis in the pig by facilitating more robust responses to adipogenic signals. Incubation temperature generally affected the magnitude rather than the direction of responses measured in the current study suggesting that previous work using primary porcine S-V cells remains valid. However, the more robust responses associated with incubating primary pig S-V cells at euthermic temperature may confer an advantage by decreasing the likelihood of committing type II errors when hypothesis testing and by presumably better modeling the development of adipose tissue in vivo.
Clonal 3T3 preadipocytes are sensitive to contact inhibition and rapidly exit the cell cycle upon reaching confluence unless cultured under high-serum conditions.Citation16,41 This promotes preadipocyte differentiation as contact inhibition coincides with increased expression of adipogenic transcription factors that drive preadipocyte differentiation in clonal cell lines.Citation26,34,35 However; primary cultures of pig preadipocytes are generally refractory to contact inhibition in the presence of serum-containing medium. While cultures of primary SV cells exhibit gradually decreasing proliferative rates and eventually undergo senescence with increasing passage number, primary SV cells will grow to several layers thick in culture if allowed to reach confluence in the presence of complete growth medium containing mitogenic activity.Citation41 Thus primary porcine SV cells incubated at euthermic temperature might undergo differentiation earlier than cultures incubated at 37°C and thus appear to have a more robust adipogenic response when compared to slower growing cultures at a similar day post-induction of differentiation. In order to address this concern, cultures were incubated at 37°C and serum was withdrawn once confluence was reached. Consistent with differentiation experiments conducted in the presence of serum, incubating cultures at 39°C produced a more robust adipogenic response despite induction of differentiation occurring after confluence and in the absence of mitogenic activity. This suggests that while an increased proliferative rate likely does promote an earlier adipogenic response when cultures are seed at subconfluent densities, incubating primary porcine preadipocytes at 39°C likewise directly potentiates adipogenesis in these cultures.
Our second objective was to investigate the effect of incubation temperature on expression of transcription factors that are known to regulate adipogenesis. Consistent with their role as adipogenic transcription factors, the mRNA expression of PPARγ and C/EBPα was increased on d8 of differentiation vs. uninduced cultures regardless of incubation temperature. These results agree with multiple previous reports wherein differentiating cultures of primary pig S-V cells were incubated at 37°C.Citation24,25,38,42 Concerning the magnitude of response to induction medium, Ding et al.Citation38 reported a fold1- induction of PPARγ mRNA by d 10 of differentiation which is less than the fold3- increase in PPARγ mRNA observed in cultures differentiated at 37°C in the present study. Generally, PPARγ expression is significantly induced in differentiating cultures of primary pig preadipocytes and results in the current study largely agree with previous reports.Citation24,25,40 Interestingly, in the present study, C/EBPβ mRNA expression was significantly decreased upon induction which is a unique observation in differentiating primary pig preadipocytes. Importantly, consistent with the increased GPDH activity and lipid accumulation in cultures differentiated at euthermic temperature, incubating differentiating cultures at 39°C potentiated the effect of induction on the expression of adipogenic transcription factors in the present study. Given this, we conclude that euthermic temperature potentiates the hyperplasia of these cells in vitro in part by increasing the expression of adipogenic transcription factors. Finally, BMP4 mRNA expression was induced during differentiation in a manner that was not affected by incubation temperature. This is consistent with the observation that porcine subcutaneous and intramuscular adipocytes express BMP4 mRNA and that BMP4 is secreted from porcine adipose tissue.Citation43–45 The novel observation that BMP4 mRNA was upregulated during differentiation in the present study further supports the hypothesis that BMP4 may regulate adipogenesis in the pig.
Chicken ovalbumin upstream promoter transcription factor (COUP-TF) is an orphan nuclear receptor that has been implicated in the inhibition of differentiation in multiple cell types including rodent and pig preadipocytes.Citation24,25,Citation46-51 In the current study, the expression of COUP-TF1 mRNA was upregulated during differentiation regardless of incubation temperature while the expression of COUP-TF2 mRNA was reduced in cultures differentiated at 37°C but not in those differentiated at 39°C. Anti-adipogenic factors such as retinoic acid or conjugated linoleic acids have been shown to increase expression of COUP-TF in differentiating cultures of pig preadipocytes though the induction of differentiation in the absence of these factors did not alter COUP-TF expression in these studies.Citation24,25 Meanwhile retinoic acid both induced the expression of COUP-TF and increased the binding of COUP-TF to a PPARγ binding sequence transfected into 3T3-L1 preadipocytes consistent with a role for COUP-TF in mediating the anti-adipogenic action of retinoids.Citation49 Further supporting a role for COUP-TF as an anti-adipogenic transcription factor, the overexpression of COUP-TF inhibited while knockout of COUP-TF stimulated the differentiation of 3T3-L1 preadipocytes through directly interacting with the C/EBPα promoter.Citation50 Interestingly, however, wild-type COUP-TF mice display increased adiposity compared to COUP-TF heterozygotes.Citation51 These studies suggest a dual and opposing role for COUP-TF in regulating adipogenesis whereby COUP-TF functions as a recruitment factor that directs mesenchymal stem cells to commit to the adipocyte lineage while sustained expression of COUP-TF by preadipocytes can inhibit adipogenesis. This work provides a rationale for further studying the potential of COUP-TF as a regulator of porcine adipogenesis. Importantly, however, the present study suggests that incubation temperature could potentially confound the study of factors that may regulate preadipocyte recruitment and differentiation given that COUP-TF2 mRNA was reduced in cultures differentiated at 37°C but not in those differentiated at 39°C.
Our final objective was to investigate the effect of incubation temperature on the expression of adipokine mRNA in differentiating pig preadipocytes. Transcriptomic and proteomic analysis have demonstrated that porcine adipose tissues express a wide array of secreted factors including leptin, adiponectin, IL-6, and TNFα and that the expression profiles of these adipokines change during adipogenesis, are altered during the rapid increase in adiposity associated with nursing in neonatal pigs, and are regulated by dietary fat in growing pigs.Citation43,Citation52-59 Furthermore, adipose tissue from obese pigs exhibits a cytokine expression profile consistent with the development of low-grade chronic inflammation seen in human obesity and porcine adipose tissue responds to LPS challenge to increase proinflammatory adipokine expression.Citation57,Citation59,Citation60 In the current study, the expression of leptin, adiponectin, IL-6, and TNFα mRNA was verified in neonatal adipose tissue from the Mangalica pig, a novel lard-type breed, for the first time. The down regulation of IL-6 mRNA during adipogenesis in the present study was expected since IL-6 is also down regulated during the differentiation of rodent preadipocytes and IL-6 is predominantly secreted from stromal vascular cells in human and rodent adipose tissue.Citation61-65 Likewise, the induction of TNFα expression during adipogenesis is consistent with previous studies in pig and human preadipocytes and mirrors the increased TNFα expression previously observed in neonatal subcutaneous adipose tissue during suckling, a time when considerable adipose tissue hyperplasia is occurring in the pig.Citation52,61,66 Leptin and adiponectin are well-established markers of adipogenesis and mRNA expression levels for both increased dramatically in differentiating cultures of pig preadipocytes consistent with the appearance of lipid-filled cells regardless of incubation temperature. Furthermore, adiponectin and leptin mRNA were roughly fold7- higher in cultures differentiated at 39°C compared to cultures differentiated at 37°C suggesting that cultures incubated at 39°C had increased rates of differentiation. Surprisingly, however, the response to induction was muted in cultures incubated at 39°C for both IL-6 and TNFα mRNA. The reason for this response is unclear but this observation suggests the activity of the innate pathway in porcine adipocytes may be impacted by incubation temperature. Finally, while the expression of these adipokines where only measured at the level of mRNA abundance in the current study, Hausman et al.Citation43 demonstrated that fetal and neonatal porcine adipose tissue synthesize and secrete these proteins. The expression patterns seen in the current study are consistent with protein levels observed by Hausman and colleagues. Further study is needed to understand the role adipokines play in regulating growth and development in the pig.
In summary, this report indicates that culturing primary pig S-V cells at euthermic temperature enhances cellular processes contributing to the hyperplasia of adipose tissue. Specifically, culturing primary pig preadipocytes at 39°C enhanced their proliferation and differentiation while potentiating the effect of induction hormones on the expression of marker gene, adipogenic transcription factor, and adipokine mRNA. Importantly, our data suggest that culturing pig preadipocytes at 39°C rather than the traditional 37°C results in a more robust model system in which to study the factors that regulate adipogenesis in the pig.
Materials and Methods
Pig preadipocyte culture and differentiation
Two-day-old Mangalica pigs were obtained from the Auburn University Swine Research and Education Center and harvested by CO2 asphyxiation in a manner approved by the Animal Care and Use Committee at Auburn University. Primary stromal-vascular (S-V) cells were harvested from neonatal pig adipose tissue according to Brandebourg et al.Citation24,25 Aliquots of S-V cells were counted using a hemacytometer and seeded in culture dishes at a density of 5 × 104 cells/cm2 and incubated at either 37°C or 39°C in 5% CO2 in air (designated d −1). Plating medium consisted of DME/F12 (1:1, vol/vol) containing 15 mmol/L of NaHCO3, 15 mmol/L of HEPES buffer, and 50 mg/L of gentamicin sulfate supplemented with 10% FBS. When cultures were incubated in the presence of serum (–), cells were plated on either 96 well, 6 well, or 10-cm plates to facilitate proliferation, differentiation, or gene expression studies respectively. After 24 h, attached cells were washed 3 times with plating medium to remove unattached cells and cellular debris (designated d 0). After washing, cells were either continually induced to differentiate in medium containing 10% FBS, 580 ng/ mL of insulin, 10 μg/mL of transferrin, 500 ng/mL of hydrocortisone and .5 μM rosiglitazone until d 8 or to facilitate proliferation studies, cells were maintained in growth medium until the indicated day cultures were terminated for cell number analysis. For cultures differentiated under serum-free conditions (), aliquots of S-V cells were seeded in culture dishes at a density of 5 × 104 cells/cm2 and incubated at 37°C in plating medium until reaching confluence. Confluent cultures were then washed in DME/F12 medium and continuously incubated at either 37°C or 39°C for 8 d in serum-free differentiation medium (DME/F12, 580 ng/ mL of insulin, 10 μg/mL of transferrin, 500 ng/mL of hydrocortisone and .5 μM rosiglitazone). In all cultures induced to differentiate, culture media was changed every 2 d until d 8 when cells were subjected to glycerol-3-phosphate dehydrogenase (GPDH) assays, oil red O staining, or gene expression analysis.
Histochemistry
In order to qualitatively assess S-V cell differentiation by microscopy, cells were exposed to differentiation media from d 0 to 8 and then fixed in 10% formalin and stained with 0.3% ORO for lipid. Extractable ORO was then measured spectrophotometrically (570 nm) by modifying the procedure of Suryawan and Hu.Citation21 Briefly, the wells were fixed with Baker's formalin for 15 min, rinsed with distilled water, equilibrated in 100% propylene glycol for 2 min, and then stained with ORO for 10 min. Wells were then treated with 60% propylene glycol (vol/vol) for 1 min to remove free ORO, and rinsed with distilled water. The ORO was extracted with the addition of isopropanol and ORO determined in aliquots from wells following shaking the culture plates 30 min at room temperature.
Glycerol-3-phosphate dehydrogenase activity
The Sn-glycerol-3-phosphate dehydrogenase (GPDH; EC 1.1.1.8) activity was determined by measuring spectrophotometrically the disappearance of NADH during the GPDH-catalyzed reduction of DHAP under zero-order conditions by the method of Kozak and Jensen,Citation67 as modified by Wise and Green.Citation68 Briefly, differentiated cells were harvested in ice-cold lysate buffer (0.25 M sucrose, 1 mM EDTA, 1 mM dithiothreitol, 5 mM Tris base, pH 7.4). Membranes were disrupted by sonication and supernatant fractions were collected following centrifugation at 13,000 × g for 10 min at 4°C to remove cellular debris. The reaction was initiated by the addition of supernatant fractions to a standard mixture containing 100 mM triethanolamine/HCl buffer (pH 7.4), 2.5 mM EDTA, 0.176 mM NADH, 0.37 mM DHAP, and 0.1 mM β-mercaptoethanol. The reaction was linear for sample time and concentration. Glycerol-3-phosphate dehydrogenase activity was expressed as units per milligram of protein, where one unit of activity is defined as the oxidation of 1 nmol of NADH/ min. Protein was measured according to Bradford.Citation69
Cell proliferation assays
Preadipocytes were plated into 96-well plates (Nunc) at a density of 500 cells/well in growth medium (DMEM/F12/10% FBS). Cell growth was evaluated on subsequent days by either 1) performing physical cell counts following trypsinization of wells, 2) incubation with [3-(4,5-dimethylthiazol-2-yl)2,5-diphenyl tetrazolium bromide (MTT) at a final concentration of 0.5 mg/ml for 2 h or incubation with resazurin at a final concentration of 50 ug/ml for 2 hr. This was followed by aspiration of the medium and solubilization of the formazan product with dimethylsulfoxide. Absorbance was read at 570 nm (MTT) while fluorescence (resazurin) was determined at 530 nm excitation and 590 nm emission using a 96-well multimode plate reader (Bio-Tek, Winooski, VT).
Gene expression analysis
Total RNA was extracted from uninduced cultures and from cultures on d 8 following induction using a 2-step purification protocol with total RNA first being extracted from whole tissue using RNAzol® RT (MRC, Inc., Cincinnati, OH) followed by purification using RNAeasy spin columns (QIAGEN, Inc., Valencia, CA) according to the manufacturers’ recommendations. RNA was quantified using a BioTek Synergy 4 plate reader utilizing the Take3 system (BioTek US, Winooski, VT) with all samples exhibiting an OD 260/280 between 1.8 and 2.0 and an OD 260/230 value between 1.8 and 2.2. Spectral scans ranging from 200 to 400 nm further verified sample purity as all RNA samples produced smooth curves exhibiting one peak at 260 nm. Total RNA integrity was accessed both visually by resolving 2 μg of RNA on a denaturing formaldehyde gel containing ethidium bromide and by determining an RNA Integrity Number (RIN) using an Agilent 2100 bioanalyzer (Agilent Technologies, Inc., Clara, CA). All samples demonstrated sharp ribosomal bands with a 28S to 18S ratio greater than one and RIN values greater than 9.0 and were thus judged intact and non-degraded. Total RNA was then reverse transcribed using Superscript II reverse transcriptase (Promega Inc., Madison, WI) and oligo-dT primers. Real-time PCR was performed on the resultant cDNA using a Roche Lightcycler® 480 Real-time PCR machine and LightCycler® 480 SYBR Green I Master Mix (Roche Applied Science, Indianapolis, IN) according to manufacturer's recommendations. All PCR reactions were performed using intron-spanning primers under optimized conditions with primer efficiencies ranging between 90–101% () as verified with standard curves. Product purity was assessed by melting curve analysis and expected amplicon sizes were verified on a 2% agarose gel stained with ethidium bromide. Values were normalized to S 15 mRNA expression. The S15 mRNA levels represent an appropriate control as the efficiency of the S15 primers was 100% () and S15 mRNA expression was not different between any groups tested (P >0.05). Data are expressed as fold change relative to baseline and calculated according to Pfaffl.Citation70
Table 1. Oligonucleotide polymerase chain reaction primers
Statistical analysis
Experimental animals were assigned to different treatments in a completely randomized design. Data are expressed as the mean ± SEM. Each replicate consisted of a single batch of S-V cells harvested from the subcutaneous adipose tissue of an individual pig. Data were analyzed by using one-way ANOVA, followed by multiple comparisons of means with Fisher's LSD using SAS (SAS Inst., Inc., Cary, NC). Differences were considered significant at P < 0.05. Changes in gene expression were calculated from the cycle threshold, after correction using S15 expression and analyzed using the Pair Wise Fixed Reallocation Randomization Test of REST-MCS v2.0 (http://rest.gene-quantification.info/).
Disclosure of Potential Conflicts of Interest
No potential conflicts of interest were disclosed.
References
- Centers for Disease Control and Prevention (CDC). The state adult obesity prevalences for 2011 and 2012. Available from www.cdc.govobesitydataadult.html” ( accessed July 27, 2014).
- Tilg H, Moschen AR. Adipocytokines: mediators linking adipose tissue, inflammation and immunity. Nat Rev Immunol 2006; 6:772-83; PMID:16998510; http://dx.doi.org/10.1038/nri1937
- Khan T, Muise ES, Iyengar P, Wang ZV, Chandalia M, Abate N, Zhang BB, Bonaldo P, Chua S, Scherer PE. Metabolic dysregulation and adipose tissue fibrosis: role of collagen VI. Mol Cell Biol 2009; 29:1575-91; PMID:19114551; http://dx.doi.org/10.1128/MCB.01300-08
- Alberti KG, Eckel RH, Grundy SM, Zimmet PZ, Cleeman JI, Donato KA, Fruchart JC, James WP, Loria CM, Smith SC Jr. Harmonizing the metabolic syndrome: a joint interim statement of the International Diabetes Federation Task Force on Epidemiology and Prevention; National Heart, Lung, and Blood Institute; American Heart Association; World Heart Federation; International Atherosclerosis Society; and International Association for the Study of Obesity. Circulation 2009; 120:1640-5; PMID:19805654; http://dx.doi.org/10.1161/CIRCULATIONAHA.109.192644
- Esser N, Legrand-Poels S, Piette J, Scheen AJ, Paquot N. Inflammation as a link between obesity, metabolic syndrome and type 2 diabetes. Diabetes Res Clin Pract 2014; 105:141-150; PMID:24798950; http://dx.doi.org/10.1016/j.diabres.2014.04.006
- Hausman GJ, Poulos SP, Pringle TD, Azain MJ. The influence of thiazolidinediones on adipogenesis in vitro and in vivo: potential modifiers of intramuscular adipose tissue deposition in meat animals. J Anim Sci 2008; 86:E236-43; PMID:17686902; http://dx.doi.org/10.2527/jas.2007-0219
- Spurlock ME, Gabler NK. The development of porcine models of obesity and the metabolic syndrome. J nutrition 2008; 138:397-402; PMID:18203910
- Lunney JK. Advances in swine biomedical model genomics. Int J of Biol Sci 2007; 3:179-84; PMID:17384736; http://dx.doi.org/10.7150/ijbs.3.179
- Gerrity RG, Natarajan R, Nadler JL, Kimsey T. Diabetes-induced accelerated atherosclerosis in swine. Diabetes 2001; 50:1654-65; PMID:11423488; http://dx.doi.org/10.2337/diabetes.50.7.1654
- Brambilla G, Cantafora A. Metabolic and cardiovascular disorders in highly inbred lines for intensive pig farming: how animal welfare evaluation could improve the basic knowledge of human obesity. Ann Ist Super Sanita 2004; 40:241-4; PMID:15536277
- Dyson MC, Alloosh M, Vuchetich JP, Mokelke EA, Sturek M. Components of metabolic syndrome and coronary artery disease in female Ossabaw swine fed excess atherogenic diet. Compar Med 2006; 56:35-45; PMID:16521858
- Skold BH, Getty R, Ramsey FK. Spontaneous atherosclerosis in the arterial system of aging swine. Am J Vet Res 1966; 27:257-73; PMID:4161799
- Rodbell M. Metabolism of isolated fat cells. I. effects of hormones on glucose metabolism and lipolysis. J Biol Chem 1964; 239:375-80; PMID:14169133
- Hausman GJ. Techniques for studying adipocytes. Stain Technol 1981; 56:149-54; PMID:6168034
- Todaro GJ, Green H. Serum albumin supplemented medium for long term cultivation of mammalian fibroblast strains. Proc Soc Exp Biol Med Soc Exp Biol Med 1964; 116:688-92; http://dx.doi.org/10.3181/00379727-116-29346
- Green H, Kehinde O. An established preadipose cell line and its differentiation in culture. II. Factors affecting the adipose conversion. Cell 1975; 5:19-27; PMID:165899; http://dx.doi.org/10.1016/0092-8674(75)90087-2
- Hausman GJ, Novakofski JE, Martin RJ, Thomas GB. The development of adipocytes in primary stromal-vascular culture of fetal pig adipose tissue. Cell Tissue Res 1984; 236:459-64; PMID:6428745; http://dx.doi.org/10.1007/BF00214250
- Ramsay TG, Hausman GJ, Martin RJ. Effects of fetal versus postnatal sera upon adipose tissue stromal-vascular cells in primary culture. Cell Tissue Res 1987; 250:185-90; PMID:3652159; http://dx.doi.org/10.1007/BF00214670
- Ramsay TG, Hausman GJ, Martin RJ. Pre-adipocyte proliferation and differentiation in response to hormone supplementation of decapitated fetal pig sera. J Anim Sci 1987; 64:735-44; PMID:3571002
- Hausman GJ, Martin RJ. The influence of human growth hormone on preadipocyte development in serum-supplemented and serum-free cultures of stromal-vascular cells from pig adipose tissue. Domest Anim Endocrinol 1989; 6:331-7; PMID:2620504; http://dx.doi.org/10.1016/0739-7240(89)90027-1
- Suryawan A, Hu CY. Effect of serum on differentiation of porcine adipose stromal-vascular cells in primary culture. Compar Biochem Physiol Compar Physiol 1993; 105:485-92; PMID:8101780; http://dx.doi.org/10.1016/0300-9629(93)90424-3
- Suryawan A, Hu CY. Effect of retinoic acid on differentiation of cultured pig preadipocytes. J Anim Sci 1997; 75:112-7; PMID:9027555
- Suryawan A, Swanson LV, Hu CY. Insulin and hydrocortisone, but not triiodothyronine, are required for the differentiation of pig preadipocytes in primary culture. J Anim Sci 1997; 75:105-11; PMID:9027554
- Brandebourg TD, Hu CY. Isomer-specific regulation of differentiating pig preadipocytes by conjugated linoleic acids. J Anim Sci 2005; 83:2096-105; PMID:16100064
- Brandebourg TD, Hu CY. Regulation of differentiating pig preadipocytes by retinoic acid. J Anim Sci 2005; 83:98-107; PMID:15583048
- Boone C, Gregoire F, Remacle C. Regulation of porcine adipogenesis in vitro, as compared with other species. Domest Anim Endocrinol 1999; 17:257-67; PMID:10527128; http://dx.doi.org/10.1016/S0739-7240(99)00042-9
- Ahima RS. Adipose tissue as an endocrine organ. Obesity 2006; 14 Suppl 5:242S-9S; PMID:17021375; http://dx.doi.org/10.1038/oby.2006.317
- Hausman DB, DiGirolamo M, Bartness TJ, Hausman GJ, Martin RJ. The biology of white adipocyte proliferation. Obesity Rev 2001; 2:239-54; PMID:12119995; http://dx.doi.org/10.1046/j.1467-789X.2001.00042.x
- Hausman GJ, Richardson RL. Adipose tissue angiogenesis. J Anim Sci 2004; 82:925-34; PMID:15032451
- Hausman GJ, Dodson MV. Stromal vascular cells and adipogenesis: cells within adipose depots regulate adipogenesis. J Genomics 2013; 1:56-66; PMID:25031656; http://dx.doi.org/10.7150/jgen.3813
- Hausman GJ, Richardson RL. Newly recruited and pre-existing preadipocytes in cultures of porcine stromal-vascular cells: morphology, expression of extracellular matrix components, and lipid accretion. J Anim Sci 1998; 76:48-60; PMID:9464884
- Poulos SP, Dodson MV, Hausman GJ. Cell line models for differentiation: preadipocytes and adipocytes. Exp Biol Med (Maywood) 2010; 235:1185-93; PMID:20864461; http://dx.doi.org/10.1258/ebm.2010.010063
- Poulos SP, Hausman DB, Hausman GJ. The development and endocrine functions of adipose tissue. Mol Cell Endocrinol 2010; 323:20-34; PMID:20025936; http://dx.doi.org/10.1016/j.mce.2009.12.011
- Cristancho AG, Lazar MA. Forming functional fat: a growing understanding of adipocyte differentiation. Nat Rev Mol Cell Biol 2011; 12:722-34; PMID:21952300; http://dx.doi.org/10.1038/nrm3198
- Lefterova MI, Haakonsson AK, Lazar MA, Mandrup S. PPARgamma and the global map of adipogenesis and beyond. Trends Endocrinol Metab 2014; 25:293-302; PMID:24793638; http://dx.doi.org/10.1016/j.tem.2014.04.001
- Yu ZK, Wright JT, Hausman GJ. Preadipocyte recruitment in stromal vascular cultures after depletion of committed preadipocytes by immunocytotoxicity. Obes Res 1997; 5:9-15; PMID:9061710; http://dx.doi.org/10.1002/j.1550-8528.1997.tb00277.x
- Lee K, Hausman GJ, Dean RG. Expression of CEBP alpha, beta and delta in fetal and postnatal subcutaneous adipose tissue. Mol Cell Biochem 1998; 178:269-74; PMID:9546609; http://dx.doi.org/10.1023/A:1006846910046
- Ding ST, McNeel RL, Mersmann HJ. Expression of porcine adipocyte transcripts: tissue distribution and differentiation in vitro and in vivo. Comp Biochem Physiol B Biochem Mol Biol 1999; 123:307-18; PMID:10481259; http://dx.doi.org/10.1016/S0305-0491(99)00077-2
- Hausman GJ. The influence of dexamethasone and insulin on expression of CCAATenhancer binding protein isoforms during preadipocyte differentiation in porcine stromal-vascular cell cultures: evidence for very early expression of CEBPalpha. J Anim Sci 2000; 78:1227-35; PMID:10834576
- Hausman GJ. Dexamethasone induced preadipocyte recruitment and expression of CCAATenhancing binding protein alpha and peroxisome proliferator activated receptor-gamma proteins in porcine stromal-vascular (S-V) cell cultures obtained before and after the onset of fetal adipogenesis. Gen Comp Endocrinol 2003; 133:61-70; PMID:12899847; http://dx.doi.org/10.1016/S0016-6480(03)00149-7
- Novakofski JE. Primary Cell Culture of Adipose Tissue. Biology of the Adipocyte: Research Approaches, Van Nostrand Reinhold Company, NY, 1987 160-97
- McNeel RL, Ding ST, Smith EO, Mersmann HJ. Expression of porcine adipocyte transcripts during differentiation in vitro and in vivo. Comp Biochem Physiol B Biochem Mol Biol 2000; 126:291-302; PMID:11007171; http://dx.doi.org/10.1016/S0305-0491(00)00185-1
- Hausman GJ, Poulos SP, Richardson RL, Barb CR, Andacht T, Kirk HC, Mynatt RL. Secreted proteins and genes in fetal and neonatal pig adipose tissue and stromal-vascular cells. J Anim Sci 2006; 84:1666-81; PMID:16775050; http://dx.doi.org/10.2527/jas.2005-539
- Hausman GJ, Barb CR, Dean RG. Gene expression profiling in developing pig adipose tissue: non-secreted regulatory proteins. Animal 2006; 5:1071-81; http://dx.doi.org/10.1017/S1751731110002727
- Zhou G, Wang S, Wang Z, Zhu X, Shu G, Liao W, Yu K, Gao P, Xi Q, Wang X, et al. Global comparison of gene expression profiles between intramuscular and subcutaneous adipocytes of neonatal landrace pig using microarray. Meat Sci 2010; 86:440-50; PMID:20573458; http://dx.doi.org/10.1016/j.meatsci.2010.05.031
- Widom RL, Rhee M, Karathanasis SK. Repression by ARP-1 sensitizes apolipoprotein AI gene responsiveness to RXR alpha and retinoic acid. Mol Cell Biol 1992; 12:3380-9; PMID:1321332
- Jonk LJ, de Jonge ME, Pals CE, Wissink S, Vervaart JM, Schoorlemmer J, Kruijer W. Cloning and expression during development of three murine members of the COUP family of nuclear orphan receptors. Mech Dev 1994; 47:81-97; PMID:7947324; http://dx.doi.org/10.1016/0925-4773(94)90098-1
- van der Wees J, Matharu PJ, de Roos K, Destree OH, Godsave SF, Durston AJ, Sweeney GE. Developmental expression and differential regulation by retinoic acid of Xenopus COUP-TF-A and COUP-TF-B. Mech Dev 1996; 54:173-84; PMID:8652410; http://dx.doi.org/10.1016/0925-4773(95)00471-8
- Brodie AE, Manning VA, Hu CY. Inhibitors of preadipocyte differentiation induce COUP-TF binding to a PPARRXR binding sequence. Biochem Biophys Res Commun 1996; 228:655-61; PMID:8941335; http://dx.doi.org/10.1006/bbrc.1996.1713
- Xu Z, Yu S, Hsu CH, Eguchi J, Rosen ED. The orphan nuclear receptor chicken ovalbumin upstream promoter-transcription factor II is a critical regulator of adipogenesis. Proc Natl Acad Sci U S A 2008; 105:2421-6; PMID:18250317; http://dx.doi.org/10.1073/pnas.0707082105
- Li L, Xie X, Qin J, Jeha GS, Saha PK, Yan J, Haueter CM, Chan L, Tsai SY, Tsai MJ. The nuclear orphan receptor COUP-TFII plays an essential role in adipogenesis, glucose homeostasis, and energy metabolism. Cell Metab 2009; 9:77-87; PMID:19117548; http://dx.doi.org/10.1016/j.cmet.2008.12.002
- Ramsay TG, Caperna TJ. Ontogeny of adipokine expression in neonatal pig adipose tissue. Comp Biochem Physiol B Biochem Mol Biol 2009; 152:72-8; PMID:18930835; http://dx.doi.org/10.1016/j.cbpb.2008.09.088
- Ramsay TG, Stoll MJ, Caperna TJ. Adipokine gene transcription level in adipose tissue of runt piglets. Comp Biochem Physiol B Biochem Mol Biol 2010; 155:97-105; PMID:19788925; http://dx.doi.org/10.1016/j.cbpb.2009.09.006
- Zhan ZP, Huang FR, Luo J, Dai JJ, Yan XH, Peng J. Duration of feeding linseed diet influences expression of inflammation-related genes and growth performance of growing-finishing barrows. J Anim Sci 2009; 87:603-11; PMID:18765842; http://dx.doi.org/10.2527/jas.2007-0177
- Qi R, Yang F, Huang J, Peng H, Liu Y, Liu Z. Supplementation with conjugated linoeic acid decreases pig back fat deposition by inducing adipocyte apoptosis. BMC Vet Res 2014; 10:141; PMID:24969229; http://dx.doi.org/10.1186/1746-6148-10-141
- Cirera S, Jensen MS, Elbrond VS, Moesgaard SG, Christoffersen BO, Kadarmideen HN, Skovgaard K, Bruun CV, Karlskov-Mortensen P, Jørgensen CB, et al. Expression studies of six human obesity-related genes in seven tissues from divergent pig breeds. Anim Genet 2013; 45:59-66; PMID:24033492; http://dx.doi.org/10.1111/age.12082
- Gondret F, Guevel B, Com E, Vincent A, Lebret B. A comparison of subcutaneous adipose tissue proteomes in juvenile piglets with a contrasted adiposity underscored similarities with human obesity. J Proteomics 2011; 75:949-61; PMID:22061664; http://dx.doi.org/10.1016/j.jprot.2011.10.012
- Gondret F, Perruchot MH, Tacher S, Berard J, Bee G. Differential gene expressions in subcutaneous adipose tissue pointed to a delayed adipocytic differentiation in small pig fetuses compared to their heavier siblings. Differentiation 2012; 81:253-60; http://dx.doi.org/10.1016/j.diff.2011.02.002
- Sauerwein H, Bendixen E, Restelli L, Ceciliani F. The adipose tissue in farm animals: a proteomic approach. Curr Protein Pept Sci 2014; 15:146-55; PMID:24555890; http://dx.doi.org/10.2174/1389203715666140221123105
- Ajuwon KM, Spurlock ME. Adiponectin inhibits LPS-induced NF-kappaB activation and IL-6 production and increases PPARgamma2 expression in adipocytes. Am J Physiol Regul Integr Comp Physiol 2005; 288:R1220-5; PMID:15604306; http://dx.doi.org/10.1152/ajpregu.00397.2004
- Hugo ER, Brandebourg TD, Comstock CE, Gersin KS, Sussman JJ, Ben-Jonathan N. LS14: a novel human adipocyte cell line that produces prolactin. Endocrinology 2006; 147:306-13; PMID:16195405; http://dx.doi.org/10.1210/en.2005-0989
- Antunes TT, Gagnon A, Chen B, Pacini F, Smith TJ, Sorisky A. Interleukin-6 release from human abdominal adipose cells is regulated by thyroid-stimulating hormone: effect of adipocyte differentiation and anatomic depot. Am J Physiol Endocrinol Metab 2006; 290:E1140-4; PMID:16682487; http://dx.doi.org/10.1152/ajpendo.00516.2005
- Harkins JM, Moustaid-Moussa N, Chung YJ, Penner KM, Pestka JJ, North CM, Claycombe KJ. Expression of interleukin-6 is greater in preadipocytes than in adipocytes of 3T3-L1 cells and C57BL6J and obob mice. J Nutr 2004; 134:2673-7; PMID:15465765
- Fried SK, Bunkin DA, Greenberg AS. Omental and subcutaneous adipose tissues of obese subjects release interleukin-6: depot difference and regulation by glucocorticoid. J Clin Endocrinol Metab 1998; 83:847-50; PMID:9506738
- Fain JN. Release of interleukins and other inflammatory cytokines by human adipose tissue is enhanced in obesity and primarily due to the nonfat cells. Vitam Horm 2006; 74:443-77; PMID:17027526; http://dx.doi.org/10.1016/S0083-6729(06)74018-3
- Li H, Chen X, Guan L, Qi Q, Shu G, Jiang Q, Yuan L, Xi Q, Zhang Y. MiRNA-181a regulates adipogenesis by targeting tumor necrosis factor-alpha (TNF-alpha) in the porcine model. PloS One 2013; 8:e71568; PMID:24098322; http://dx.doi.org/10.1371/journal.pone.0071568
- Kozak LP, Jensen JT. Genetic and developmental control of multiple forms of L-glycerol 3-phosphate dehydrogenase. J Biol Chem 1974; 249:7775-81; PMID:4430673
- Wise LS, Green H. Participation of one isozyme of cytosolic glycerophosphate dehydrogenase in the adipose conversion of 3T3 cells. J Biol Chem 1979; 254:273-5; PMID:762059
- Bradford MM. A rapid and sensitive method for the quantitation of microgram quantities of protein utilizing the principle of protein-dye binding. Anal Biochem 1976; 72:248-54; PMID:942051; http://dx.doi.org/10.1016/0003-2697(76)90527-3
- Pfaffl MW. The ongoing evolution of qPCR. Methods 2010; 50:215-6; PMID: 20215019; http://dx.doi.org/10.1016/j.ymeth.2010.02.005